- 1Shanghai Key Laboratory of Infectious Diseases and Biosafety Emergency Response, Department of Infectious Diseases, National Medical Center for Infectious Diseases, Huashan Hospital, Shanghai Medical College, Fudan University, Shanghai, China
- 2Shanghai Sci-Tech Inno Center for Infection and Immunity, Shanghai, China
- 3Department of Biostatistics and Computational Biology, State Key Laboratory of Genetic Engineering (SKLG), School of Life Sciences, Fudan University, Shanghai, China
Background: Tumor necrosis factor receptor-associated periodic syndrome (TRAPS) is a rare autosomal dominant disorder with a low incidence in Asia. The most frequent clinical manifestations include fever, rash, myalgia, joint pain and abdominal pain. Misdiagnosis rates are high because of the clinical and genetic variability of the disease. The pathogenesis of TRAPS is complex and yet to be fully defined. Early genetic diagnosis is the key to precise treatment.
Methods: In this study, a Chinese family with suspected TRAPS were analyzed by genome-wide SNP genotyping, linkage analysis and targeted sequencing for identification of mutations in causative genes. To study the pathogenicity of the identified gene mutation, we performed a conservation analysis of the mutation site and protein structure analysis. Flow cytometry was used to detect TNFRSF1A shedding and quantitative real-time PCR were used to assess the activation of unfolded protein response (UPR) in the mutation carriers and healthy individuals.
Results: A typical TRAPS family history, with a pattern of autosomal dominant inheritance, led to the identification of a rare mutation in the TNFRSF1A gene (c.G374A [p.Cys125Tyr]) with unknown significance. The patient responded well to corticosteroids, and long-term therapy with colchicine effectively reduced the inflammatory attacks. No amyloid complications occurred during the 6-year follow-up. In silico protein analysis showed that the mutation site is highly conversed and the mutation prevents the formation of intrachain disulfide bonds in the protein. Despite a normal shedding of the TNFRSF1A protein from stimulated monocytes in the TRAPS patients with p.C125Y mutation, the expression of CHOP and the splicing of XBP1 was significantly higher than healthy controls, suggesting the presence of an activation UPR.
Conclusion: This is the first report of a Chinese family with the rare p.C125Y mutation in TNFRSF1A. The p.C125Y mutation does not result in aberrant receptor shedding, but instead is associated with an activated UPR in these TRAPS patients, which may provide new insights into the pathogenesis of this rare mutation in TRAPS.
Introduction
Tumor necrosis factor receptor-associated periodic syndrome (TRAPS, MIM: 142680) is an autosomal dominant autoinflammatory disease caused by mutations in TNFRSF1A, which is located on chromosome 12p13 (McDermott et al., 1999). This gene encodes the tumor necrosis factor receptor (TNFRSF1A), which is widely expressed in a number of hematopoietic and non-hematopoietic cells (Idriss and Naismith, 2000). TNFRSF1A consists of an N-terminal signal peptide, an extracellular domain, transmembrane domain and C-terminal cytoplasmic domain (Cudrici et al., 2020).
The first case of TRAPS was documented in Ireland in 1982. In general, European populations have a higher incidence of the disease, when compared to Asian populations (Stojanov and McDermott, 2005). To date, 183 TNFRSF1A mutations have been reported in the Infevers database (http://fmf.igh.cnrs.fr/ISSAID/infevers/), with 44 missense mutations identified as pathogenic and 62 variations considered to be potentially pathogenic. Mutations in TNFRSF1A cause protein misfolding and retention in the endoplasmic reticulum (ER), thereby activating the unfolded protein response (UPR) (Lobito et al., 2006). The UPR is triggered by three ER transmembrane sensor proteins, PRKR-like endoplasmic reticulum kinase (PERK), activating transcription factor 6 (ATF6), and inositol-requiring protein 1α, which represent three different arms, and then change the state of the ER to adapt the stress such as accumulation of misfolded proteins (Chen et al., 2023). In addition to this hypothesis of TRAPS, increased reactive oxygen species production, impaired autophagy and increased production of pro-inflammatory factors are also implicated in the pathogenesis of the disease (Lobito et al., 2006; Kimberley et al., 2007; Simon et al., 2010; Bulua et al., 2011; Bachetti et al., 2013; Jarosz-Griffiths et al., 2019).
TRAPS is characterized by periodic fever, accompanied by a variety of non-specific symptoms (Romano et al., 2022). Renal amyloidosis is the most serious long-term complication but is rare in pediatric patients (Hodgson et al., 2014; Cody et al., 2021). Physical stress or emotion, mild infections, fatigue, hormonal changes, trauma and vaccinations can trigger the symptoms but the exact cause remains unknown (Li et al., 2023). Laboratory features that are typical of TRAPS, during febrile episodes, include elevated acute phase reactants, leukocytosis and thrombocytosis (Magnotti et al., 2013). A variety of treatment options are available, which not only reduce the acute symptoms but also prevent the chronic inflammation that leads to amyloidosis.
The disease typically manifests in early childhood, with a median age of symptom onset reported to be 4.3 years (Lachmann et al., 2014). However significant variation in the age of onset is observed in patients with TRAPS, with reports of disease onset or diagnosis in adulthood (Nakamura et al., 2009; Cantarini et al., 2014; Lopalco et al., 2015; Hernández-Rodríguez et al., 2016; Youngstein et al., 2018). There are limited reports of TRAPS in Chinese populations (Stojanov et al., 2004; Zhao et al., 2020; Li et al., 2023), with only nine adult cases documented in China and a median age of onset of 3 years (range: 0.5–38.5). In this study, we reported a Chinese patient with a family history of recurrent fever and abdominal pain, and identified a rare mutation in TNFRSF1A. To our knowledge, this is the first report of this mutation in the Chinese population.
Methods
Editorial policies and ethical considerations
This study was approved by the Ethical Review Committee of Huashan Hospital, Fudan University. All enrolled patients and non-affected family members who agreed to participate in this study provided written informed consent.
Genome-wide single nucleotide polymorphism (SNP) genotyping and linkage analysis
Peripheral blood samples were collected from eight family members, denoted as II1, II2, II4, II5, II9, III2, III4 and III5 (Figure 1A), for genetic analysis. Genomic DNA was extracted using QIAGEN DNeasy Blood and Tissue kits (QIAGEN, Germany). Genome-wide genotyping was conducted using the Illumina GSA SNP chip, which includes 887,270 SNPs. The SNPs with “no-call”, allele frequency of <5% or those that did not match the parental genotype were excluded from the analysis. Subsequently, genome-wide SNP genotyping profiles were used for linkage analysis (five patients and three unaffected family members were analyzed). A total of 5,266 high-quality SNPs (one SNP per 0.5 cM) were included in the linkage analysis. Multipoint linkage analysis was conducted using MERLIN 1.1.2 software. Markers with the logarithm of odds score >1.5 were identified. Haplotypes were analyzed and visualized using the MERLIN 1.1.2 and HaploPainter software programs, respectively.
