- Plant Molecular Biology and Genetics Laboratory, Institute of Biology, College of Science, University of the Philippines Diliman, Quezon City, Philippines
Introduction
Cyanobacteria are known for their wide distribution, even in extreme environmental conditions, primarily due to their ability to produce secondary metabolites or bioactive compounds specialized for survival. These autotrophic Gram-negative bacteria have been studied for the discovery of novel antimicrobial compounds such as terpenes, alkaloids, phenols, carbohydrates, polyketides, and peptides (Micallef et al., 2015b; Srivastava et al., 2022).
Recent advancements in genome sequencing have enabled screening of genes responsible for encoding a novel natural compound with biological significance through genome mining. This was applied previously in some species from subsection V cyanobacteria where a broad range of impressive bioactive compounds were synthesized by giant non-ribosomal peptide synthetases (NRPS), smaller ribosomally-synthesized and post-translationally modified peptides (RiPPs), polyketides synthases (PKS), terpenes, alkaloids, fatty acids and UV-absorbing compounds (Micallef et al., 2015a; 2015b). These cyclopeptides can be of ribosomal or non-ribosomal origin. Among all, the cyclic lipopolypeptides (CLPs) have the reported widest range of bioactivities (Saurav et al., 2022). They possess a peptidic backbone, a protein or non-protein amino acids attached to a fatty acid tail that forms a ring structure (Götze and Stallforth, 2020), which promote integration into the membrane of target microbes (Fiedler and Heerklotz, 2015).
Most of the cyanobacterial BGCs are non-ribosomal peptides (NRPs), polyketides (PKs), or hybrid peptide-polyketide. NRPs in cyanobacteria are synthesized by multidomain mega-enzymes NRPS assembled outside of ribosomal translation. Their complex and diverse molecular scaffolds contribute to their biotherapeutic potentials as antibacterial, antiviral, antiprotozoal and antitumor agents. On the other hand, the RiPPs superfamily has rapidly increased its genomic sequence data of natural products from the linear chains of precursor peptides, consisting of leader peptides and core peptides produced by ribosomes (Do and Link, 2023; Ongpipattanakul et al., 2022). RiPPs have very high antimicrobial potential since they target bacterial cell wall especially those of Gram-negative bacteria that eventually leads to cell death (Cao et al., 2021). Polyketides are synthesized in a Claissen-type acyl condensation pathway by the NRPS or PKS as a substrate (Entfellner et al., 2022; Fischbach and Walsh, 2006).
Furthermore, the hybrid NRPS-PKS are also prolific cyanobacterial products. Some of these BGCs have been identified from multiple genomes across numerous genera and species. Among these include microcystin from Microcystis, Anabaena, Fischerella and Planktothrix; nodularin from Nodularia; cylindrospermopsin from Cylindrospermopsis and Oscillatoria; curacin and barbamide from Lyngbya and Moorea; anabaenopeptilides from Anabaena sp.; anabaenopeptins from Microcystis, Anabaena, Planktothrix and Nodularia (Micallef et al., 2015b). Lanthipeptides are RiPPs abundantly found in cyanobacteria that exhibit high antimicrobial potential attributed to its thioether amino acids lanthionine and methyllanthionine (Fu et al., 2023; Tang and van der Donk, 2012)
Chlorogloeopsis is a member of Nostocales with varying morphology reportedly influenced by the culture condition. For example, the Chlorogloeopsis sp. ULAP02 analyzed in this study is similar to the morphology described for culture grown under photoautotrophic conditions with cells dividing up to three planes (Evans et al., 1976). Meanwhile, limited genomic data and studies on bioactive metabolites are available for this cyanobacterium. To date, there are only three uploaded whole genome sequences of Chlorogloeopsis sp. at the National Center for Biotechnology Information (NCBI) isolated from soil, non-acidic hot spring and thermal spring. The strain used in the present study was isolated from copper mine tailings. This study aims to construct and characterize the whole genome sequence of Chlorogloeopsis sp. ULAP02 isolated from a small-scale private mining site in Itogon, Benguet, Philippines. Various genome mining tools were employed to identify BGCs with associated antimicrobial properties. These results can add to the existing genomic database information about another freshwater cyanobacterium which we previously isolated from a copper mine tailing environment. Furthermore, genome mining leads to more productive cultivation techniques and a faster discovery approach of secondary metabolites in general.
Value of data
This study presents an assembled genome of a unicellular Chlorogloeopsis sp. ULAP02 isolated from a stressful condition in a mining environment. Results of the phylogenetic analysis showed a cluster separate from the common Chlorogloeopsis fritschii, which might indicate a different species or a novel species. Furthermore, this paper lists BGCs with known antimicrobial properties mined from the generated genome. Results of this study may serve as reference for research on the organism’s genome and provides significant insights on the synthesis and potential biotherapeutic applications of these bioactive compounds.
