- 1Department of Pharmacy, Qingdao University Medical College Affiliated Yantai Yuhuangding Hospital, Yantai, China
- 2Department of Ultrasound, Qingdao University Medical College Affiliated Yantai Yuhuangding Hospital, Yantai, China
- 3Center for Evidence-Based and Translational Medicine, Zhongnan Hospital of Wuhan University, Wuhan, China
- 4Department of Intensive Care Unit, Yantai Yeda Hospital, Yantai, China
Purpose: Major depressive disorder (MDD) and venous thromboembolism (VTE) may be linked in observational studies. However, the causal association remains ambiguous. Therefore, this study investigates the causal associations between them.
Methods: We performed a two-sample univariable and multivariable bidirectional Mendelian randomization (MR) analysis to evaluate the associations between MDD and VTE. The summary genetic associations of MDD statistics were obtained from the Psychiatric Genomics Consortium and UK Biobank. Information on VTE, deep vein thrombosis (DVT), and pulmonary embolism (PE) were obtained from the FinnGen Biobank. Inverse-variance weighting was used as the main analysis method. Other methods include weighted median, MR-Egger, Simple mode, and Weighted mode.
Results: Univariable MR analysis revealed no significant associations between MDD and VTE risk (odds ratio (OR): 0.936, 95% confidence interval (CI): 0.736–1.190, p = 0.590); however, after adjusting the potential relevant polymorphisms of body mass index and education, the multivariable MR analysis showed suggestive evidence of association between them (OR: 1.163, 95% CI: 1.004–1.346, p = 0.044). Univariable MR analysis also revealed significant associations between MDD and PE risk (OR: 1.310, 95% CI: 1.073–1.598, p = 0.008), but the association between them was no longer significant in MVMR analysis (p = 0.072). We found no significant causal effects between MDD and DVT risk in univariable or multivariable MR analyses. There was also no clear evidence showing the causal effects between VTE, PE, or DVT and MDD risk.
Conclusion: We provide suggestive genetic evidence to support the causal association between MDD and VTE risk. No causal associations were observed between VTE, PE, or DVT and MDD risk. Further validation of these associations and investigations of potential mechanisms are required.
1 Introduction
Venous thromboembolism (VTE), including deep vein thrombosis (DVT) and pulmonary embolism (PE), is a multifactorial disorder disease with hereditary and acquired risk factors (Rosendaal, 1999). VTE has a high annual incidence of 1–2 persons per 1,000 individuals and is a major contributor to the global disease burden (Wendelboe and Raskob, 2016; Khan et al., 2021). Any cause that can lead to venous endothelial injury, stagnant venous blood flow, and a hypercoagulable state of the blood is a risk factor for VTE (Piazza, 2015). Regarding the risk factors for VTE, in addition to the identified risk factors such as trauma, surgery, obesity, and genetic susceptibility to thrombosis, more unidentified factors may be related to VTE etiology (Pastori et al., 2023). Identifying high-risk patients for early risk stratification of patients may result in more effective treatment strategies (Barco et al., 2016; Barco et al., 2020).
Major depressive disorder (MDD) is a psychological disorder that changes an individual’s emotional state, leading to diminished experience of positive emotions, cognitive impairments, and poor concentration; it is a serious threat to the physical and mental health of individuals (Dehn and Beblo, 2019). Increasing evidence shows that depression and prothrombotic states are associated with dysfunction of the stress response system (Gold, 2015; Hunter et al., 2017; Sandrini et al., 2020). Several common mechanistic pathways, such as increased platelet activation, procoagulant activity, endothelial dysfunction, and inflammatory processes, may trigger the development of depression and VTE (Gold, 2015). Despite the plausibility of behavioral and biological mechanisms, the existing findings are inconsistent. In a prospective study, the risk of venous thrombosis was 1.6 times higher in patients with depression than in those without depression (Austin et al., 2013). However, a large prospective study showed that VTE risk was not significantly increased in women who reported depression or anxiety (Parkin et al., 2017). Concurrently, there may be a reverse causality between depression and VTE; a previous study showed a 2.35-fold increased risk of depression in individuals with VTE compared with the general population [9]. To date, based on the inconsistent results derived from observational studies, the relationship between MDD and VTE remains unclear. Additionally, previous studies investigating the relationship between MDD and VTE were observational, and conventional observational studies have difficulty determining whether noted correlations are causal because of limitations, including potential confounders, reversal causality bias, and measurement error (Sekula et al., 2016). Therefore, further evidence is required to elucidate the causal relationship between depression and VTE and the pathways that are potentially involved.
Mendelian randomization (MR) is a statistical method based on genetic variation that uses genetic variation already present in nature as an instrumental variable (IV) to simulate randomly assigned experimental conditions to investigate the causal association between risk factors and disease development (Sekula et al., 2016; Emdin et al., 2017). The central idea is that genetic variation is randomly distributed among individuals and is independent of environmental and behavioral factors. By exploiting the association between these genetic variants and the exposure factor of interest, the causal effect of that exposure on a particular outcome can be inferred (Lawlor et al., 2008). Therefore, this study aimed to investigate the causal associations between MDD and VTE (including DVT and PE) using univariable and multivariable bidirectional two-sample MR analyses.
2 Materials and methods
2.1 Study design
This bidirectional two-sample MR analysis was based on the summary of genetic associations for different genome-wide association studies (GWAS) using single-nucleotide polymorphisms (SNPs) as IVs to investigate the causal associations between MDD and VTE. To gain reliable results, the effective IVs must satisfy three key assumptions during the MR analysis process: 1) the IVs must be strongly associated with the exposure; 2) IVs should not be associated with any confounders influencing the exposure and outcome association; and 3) IVs should be associated with the outcomes only via the exposures (Emdin et al., 2017). First, a forward MR analysis was performed to examine the associations between MDD and VTE. Next, a reverse MR analysis was performed to examine the associations of genetically determined VTE with MDD. The study design of MR analysis is shown in Figure 1.