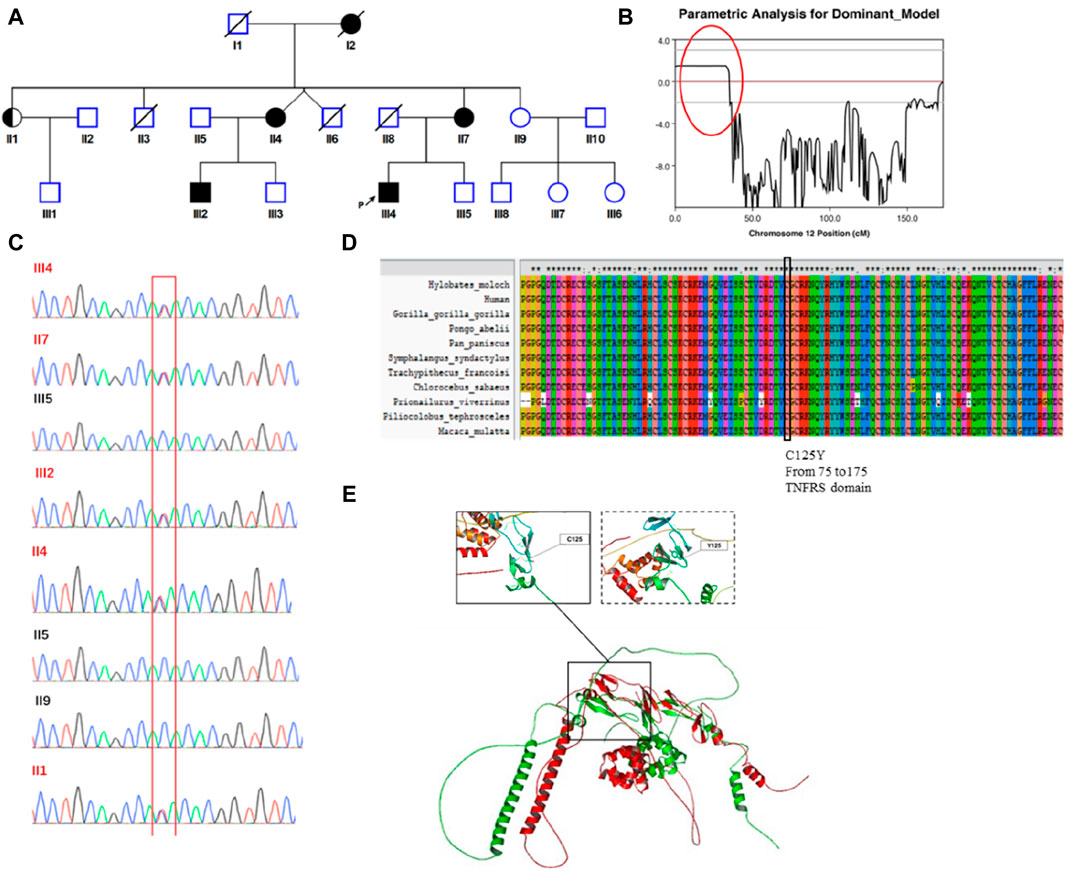
Figure 1. Identification of a germline missense mutation in TNFRSF1A. (A) Family Pedigree of the patient. The proband is indicated by a filled square with a black arrow. (B) Chromosome linkage analysis. A strongly linked segment was found on chromosome 12, marked with a red circle. (C) Sanger sequencing. The mutated TNFRSF1A gene (c.374G>A [p.Cys125Tyr]) was verified by Sanger sequencing in eight family members. (D) Conservation analysis. This mutation site was highly conserved across species. (E) Protein structure simulation. Structural alignment between the wild type protein structure and mutant protein. The left-hand box shows the normal structure surrounding the site of the wild type amino acid, with disulfide bonds present. The structure of the mutant protein is shown in the right-hand box, with no disulfide bonds.
Targeted panel sequencing and genotyping
We applied next-generation sequencing using a targeted panel of 535 genes to screen for primary immunodeficiency genes within this family. The biotinylated oligonucleotide probes specific to the exome regions of these 535 genes were designed by Roche® NimbleDesign and manufactured by SeqCap® EZ Prime Choice Probes. To minimize cost, two patients (III2 and III9) and one unaffected family member (III5) were selected for targeted panel sequencing using the Illumina Hiseq X-ten platform. The raw data (at least 1 Gb per sample/average 250 × mapping depth) were generated with a 150 bp paired-end read length. The adapter sequences and low-quality reads were filtered using trim-galore software. FastQC (http://www.bioinformatics.babraham.ac.uk/projects/fastqc/) software was used to evaluate the quality of the sequencing data. The remaining high-quality, clean reads were aligned to the human reference genome (hg19 version) using the Bowtie2 algorithm. The alignment results were sorted and marked PCR-duplicates using Picard. Only uniquely mapped reads were used for genotyping analysis. The SNPs and short insertions and deletions were identified using the GATK software. The ANNOVAR program was used for annotating mutations at both the gene and region levels. Sanger sequencing was conducted to confirm the variant of the gene using the following primers: TNFRSF1A-F: 5′- GGGAAGGAAAGGAAGTGCCA-3′, TNFRSF1A-R: 5′-AATGCCGAAAGGGTGAGTGT-3’
In silico protein analysis
To assess the level of conservation of the TNFRSF1A protein, the amino acid sequences of TNFRSF1A from humans and multiple other species were obtained from the NCBI protein database and analyzed using ClustalX v2 software (University College Dublin, Ireland). To assess the impact of the TNFRSF1A mutation on protein structure, we utilized the online AlphaFold2 website (https://colab.research.google.com/github/sokrypton/ColabFold/blob/main/AlphaFold2.ipynb) to predict the structures of both the wild-type and mutant TNFRSF1A proteins. The resulting protein structures were visualized by the Pymol software (The PyMOL Molecular Graphics System, Version 1.8.4.0.), using default parameters.
Flow cytometry analysis of TNFRSF1A
Cryopreserved peripheral blood mononuclear cells (PBMCs) from the patient and his family members were thawed and allowed to rest in RPMI 1640, supplemented with 10% fetal bovine serum, for 6 h. The PBMCs were stimulated with 20 ng/mL of phorbol 12-myristate 13-acetate (PMA; BioGems, U.S.A.) at 37°C. Cells were collected after a 10 or 60 min stimulation and washed with ice-cold PBS buffer. After incubation with Fc Receptor Blocker (Biolegend, U.S.A.) for 15 min at 4°C, the cells were stained with PE-conjugated anti-human TNFRSF1A (Clone: W15099A) and FITC-conjugated anti-human CD14 (Clone: M5E2) antibodies (Biolegend, U.S.A.) for 30 min at 4°C. Stained cells were analyzed using CytoFLEX (Beckman Coulter, U.S.A), and the data were analyzed using FlowJo v10 (BD, U.S.A).
