- H. Lee Moffitt Cancer Center, Tampa, FL, United States
Introduction: Medullary thyroid carcinoma (MTC) is an aggressive cancer that is often caused by driver mutations in RET. Splice site variants (SSV) reflect changes in mRNA processing, which may alter protein function. RET SSVs have been described in thyroid tumors in general but have not been extensively studied in MTC.
Methods: The prevalence of RET SSVs was evaluated in 3,624 cases with next generation sequence reports, including 25 MTCs. Fisher exact analysis was performed to compare RET SSV frequency in cancers with/without a diagnosis of MTC.
Results: All 25 MTCs had at least one of the two most common RET SSVs versus 0.3% of 3,599 cancers with other diagnoses (p < 0.00001). The 11 cancers with non-MTC diagnoses that had the common RET SSVs were 4 neuroendocrine cancers, 4 non-small cell lung carcinomas, 2 non-MTC thyroid cancers, and 1 melanoma. All 25 MTCs analyzed had at least one of the two most common RET SSVs, including 4 with no identified mutational driver.
Discussion: The identification of RET SSVs in all MTCs, but rarely in other cancer types, demonstrates that these RET SSVs distinguish MTCs from other cancer types. Future studies are needed to investigate whether these RET SSVs play a pathogenic role in MTC.
Introduction
Medullary thyroid carcinoma (MTC) is a rare but aggressive carcinoma that arises from the neuroendocrine parafollicular C cells of the thyroid (NCCN, 2022). MTC represents 1%–2% of all thyroid cancers in the United States—they can occur sporadically, 80%, or via the germline within the spectrum of multiple endocrine neoplasia 2 (MEN2) syndrome (NCCN, 2022; Thyroid, 2023). The 5-year relative survival for stage I and II MTC is approximately 95%, whereas the 5-year survival for stage IV is approximately 28% (Hundahl et al., 1998; Edge and Compton, 2010). RET, a 20-exon proto-oncogene, encodes a tyrosine kinase receptor involved in the control of cell differentiation and proliferation. RET alterations play a significant role in the development and progression of MTC (Chakravarty et al., 2017). Somatic RET mutations have been reported in 40%–65% of sporadic MTCs and germline RET gain-of-function alterations predispose individuals to MEN2 syndrome (Boichard et al., 2012; Ciampi et al., 2013; Verrienti et al., 2016; Ciampi et al., 2019; Verrienti et al., 2022).
Splice site variants (SSV) are genetic mutations that occur in the regions of the genome that control the way pre-messenger RNA is processed. Specifically, these variants occur in the sequences of nucleotides that direct the removal of introns (non-coding regions) and the joining of exons (coding regions) to form mature messenger RNA. Splice variants can lead to the production of abnormal or truncated proteins, with potentially altered functional properties, that may have a significant impact on cell biology and tumorigenesis (Chen et al., 2015).
The literature about splice variants in thyroid cancers is limited. Two functional isoforms, RET51 and RET9, formed via alternative splicing near the C-terminus (3’ end) of RET have been found to play distinct roles in tumorigenesis and/or development (Richardson et al., 2012). Furthermore, both RET51 and RET9 functional isoforms have been identified in MTC; however, neither played a role in MTC tumorigenesis (Mule et al., 2021). Lorenzo et al. described three alternative splice variants in a MTC cell line (Lorenzo et al., 1995). These variants were described as lacking only exon 3 (RET 2/4), lacking exons 3 and 4 (RET 2/5), and lacking exons 3, 4, and 5 (RET 2/6). A study of papillary thyroid cancer (PTC) biopsies in 2001 identified different splice variants in the RET extracellular domain described as in-frame changes with preservation of the tyrosine kinase domain (RET 1/8, RET 2/8, RET 3/8) (Fluge et al., 2001). In this study, they also identified RET 3/8 in two MTC biopsies and RET2/8 in one MTC biopsy. In 2002, a study of germline (MEN2-related) and sporadic pheochromocytomas described the presence of the same 3 RET splice variants identified in the MTC cell line in pheochromocytomas (RET 2/4, RET 2/5 and RET 2/6) (Le Hir et al., 2002). In 2010, McIver et al. described how abnormal RNA processing may be common in thyroid neoplasms with a possible pathogenetic role (McIver et al., 2000). However, to the best of our knowledge, RET splice variants have not been extensively studied in clinical samples diagnosed with MTC.
In this study, we used retrospective clinical next-generation sequencing (NGS) results to identify RET splice variants and aimed to explore their frequency and potential significance for MTC diagnosis.