Methods
Isolation, culture maintenance, and morphological characterization
Chlorogloeopsis sp. ULAP02 was isolated from tailings samples collected from a small-scale, private copper mining site at Mt. Ulap in Ampucao, Itogon, Benguet, Philippines (16.2505°N, 120.6775°E). Environmental samples obtained were processed in the Plant Molecular Biology and Genetics Laboratory (PMBGL) of the Institute of Biology, College of Science, University of the Philippines, Diliman, Quezon City. The isolation was performed using classical microbiological techniques in both solid and liquid BG-11 media amended with Kanamycin and Nystatin at a concentration of 10 μg/mL and 100 μg/mL, respectively. All cultures were maintained in the same laboratory and exposed to white fluorescent lamps for a 12-h light-dark cycle.
The morphology of Chlorogloeopsis sp. ULAP02 isolates was examined using a light compound microscope (Labomed LB-221, United States). The observed morphological characters such as cell size, shape, presence of sheath and color were compared to the descriptions from the literature (Anagnostidis and Komarek, 2005). The photomicrographs were taken with the aid of ScopeImage 9.0.
Genomic DNA extraction, initial identification, and whole genome sequencing
Genomic DNA of the unialgal culture was extracted using the Zymo Research Quick DNA Fungal/Bacterial Miniprep Kit (Irvine, CA, United States), following the manufacturer’s instructions. The quality (A260/280) and quantity of the extracted DNA were assessed using a spectrophotometer (Epoch™) and visualized on 1% agarose gel. Initial molecular identification was performed by amplifying and sequencing the 16S rRNA gene region following the protocol of Nübel, 1997). Upon confirmation, the genomic DNA was sent to Macrogen Inc., South Korea for whole genome shotgun sequencing (2 × 150 Paired-End) on the Illumina NovaSeq platform.
Phylogenetic analysis
The 16s rRNA sequence of the isolate was aligned with available sequences of Chlorogloeopsis (Chlorogloeopsis sp.) (7) and C. fritschii (10)] and other closely-related cyanobacteria genera [Anabaena (2), Calothrix (1), Cylindrospermopsis (1), Cylindrospermum (1), Desmonostoc (2), Fischerella (1), Halotia (2), Hapalosiphon (1), Nodularia (1), Nostoc (2), Scytonema (1), Tolypothrix (1)] for phylogenetic analysis in MEGA X: Molecular Evolutionary Genetics Analysis (Kumar et al., 2018) using MUSCLE. Maximum likelihood (ML) tree was generated using Kimura-2-Parameter Model (+G + I), as suggested by the model testing in the same software. Partial 16S rRNA sequence of Gloeobacter violaceus was used as the outgroup.
Genome assembly, taxonomic classification, and functional analysis
The quality of the paired-end reads was assessed using FastQC v0.12.1 (Andrews, 2010) and preprocessed using BBDuk v38.22 (Bushnell, 2014) to remove adapters and trim low-quality reads. Additionally, PRINSEQ v0.20.4 (Schmieder and Edwards, 2011) was employed to filter out low-complexity reads.
De novo genome assembly was conducted using SPAdes v3.15.3 on the KBase web platform (https://kbase.us) (Nurk et al., 2017). The process utilized multiple k-mer sizes (21, 33, 55, 77, 99, and 127) to optimize the quality and accuracy of assembly. The assembled genome was evaluated for completeness and contamination using CheckM v1.0.18 (Parks et al., 2015), a genome quality assessment tool based on lineage-specific marker genes.
The Genome Taxonomy Database Tool Kit (GTDB-Tk) v1.7.0 (Chaumeil et al., 2022) was used for the taxonomic classification of the assembled cyanobacterial genome. Functional annotation was then performed using the RASTtk v1.073 (Overbeek et al., 2005; Brettin et al., 2015) annotation pipeline, which incorporates a variety of computational methods to predict coding sequences, assign functions, and annotate genomic features.
Genome mining of biosynthetic gene clusters (BGCs)
The genome of Chlorogloeopsis sp. ULAP02 as well as the RefSeq genomes of the three Chlorogloeopsis species available in NCBI [GCF_015272425.1, GCF_000317265.1, GCF_000317285.1] were subjected to different genome mining tools to identify and explore BGCs comprehensively.
The webtools antiSMASH 6.0 (Blin et al., 2019) and PRISM4 v4.4.5 (Skinnider et al., 2017) were used to predict gene clusters associated with secondary metabolite synthesis. Meanwhile, ketosynthase (KS) domains and condensation (C) domains were identified using the online server NaPDoS2 (Klau et al., 2022). RiPPMiner (Agrawal et al., 2017), a machine learning webserver, was also used to determine Ribosomally synthesized Post translationally modified Peptides (RiPPs). Finally, DeepBGC v0.1.29 (Hannigan et al., 2019) was employed to detect BGCs that may encode novel biological functions. Default parameters were applied to all the tools unless otherwise stated.