Quantitative PCR analysis of UPR
Total RNA was extracted from peripheral blood using TRIzol reagent (Invitrogen, U.S.A.). The cDNA was synthesized using PrimeScript RT Master Mix (Takara, Japan). Quantitative real-time PCR was performed using the TB Green Premix Ex Taq II kit (Takara). The relative expression of spliced XBP1 (sXBP1, F 5′-CTGAGTCCGCAGCAGGTG-3′, R 5′-AGTTGTCCAGAATGCCCAACA -3′), CHOP (F 5′- CAGAACCAGCAGAGG TCACA-3′, R 5′- AGCTGTGCCACTTTCCTTTC-3′) and BIP (F 5′- CATCACGCCGTCCTATGTCG-3′, R 5′-CGTCAAAGACCGTGTTCTCG-3′) in the TRAPS patients was calculated using the ΔΔCt method, with GAPDH (F 5′-CTGGGCTACACTGAGCACC-3′, R 5′-AAGTGGTCGTTGAGGGCAATG-3′) serving as an internal control and unrelated individuals with wild-type TNFRSF1A serving as the control group.
Statistical analysis
Data were represented using mean and standard deviation and analyzed using unpaired t-test with the GraphPad Prism nine software (Dotmatics, USA). p < 0.05 was considered statistically significant.
Results
Clinical features of the patient
On the 15th of April, 2018, a 29-year-old Chinese patient presented with fever and abdominal pain. Three weeks earlier, he had traveled to XinJiang and developed a fever of 40°C, with thoracalgia and muscular soreness. Two weeks before admission, he had developed right lower quadrant abdominal pain. There was tenderness and rebound tenderness on physical examination. The patient had an elevated white blood cell level of 14.52 × 109/L, with 81% neutrophils, an elevated C-reactive protein level of 128.5 mg/L and an elevated IL-6 level of 9.4 pg/mL An abdominal CT scan revealed thickening of the wall of the ascending colon, accompanied by surrounding infiltration changes. The patient did not respond to potent empirical antibiotic therapy. After admission, small bowel obstruction was suspected because of dilated small bowel loops, with multiple stepwise fluid levels, on abdominal Xray. Routine stool and occult blood tests, plus a T-SPOT.TB assay, gave negative results. Screening tests for hepatitis virus, HIV and syphilis were also negative. A colonoscopy failed to reveal organic lesions in the terminal ileum and entire colorectal mucosa. There was no evidence of infection or malignant disease. The patient’s body temperature returned to normal 1 month after onset of the fever and all inflammatory markers returned to normal.
After examining the patient’s medical history in great detail, we found that he had experienced fever and abdominal pain approximately every 6 months since junior high school. He was diagnosed with appendicitis in 2003, due to significant acute lower quadrant pain. Following the appendectomy, he complained of discomfort in his abdomen and had a fever that peaked at 39°C. However, postoperative pathology showed no obvious inflammation. His fever and all other symptoms subsided after receiving empirical antibiotics for 3 days. Over the next 15 years, he experienced regular attacks of recurrent fever and abdominal pain, lasting more than 10 days and returning periodically on a semi-annual basis. Corticoids and immunosuppressive agents were never employed in the treatment of the event but non-steroidal anti-inflammatory drugs and empirical antibiotics were usually administered.
According to the patient’s family history, his mother (II 7), grandmother (I2), an aunt (II 4) and one elder male cousin (III 2) also suffered from recurrent fever (temperatures over 38°C, lasting for more than 3 days) with arthralgia or abdominal discomfort (Figure 1A). The female patients did not undergo systemic examination due to relatively mild symptoms, with inflammation that subsided spontaneously. His cousin underwent an exploratory laparotomy due to unknown “fever with abdominal pain” but ultimately no abnormalities were found.
Identification of the germline missense mutation in TNFRS1A
The patient complained of recurring fever and abdominal discomfort, with no evidence of infection or malignant disease. In addition, he had a family history that was suggestive of a hereditary condition with autosomal dominant inheritance. Using samples from eight family members, we performed a genome-wide linkage analysis and discovered a significantly linked region on chromosome 12 (Figure 1B). Subsequently, we performed targeted next-generation sequencing of 535 primary immunodeficiency causative genes. We discovered 15 genes with non-synonymous single nucleotide variations (CD46, NBAS, TGFBRAP1, C2, FGFR1, NUP214, DNLZ, ADRB1, TNFRSF1A, POLE, CIITA, STAT5B, ACE, LIG1KIR3DL3 and RTEL1), two with stop-gain mutations (ENTPD1 and BTNL2) and one with a splice site mutation (TTN). The allele frequencies of those variants are lower than 0.01 in the population databases. These databases consisted of the 1000 Genomes Project database, dbSNP, the Exome Aggregation Consortium and the Genome Aggregation Database. On chromosome 12, there were only two genes: POLE and TNFRSF1A. Based on the patient’s clinical manifestations, we excluded the POLE mutation from being the suspected cause, and located the causative mutation on the TNFRSF1A gene (c.374G>A [p.Cys125Tyr]). Family members II1, II4, II7 and III2 also carried the mutation. Sanger sequencing verified the presence of the mutation (Figure 1C). This mutation was predicted to be pathogenic by multiple in silico pathogenicity prediction tools, such as CADD (score = 32), REVEL (0.992, pathogenic), SIFT (0, deleterious), PolyPhen-2 (1.0, probably damaging) and MutationTaster (0.88, disease causing). An analysis of interspecies conservation showed that there is a high degree of conservation of the amino acid at this site in the protein (Figure 1D). We then implemented PyMol for protein structure analysis and simulated the altered protein’s molecular structure. We discovered that the original location aids in the formation of disulfide bonds in the protein and the mutation may result in the absence of this process. When we analyzed the structure of the protein, we found that mutations can result in structural anomalies (Figure 1E). Most missense variants affected cysteine residues that are involved in disulfide bond formation (Aksentijevich et al., 2001; Rebelo et al., 2006). Lineage analysis revealed that changes in cysteine residues, which interrupt normal disulfide bond formation, result in more severe clinical phenotypes (Akagi et al., 2022). Notably, all carriers experienced recurring fever, except for individual II1, who remained asymptomatic. With an 80% penetrance rate in this family, it was hypothesized that the p.C125Y mutation exhibits incomplete penetrance and variations exist in the manifestation and severity of the clinical symptoms in p.C125Y mutation carriers.