Materials and methods
An IRB-approved retrospective review of our institutional database of NGS results from 2018 to 2022 was performed to identify all sequenced cases with a pathological diagnosis of MTC. The database includes results from testing lung, colorectal, melanoma, thyroid, neuroendocrine, pancreatic, soft tissue, prostate, bladder, kidney, head and neck, hepatocellular, gastroesophageal, endometrial, ovarian, cervical, and other various cancers. We then reviewed these cases and recorded all molecular variants, including RET splice variants. We also queried the same database to identify all cases, irrespective of tumor histology, with RET splice variants. NGS was performed with the Illumina TruSight Tumor 170 (Illumina Inc., San Diego, CA) platform, a hybrid-capture 170-gene panel designed to identify clinically important small variants and copy number variants by DNA-based testing and splice variants and fusions by simultaneous RNA-based testing of tissue from solid tumors (Boyle et al., 2021). For a case to be considered positive for a RET splice variant, it needed to have passed RNA quality control (QC) metrics (including greater than 1,000,000 total uniquely mapped reads) with a “true” RET splice variant identified by the Illumina TruSight Tumor 170 pipeline and/or have ≥10 splice variants reads identified via sequence review in Integrative Genomics Viewer (IGV, Broad Institute) software (Robinson et al., 2011). Cases which failed these parameters or had inadequate tumor cellularity (<20%) to properly assess for RET splice variants were excluded. Only cases that passed QC metrics were included in this study. When applicable, results from a targeted seven gene thyroid cancer panel (Invitae Genetics, San Francisco, CA) were used for RET germline analysis. We performed a Student’s t-test to compare the tumor size between primary MTCs with two specific RET SSV versus those with just one and Fisher Exact analysis to compare the frequency of RET splice variants in MTC versus non-MTC cases.
Results
Of the 3,624 cases with NGS results in our institutional database, 37 (1.0%) had a pathological diagnosis of MTC, either primary or metastatic. Of these 37 cases, 12 were excluded due to not meeting the inclusion requirements of this study (tumor cellularity >20% and at least 1,000,000 total unique RNA reads). Of the 25 remaining MTC cases, all (100%) had at least one RET splice variant, with most having multiple. All 25 had at least one of the following two in-frame RET splice variants, t (10; 10) (q11.2; q11.2) (chr10:g.43596172chr10:g.43607546), which we will label RET 2/8 to be consistent with the RET splice variant labeling by Le Hir et al. (Le Hir et al., 2002), or t (10; 10) (q11.2; q11.2) (chr10:g.43596172chr10:g.43600398), which we will label RET 2/4. RET 2/8 splices the 3′ end of exon two amino acid 113 to the 5′ end of exon eight amino acid 508 resulting in the skipping of exons three through 7 (NM_20975.6:r.339_1522del, Figures 1, 2). RET 2/4 splices the 3′ end of exon two amino acid 113 to the 5’ end of exon four amino acid 209 resulting in the skipping of exon 3 (NM_20975.6:r.339_625del).
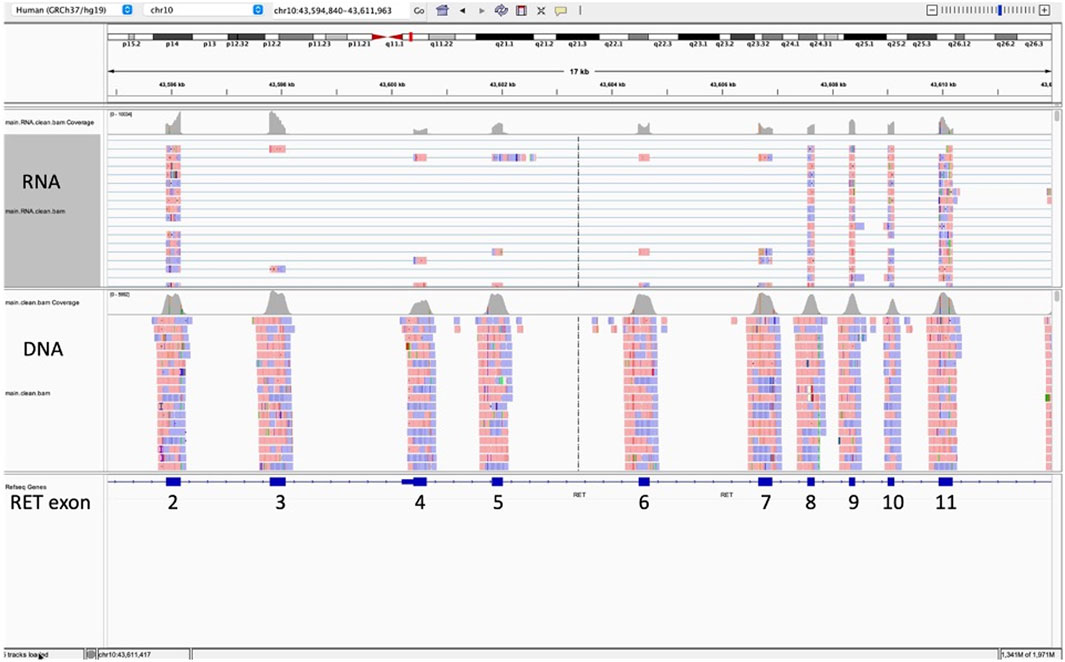
Figure 1. This Integrative Genomics Viewer (IGV) snapshot of sequence (horizontal lines) for RET exons 2 through 11 represents the results for a medullary thyroid carcinoma with RET splice variant 2/8. This splice variant splices the 3′ end of exon two amino acid 113 to the 5′ end of exon eight amino acid 508 with skipping of exons three through 7 (NM_20975.6:r.339_1522del). The splice variant is represented by the blue horizontal lines connecting sequence from exon 2 (forward is red; reverse is blue) directly to exon 8 with no sequence (no red or blue boxes) for exons three to 7.