Results and discussion
Morphology of Chlorogloeopsis sp. ULAP02
The Chlorogloeopsis sp. grown in BG-11 medium appeared as deep blue-green cells that do not form mats (Figure 1A). Microscopic examination revealed a unicellular cyanobacterium that tends to form clusters (Figure 1B). Each cell is enclosed in a conspicuous colorless sheath with a diameter ranging from 17 to 21 μm (Figure 1C). Cells divide at right angles forming equal halves (Figure 1D) or irregularly in 1–3 planes (Figure 1E).
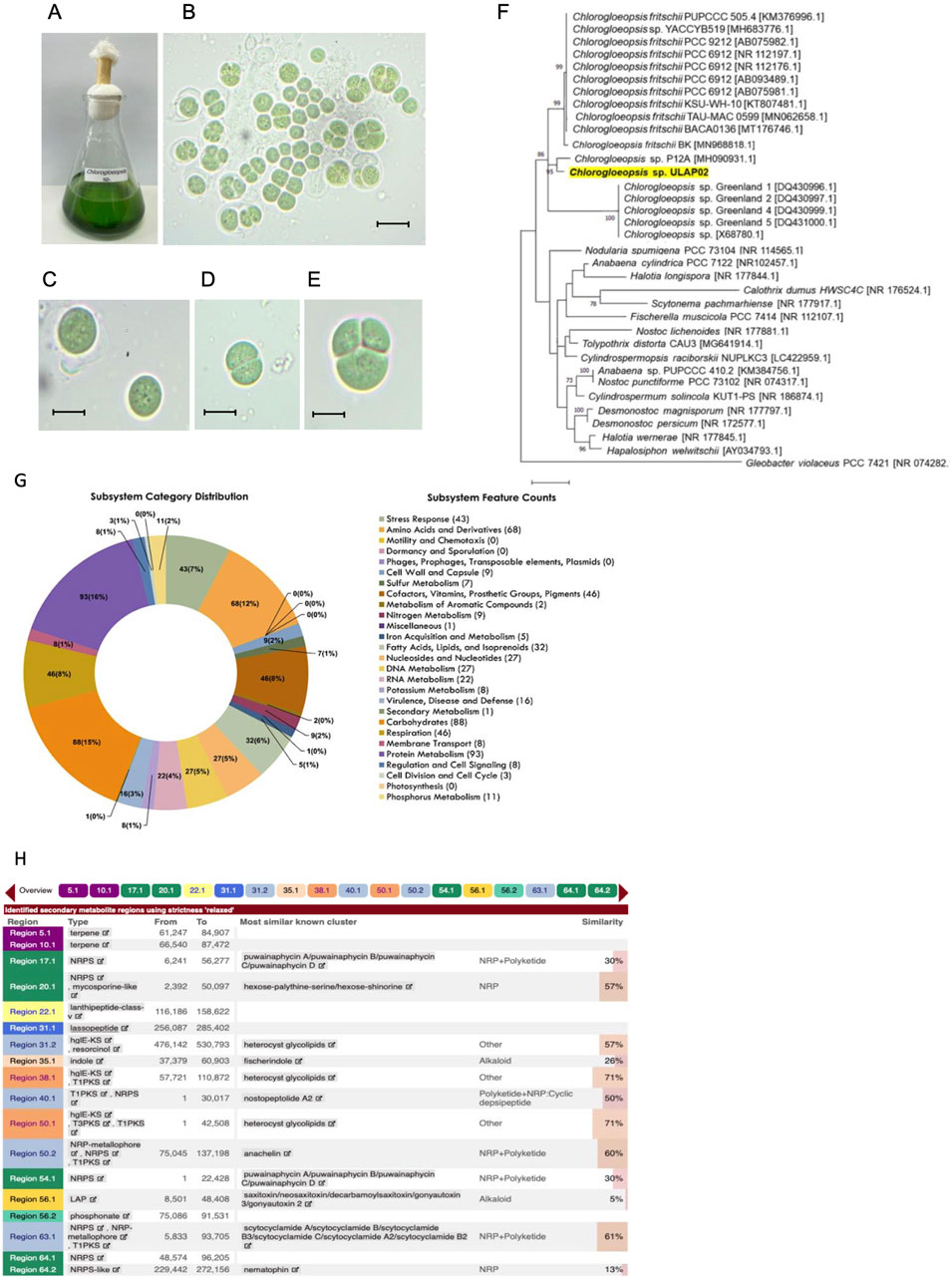
Figure 1. Morphological, molecular characterization and genome mining of biosynthetic gene clusters (BGCs) in Chlorogloeopsis sp. ULAP02. (A) Blue-green isolates in BG-11 medium. (B) Clusters of unicellular cells. (C) Single cell with a diameter of 17–21 μm. (D) Cell division in one plane at right angle producing two cells. (E) Cell division in irregular planes producing three cells of varying sizes. Microscopic figures were observed at 100X with a scale= 25 μm. (F) The Maximum Likelihood (ML) phylogenetic tree of Chlorogloeopsis sp. ULAP02 (highlighted in yellow) was generated using 16S rRNA gene sequencing based on Kimura-2-Parameter model (+G+I) generated by MEGA X. The local isolate Chlorogloeopsis sp. ULAP02 had a high bootstrap value of 86 that clustered with other Chlorogloeopsis sp. (G) The pie chart represents the cellular subsystem category and the number of protein-coding genes (in parentheses) that are predicted to be involved in the cellular process using the RASTtk. (H) Putative BGCs associated with antimicrobial metabolites, of which, heterocyst glycolipids, NRP+Polyketide and NRP are the most abundant.