Normal shedding of TNFRSF1A from stimulated monocytes in patients with p.C125Y TNFRSF1A mutation
Proteolytic cleavage of TNFRSF1A is mediated in part by metalloproteases (Silke and Brink, 2010). Impaired surface TNFRSF1A shedding has been observed and is associated with dysregulated inflammation in TRAPS patients (McDermott et al., 1999). To assess whether the p.C125Y mutation in TNFRSF1A is associated with impaired shedding, PBMCs from the patient, family members and healthy controls were stimulated with PMA, which is a potent inducer of the metalloproteases that mediate receptor shedding (McDermott et al., 1999). The levels of TNFRSF1A in CD14+ monocytes were then determined (Figure 2A). In TRAPS patients with the p.C125Y mutation, the level of TNFRSF1A decreased in CD14+ monocytes during PMA stimulation. However, the decrease was comparable with healthy controls and the family member (III5) without the mutation (Figures 2B, C). This result indicated that normal shedding of TNFRSF1A is observed in TRAPS patients with the p.C125Y mutation.
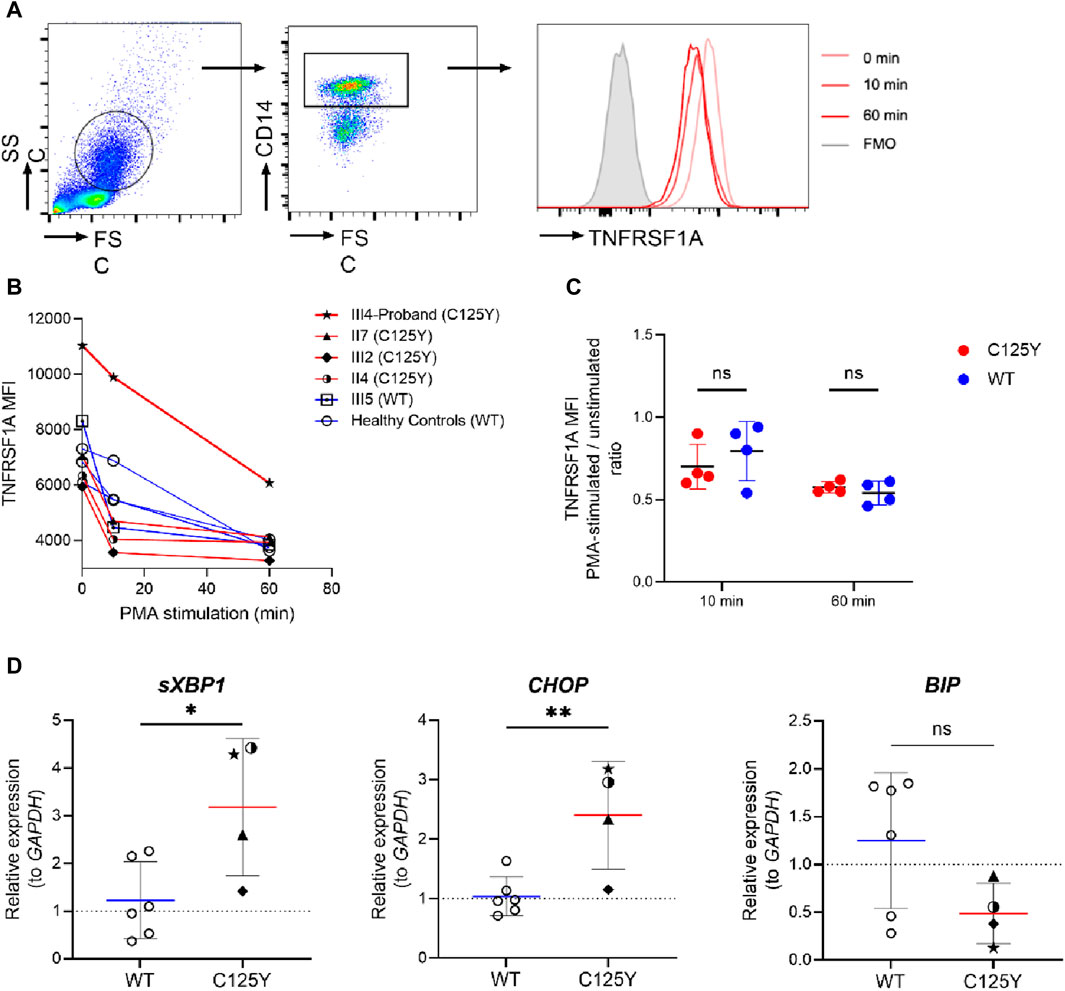
Figure 2. TNFRSF1A shedding and the activation of unfolded protein response (UPR) in patients with tumor necrosis factor receptor-associated periodic syndrome (TRAPS). (A) Gating strategy and representative histograms of TNFRSF1A level in a patient with the mutation. (B) Change in TNFRSF1A median fluorescence intensity (MFI) in peripheral CD14+ monocytes during 20 ng/mL phorbol 12-myristate 13-acetate (PMA) stimulation in patients with the mutation (red lines, n = 4), a family member with wild type (WT) TNFRSF1A (square icon, blue line, n = 1) and healthy controls (circle icon, blue line, n = 3). (C) Change in TNFRSF1A level in peripheral CD14+ monocytes after 10-min and 60-min PMA stimulation (MFI of PMA-stimulated cells/unstimulated cells ratio), in patients with the mutation (n = 4) and individuals with WT TNFRSF1A (n = 4). (D) The relative mRNA expression of spliced XBP1 (sXBP1, left), CHOP (middle) and BIP (right) in TRAPS patients with the p.C125Y mutation (n = 4) and individuals with WT TNFRSF1A (n = 6) were assessed by quantitative real-time PCR. Results were analyzed using the ΔΔCt method, with GAPDH serving as an internal control and unrelated individuals with WT TNFRSF1A serving as the control group. Data are shown as mean ± SD and compared using unpaired t-test. *, p < 0.05. **, p < 0.01.ns, not significant.
Elevated activation of UPR in patients with p.C125Y TNFRSF1A mutation
Another highly plausible pathogenesis of TRAPS involves an activated UPR triggered by accumulation of TNFRSF1A mutant in the ER (Lobito et al., 2006). Cells subsequently respond to this stress by inducing gene expression to restore proper ER function (Pahl and Baeuerle, 1997). To investigate the presence of an activated UPR, we therefore assessed the transcription of UPR-associated genes CHOP and BIP, and the splicing of XBP1 (Sicari et al., 2020; Sen Santara et al., 2023). As shown in Figure 2D, there was no significant difference in the transcript level of BIP in these TRAPS patients with the p.C125Y mutation and healthy controls. However, a significantly elevated expression of sXBP1 and CHOP was observed in TRAPS patients with the p.C125Y mutation. These results indicated a strong correlation between the p.C125Y mutation and the activation of UPR, which could subsequently induce cascades of inflammatory reactions, and therefore provided a possible explanation for the underlying mechanism of this p.C125Y mutation in TRAPS.
Follow-up of the patients
After the patient received a definitive diagnosis, methylprednisolone, at a dosage of 24 mg per day, was prescribed to alleviate the acute symptoms. In addition, the patient was prescribed colchicine to reduce the episode attacks. He took the medication intermittently for several years and he occasionally experienced an episode attack. Over a 6-year follow-up, there was no evidence of secondary renal amyloidosis. His mother, two aunts and cousin did not take colchicine because of the low frequency of their episode attacks. The disease tended to alleviate with age.