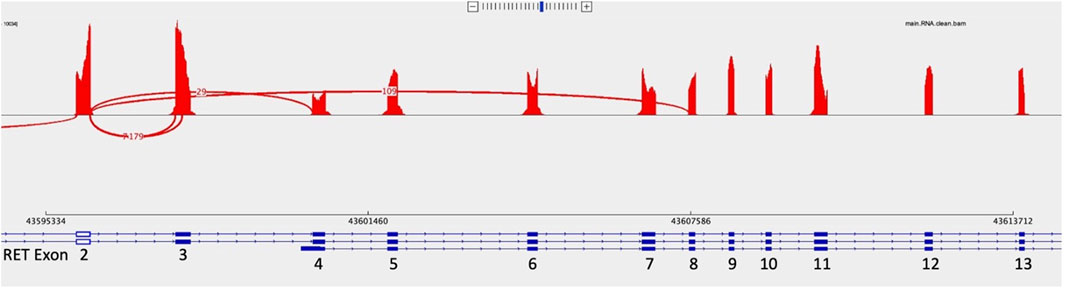
Figure 2. Sashimi plots represent a way to visualize splicing between exons. In this Sashimi plot from a medullary thyroid carcinoma case with a RET 2/8 splice variant, a curved red line represents sequence reads with alternative splicing from the end of exon two through exon 8, skipping exons 3 through 7.
RET 2/8 was identified in 96% of the MTC cases (24/25; 96%) with supporting reads ranging from 10 to 318. We reviewed the sequence of the one negative case for RET 2/8 in IGV and identified four reads consistent with RET 2/8 which was below our reportable threshold of 10 reads to definitively call the case positive for RET 2/8. RET 2/4 was identified in 76% (19/25; 76%) of MTC cases with supporting reads ranging from 10 to 123. Neither RET 2/8 nor RET 2/4 impacted expression of the tyrosine kinase domain, which spans amino acid 724 to amino acid 1005, exons 12 to 19 (Gabreski et al., 2016; Chakravarty et al., 2017; Paratala et al., 2018). Both RET 2/8 and RET 2/4 were identified in 72% of the MTC cases (18/25; 72%). Other splice variants were identified, but at lower frequency.
Of the 25 MTC cases with RET splice variants, 16 (64%) had a concurrent RET mutation with p.M918T as the most prevalent (9/16; 56%) mutation. Of the 16 patients with concurrent RET splice variants and mutations, 12 had a follow-up RET germline test. A germline origin of the mutation was identified in 2 (16.7%) of the 12 resulting in diagnoses of MEN type 2A. The RET germline tests for the other 10 patients were negative, consistent with a somatic origin for the cancers. Of the 9 MTC splice variant positive cases without a concurrent RET mutation, 5 (56%) had HRAS mutations, with p. Q61R (3/5; 60%) as the most prevalent specific mutation. There were 4 (4/25; 16%) RET splice variant positive MTCs with no significant driver mutation identified. Of the 5 cases without RET 2/4, 4 (4/5, 80%) had RET mutations and 1 (1/5, 20%) had a HRAS mutation without a concurrent RET mutation (Table 1).