Phylogenetic analysis
The partial 16S rRNA gene sequence of the local isolate Chlorogloeopsis sp. ULAP02 had a high percentage identity (96.12%) with the available Chlorogloeopsis sequences in NCBI. Furthermore, the local isolate clustered with other Chlorogloeopsis sp. with a high bootstrap value of 86 (Figure 1F), supporting the morphological identification. A separate cluster was formed by C. fritschii. Hence, the local isolate was not fully described up to the species level since it may belong to another species or a novel species yet to be identified.
Genome assembly, taxonomic classification, and functional analysis
The whole genome sequencing using the Illumina NovaSeq platform generated 23,723,010 reads. After preprocessing, de novo assembly, and binning, 68 contigs were recovered. The cyanobacterial genome has an estimated size of 7,738,087 bp and a GC content of 40.90%, which is comparable to other Chlorogloeopsis genomes in NCBI as shown in Table 1.
The results suggest that the isolate Chlorogloeopsis sp. ULAP02 belongs to the phylum Cyanobacteria based on taxonomic lineage obtained from the clade-specific marker gene sets. The completeness of the assembled genome was validated using CheckM tool giving a precise 99.76% completeness with only 1.04% contamination. Based on 68 genomes, 520 gene markers, and 415 marker sets, 510 single-copy genes were identified. There was a single orphan gene cluster and 9 heterogeneous genes. All 520 genes were grouped into a single bin.
The isolated strain Chlorogloeopsis sp. ULAP02 generated by GTDB-tk was predicted to belong to Phylum Cyanobacteriota, Class Cyanophyceae, Order Nostocales, Family Chlorogloeopsidaceae, and Genus Chlorogloeopsis.
RASTtk annotations were grouped according to their likelihood to be involved in a cellular process or subsystem (Figure 1G). Following the pie chart counterclockwise, subsystem categories are listed in the legend from top to bottom. Among all the cellular subsystem components, the (first) Protein Metabolism, (second) Carbohydrates, and (third) Amino Acids and Derivatives were the biggest groups, with 93, 88, and 68 annotated protein-coding genes, respectively.
Prediction and putative identification of BGCs
Genome mining reveals high diversity of BGCs in Chlorogloeopsis sp. ULAP02, which is consistent with the C. fritschii found in the NCBI database. Chlorogloeopsis sp. ULAP02 was isolated from a copper mine tailing environment while the other C. fritschii were also isolated from freshwater sources except for strain PCC 6912, which was isolated in a soil habitat in India (Table 1). NRPs, PKS, RiPP lanthipeptides and lasso peptides are the major secondary metabolites of Chlorogloepsis sp. ULAP02 putatively identified in all mining tools namely: antiSMASH, DeepBGC, PRISM4, RiPPMiner and NaPDoS2, with the latter providing the greatest number of secondary metabolites. There are eight identified gene clusters common to antiSMASH, DeepBGC and PRISM4 namely: terpene, NRP, NRPS-like, resorcinol, TiPKS, T3PKS, phosphonate and lasso peptide. The latter is the only cluster predicted in RiPPMiner. Lasso peptide is a class of RiPPs with a unique lariat-like structure responsible for heat and chemical resistance, antimicrobial properties and resistance to protease degradation (Meyer et al., 2023; Zeng et al., 2022). In comparison with the Chlorogloeopsis fristchii found in the NCBI database (Table 1), lasso peptide was not detected in strains PCC9212 and PCC 6912 using the 454-sequencing platform. Whereas, trans-AT-PKS-like, Class II lantipeptide, difficidin, and anabaenopeptilide were not detected in an Illumina platform. Perhaps the more sophisticated Illumina Novaseq6000 compared to Illumina Hi-seq was able to sequence the linear azol (in)e- containing peptide, RiPP, resorcinol, Shewanella PUFA, nosperin, macrolactin, Eschericoli FAS, tubulysin and viamycin at 100x genome coverage.