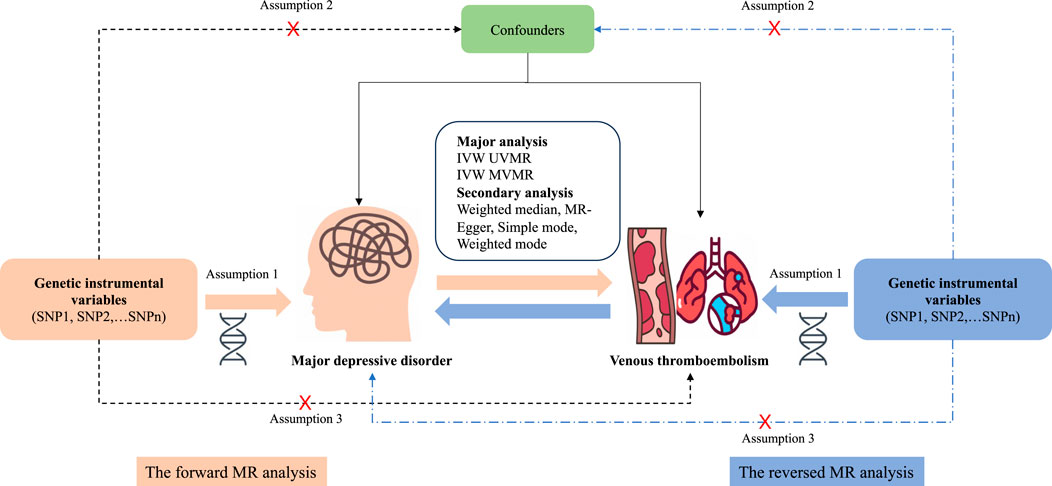
Figure 1. Overview and assumptions of the Mendelian randomization study design. Assumption 1: The instrumental variables should be closely related to the risk factor of interest; assumption 2: The instrumental variables should not be associated with potential confounders; and assumption 3: The instrumental variables should affect the risk of outcome only through risk factors and not through other alternative pathways. SNP, single-nucleotide polymorphisms; MDD, major depressive disorder; VTE, venous thromboembolism; PE, pulmonary embolism; DVT, deep venous thrombosis; IVW, inverse-variance weighting; UVMR, univariable Mendelian randomization; MVMR, multivariable Mendelian randomization.
2.2 Genetic data source of MDD and VTE
For our analyses, data regarding MDD and VTE were obtained from publicly available large-scale GWAS databases. The summary genetic associations of MDD statistics were obtained from the Psychiatric Genomics Consortium and UK Biobank, extracted from 170,756 cases and 329,443 controls based on European samples (Howard et al., 2019). To investigate the genetic associations with VTE, we extracted GWAS datasets from the FinnGen Biobank (Release 8, https://finngen.gitbook.io/documentation/v/r8/data-download). The latest version of the database was used for VTE (17,048 cases and 325,451 controls), PE (8,170 cases and 333,487 controls), and DVT (8,077 cases and 295,014 controls). Based on the International Classification of Diseases version 9, the definitions of VTE, DVT, and PE were developed. Detailed information on the GWAS in our study is presented in Supplementary Table S1.
2.3 Selection of IVs
From GWAS summary data of MDD and VTE, only SNPs strongly associated with exposure (p < 5 × 10−8) were extracted as genetic instruments. Linkage disequilibrium among SNPs defined by r2 > 0.001 or clump distance <10,000 kb and those with higher p values were discarded, and the remaining SNPs were used as instruments. Next, the SNPs were then harmonized to ensure that the effect alleles were consistent in terms of both outcome and exposure data. Subsequently, we performed Steiger filtering to determine whether the directionality of a single SNP was correct and removed SNPs that were more strongly associated with outcome than with exposure (Hemani et al., 2017). The strength of IVs was examined using F-statistics; when the corresponding F statistic was >10, the instrument’s strength was deemed to be sufficient (Brion et al., 2013). We applied mRnd for power calculation (https://shiny.cnsgenomics.com/mRnd/), assuming a 5% type I error rate and power ≥80%. We used the PhenoScanner database to investigate the previously reported associations between instrument SNPs and potential confounding factors, and SNPs associated with potential confounders or outcome variables at genome-wide significance (p < 5 × 10−8) were removed (Kamat et al., 2019). The PhenoScanner database search revealed associations of instruments with education and body mass index (BMI) traits. Observational studies have also demonstrated that education and BMI are associated with the incidence of MDD and VTE (Zöller et al., 2012; Rahmani et al., 2020; Huet et al., 2021; Wickersham et al., 2021; de Wit et al., 2022). Therefore, we chose SNPs associated with education and BMI from GWAS for multivariable MR analysis. Details of the GWAS of risk cofounders are presented in Supplementary Table S2.