Nine of the 11 primary MTCs had associated pathological data. The size of these nine primary MTCs tumors ranged from one to 3.8 cm with an average size of 2.1 cm and a median of 1.6 cm. Of the nine primary MTCs, six had both RET SSV 2/8 and RET SSV 2/4, while three had only RET SSV 2/8. The three primary MTCs with only RET SSV 2/8 measured 1, 1.2, and 3.8 cm with an average of 2 cm and a median of 1.2 cm versus a range of 1.2–3.7 cm with an average of 2.1 cm and median of 1.7 cm for the six primary MTCs with both RET SSV 2/8 and RET SSV 2/4. There was no statistically significant difference in tumor size between the two groups (t (9) = 0.16, p = 0.88; Table 2).
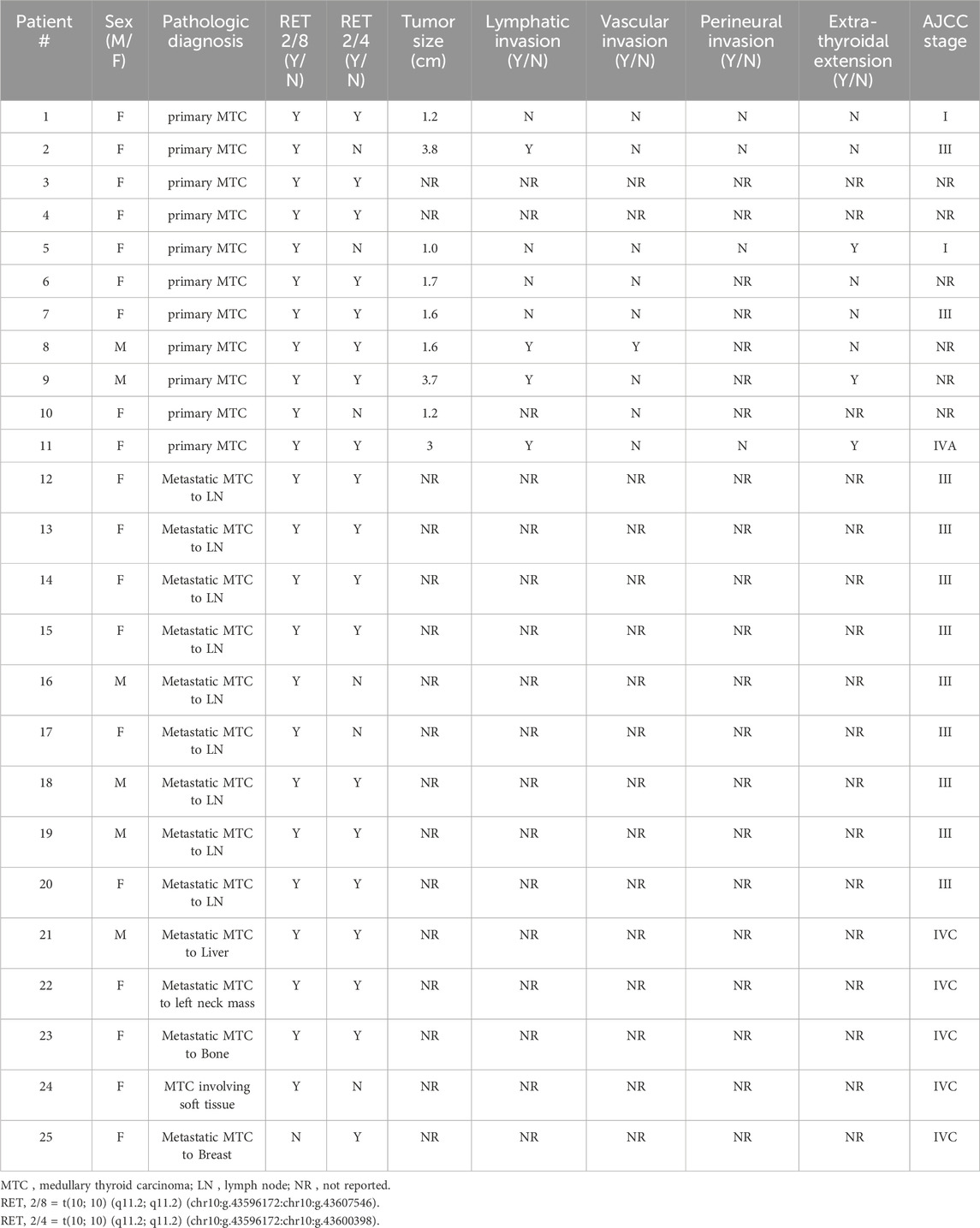
Table 2. Pathological data associated with RET splice site variant positive medullary thyroid carcinomas.