Figure 1H summarizes the putatively identified secondary metabolites, type of BGCs, most similar known cluster, and the percent similarity to the region in the genome of Chlorogloepsis sp. ULAP02 using antiSMASH. A total of 18 BGCs were identified and characterized as terpene, NRPS, NRP-metallophore, mycosporine-like, Lanthipeptide Class V, lasso peptide, hgIE-KS, Resorcinol, Indole, T1PKS, T3PKS, LAP, and phosphonate (Supplementary Figures S1–S18). Most of the BGCs identified were assigned to hybrid NRPS-PKS, which have been found to be structurally and functionally diverse across cyanobacteria (Kudo et al., 2023). The hits of the database search were found to be BGC of puwainaphycin A, B, C and D; nostopeptolide A2, anachelin and scytoclamide A, A2, B, B2, B3 and C, with the latter having the highest 61% similarity to known clusters. Whereas the lowest percentage similarity to alkaloids is fischerindole and saxitoxin at 26% and 5%, respectively.
Scytoclamide is a hybrid PK-NRP isolated from Scytonema hoffmanni PCC 7110. It is a laxaphycin with a unique macrocyclic lipopeptides acting synergistically to show antiproliferative and antifungal activities. They are CLPs with macrocycles made up of either 11 amino acids (type A Laxaphycin) or 12 amino acids (type B laxaphycin) (Heinilä et al., 2020). Scytoclamide might have been present in Chlorogloeopsis sp. UPAL02 since it also possesses a hydrophobic alkaloid scytonemin that are usually observed among closely related species where Scytonema and Chlorogloeopsis belong to order Nostocales.
Puwainaphycins are widespread hybrid PK-NRP in cyanobacteria. They are CLPs consisting of a β-fatty amino acid and a 10-membered peptide ring. Puwainaphycin A, B, C and D were isolated from the soil bacterium Cylindrospermum alatosporum CCALA 988 (Mareš et al., 2014). They are homologous CLPs with high antifungal and cytotoxic activities (Saurav et al., 2022).
Another BGC mined with 60% similarity to the hybrid PK-NRP is anachelin from the first genuine siderophore of Anabaena cylindrica PCC 722 (Hoffmann et al., 2003). It also has a distinct structure being an alkaloid bound to a polyketide through a tripeptide of l-Thr, d-Ser and l-Ser. Anachelin H is the most common form consisting of a terminal salicylamide in its polyketide (Årstøl and Hohmann-Marriott, 2019). Being an iron-metal chelator, it has a diverse medical applications as antimalarial, antimicrobial, or anticancer drugs (Passari et al., 2023). The presence of anachelin siderophore in Chlorogloeopsis sp. ULAP02 might be due to its coping mechanism under iron limitation while it is living in a copper mine tailing.
Nostopeptolide A2, on the other hand, is a hybrid PK-NRP cyclic depsipeptide from a cryptophycin-producing terrestrial Nostoc sp. GSV22. Both nostopeptolides A1 and A2 possess a butyric group, and nine amino acid residues and an acetate-derived unit linked by ester and peptide bonds (Hoffmann et al., 2003). Its capacity to inhibit hepatoxin-induced apoptosis is a probable indication of its potential as potent antitoxin (Fidor et al., 2019). Nostopeptolide in Chlorogloeopsis sp. ULAP02 might be express in its free-living state and is able to repress hormogonia formation.
BGCs assigned to NRP include hexose-palythine-serine/hexose-shinorine at 57% and nematophin at 13% from Heteroscytonema crispum UCFS10 and Xenorhabdus netophila ATCC 19061, respectively.
Analysis in the NaPDoS2 predicted 14 CDSs related to metabolite production in ketosynthase (KS) domain pathways and 27 CDSs related to metabolite production in condensation (C) domain pathway.
The PRISM4 analysis identified three distinct gene clusters prochlorosin, maleimide and indolocarbazole. Prochlorosin is a lanthipeptide produced by an oligotrophic Prochlorococcus. They are unicellular organisms, similar to Chlorogloeopsis sp. ULAP02, that live in extremely diluted concentrations and were found to contain natural products as antimicrobial defenses (Tang and van der Donk, 2012). Indolocarbazole metabolite was extracted from Nostoc sphaericum EX-5-1 that showed an antiviral activity against type 2 Herpes-simplex virus (Sánchez et al., 2006).
Conclusion
This study sequenced and assembled the genome of the freshwater Chlorogloeopsis sp. ULAP02 isolated from a copper mine tailing site. The genome size of 7,738,087 bp and GC content of 40.90% are comparable to other Chlorogloeopsis genomes in NCBI. This is the fourth genome assembly for the family Chlorogloeopsidaceae, where the two assembled data of C. fritschii were isolated from Germany and another C. fritschii from Chile. This is the first assembled genome of a Chlorogloeopsis strain isolated from the tropical region that could serve as a potential source of biosynthetic gene clusters for scytonamide, puwainaphycins, nostopeptolide and prochlorosin among others that possess a wide array of antimicrobial properties. Further experimentation and product extraction is suggested for the actual characterization and chemical structure of the putative in silico substances revealed through genome mining.