2.4 Statistical analyses
In our study, we tested the heterogeneity using Cochran’s Q test, and Cochran’s Q-derived p < 0.05 indicated heterogeneity. We applied inverse-variance weighting (IVW) as the primary analysis method to evaluate the relationship between MDD and VTE (Burgess et al., 2013). Furthermore, to assess the robustness of the results, other sensitivity analyses, including the weighted median (Bowden et al., 2016), MR-Egger regression (Bowden et al., 2015), weighted mode (Hartwig et al., 2017), simple mode (Zhu et al., 2022), and MR–pleiotropy residual sum and outlier (MR-PRESSO) (Verbanck et al., 2018) were conducted. The IVW method synthesizes the effect derived from each instrument by using the inverse variance as the weight and is applied on the assumption that all SNPs are valid instrumental variables and completely independent of each other (Burgess et al., 2013). The weighted median model can provide consistent estimates when ≥50% of the weights come from valid IVs (Bowden et al., 2016). In contrast, the MR-Egger method allows each IV to exhibit pleiotropy, and if the instrument strength is not related to these pleiotropic effects, the method is consistent (Bowden et al., 2015). The MR-Egger regression can inspect the horizontal pleiotropy through its intercept, and a p-value of >0.05 indicates no potential horizontal pleiotropy (Bowden et al., 2015). The MR-PRESSO method can detect the outliers and generate estimates after removing the outliers, which is designed to test the difference in the estimation before and after the outlier correction, with p < 0.05 of the distortion tests indicating a significant difference (Verbanck et al., 2018). To identify whether the association could be affected by a particular single SNP, we used the leave-one-out test to test the sensitivity of the results. Finally, a multivariable MR analysis was performed to assess the causal relationship between MDD and VTE, adjusting for education and BMI in our models to assess the effect of potential confounders. The multivariable MR simultaneously considers multiple exposure factors; it limits the corresponding effects of SNP exposure on the characteristics of other assumed risk factors and eliminates possible biases (Huang G. et al., 2023).
All statistical analysis was performed using R Software (version 4.1.2). TwoSampleMR (0.5.7), MR-PRESSO (1.0), and Mendelian Randomization (0.7.0) packages were used for the MR analysis. After Bonferroni correction, results with p < 0.05/3 (1 exposure × 3 outcomes) = 0.017 were considered statistically significant; estimates with a p-value between 0.017 and 0.05 were regarded as evidence suggesting an association in our study (Huang Y. et al., 2023).
3 Results
3.1 Basic information of IVs
The F-statistic of all included SNPs was >10, suggesting strong instruments (Supplementary Tables S3, S4). The MR-Steiger showed that the SNPs were more strongly associated with exposure than with outcome. For the MR statistical power, assuming a 5% type I error rate and power ≥80%, the power was low in both forward and reversed MR analysis (Supplementary Table S5).
3.2 Univariable MR analysis of MDD and VTE risk
In the univariable MR analysis, independent SNPs (37 each) were selected and used as instruments in the MR analysis for the associations of MDD with VTE, PE, and DVT (Supplementary Table S3). The IVW results showed no significant causal association between MDD and VTE (odds ratio (OR): 0.936, 95% confidence interval (CI): 0.736–1.190, p = 0.590) or DVT risk (OR: 0.936, 95% CI: 0.736–1.190, p = 0.590); furthermore, we observed similar results by using weighted median, MR Egger, weighted mode, and simple mode. The IVW results suggested a significant causal association between MDD and PE risk (OR: 1.310, 95% CI: 1.073–1.598, p = 0.008); however, we observed different results by using weighted median, MR Egger, weighted mode, and simple mode (Figure 2 and Supplementary Table S6). No significant association was observed between MDD and VTE, PE, or DVT risk using MR-PRESSO (Supplementary Table S5). Cochran’s Q test supported the existence of significant heterogeneity between exposure and outcome (P-heterogeneity <0.05; Supplementary Table S6). The MR-Egger intercept provided no statistical evidence of directional horizontal pleiotropy (all p > 0.05, Supplementary Figures S1–S3). The leave-one-out analyses identified no individual IV, which largely affected the causal magnitude between MDD and the risk of VTE, PE, and DVT under the IVW model (Supplementary Figures S4–S6).
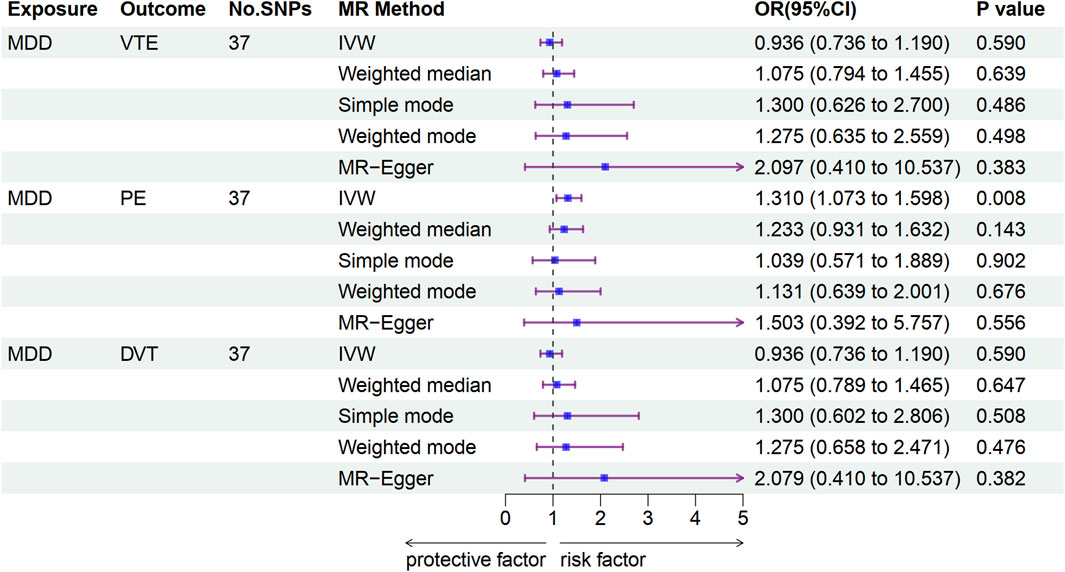
Figure 2. Associations of genetically predicted MDD with VTE development according to Mendelian randomization analyses. MDD, major depressive disorder; VTE, venous thromboembolism; PE, pulmonary embolism; DVT, deep venous thrombosis; OR, odds ratio; CI, confidence interval.