At least one RET splice variant was identified in 11 of 3,599 (0.3%) non-MTC cases. Of these 11 cases, four were neuroendocrine carcinomas (4.7%, 4/86 neuroendocrine carcinomas), four were non-small cell lung carcinomas (0.6%, 4/697 non-small cell lung carcinomas), two were non-MTC thyroid cancers (1.2%, 2/168 non-MTC thyroid cancers) (1 PTC (1.2%, 1/82 PTCs), and one not otherwise specified), and one was a melanoma (0.1%, 1/791 melanomas). Of these 11 non-MTC cancers with a RET splice variant, eight harbored RET 2/8, six had RET 2/4, and six had both splice variants. One case, a mixed neuroendocrine non-neuroendocrine neoplasm (MINEN), had a RET splice variant different than RET 2/8 and RET 2/4 (Table 3).
Overall, a total of 36 of the 3,624 (0.99%) cases were positive for a RET splice variant, of which 25 (69%) were MTC. All 25 MTC cases were RET splice variant positive versus 11 of 3,599 total non-MTC cases (100% versus 0.3%, p < 0.00001).
Disscussion
Medullary thyroid carcinoma is a rare aggressive form of thyroid cancer that can have a sporadic or germline origin. The RET proto-oncogene encodes a receptor tyrosine kinase that when activated either by a point mutation or gene rearrangement can result in a constitutively active cytosolic oncoprotein (Mulligan, 2014; Chakravarty et al., 2017). Although RET mutations are well described in MTC (Ciampi et al., 2019), there is a paucity of information about RET splice variants in MTC, though one study of a MTC cell line described three splice variants, RET 2/4, RET 2/5, and RET 2/6 and another described functional isoforms RET51 and RET9; however, neither played a role in MTC tumorigenesis (Lorenzo et al., 1995; Mule et al., 2021). Our results demonstrate that irrespective of a driving mutation, there is a high frequency of RET splice variants in MTC, with all 25 MTCs in this study harboring at least one RET splice variant versus only 0.3% of 3,599 non-MTCs.
The two main RET splice variants identified by the clinical NGS for this study were in-frame: RET 2/8 with skipping of exons three to seven and RET 2/4 with skipping of only exon 3. Although several other RET splice variants were identified, they were observed at a lower frequency and in conjunction with one of these two more common splice variants. The RET 2/8 and 2/4 splice variants were identified in the RNA-based NGS sequence; review of the accompanying DNA sequence did not reveal the causative DNA changes. The lack of detectable DNA causative changes in cases with appreciable RNA splice events has been extensively reported in the literature, particularly with the well-studied MET exon 14 skipping events. Numerous studies have demonstrated that DNA changes leading to these skipping events can be variable in size and position and can involve extremely large deletions bordering the intron-exon junctions (Dirlon, 2016; Poirot et al., 2017; Davies et al., 2019; Puris et al., 2020). Davies et al. demonstrated that in a cohort positive for MET exon 14 skipping events by RNA-based assays, DNA changes were detected only 60% of the time by DNA-based assays (Davies et al., 2019). They concluded that for accurate detection, a DNA assay should cover all regions involved in splicing, such as the branching site, polypyrimidine tract, splice acceptor and splice donor sites or an RNA-based assay should be used to directly detect the splice variants (Davies et al., 2019). Accordingly, the RET splice variants detected in MTC cases in this study were only identifiable by RNA-based NGS testing, with no evidence for the cause in the DNA-based sequence. The lack of DNA evidence for this change and the predominant use of DNA-based NGS may also explain why these RET splice variants have not been previously reported in clinical MTC cases.
Of note, the two predominant RET splice variants identified in our study cause skipping of exons three through 7, which encode part of the RET extracellular domain. This domain is distant from the intracellular tyrosine kinase domain which is altered by typical RET driver mutations, such as RET p.M918 or p. C634. Since these RET point mutations are so distant from our observed splicing events, we believe it is unlikely that they play any role in the observed RET splicing events.
Although the functional and clinical significance of RET splice variants in humans is not well characterized, two similar splice variants involving skipping of exon 3 (RET 2/4) and exons 3–5 (RET 2/6) were previously described in zebrafish, mice, and rats by Gabreski et al. (Gabreski et al., 2016) They demonstrated that RET 2/4 or RET 2/6 both translated into RET proteins with deletions in the extracellular domain that likely impacted the overall stability of the proteins. Signaling experiments demonstrated that RET 2/4 was phosphorylated similarly to full-length RET, but that RET 2/6 had a higher baseline autophosphorylation on one of the most important signaling residues, Tyr1062 (Gabreski et al., 2016). Further experiments revealed that RET 2/4 and 2/6 were co-expressed with the full-length RET transcript at several developmental time points with particularly high expression in the dorsal root ganglion in mice (Gabreski et al., 2016). Although exons 3, 4, and five are less than 50% conserved between species, these RET 2/4, 2/5, and 2/6 transcripts have also been identified in human kidney and substantia nigra fetal tissues (Lorenzo et al., 1995), and at very low levels (less than 1% of all RET transcripts) in germline (MEN2) and sporadic pheochromocytomas (Le Hir et al., 2002). The authors surmised that it is possible that RET RNA splicing might be dysregulated in tumor cells.