Discussion
The prominent clinical features of our case were recurrent fevers, each lasting at least 7 days, accompanied by obvious abdominal pain. These symptoms occurred once every 6 months. Inflammatory indicators increased significantly during the attacks and treatment with methylprednisolone significantly controlled the symptoms in the acute phase. Genetic testing revealed that the patient had a c.G374A (p.C125Y) mutation in exon four of the TNFRSF1A gene. This mutation has been recorded in a large case cohort and in a sporadic case report, with no family history or functional analysis (Dodé et al., 2002; Lachmann et al., 2014). This study demonstrated the process of diagnosing TRAPS in a patient with a 15-year history of recurrent fever, and a preliminary functional analysis was performed. To our knowledge, this is the first Asian case of this mutation being detected in adulthood.
There were no obvious abnormalities in the shedding of cell surface receptors, caused by the mutation, after PMA stimulation. This was consistent with previous studies by Mary L, Huggis, who observed that shedding was normal in individuals with amino acid changes such as p.C33Y (Huggins et al., 2004). The original pathogenic mechanism was thought to involve mutations that result in a receptor shedding disorder, causing a persistent inflammatory phenotype. However, shedding deficiency disorders display significant inter-patient variability (Aganna et al., 2003; Churchman et al., 2008; Greco et al., 2015).
We found the increased mRNA expression of sXBP1 and CHOP in patients. Unconventional splicing of XBP1 results from phosphorylation of inositol-requiring protein α, which activates transcription factors to be expressed (Calfon et al., 2002). CHOP is one of the important target downstream genes of the PERK arm, reflecting the activation status of this pathway (Avivar-Valderas et al., 2011). Besides, ER chaperone BiP/GRP78 can bind ATF6 and dissociate in response to ER stress (Shen et al., 2002). Our findings strongly suggest that this mutation activates inositol-requiring protein α arm and PERK arm, and it may have nothing to do with the ATF6 pathway. UPR can activate the mitogen-activated protein kinase and mitochondrial reactive oxygen species, then induce the secretion of pro-inflammatory cytokines, such as IL-1 and IL-6, to cause constitutive inflammation (Bulua et al., 2011). Our patient had elevated IL-6 levels, consistent with this possible causative mechanism. Autophagy dysfunction (Yang et al., 2015), excessive activation of NF-κb transcription factors and apoptosis suppression are also thought to play a role in the pathophysiology (Churchman et al., 2008; Greco et al., 2015). Indeed, there is a chance that these anomalies are related to one another, posing obstacles to our understanding of the relationships between genotype and phenotype. More research is needed to fully understand the functional consequences of this mutation.
TRAPS patients exhibit an extensive spectrum of clinical symptoms at onset and the disease is unquestionably genetically heterogeneous (Gaggiano et al., 2020). There is limited evidence that age or genotype significantly impact the disease characteristics at presentation (Lachmann et al., 2014). The disease is characterized by periodic fever, usually lasting 10–14 days, with a variety of non-specific symptoms such as prolonged or recurrent abdominal pain (70%), arthralgia (69%), myalgia (69%), swimming erythematous rash (60%), eye inflammation (37%) and periorbital edema (28%) (Cudrici et al., 2020). Amyloidosis, recurrent pericarditis, pleurisy, peritonitis and testicular inflammation are also possible complications. Previously, it was considered that a rash was more common among Chinese patients, when compared to European and Japanese patients, whereas chest pain was relatively uncommon (Zhao et al., 2020). However, the patient in this study had a 15-year medical history but lacked rash, which may change the distribution of clinical symptoms in the Chinese population. Recurrent abdominal pain also constitutes a clinical indication of TRAPS that requires more attention. More than one patient has been through an appendectomy and laparotomy due to signs of acute abdominal pain (Chen et al., 2014). Early diagnosis can eliminate the need for invasive procedures. For Chinese individuals, the average diagnostic delay is approximately 16.5 years and there is only an 11.1% possibility of having a positive family history (Zhao et al., 2020). Our patient had a family history and the diagnosis was established 15 years after the disease first emerged. The family history ruled out the possibility of a somatic mutation.
This pedigree had incomplete penetrance, which is an intriguing finding. Specifically, one carrier of the variant did not show any clinical features of TRAPS. There have also been reports of partial penetration with other variants in the past (Aksentijevich et al., 2001; Hull et al., 2002). The guidelines distinguish TRAPS mutations as high-penetrance or low-penetrance variants that are either pathogenic or variants of uncertain significance (Shinar et al., 2012). Luca Cantarini et al. compared the clinical manifestations and treatment responses between high-penetrance and low-penetrance variants. They suggested that low penetrance may be related to the autoinflammatory phenotype (Cantarini et al., 2014). Therefore, the phenomenon of incomplete penetrance in autosomal dominant diseases is a noteworthy and important aspect to consider.
We controlled the patient’s paroxysm with glucocorticoids, with a satisfactory outcome. Colchicine was used to prevent attacks. Corticosteroids and nonsteroidal anti-inflammatory drugs have been widely used for the control of acute symptoms (Ter Haar and Frenkel, 2014). With the expansion of disease research, biological agents are also being used in the treatment of TRAPS. These include TNF blockers (Etanercept), IL-1β receptor antagonists (Anakinra, Canakinumab) and IL-6 blockers (Tocilizumab), which are thought to be advantageous for avoiding complications (Vaitla et al., 2011; Ter Haar et al., 2013; Gentileschi et al., 2017; Kuemmerle-Deschner et al., 2020; Gattorno et al., 2024). However, IL-1β blockers were not available in China at that time. In addition, colchicine monotherapy may be tested in patients with milder phenotypes and at a lower risk of developing reactive amyloidosis (Vitale et al., 2020). The monitoring procedure had an enormous preventative impact, as evidenced by a marked decrease in strikes and the absence of amyloidosis. However, complete remission may not be possible with a single biologic treatment, since different pathophysiology may be involved in specific variations. Therefore, personalized treatment is highly recommended.
Conclusion
This is the first family with TRAPS to be identified with the p.C125Y TNFRSF1A mutation in China. The p.C125Y mutation does not result in aberrant receptor shedding, but instead is associated with an activated UPR in these TRAPS patients. This case enriches the spectrum of human gene mutations and deepens our understanding of monogenic genetic diseases. TRAPS is a rare disease and should be taken into consideration if patients demonstrate recurrent fever. Family history and genetic sequencing provide critical evidence for diagnosis. Early diagnosis, standardized treatment and effective symptom and complication management can lead to improved patient outcomes, such as an extended lifespan.
Data availability statement
The original contributions presented in the study are included in the article/Supplementary Material, further inquiries can be directed to the corresponding authors.