3.3 Multivariable MR analysis of MDD and VTE risk
After adjusting for potentially relevant polymorphisms related to education and BMI, the multivariable MR results showed evidence suggestive of an association between MDD and VTE risk (OR: 1.163, 95% CI: 1.004–1.346, p = 0.044). The likely reason for this is that the true effect was masked by confounding factors since the univariable analyses were not adjusted for them. After the relevant adjustments were performed, the effect became evident.
No significant causal association was observed between MDD and the risk of PE (OR: 1.197, 95% CI: 0.984–1.457, p = 0.072). This difference may be because education and BMI are more strongly correlated with PE and are potential risk factors for PE. When these two variables were considered, their stronger explanatory power for PE caused that of MDD for PE to be relatively weaker, resulting in a non-significant correlation between MDD and PE. No genetic causal associations were observed between MDD and the risk of DVT in multivariable analyses (OR: 0.930, 95% CI: 0.755–1.147, p = 0.499) (Table 1).
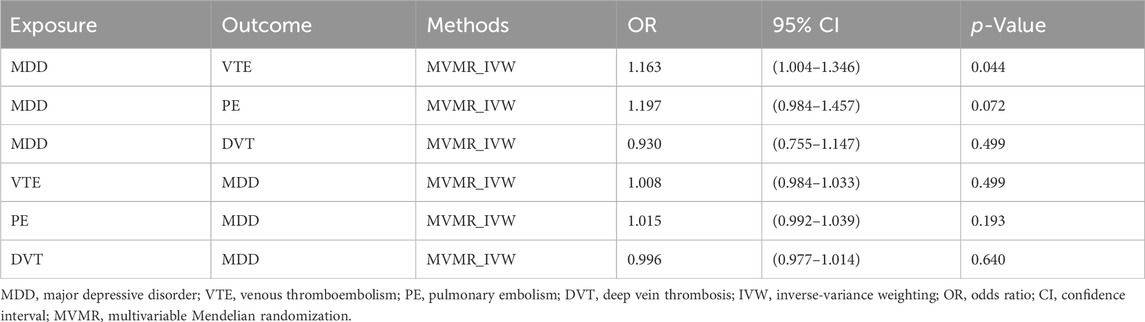
Table 1. Associations between MDD and VTE risk according to multivariable Mendelian randomization sensitivity analyses.
3.4 Univariable MR analysis of VTE and MDD risk
A total of 17, 10, and 11 independent SNPs were used to explore the causal associations of VTE, PE, and DVT with the MDD risk, respectively (Supplementary Table S4). The IVW results suggested no significant associations between VTE (OR: 1.004, 95% CI: 0.989–1.020, p = 0.597), PE (OR: 1.019, 95% CI: 0.999–1.039, p = 0.066), or DVT (OR: 1.000, 95% CI: 0.984–1.016, p = 0.971) and MDD risk. We observed similar results by using weighted median, MR Egger, weighted mode, and simple mode (Figure 3 and Supplementary Table S7). Additionally, no significant association was observed between VTE, PE, or DVT and MDD risk using MR-PRESSO (Supplementary Table S5). Cochran’s Q test supported the existence of significant heterogeneity between exposure and outcome (P-heterogeneity <0.05; Supplementary Table S7). The MR-Egger intercept provided no existence of horizontal pleiotropy (all p > 0.05, Supplementary Figures S7–S9). The leave-one-out analyses showed that the MR analyses were stable and not affected by individual SNPs (Supplementary Figures S10–S12).
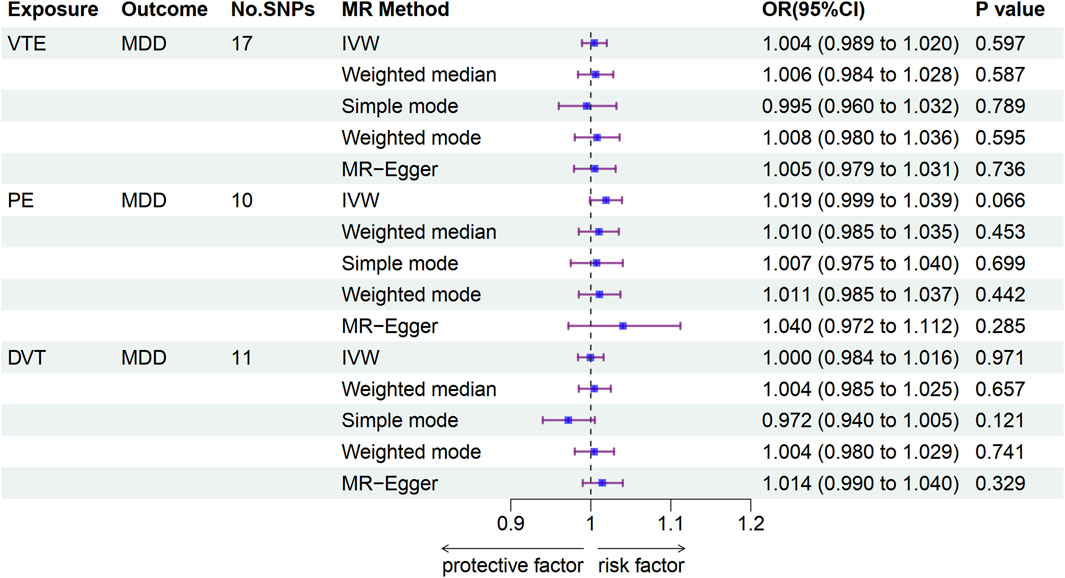
Figure 3. Associations of genetically predicted VTE with MDD according to Mendelian randomization analyses. MDD, major depressive disorder; VTE, venous thromboembolism; PE, pulmonary embolism; DVT, deep venous thrombosis; OR, odds ratio; CI, confidence interval.