Interestingly, PTCs have been found to overexpress wild type tyrosine kinase RET mRNA with over expression in 70% of papillary thyroid cancers relative to expression in non-neoplastic thyroid tissue (Fluge et al., 2001; Shakiba et al., 2019). The RET promoter is silent in follicular cells and with wild RET expression but not overexpression in follicular cells and tumors derived from them. This study identified the presence of several RET splice variants, including RET 2/8 in PTC (Fluge et al., 2001). Consistent with this study, we identified RET 2/8 in one PTC in our study (Table 3). The authors concluded that wild-type and alternatively spliced RET transcripts co-exist with rearrangements in PTC and may play a role in thyroid tumorigenesis. In MTC though, high RET gene expression levels have not been associated with an alternative RET activation mechanism (Mule et al., 2021).
A couple of studies have described two RET protein isoforms, RET9 and RET51, that are generated by alternative splicing at the 3′ end of RET (C-terminus) in contrast to the 5’ end splice variants, RET 2/4 and RET 2/8, described in this study which were detected by our clinical NGS assay (Learoyd et al., 1998). The splice variants leading to the RET9 and RET51 isoforms are not covered by our clinical NGS assay. RET51 expression was higher in MTC than RET9, but both were identified in 19 patients with MTC. These RET isoforms displayed unique levels of auto-phosphorylation and had differential interactions with adaptor proteins and alternative splicing in intron 19 (Richardson et al., 2012; Ramone et al., 2019). These isoforms also displayed distinct subcellular localizations, trafficking properties, and downstream signaling; however, neither was demonstrated to play a role in MTC tumorigenesis (Mule et al., 2021).
The precise role of the detected alternate RET transcripts is unknown. It is possible that these are non-pathogenic and merely reflect altered transcriptional regulation during the carcinogenic process, perhaps reverting these cells to an earlier developmental stage, or can be attributed to the increased number of overall RET transcripts. Since the splice variants observed in the MTC cases have been observed during normal neural development, the consistent detection of these splice variants in MTC could reflect a non-oncogenic but cancer specific change in transcriptional regulation. However, the 4 cases in our study that lacked specific driver mutations but had multiple expressed RET splice variants with predicted intact kinase domains, raises the intriguing possibility that the RET splice variants themselves could represent the primary pathologic driver in these cases.
It has been demonstrated that while MTCs overexpress RET mRNA this overexpression does not play a significant role in tumorigenesis (Lian et al., 2017; Mule et al., 2021). It is possible that RET 2/4 and 2/8 are detected secondary to RET mRNA overexpression and play no significant role in tumorigenesis. However, if true, this would detract from the potential diagnostic significance for these specific splice variants.
The identification of RET splice variants in MTC represents a distinguishing genetic diagnostic feature of this tumor and provides an opportunity for a better understanding MTC pathogenesis. Further studies are needed to confirm the high prevalence of RET splice variants in MTC, to understand their cause, correlate clinically, and to investigate whether they have a silent or oncogenic role.
Data availability statement
The raw data supporting the conclusion of this article will be made available by the authors, without undue reservation.
Ethics statement
The studies involving humans were approved by the Advarra IRB (Moffitt Cancer Center). The studies were conducted in accordance with the local legislation and institutional requirements. Written informed consent for participation was not required from the participants or the participants’ legal guardians/next of kin in accordance with the national legislation and institutional requirements.
Author contributions
DS-V: Conceptualization, Data curation, Formal Analysis, Investigation, Methodology, Project administration, Visualization, Writing–original draft, Writing–review and editing. KC: Writing–review and editing. JH-P: Conceptualization, Data curation, Writing–review and editing. PC: Methodology, Software, Supervision, Validation, Writing–review and editing. JS: Data curation, Writing–review and editing. JH: Conceptualization, Investigation, Project administration, Writing–review and editing. BM: Conceptualization, Methodology, Writing–review and editing. TB: Conceptualization, Data curation, Investigation, Methodology, Project administration, Supervision, Validation, Visualization, Writing–original draft, Writing–review and editing.
Funding
The author(s) declare that no financial support was received for the research, authorship, and/or publication of this article.