Ethics statement
The studies involving humans were approved by the Huashan Hospital, Fudan University. The studies were conducted in accordance with the local legislation and institutional requirements. The participants provided their written informed consent to participate in this study. Written informed consent was obtained from the individual(s) for the publication of any potentially identifiable images or data included in this article.
Author contributions
MQ: Data curation, Formal Analysis, Investigation, Writing–original draft. JZ: Data curation, Formal Analysis, Investigation, Writing–original draft. JW: Investigation, Methodology, Software, Writing–review and editing. HZ: Data curation, Formal Analysis, Writing–original draft. SY: Data curation, Formal Analysis, Writing–original draft. HX: Data curation, Formal Analysis, Writing–original draft. YY: Formal Analysis, Funding acquisition, Writing–original draft. FZ: Formal Analysis, Funding acquisition, Writing–original draft. QY: Data curation, Formal Analysis, Writing–original draft. LS: Data curation, Formal Analysis, Writing–review and editing. WZ: Project administration, Writing–review and editing. NJ: Funding acquisition, Investigation, Resources, Writing–review and editing. QR: Funding acquisition, Investigation, Resources, Writing–review and editing.
Funding
The author(s) declare that financial support was received for the research, authorship, and/or publication of this article. This work was supported by National Natural Science Foundation of China (82271794) and Clinical Research Project Supported by Huashan Hospital, Fudan University.
Acknowledgments
The authors thank all the subjects for participating in this study.
Conflict of interest
The authors declare that the research was conducted in the absence of any commercial or financial relationships that could be construed as a potential conflict of interest.
The reviewer XM declared a shared affiliation with the authors to the handling editor at the time of the review.
The reviewer SW declared a shared affiliation with the authors to the handling editor at the time of the review.
Publisher’s note
All claims expressed in this article are solely those of the authors and do not necessarily represent those of their affiliated organizations, or those of the publisher, the editors and the reviewers. Any product that may be evaluated in this article, or claim that may be made by its manufacturer, is not guaranteed or endorsed by the publisher.
Supplementary Material
The Supplementary Material for this article can be found online at: https://www.frontiersin.org/articles/10.3389/fgene.2024.1413641/full#supplementary-material
References
Aganna, E., Hammond, L., Hawkins, P. N., Aldea, A., Mckee, S. A., van Amstel, H. K., et al. (2003). Heterogeneity among patients with tumor necrosis factor receptor-associated periodic syndrome phenotypes. Arthritis Rheum. 48, 2632–2644. doi:10.1002/art.11215
Akagi, T., Hiramatsu-Asano, S., Ikeda, K., Hirano, H., Tsuji, S., Yahagi, A., et al. (2022). TRAPS mutations in Tnfrsf1a decrease the responsiveness to TNFα via reduced cell surface expression of TNFR1. Front. Immunol. 13, 926175. doi:10.3389/fimmu.2022.926175
Aksentijevich, I., Galon, J., Soares, M., Mansfield, E., Hull, K., Oh, H. H., et al. (2001). The tumor-necrosis-factor receptor-associated periodic syndrome: new mutations in TNFRSF1A, ancestral origins, genotype-phenotype studies, and evidence for further genetic heterogeneity of periodic fevers. Am. J. Hum. Genet. 69, 301–314. doi:10.1086/321976
Avivar-Valderas, A., Salas, E., Bobrovnikova-Marjon, E., Diehl, J. A., Nagi, C., Debnath, J., et al. (2011). PERK integrates autophagy and oxidative stress responses to promote survival during extracellular matrix detachment. Mol. Cell. Biol. 31, 3616–3629. doi:10.1128/MCB.05164-11
Bachetti, T., Chiesa, S., Castagnola, P., Bani, D., di Zanni, E., Omenetti, A., et al. (2013). Autophagy contributes to inflammation in patients with TNFR-associated periodic syndrome (TRAPS). Ann. Rheum. Dis. 72, 1044–1052. doi:10.1136/annrheumdis-2012-201952
Bulua, A. C., Simon, A., Maddipati, R., Pelletier, M., Park, H., Kim, K. Y., et al. (2011). Mitochondrial reactive oxygen species promote production of proinflammatory cytokines and are elevated in TNFR1-associated periodic syndrome (TRAPS). J. Exp. Med. 208, 519–533. doi:10.1084/jem.20102049
Calfon, M., Zeng, H., Urano, F., Till, J. H., Hubbard, S. R., Harding, H. P., et al. (2002). IRE1 couples endoplasmic reticulum load to secretory capacity by processing the XBP-1 mRNA. Nature 415, 92–96. doi:10.1038/415092a
Cantarini, L., Rigante, D., Merlini, G., Vitale, A., Caso, F., Lucherini, O. M., et al. (2014). The expanding spectrum of low-penetrance TNFRSF1A gene variants in adults presenting with recurrent inflammatory attacks: clinical manifestations and long-term follow-up. Semin. Arthritis Rheum. 43, 818–823. doi:10.1016/j.semarthrit.2013.12.002
Chen, Y. J., Yu, H. H., Yang, Y. H., Lau, Y. L., Lee, W. I., and Chiang, B. L. (2014). Recurrent abdominal pain as the presentation of tumor necrosis factor receptor-associated periodic syndrome (TRAPS) in an Asian girl: a case report and review of the literature. J. Microbiol. Immunol. Infect. 47, 550–554. doi:10.1016/j.jmii.2012.07.003
Chen, X., Shi, C., He, M., Xiong, S., and Xia, X. (2023). Endoplasmic reticulum stress: molecular mechanism and therapeutic targets. Signal Transduct. Target. Ther. 8, 352. doi:10.1038/s41392-023-01570-w
Churchman, S. M., Church, L. D., Savic, S., Coulthard, L. R., Hayward, B., Nedjai, B., et al. (2008). A novel TNFRSF1A splice mutation associated with increased nuclear factor kappaB (NF-kappaB) transcription factor activation in patients with tumour necrosis factor receptor associated periodic syndrome (TRAPS). Ann. Rheumatic Dis. 67, 1589–1595. doi:10.1136/ard.2007.078667