3.5 Multivariable MR analysis of VTE and MDD risk
The multivariable MR analysis results were consistent with the univariable MR analysis results. No significant causal association was observed between VTE (OR: 1.008, 95% CI: 0.984–1.033, p = 0.499), PE (OR: 1.015, 95% CI: 0.992–1.039, p = 0.193), or DVT (OR: 1.014, 95% CI: 0.977–1.014, p = 0.640) and MDD risk (Table 1).
4 Discussion
In this study, we performed a bidirectional two-sample MR analysis to investigate a genetically predicted causal association between MDD and VTE. Our findings provided suggestive evidence of the association between MDD and VTE risk. Furthermore, the reversed MR analysis found no genetic evidence of any causal association between VTE, PE, or DVT and MDD risk in either the univariable or the multivariable analyses.
We used BMI and education as confounders in our study. Previous studies have shown the correlation of BMI with depression and VTE. A representative psychiatric cohort study in the Netherlands showed that individuals with obesity at baseline had a significantly increased risk of developing any mood or anxiety disorder, even after adjusting for covariates compared to persons with a normal BMI (de Wit et al., 2022). Another observational study indicated that adiposity characteristics may help identify individuals at increased risk for neuropsychiatric comorbidity (Huet et al., 2021). A meta-analysis with 3,910,747 participants highlights obesity as a significant risk factor related to the incidence of VTE and PE (Rahmani et al., 2020). Additionally, the level of education was associated with depression and the incidence of VTE. A meta-analysis of 22 studies revealed that depression was associated with lower educational attainment (Wickersham et al., 2021). Furthermore, an epidemiological study from Sweden showed that individuals with high educational levels and those in several occupations requiring high levels of education had a lower risk of VTE, while those with low levels of education had an increased risk of VTE (Zöller et al., 2012).
4.1 MDD and VTE risk
Overall, we verified the relationship between MDD and VTE, which is consistent with several experimental studies. Recently, studies have shown that depression may increase the risk of VTE through genetic and biological mechanisms as well as behavioural mechanisms (Gold, 2015; Adelborg et al., 2017). Amadio et al. (Amadio et al., 2017) investigated the relationship between depression and VTE at a molecular level and found that it may be related to the presence of SNP variants on the gene encoding brain-derived neurotrophic factor, which increases susceptibility to depression while leading to concomitant hypercoagulability and platelet hyperreactivity in individuals. Moreover, the levels of numerous pro-inflammatory markers are higher in patients with depression than in the general population (van Aken et al., 2002; Reitsma and Rosendaal, 2004). Pro-inflammatory markers may reduce the activity of vasodilatory factors through a microvascular endothelium-dependent mechanism, inhibit endothelial nitric oxide synthase activity, and increase endothelin-1 secretion, leading to abnormal microvessel constriction, vasodilatory dysfunction, narrowing of the lumen, slowing of the blood flow, and thrombosis (Zi-xin and Yong-gui, 2021). Furthermore, acquired behavioural habits in patients with depression are associated with VTE. An epidemiological survey in Europe (Austin et al., 2013) showed that 73% of patients with depression had hypodynamic symptoms, including reduced energy and fatigue, which further increased the risk of thrombosis.
Several previous observational studies have demonstrated the association between depression and VTE, while others have shown opposite results (Austin et al., 2013; Lee et al., 2015; Parkin et al., 2017; Kunutsor et al., 2018; Kowal et al., 2020). A retrospective cohort study in Taiwan found that the risk of venous thrombosis was 1.38 times higher in patients with depression than in those without depression (Lee et al., 2015). In a prospective study, the risk of venous thrombosis was 1.6 times higher in patients with depression than in those without depression over a 12-year follow-up period (Austin et al., 2013). A systematic analysis of the risk of venous thrombosis in all psychiatric disorders collected since 1998 showed that the risk of venous thrombosis in patients with depression was 1.29 times higher than that in controls (Kowal et al., 2020). The systematic review and meta-analysis of observational studies published in 2018 included eight observational studies involving 960,113 non-overlapping participants and 9,027 VTE cases. Their results showed an increased risk of VTE in individuals with depression (RR = 1.31) compared with those without depression (Kunutsor et al., 2018). However, some studies showed inconsistent results. For example, a large prospective study involving women in the United Kingdom demonstrated that VTE risk was not significantly increased in women who reported being treated for depression or anxiety but did not use antidepressants or other psychotropic drugs (hazard ratio, 1.19) (Parkin et al., 2017).
Nevertheless, the precise pathophysiology underlying the association between MDD and VTE remains unknown. Therefore, our MR analysis was conducted to clarify the connection between them, and the results provide novel insights into the impact of MDD on VTE and potential avenues for prevention.
4.2 VTE and MDD risk
In our study, we observed no association between VTE and MDD risk, which differed from the results of previous studies.