Data availability statements
The original contributions presented in the study are publicly available. This data can be found here: https://www.ncbi.nlm.nih.gov/genbank/, accession number SAMN38792795.
Author contributions
LS: Conceptualization, Data curation, Formal Analysis, Investigation, Methodology, Resources, Software, Supervision, Validation, Visualization, Writing–original draft, Writing–review and editing. DU: Data curation, Investigation, Methodology, Software, Validation, Visualization, Writing–original draft, Writing–review and editing, Formal Analysis. MT: Investigation, Methodology, Validation, Visualization, Writing–original draft, Writing–review and editing, Data curation, Software. EC: Formal Analysis, Investigation, Methodology, Validation, Visualization, Writing–original draft, Writing–review and editing, Conceptualization, Funding acquisition, Project administration, Resources, Supervision.
Funding
The author(s) declare that financial support was received for the research, authorship, and/or publication of this article. This study was funded by a grant from the University of the Philippines Office of the Vice-President for Academic Affairs (UP OVPAA) through the Emerging Interdisciplinary Research (EIDR) Program (OVPAA-EIDR-C09-08).
Acknowledgments
The authors would like to thank the University of the Philippines Office of the Vice-President for Academic Affairs (UP OVPAA) for the funding under the Emerging Inter-Disciplinary Research Program (EIDR-C09-08) and the Department of Science and Technology–Advance Science and Technology–Computing and Archiving Research Environment (DOST-ASTI-COARE) for allowing access to their High-Performance Computing (HPC) services.
Conflict of interest
The authors declare that the research was conducted in the absence of any commercial or financial relationships that could be construed as a potential conflict of interest.
Publisher’s note
All claims expressed in this article are solely those of the authors and do not necessarily represent those of their affiliated organizations, or those of the publisher, the editors and the reviewers. Any product that may be evaluated in this article, or claim that may be made by its manufacturer, is not guaranteed or endorsed by the publisher.
Supplementary material
The Supplementary Material for this article can be found online at: https://www.frontiersin.org/articles/10.3389/fgene.2024.1422274/full#supplementary-material
References
Agrawal, P., Khater, S., Gupta, M., Sain, N., and Mohanty, D. (2017). RiPPMiner: a bioinformatics resource for deciphering chemical structures of RiPPs based on prediction of cleavage and cross-links. Nucleic Acids Res. 45 (W1), W80–W88. doi:10.1093/nar/gkx408
Anagnostidis, K., and Komarek, J. (2005). Teil 2: Oscillatoriales Süßwasserflora von Mitteleuropa. Heidelberg: Spektrum Akademischer Verlag.
Andrews, S. (2010). FastQC: a quality control tool for high throughput sequence data [Online]. Available at: http://www.bioinformatics.babraham.ac.uk/projects/fastqc/.
Årstøl, E., and Hohmann-Marriott, M. F. (2019). Cyanobacterial siderophores—physiology, structure, biosynthesis, and applications. Mar. Drugs 17, 281. doi:10.3390/md17050281
Blin, K., Shaw, S., Steinke, K., Villebro, R., Ziemert, N., Lee, S., et al. (2019). antiSMASH 5.0: updates to the secondary metabolite genome mining pipeline. Nucleic Acids Res. 47 (1), W81–87. doi:10.1093/nar/gkz310
Brettin, T., Davis, J. J., Disz, T., Edwards, R. A., Gerdes, S., Olsen, G. J., et al. (2015). RASTtk: a modular and extensible implementation of the RAST algorithm for building custom annotation pipelines and annotating batches of genomes. Sci. Rep. 5, 8365. doi:10.1038/srep08365
Bushnell, B. (2014). BBTools, DOE Joint Genome Institute. Available at: https://jgi.doe.gov/data-and-tools/software-tools/bbtools/ (Accessed March 6, 2023).
Cao, L., Do, T., and Link, A. J. (2021). Mechanisms of action of ribosomally synthesized and posttranslationally modified peptides (RiPPs). J. Ind. Microbiol. Biotechnol. 48, kuab005. doi:10.1093/jimb/kuab005
Chaumeil, P. A., Mussig, A. J., Hugenholtz, P., and Parks, D. H. (2022). GTDB-Tk v2: memory friendly classification with the genome taxonomy database. Bioinform. (Oxford, England) 38 (23), 5315–5316. doi:10.1093/bioinformatics/btac672
Do, T., and Link, A. J. (2023). Protein engineering in ribosomally synthesized and post-translationally modified peptides (RiPPs). Biochemistry 62, 201–209. doi:10.1021/acs.biochem.1c00714
Entfellner, E., Li, R., Jiang, Y., Ru, J., Blom, J., Deng, L., et al. (2022). Toxic/bioactive peptide synthesis genes rearranged by insertion sequence elements among the bloom-forming cyanobacteria Planktothrix. Front. Microbiol. 13, 901762. doi:10.3389/fmicb.2022.901762
Evans, E. H., Foulds, I., and Carr, N. G. (1976). Environmental conditions and morphological variation in the blue-green alga Chlorogloea fritschii. J. Gen. Microbiol. 92, 147–155.