Conflict of interest
Authors DS-V, KC, JH-P, PC, JS, JH, BM, and TB were employed by H. Lee Moffitt Cancer Center.
Publisher’s note
All claims expressed in this article are solely those of the authors and do not necessarily represent those of their affiliated organizations, or those of the publisher, the editors and the reviewers. Any product that may be evaluated in this article, or claim that may be made by its manufacturer, is not guaranteed or endorsed by the publisher.
References
Boichard, A., Croux, L., Al Ghuzlan, A., Broutin, S., Dupuy, C., Leboulleux, S., et al. (2012). Somatic RAS mutations occur in a large proportion of sporadic RET-negative medullary thyroid carcinomas and extend to a previously unidentified exon. J. Clin. Endocrinol. Metab. 97 (10), E2031–E2035. doi:10.1210/jc.2012-2092
Boyle, T. A., Mondal, A. K., Saeed-Vafa, D., Ananth, S., Ahluwalia, P., Kothapalli, R., et al. (2021). Guideline-adherent clinical validation of a comprehensive 170-gene DNA/RNA panel for determination of small variants, copy number variations, splice variants, and fusions on a next-generation sequencing platform in the CLIA setting. Front. Genet. 12, 503830. doi:10.3389/fgene.2021.503830
Chakravarty, D., Gao, J., Phillips, S. M., Kundra, R., Zhang, H., Wang, J., et al. (2017). OncoKB: a precision oncology knowledge base. JCO Precis. Oncol. 2017, 1–16. doi:10.1200/PO.17.00011
Chen, K., Dai, X., and Wu, J. (2015). Alternative splicing: an important mechanism in stem cell biology. World J. Stem Cells 7 (1), 1–10. doi:10.4252/wjsc.v7.i1.1
Ciampi, R., Mian, C., Fugazzola, L., Cosci, B., Romei, C., Barollo, S., et al. (2013). Evidence of a low prevalence of RAS mutations in a large medullary thyroid cancer series. Thyroid 23 (1), 50–57. doi:10.1089/thy.2012.0207
Ciampi, R., Romei, C., Ramone, T., Prete, A., Tacito, A., Cappagli, V., et al. (2019). Genetic landscape of somatic mutations in a large cohort of sporadic medullary thyroid carcinomas studied by next-generation targeted sequencing. iScience 20, 324–336. doi:10.1016/j.isci.2019.09.030
Davies, K. D., Lomboy, A., Lawernce, C. A., Yourshaw, M., Bocsi, G. T., Camidge, D. R., et al. (2019). DNA-based versus RNA-based detection of MET exon 14 skipping events in lung cancer. J. Thorac. Oncol. 14 (4), 737–741. doi:10.1016/j.jtho.2018.12.020
Dirlon, A. (2016). MET exon 14 alterations in lung cancer: exon skipping extends half-life. Clin. Cancer Res. 22 (12), 2832–2834. doi:10.1158/1078-0432.CCR-16-0229
Edge, S. B., and Compton, C. C. (2010). The American Joint Committee on Cancer: the 7th edition of the AJCC cancer staging manual and the future of TNM. Ann. Surg. Oncol. 17 (6), 1471–1474. doi:10.1245/s10434-010-0985-4
Fluge, O., Haugen, D. R., Akslen, L. A., Marstad, A., Santoro, M., Fusco, A., et al. (2001). Expression and alternative splicing of c-ret RNA in papillary thyroid carcinomas. Oncogene 20 (7), 885–892. doi:10.1038/sj.onc.1204161
Gabreski, N. A., Vaghasia, J. K., Novakova, S. S., McDonald, N. Q., and Pierchala, B. A. (2016). Exon skipping in the RET gene encodes novel isoforms that differentially regulate RET protein signal transduction. J. Biol. Chem. 291 (31), 16249–16262. doi:10.1074/jbc.M115.709675
Hundahl, S. A., Fleming, I. D., Fremgen, A. M., and Menck, H. R. (1998). A National Cancer Data Base report on 53,856 cases of thyroid carcinoma treated in the U.S., 1985-1995 [see commetns]. Cancer 83 (12), 2638–2648. doi:10.1002/(sici)1097-0142(19981215)83:12<2638::aid-cncr31>3.0.co;2-1
Learoyd, D. L., Messina, M., Zedenius, J., Guinea, A. I., Delbridge, L. W., and Robinson, B. G. (1998). RET/PTC and RET tyrosine kinase expression in adult papillary thyroid carcinomas. J. Clin. Endocrinol. Metab. 83 (10), 3631–3635. doi:10.1210/jcem.83.10.5152
Le Hir, H., Charlet-Berguerand, N., de Franciscis, V., and Thermes, C. (2002). 5'-End RET splicing: absence of variants in normal tissues and intron retention in pheochromocytomas. Oncology 63 (1), 84–91. doi:10.1159/000065725
Lian, E. Y., Maritan, S. M., Cockburn, J. G., Kasaian, K., Crupi, M. J. F., Hurlbut, D., et al. (2017). Differential roles of RET isoforms in medullary and papillary thyroid carcinomas. Endocr. Relat. Cancer 24 (1), 53–69. doi:10.1530/ERC-16-0393
Lorenzo, M. J., Eng, C., Mulligan, L. M., Stonehouse, T. J., Healey, C. S., Ponder, B. A., et al. (1995). Multiple mRNA isoforms of the human RET proto-oncogene generated by alternate splicing. Oncogene 10 (7), 1377–1383.