Previous studies have shown that VTE may lead to depression through various psychosocial mechanisms. VTE is an acute, life-threatening event that causes deterioration in functioning, including pain, swelling, and reduced mobility, resulting in a reduced quality of life; additionally, an increased risk of bleeding due to the prolonged use of anticoagulants may lead to depression (Ghanima et al., 2018; Jørgensen et al., 2021). A 10-year population-cohort study involving more than 380,000 patients in Sweden showed that individuals diagnosed with VTE had a 2.35-fold increased risk of depression compared with the general population, and the risk estimates were moderately attenuated after adjusting for socioeconomic status and comorbidities (Jørgensen et al., 2023). However, the observed associations may be due to confounding factors such as education level, lifestyle, diet, or mobility problems, which are common features in some of these patients. In our study, we eliminated the confounders and found no association between VTE and MDD risk. To the best of our knowledge, this is the first comprehensive MR examination of the risks associated with venous thrombosis and depression.
4.3 Strengths and limitations
Our study has several strengths. First, according to the core principles of MR, this method can largely avoid the influence of reverse causality and confounding factors, which typically affect the results of conventional observational studies. Second, in this study, two-sample bidirectional MR was first applied to investigate the causal relationship between MDD and VTE, considering the large sample size and the comprehensive types of VTE. Third, regarding instrument selection, we had a strict selection threshold (p < 5 × 10−8) to reduce weak instrument bias. The F-statistics of all associations in our study exceeded 10, indicating the appropriate strength of the genetic instruments and the absence of weak instrument bias. Additionally, we used the multivariable MR methods to account for the potential pleiotropic associations between MDD and VTE.
However, our study has some limitations. First, to ensure homogeneity of genetic backgrounds, our study only included individuals of European descent, thereby limiting the generalizability of our results to other ethnic populations. Second, multivariable analysis could not overcome bias owing to pleiotropic effects from confounders other than education or BMI. Furthermore, because our findings may have had insufficient statistical power, a large amount of data from the GWAS databases is required to confirm the relationship between depression and different types of VTE.
5 Conclusion
Our MR analysis provided suggestive genetic evidence to support the causal association between MDD and VTE risk. Moreover, no causal associations were observed between VTE, PE, or DVT and MDD risk. Further validation of these associations and investigations of potential mechanisms are required.
Data availability statement
The datasets presented in this study can be found in online repositories. The names of the repository/repositories and accession number(s) can be found in the article/Supplementary Material.
Ethics statement
Ethical approval was not required for the study involving humans in accordance with the local legislation and institutional requirements. Written informed consent to participate in this study was not required from the participants or the participants’ legal guardians/next of kin in accordance with the national legislation and the institutional requirements.
Author contributions
H-YL: Conceptualization, Formal Analysis, Software, Writing–original draft, Writing–review and editing. L-HW: Conceptualization, Data curation, Formal Analysis, Methodology, Writing–original draft. JW: Data curation, Methodology, Writing–original draft. Y-BW: Methodology, Software, Supervision, Validation, Writing–review and editing. H-SW: Conceptualization, Project administration, Supervision, Validation, Visualization, Writing–review and editing.
Funding
The author(s) declare that no financial support was received for the research, authorship, and/or publication of this article.
Acknowledgments
We gratefully acknowledge the following consortiums: the Psychiatric Genomics Consortium (PGC), the United Kingdom Biobank (UKB), and the FinnGen Consortium for making their GWAS summary-level statistics publicly available. We thank all investigators for sharing these data.
Conflict of interest
The authors declare that the research was conducted in the absence of any commercial or financial relationships that could be construed as a potential conflict of interest.
Publisher’s note
All claims expressed in this article are solely those of the authors and do not necessarily represent those of their affiliated organizations, or those of the publisher, the editors and the reviewers. Any product that may be evaluated in this article, or claim that may be made by its manufacturer, is not guaranteed or endorsed by the publisher.
Supplementary material
The Supplementary Material for this article can be found online at: https://www.frontiersin.org/articles/10.3389/fgene.2024.1383333/full#supplementary-material
References
Adelborg, K., Sundbøll, J., Videbech, P., and Grove, E. L. (2017). The risk of thromboembolism in users of antidepressants and antipsychotics. Adv. Exp. Med. Biol. 906, 351–361. doi:10.1007/5584_2016_125