Fidor, A., Konkel, R., and Mazur-Marzec, H. (2019). Bioactive peptides produced by cyanobacteria of the Genus Nostoc: a review. Mar. Drugs 17, 561. doi:10.3390/md17100561
Fiedler, S., and Heerklotz, H. (2015). Vesicle leakage reflects the target selectivity of antimicrobial lipopeptides from Bacillus subtilis. Biophys. J. 109, 2079–2089. doi:10.1016/j.bpj.2015.09.021
Fischbach, M. A., and Walsh, C. T. (2006). Assembly-line enzymology for polyketide and nonribosomal Peptide antibiotics: logic, machinery, and mechanisms. Chem. Rev. 106, 3468–3496. doi:10.1021/cr0503097
Fu, Y., Zhou, L., and Kuipers, O. P. (2023). Discovery, biosynthesis, and characterization of a lanthipeptide from Bacillus subtilis EH11 with a unique lanthionine ring pattern. Cell Rep. Phys. Sci. 4, 101524. doi:10.1016/j.xcrp.2023.101524
Götze, S., and Stallforth, P. (2020). Structure elucidation of bacterial nonribosomal lipopeptides. Org. Biomol. Chem. 18, 1710–1727. doi:10.1039/C9OB02539A
Hannigan, G. D., Prihoda, D., Palicka, A., Soukup, J., Klempir, O., and Rampula, L. (2019). A deep learning genome-mining strategy for biosynthetic gene cluster prediction. Nucleic Acids Res. 47 (18), e110. doi:10.1093/nar/gkz654
Heinilä, L. M. P., Fewer, D. P., Jokela, J. K., Wahlsten, M., Jortikka, A., and Sivonen, K. (2020). Shared PKS module in biosynthesis of synergistic laxaphycins. Front. Microbiol. 11, 578878. doi:10.3389/fmicb.2020.578878
Hoffmann, D., Hevel, J. M., Moore, R. E., and Moore, B. S. (2003). Sequence analysis and biochemical characterization of the nostopeptolide A biosynthetic gene cluster from Nostoc sp. GSV224. GSV224. Gene 311, 171–180. doi:10.1016/s0378-1119(03)00587-0
Klau, L. J., Podell, S., Creamer, K. E., Demko, A. M., Singh, H. W., Allen, E. E., et al. (2022). The Natural Product Domain Seeker version 2 (NaPDoS2) webtool relates ketosynthase phylogeny to biosynthetic function. J. Biol. Chem. 298 (10), 102480. doi:10.1016/j.jbc.2022.102480
Kudo, F., Chikuma, T., Nambu, M., Chisuga, T., Sumimoto, S., Iwasaki, A., et al. (2023). Unique initiation and termination mechanisms involved in the biosynthesis of a hybrid polyketide-nonribosomal peptide lyngbyapeptin B produced by the marine cyanobacterium moorena bouillonii. ACS Chem. Biol. 18, 875–883. doi:10.1021/acschembio.3c00011
Kumar, S., Stecher, G., Li, M., Knyaz, C., and Tamura, K. (2018). MEGA X: molecular evolutionary Genetics analysis across computing platforms. Mol. Biol. Evol. 35, 1547–1549. doi:10.1093/molbev/msy096
Mareš, J., Hájek, J., Urajová, P., Kopecký, J., and Hrouzek, P. (2014). A hybrid non-ribosomal peptide/polyketide synthetase containing fatty-acyl ligase (FAAL) synthesizes the β-amino fatty acid lipopeptides puwainaphycins in the Cyanobacterium Cylindrospermum alatosporum. PloS One 9, e111904. doi:10.1371/journal.pone.0111904
Meyer, J. L., Gunasekera, S. P., Brown, A. L., Ding, Y., Miller, S., Teplitski, M., et al. (2023). Cryptic diversity of black band disease cyanobacteria in Siderastrea siderea corals revealed by chemical ecology and comparative genome-resolved metagenomics. Mar. Drugs 21, 76. doi:10.3390/md21020076
Micallef, M. L., D’Agostino, P. M., Al-Sinawi, B., Neilan, B. A., and Moffitt, M. C. (2015a). Exploring cyanobacterial genomes for natural product biosynthesis pathways. Mar. Genomics 21, 1–12. doi:10.1016/j.margen.2014.11.009
Micallef, M. L., D’Agostino, P. M., Sharma, D., Viswanathan, R., and Moffitt, M. C. (2015b). Genome mining for natural product biosynthetic gene clusters in the Subsection V cyanobacteria. BMC Genomics 16, 669. doi:10.1186/s12864-015-1855-z
Nübel, U., Garcia-Pichel, F., and Muyzer, G. (1997). PCR primers to amplify 16S rRNA genes from cyanobacteria. Appl. Environ. Microbiol. 63, 3327–3332. doi:10.1128/AEM.63.8.3327-3332.1997