Cody, E., Ayoob, R., and Mitsnefes, M. (2021). Renal AA amyloidosis as rare presentation of tumor necrosis factor receptor-associated periodic syndrome in pediatric patient. Kidney Int. Rep. 6, 2926–2929. doi:10.1016/j.ekir.2021.07.016
Cudrici, C., Deuitch, N., and Aksentijevich, I. (2020). Revisiting TNF receptor-associated periodic syndrome (TRAPS): current perspectives. Int. J. Mol. Sci. 21, 3263. doi:10.3390/ijms21093263
Dodé, C., André, M., Bienvenu, T., Hausfater, P., PêCHEUX, C., Bienvenu, J., et al. (2002). The enlarging clinical, genetic, and population spectrum of tumor necrosis factor receptor-associated periodic syndrome. Arthritis Rheum. 46, 2181–2188. doi:10.1002/art.10429
Gaggiano, C., Vitale, A., Obici, L., Merlini, G., Soriano, A., Viapiana, O., et al. (2020). Clinical features at onset and genetic characterization of pediatric and adult patients with TNF-α receptor-associated periodic syndrome (TRAPS): a series of 80 cases from the aida network. Mediat. Inflamm. 2020, 8562485. doi:10.1155/2020/8562485
Gattorno, M., Obici, L., PenadéS, I. C., Kallinich, T., Benseler, S., Dekker, E., et al. (2024). Long-term efficacy and safety of Canakinumab in patients with tumor necrosis factor receptor-associated periodic syndrome: results from a phase III trial. Arthritis Rheumatol. 76, 304–312. doi:10.1002/art.42695
Gentileschi, S., Rigante, D., Vitale, A., Sota, J., Frediani, B., Galeazzi, M., et al. (2017). Efficacy and safety of anakinra in tumor necrosis factor receptor-associated periodic syndrome (TRAPS) complicated by severe renal failure: a report after long-term follow-up and review of the literature. Clin. Rheumatol. 36, 1687–1690. doi:10.1007/s10067-017-3688-4
Greco, E., Aita, A., Galozzi, P., Gava, A., Sfriso, P., Negm, O. H., et al. (2015). The novel S59P mutation in the TNFRSF1A gene identified in an adult onset TNF receptor associated periodic syndrome (TRAPS) constitutively activates NF-κB pathway. Arthritis Res. Ther. 17, 93. doi:10.1186/s13075-015-0604-7
HernáNDEZ-RodríGUEZ, J., RuíZ-Ortiz, E., Tomé, A., Espinosa, G., GonzáLEZ-Roca, E., Mensa-Vilaró, A., et al. (2016). Clinical and genetic characterization of the autoinflammatory diseases diagnosed in an adult reference center. Autoimmun. Rev. 15, 9–15. doi:10.1016/j.autrev.2015.08.008
Hodgson, K. A., Crawford, N. W., and Akikusa, J. D. (2014). Recurrent fevers in children: TRAPS for young players. BMJ Case Rep. 2014, bcr2013201627. doi:10.1136/bcr-2013-201627
Huggins, M. L., Radford, P. M., Mcintosh, R. S., Bainbridge, S. E., Dickinson, P., Draper-Morgan, K. A., et al. (2004). Shedding of mutant tumor necrosis factor receptor superfamily 1A associated with tumor necrosis factor receptor-associated periodic syndrome: differences between cell types. Arthritis Rheum. 50, 2651–2659. doi:10.1002/art.20380
Hull, K. M., Drewe, E., Aksentijevich, I., Singh, H. K., Wong, K., Mcdermott, E. M., et al. (2002). The TNF receptor-associated periodic syndrome (TRAPS): emerging concepts of an autoinflammatory disorder. Medicine 81, 349–368. doi:10.1097/00005792-200209000-00002
Idriss, H. T., and Naismith, J. H. (2000). TNF alpha and the TNF receptor superfamily: structure-function relationship(s). Microsc. Res. Tech. 50, 184–195. doi:10.1002/1097-0029(20000801)50:3<184::AID-JEMT2>3.0.CO;2-H
Jarosz-Griffiths, H. H., Holbrook, J., Lara-Reyna, S., and Mcdermott, M. F. (2019). TNF receptor signalling in autoinflammatory diseases. Int. Immunol. 31, 639–648. doi:10.1093/intimm/dxz024
Kimberley, F. C., Lobito, A. A., Siegel, R. M., and Screaton, G. R. (2007). Falling into TRAPS--receptor misfolding in the TNF receptor 1-associated periodic fever syndrome. Arthritis Res. Ther. 9, 217. doi:10.1186/ar2197
Kuemmerle-Deschner, J. B., Gautam, R., George, A. T., Raza, S., Lomax, K. G., and Hur, P. (2020). Systematic literature review of efficacy/effectiveness and safety of current therapies for the treatment of cryopyrin-associated periodic syndrome, hyperimmunoglobulin D syndrome and tumour necrosis factor receptor-associated periodic syndrome. RMD Open 6, e001227. doi:10.1136/rmdopen-2020-001227
Lachmann, H. J., Papa, R., Gerhold, K., Obici, L., Touitou, I., Cantarini, L., et al. (2014). The phenotype of TNF receptor-associated autoinflammatory syndrome (TRAPS) at presentation: a series of 158 cases from the Eurofever/EUROTRAPS international registry. Ann. Rheum. Dis. 73, 2160–2167. doi:10.1136/annrheumdis-2013-204184
Li, Z., Jing, X., Zhang, S., Liu, T., and Guo, Q. (2023). Tumor necrosis factor receptor-associated cycle syndrome: a case report and literature review. Front. Pediatr. 11, 1296487. doi:10.3389/fped.2023.1296487
Lobito, A. A., Kimberley, F. C., Muppidi, J. R., Komarow, H., Jackson, A. J., Hull, K. M., et al. (2006). Abnormal disulfide-linked oligomerization results in ER retention and altered signaling by TNFR1 mutants in TNFR1-associated periodic fever syndrome (TRAPS). Blood 108, 1320–1327. doi:10.1182/blood-2005-11-006783
Lopalco, G., Rigante, D., Vitale, A., Galeazzi, M., Iannone, F., and Cantarini, L. (2015). Adult-onset tumour necrosis factor receptor-associated periodic syndrome presenting with refractory chronic arthritis. Clin. Exp. Rheumatol. 33, S171–S172.
Magnotti, F., Vitale, A., Rigante, D., Lucherini, O. M., Cimaz, R., Muscari, I., et al. (2013). The most recent advances in pathophysiology and management of tumour necrosis factor receptor-associated periodic syndrome (TRAPS): personal experience and literature review. Clin. Exp. Rheumatol. 31, 141–149.