Amadio, P., Colombo, G. I., Tarantino, E., Gianellini, S., Ieraci, A., Brioschi, M., et al. (2017). BDNFVal66met polymorphism: a potential bridge between depression and thrombosis. Eur. Heart J. 38 (18), 1426–1435. doi:10.1093/eurheartj/ehv655
Austin, A. W., Wissmann, T., and Von Kanel, R. (2013). Stress and hemostasis: an update. Semin. Thromb. Hemost. 39 (8), 902–912. doi:10.1055/s-0033-1357487
Barco, S., Mahmoudpour, S. H., Valerio, L., Klok, F. A., Münzel, T., Middeldorp, S., et al. (2020). Trends in mortality related to pulmonary embolism in the European Region, 2000-15: analysis of vital registration data from the WHO Mortality Database. Lancet Respir. Med. 8 (3), 277–287. doi:10.1016/S2213-2600(19)30354-6
Barco, S., Woersching, A. L., Spyropoulos, A. C., Piovella, F., and Mahan, C. E. (2016). European Union-28: an annualised cost-of-illness model for venous thromboembolism. Thromb. Haemost. 115 (4), 800–808. doi:10.1160/TH15-08-0670
Bowden, J., Davey Smith, G., and Burgess, S. (2015). Mendelian randomization with invalid instruments: effect estimation and bias detection through Egger regression. Int. J. Epidemiol. 44 (2), 512–525. doi:10.1093/ije/dyv080
Bowden, J., Davey Smith, G., Haycock, P. C., and Burgess, S. (2016). Consistent estimation in mendelian randomization with some invalid instruments using a weighted median estimator. Genet. Epidemiol. 40 (4), 304–314. doi:10.1002/gepi.21965
Brion, M. J., Shakhbazov, K., and Visscher, P. M. (2013). Calculating statistical power in Mendelian randomization studies. Int. J. Epidemiol. 42 (5), 1497–1501. doi:10.1093/ije/dyt179
Burgess, S., Butterworth, A., and Thompson, S. G. (2013). Mendelian randomization analysis with multiple genetic variants using summarized data. Genet. Epidemiol. 37 (7), 658–665. doi:10.1002/gepi.21758
Dehn, L. B., and Beblo, T. (2019). Depressed, biased, forgetful: the interaction of emotional and cognitive dysfunctions in depression. Neuropsychiatr 33 (3), 123–130. doi:10.1007/s40211-019-0307-4
De Wit, L., Have, M. T., Cuijpers, P., and De Graaf, R. (2022). Body mass index and risk for onset of mood and anxiety disorders in the general population: results from The Netherlands mental health survey and incidence study-2 (NEMESIS-2). BMC Psychiatry 22 (1), 522. doi:10.1186/s12888-022-04077-w
Emdin, C. A., Khera, A. V., and Kathiresan, S. (2017). Mendelian randomization. Jama 318 (19), 1925–1926. doi:10.1001/jama.2017.17219
Ghanima, W., Wik, H. S., Tavoly, M., Enden, T., and Jelsness-Jørgensen, L.-P. (2018). Late consequences of venous thromboembolism: measuring quality of life after deep vein thrombosis and pulmonary embolism. Thrombosis Res. 164, 170–176. doi:10.1016/j.thromres.2017.07.025
Gold, P. W. (2015). The organization of the stress system and its dysregulation in depressive illness. Mol. Psychiatry 20 (1), 32–47. doi:10.1038/mp.2014.163
Hartwig, F. P., Davey Smith, G., and Bowden, J. (2017). Robust inference in summary data Mendelian randomization via the zero modal pleiotropy assumption. Int. J. Epidemiol. 46 (6), 1985–1998. doi:10.1093/ije/dyx102
Hemani, G., Tilling, K., and Davey Smith, G. (2017). Orienting the causal relationship between imprecisely measured traits using GWAS summary data. PLoS Genet. 13 (11), e1007081. doi:10.1371/journal.pgen.1007081
Howard, D. M., Adams, M. J., Clarke, T. K., Hafferty, J. D., Gibson, J., Shirali, M., et al. (2019). Genome-wide meta-analysis of depression identifies 102 independent variants and highlights the importance of the prefrontal brain regions. Nat. Neurosci. 22 (3), 343–352. doi:10.1038/s41593-018-0326-7
Huang, G., Chen, X., Chen, Y., Liu, W., Chen, C., Song, W., et al. (2023a). Causal relationship between type 2 diabetes mellitus and bone mineral density: a Mendelian randomization study in an East Asian population. Osteoporos. Int. 34, 1719–1727. doi:10.1007/s00198-023-06807-6
Huang, Y., Wang, J., Yang, H., Lin, Z., and Xu, L. (2023b). Causal associations between polyunsaturated fatty acids and kidney function: a bidirectional Mendelian randomization study. Am. J. Clin. Nutr. 117 (1), 199–206. doi:10.1016/j.ajcnut.2022.11.010
Huet, L., Delgado, I., Dexpert, S., Sauvant, J., Aouizerate, B., Beau, C., et al. (2021). Relationship between body mass index and neuropsychiatric symptoms: evidence and inflammatory correlates. Brain Behav. Immun. 94, 104–110. doi:10.1016/j.bbi.2021.02.031
Hunter, R., Lewis, S., Noble, S., Rance, J., and Bennett, P. D. (2017). Post-thrombotic panic syndrome": a thematic analysis of the experience of venous thromboembolism. Br. J. Health Psychol. 22 (1), 8–25. doi:10.1111/bjhp.12213
Jørgensen, H., Horváth-Puhó, E., Laugesen, K., Brækkan, S., Hansen, J. B., and Sørensen, H. T. (2021). Risk of a permanent work-related disability pension after incident venous thromboembolism in Denmark: a population-based cohort study. PLoS Med. 18 (8), e1003770. doi:10.1371/journal.pmed.1003770
Jørgensen, H., Horváth-Puhó, E., Laugesen, K., Brækkan, S. K., Hansen, J. B., and Sørensen, H. T. (2023). Venous thromboembolism and risk of depression: a population-based cohort study. J. Thromb. Haemost. 21 (4), 953–962. doi:10.1016/j.jtha.2022.12.006
Kamat, M. A., Blackshaw, J. A., Young, R., Surendran, P., Burgess, S., Danesh, J., et al. (2019). PhenoScanner V2: an expanded tool for searching human genotype-phenotype associations. Bioinformatics 35 (22), 4851–4853. doi:10.1093/bioinformatics/btz469
Khan, F., Tritschler, T., Kahn, S. R., and Rodger, M. A. (2021). Venous thromboembolism. Lancet 398 (10294), 64–77. doi:10.1016/S0140-6736(20)32658-1