Nurk, S., Meleshko, D., Korobeynikov, A., and Pevzner, P. A. (2017). metaSPAdes: a new versatile metagenomic assembler. Genome Res. 27 (5), 824–834. doi:10.1101/gr.213959.116
Ongpipattanakul, C., Desormeaux, E. K., DiCaprio, A., van der Donk, W. A., Mitchell, D. A., and Nair, S. K. (2022). Mechanism of action of ribosomally synthesized and post-translationally modified peptides. Chem. Rev. 122, 14722–14814. doi:10.1021/acs.chemrev.2c00210
Parks, D. H., Imelfort, M., Skennerton, C. T., Hugenholtz, P., and Tyson, G. W. (2015). CheckM: assessing the quality of microbial genomes recovered from isolates, single cells, and metagenomes. Genome Res. 25 (7), 1043–1055. doi:10.1101/gr.186072.114
Passari, A. K., Ruiz-Villafán, B., Cruz-Bautista, R., Díaz-Domínguez, V., Rodríguez-Sanoja, R., and Sanchez, S. (2023). Opportunities and challenges of microbial siderophores in the medical field. Appl. Microbiol. Biotechnol. 107, 6751–6759. doi:10.1007/s00253-023-12742-7
Overbeek, R., Begley, T., Butler, R. M., Choudhuri, J. V., Chuang, H. Y., Cohoon, M., et al. (2005). The subsystems approach to genome annotation and its use in the project to annotate 1000 genomes. Nucleic Acids Res. 33 (17), 5691–5702. doi:10.1093/nar/gki866
Sánchez, C., Méndez, C., and Salas, J. A. (2006). Indolocarbazole natural products: occurrence, biosynthesis, and biological activity. Nat. Prod. Rep. 23, 1007–1045. doi:10.1039/B601930G
Saurav, K., Caso, A., Urajová, P., Hrouzek, P., Esposito, G., Delawská, K., et al. (2022). Fatty acid substitutions modulate the cytotoxicity of puwainaphycins/minutissamides isolated from the baltic sea cyanobacterium Nodularia harveyana UHCC-0300. ACS Omega 7, 11818–11828. doi:10.1021/acsomega.1c07160
Schmieder, R., and Edwards, R. (2011). Quality control and preprocessing of metagenomic datasets. Bioinform. 27 (6), 863–864. doi:10.1093/bioinformatics/btr026
Skinnider, M. A., Merwin, N. J., Johnston, C. W., and Magarvey, N. A. (2017). PRISM 3: expanded prediction of natural product chemical structures from microbial genomes. Nucleic Acids Res. 45 (W1), W49–W54. doi:10.1093/nar/gkx320
Srivastava, N., Sarethy, I. P., Jeevanandam, J., and Danquah, M. (2022). Emerging strategies for microbial screening of novel chemotherapeutics. J. Mol. Struct. 1255, 132419. doi:10.1016/j.molstruc.2022.132419
Tang, W., and van der Donk, W. A. (2012). Structural characterization of four prochlorosins: a novel class of lantipeptides produced by planktonic marine cyanobacteria. Biochemistry 51, 4271–4279. doi:10.1021/bi300255s
Keywords: Chlorogloeopsis sp., cyanobacteria, genome mining, biosynthetic gene clusters (BGCs), antimicrobial properties
Citation: Sanchez LRS, Untiveros DPM, Tengco MTT and Cao EP (2024) Genome assembly, characterization, and mining of biosynthetic gene clusters (BGCs) from Chlorogloeopsis sp. ULAP02 isolated from Mt. Ulap, Itogon, Benguet, Philippines. Front. Genet. 15:1422274. doi: 10.3389/fgene.2024.1422274
Received: 23 April 2024; Accepted: 19 August 2024;
Published: 30 August 2024.
Edited by:
Rongbin Hu, University of California, Riverside, United StatesReviewed by:
Hengqian Ren, University of Illinois at Urbana-Champaign, United StatesRima Ouchene, Sorbonne Universités, France
Copyright © 2024 Sanchez, Untiveros, Tengco and Cao. This is an open-access article distributed under the terms of the Creative Commons Attribution License (CC BY). The use, distribution or reproduction in other forums is permitted, provided the original author(s) and the copyright owner(s) are credited and that the original publication in this journal is cited, in accordance with accepted academic practice. No use, distribution or reproduction is permitted which does not comply with these terms.
*Correspondence: Libertine Rose S. Sanchez, bHNzYW5jaGV6QHVwLmVkdS5waA==