Mcdermott, M. F., Aksentijevich, I., Galon, J., Mcdermott, E. M., Ogunkolade, B. W., Centola, M., et al. (1999). Germline mutations in the extracellular domains of the 55 kDa TNF receptor, TNFR1, define a family of dominantly inherited autoinflammatory syndromes. Cell. 97, 133–144. doi:10.1016/s0092-8674(00)80721-7
Nakamura, M., Kobayashi, M., and Tokura, Y. (2009). A novel missense mutation in tumour necrosis factor receptor superfamily 1A (TNFRSF1A) gene found in tumour necrosis factor receptor-associated periodic syndrome (TRAPS) manifesting adult-onset Still disease-like skin eruptions: report of a case and review of the Japanese patients. Br. J. Dermatol 161, 968–970. doi:10.1111/j.1365-2133.2009.09409.x
Pahl, H. L., and Baeuerle, P. A. (1997). The ER-overload response: activation of NF-kappa B. Trends Biochem. Sci. 22, 63–67. doi:10.1016/s0968-0004(96)10073-6
Rebelo, S. L., Bainbridge, S. E., Amel-Kashipaz, M. R., Radford, P. M., Powell, R. J., Todd, I., et al. (2006). Modeling of tumor necrosis factor receptor superfamily 1A mutants associated with tumor necrosis factor receptor-associated periodic syndrome indicates misfolding consistent with abnormal function. Arthritis Rheum. 54, 2674–2687. doi:10.1002/art.21964
Romano, M., Arici, Z. S., Piskin, D., Alehashemi, S., Aletaha, D., Barron, K. S., et al. (2022). The 2021 EULAR/American College of Rheumatology points to consider for diagnosis, management and monitoring of the interleukin-1 mediated autoinflammatory diseases: cryopyrin-associated periodic syndromes, tumour necrosis factor receptor-associated periodic syndrome, mevalonate kinase deficiency, and deficiency of the interleukin-1 receptor antagonist. Ann. Rheum. Dis. 81, 907–921. doi:10.1136/annrheumdis-2021-221801
Sen Santara, S., Lee, D.-J., Crespo, Â., Hu, J. J., Walker, C., Ma, X., et al. (2023). The NK cell receptor NKp46 recognizes ecto-calreticulin on ER-stressed cells. Nature 616, 348–356. doi:10.1038/s41586-023-05912-0
Shen, J., Chen, X., Hendershot, L., and Prywes, R. (2002). ER stress regulation of ATF6 localization by dissociation of BiP/GRP78 binding and unmasking of Golgi localization signals. Dev. Cell. 3, 99–111. doi:10.1016/s1534-5807(02)00203-4
Shinar, Y., Obici, L., Aksentijevich, I., Bennetts, B., Austrup, F., Ceccherini, I., et al. (2012). Guidelines for the genetic diagnosis of hereditary recurrent fevers. Ann. Rheum. Dis. 71, 1599–1605. doi:10.1136/annrheumdis-2011-201271
Sicari, D., Delaunay-Moisan, A., Combettes, L., Chevet, E., and Igbaria, A. (2020). A guide to assessing endoplasmic reticulum homeostasis and stress in mammalian systems. FEBS J. 287, 27–42. doi:10.1111/febs.15107
Silke, J., and Brink, R. (2010). Regulation of TNFRSF and innate immune signalling complexes by TRAFs and cIAPs. Cell. Death Differ. 17, 35–45. doi:10.1038/cdd.2009.114
Simon, A., Park, H., Maddipati, R., Lobito, A. A., Bulua, A. C., Jackson, A. J., et al. (2010). Concerted action of wild-type and mutant TNF receptors enhances inflammation in TNF receptor 1-associated periodic fever syndrome. Proc. Natl. Acad. Sci. U. S. A. 107, 9801–9806. doi:10.1073/pnas.0914118107
Stojanov, S., Lohse, P., Mcdermott, M. F., Renner, E. D., KéRY, A., Mirakian, R., et al. (2004). Periodic fever due to a novel TNFRSF1A mutation in a heterozygous Chinese carrier of MEFV E148Q. Rheumatol. Oxf. 43, 526–527. doi:10.1093/rheumatology/keh066
Stojanov, S., and Mcdermott, M. F. (2005). The tumour necrosis factor receptor-associated periodic syndrome: current concepts. Expert Rev. Mol. Med. 7, 1–18. doi:10.1017/S1462399405009749
Ter Haar, N. M., and Frenkel, J. (2014). Treatment of hereditary autoinflammatory diseases. Curr. Opin. Rheumatol. 26, 252–258. doi:10.1097/BOR.0000000000000059
Ter Haar, N., Lachmann, H., Özen, S., Woo, P., Uziel, Y., Modesto, C., et al. (2013). Treatment of autoinflammatory diseases: results from the Eurofever Registry and a literature review. Ann. Rheum. Dis. 72, 678–685. doi:10.1136/annrheumdis-2011-201268
Vaitla, P. M., Radford, P. M., Tighe, P. J., Powell, R. J., Mcdermott, E. M., Todd, I., et al. (2011). Role of interleukin-6 in a patient with tumor necrosis factor receptor-associated periodic syndrome: assessment of outcomes following treatment with the anti-interleukin-6 receptor monoclonal antibody tocilizumab. Arthritis Rheum. 63, 1151–1155. doi:10.1002/art.30215
Vitale, A., Sota, J., Obici, L., Ricco, N., Maggio, M. C., Cattalini, M., et al. (2020). Role of colchicine treatment in tumor necrosis factor receptor associated periodic syndrome (TRAPS): real-life data from the AIDA network. Mediat. Inflamm. 2020, 1936960. doi:10.1155/2020/1936960
Yang, Z., Goronzy, J. J., and Weyand, C. M. (2015). Autophagy in autoimmune disease. J. Mol. Med. Berl. 93, 707–717. doi:10.1007/s00109-015-1297-8
Youngstein, T., M, B. C. K., Ros Soto, J., Rowczenio, D., Trojer, H., Lachmann, H. J., et al. (2018). Adult-onset tumour necrosis factor receptor-associated periodic syndrome presenting as transfusion-dependent refractory haemophagocytosis. Rheumatol. Oxf. 57, 582–583. doi:10.1093/rheumatology/kex427
Keywords: autoinflammatory disease, primary immunodeficiency disease, fever, tumor necrosis factor receptor-associated periodic syndrome, TNFRSF1A summary
Citation: Qian M, Zhou J, Wu J, Zhang H, Yu S, Xu H, Yang Y, Zhou F, Yang Q, Shao L, Zhang W, Jiang N and Ruan Q (2024) A rare missense p.C125Y mutation in the TNFRSF1A gene identified in a Chinese family with tumor necrosis factor receptor-associated periodic fever syndrome. Front. Genet. 15:1413641. doi: 10.3389/fgene.2024.1413641
Received: 07 April 2024; Accepted: 31 May 2024;
Published: 24 June 2024.
Edited by:
Yiping Shen, Harvard Medical School, United StatesReviewed by:
Shengnan Wu, Shanghai Children’s Hospital, ChinaQiwei Guo, Xiamen University, China
Xin Meng, Fudan University, China
Copyright © 2024 Qian, Zhou, Wu, Zhang, Yu, Xu, Yang, Zhou, Yang, Shao, Zhang, Jiang and Ruan. This is an open-access article distributed under the terms of the Creative Commons Attribution License (CC BY). The use, distribution or reproduction in other forums is permitted, provided the original author(s) and the copyright owner(s) are credited and that the original publication in this journal is cited, in accordance with accepted academic practice. No use, distribution or reproduction is permitted which does not comply with these terms.
*Correspondence: Qiaoling Ruan, qlruan07@fudan.edu.cn; Ning Jiang, ningjiang@fudan.edu.cn
†These authors have contributed equally to this work