Kowal, C., Peyre, H., Amad, A., Pelissolo, A., Leboyer, M., Schürhoff, F., et al. (2020). Psychotic, mood, and anxiety disorders and venous thromboembolism: a systematic review and meta-analysis. Psychosom. Med. 82 (9), 838–849. doi:10.1097/PSY.0000000000000863
Kunutsor, S. K., Seidu, S., and Khunti, K. (2018). Depression, antidepressant use, and risk of venous thromboembolism: systematic review and meta-analysis of published observational evidence. Ann. Med. 50 (6), 529–537. doi:10.1080/07853890.2018.1500703
Lawlor, D. A., Harbord, R. M., Sterne, J. A., Timpson, N., and Davey Smith, G. (2008). Mendelian randomization: using genes as instruments for making causal inferences in epidemiology. Stat. Med. 27 (8), 1133–1163. doi:10.1002/sim.3034
Lee, C. W., Liao, C. H., Lin, C. L., Liang, J. A., Sung, F. C., and Kao, C. H. (2015). Depression and risk of venous thromboembolism: a population-based retrospective cohort study. Psychosom. Med. 77 (5), 591–598. doi:10.1097/PSY.0000000000000193
Parkin, L., Balkwill, A., Sweetland, S., Reeves, G. K., Green, J., Beral, V., et al. (2017). Antidepressants, depression, and venous thromboembolism risk: large prospective study of UK women. J. Am. Heart Assoc. 6 (5), e005316. doi:10.1161/JAHA.116.005316
Pastori, D., Cormaci, V. M., Marucci, S., Franchino, G., Del Sole, F., Capozza, A., et al. (2023). A comprehensive review of risk factors for venous thromboembolism: from epidemiology to pathophysiology. Int. J. Mol. Sci. 24 (4), 3169. doi:10.3390/ijms24043169
Piazza, G. (2015). Beyond Virchow's Triad: does cardiovascular inflammation explain the recurrent nature of venous thromboembolism? Vasc. Med. 20 (2), 102–104. doi:10.1177/1358863X14568706
Rahmani, J., Haghighian Roudsari, A., Bawadi, H., Thompson, J., Khalooei Fard, R., Clark, C., et al. (2020). Relationship between body mass index, risk of venous thromboembolism and pulmonary embolism: a systematic review and dose-response meta-analysis of cohort studies among four million participants. Thromb. Res. 192, 64–72. doi:10.1016/j.thromres.2020.05.014
Reitsma, P. H., and Rosendaal, F. R. (2004). Activation of innate immunity in patients with venous thrombosis: the Leiden Thrombophilia Study. J. Thromb. Haemost. 2 (4), 619–622. doi:10.1111/j.1538-7836.2004.00689.x
Rosendaal, F. R. (1999). Venous thrombosis: a multicausal disease. Lancet 353 (9159), 1167–1173. doi:10.1016/s0140-6736(98)10266-0
Sandrini, L., Ieraci, A., Amadio, P., Zarà, M., and Barbieri, S. S. (2020). Impact of acute and chronic stress on thrombosis in healthy individuals and cardiovascular disease patients. Int. J. Mol. Sci. 21 (21), 7818. doi:10.3390/ijms21217818
Sekula, P., Del Greco, M. F., Pattaro, C., and Köttgen, A. (2016). Mendelian randomization as an approach to assess causality using observational data. J. Am. Soc. Nephrol. 27 (11), 3253–3265. doi:10.1681/ASN.2016010098
Van Aken, B. E., Reitsma, P. H., and Rosendaal, F. R. (2002). Interleukin 8 and venous thrombosis: evidence for a role of inflammation in thrombosis. Br. J. Haematol. 116 (1), 173–177. doi:10.1046/j.1365-2141.2002.03245.x
Verbanck, M., Chen, C. Y., Neale, B., and Do, R. (2018). Detection of widespread horizontal pleiotropy in causal relationships inferred from Mendelian randomization between complex traits and diseases. Nat. Genet. 50 (5), 693–698. doi:10.1038/s41588-018-0099-7
Wendelboe, A. M., and Raskob, G. E. (2016). Global burden of thrombosis: epidemiologic aspects. Circ. Res. 118 (9), 1340–1347. doi:10.1161/CIRCRESAHA.115.306841
Wickersham, A., Sugg, H. V. R., Epstein, S., Stewart, R., Ford, T., and Downs, J. (2021). Systematic review and meta-analysis: the association between child and adolescent depression and later educational attainment. J. Am. Acad. Child. Adolesc. Psychiatry 60 (1), 105–118. doi:10.1016/j.jaac.2020.10.008
Zhu, G., Zhou, S., Xu, Y., Gao, R., Li, H., Zhai, B., et al. (2022). Mendelian randomization study on the causal effects of omega-3 fatty acids on rheumatoid arthritis. Clin. Rheumatol. 41 (5), 1305–1312. doi:10.1007/s10067-022-06052-y
Zi-Xin, Z., and Yong-Gui, Y. (2021). Effect of antidepressants on venous thrombosis in patients with depression. J. Clin. Neurology 34 (04), 300–303.
Keywords: major depressive disorder, venous thromboembolism, risk factor, singlenucleotide polymorphisms, mendelian randomization
Citation: Li H-Y, Wang L-H, Wang J, Wang Y-B and Wang H-S (2024) Causal association between major depressive disorder and venous thromboembolism: a bidirectional mendelian randomization study. Front. Genet. 15:1383333. doi: 10.3389/fgene.2024.1383333
Received: 07 February 2024; Accepted: 10 June 2024;
Published: 25 June 2024.
Edited by:
Ilya Blokhin, University of Miami, United StatesReviewed by:
Zachary Freyberg, University of Pittsburgh, United StatesLoay Alrojolah, Yale University, United States
Copyright © 2024 Li, Wang, Wang, Wang and Wang. This is an open-access article distributed under the terms of the Creative Commons Attribution License (CC BY). The use, distribution or reproduction in other forums is permitted, provided the original author(s) and the copyright owner(s) are credited and that the original publication in this journal is cited, in accordance with accepted academic practice. No use, distribution or reproduction is permitted which does not comply with these terms.
*Correspondence: Yong-Bo Wang, wangyb20172030@163.com; Hai-Shan Wang, haishan003@163.com
†These authors have contributed equally to this work