McIver, B., Grebe, S. K., Wang, L., Hay, I. D., Yokomizo, , Liu, W., et al. (2000). FHIT and TSG101 in thyroid tumours: aberrant transcripts reflect rare abnormal RNA processing events of uncertain pathogenetic or clinical significance. Clin. Endocrinol. (Oxf) 52 (6), 749–757. doi:10.1046/j.1365-2265.2000.01009.x
Mule, C., Ciampi, R., Ramone, T., Prete, A., Matrone, A., Cappagli, V., et al. (2021). Higher RET gene expression levels do not represent anAlternative RET activation mechanism in medullary thyroid carcinoma. Biomolecules 11 (10), 1542. doi:10.3390/biom11101542
Mulligan, L. M. (2014). RET revisited: expanding the oncogenic portfolio. Nat. Rev. Cancer 14 (3), 173–186. doi:10.1038/nrc3680
NCCN (2022). NCCN guidelines version 3.2022 thyroid carcinoma. Version 3 Accessed 01.30.2023. Available at: https://www.nccn.org/professionals/physician_gls/pdf/thyroid.pdf.
Paratala, B. S., Chung, J. H., Williams, C. B., Yilmazel, B., Petrosky, W., Williams, K., et al. (2018). RET rearrangements are actionable alterations in breast cancer. Nat. Commun. 9 (1), 4821. doi:10.1038/s41467-018-07341-4
Poirot, B., Doucet, L., Benhenda, S., Champ, J., Meignin, V., and Lehmann-Che, J. (2017). MET exon 14 alterations and new resistance mutations to tyrosine kinase inhibitors: risk of inadequate detection with current amplicon-based NGS panels. J. Thorac. Oncol. 12 (10), 1582–1587. doi:10.1016/j.jtho.2017.07.026
Puris, M. A., Geurts-Giele, W. R., Von der, T. H., Meijssen, I. C., Dinjens, W. N. M., Aerts, J. G. J. V., et al. (2020). Highly accurate DNA-based detection and treatment results of MET exon 14 skipping mutations in lung cancer. Lung Cancer 140, 46–54. doi:10.1016/j.lungcan.2019.11.010
Ramone, T., Romei, C., Ciampi, R., Tacito, A., Piaggi, P., Torregrossa, L., et al. (2019). Differential expression of RET isoforms in normal thyroid tissues, papillary and medullary thyroid carcinomas. Endocrine 65 (3), 623–629. doi:10.1007/s12020-019-01957-2
Richardson, D. S., Rodrigues, D. M., Hyndman, B. D., Crupi, M. J., Nicolescu, A. C., and Mulligan, L. M. (2012). Alternative splicing results in RET isoforms with distinct trafficking properties. Mol. Biol. Cell 23 (19), 3838–3850. doi:10.1091/mbc.E12-02-0114
Robinson, J. T., Thorvaldsdottir, H., Winckler, W., Guttman, M., Lander, E. S., Getz, G., et al. (2011). Integrative genomics viewer. Nat. Biotechnol. 29 (1), 24–26. doi:10.1038/nbt.1754
Shakiba, E., Movahedi, M., Majd, A., and Hedayati, M. (2019). Investigating the expression and promoter methylation of RET gene in patients with medullary thyroid cancer with unmutated RET. J. Cell Physiol. 234 (9), 16304–16311. doi:10.1002/jcp.28295
Thyroid (2023). Medullary-thyroid-cancer. Available at: https://www.thyroid.org/medullary-thyroid-cancer/(Accessed January 30, 2023).
Verrienti, A., Grani, G., Sponziello, M., Pecce, V., Damante, G., Durante, C., et al. (2022). Precision oncology for RET-related tumors. Front. Oncol. 12, 992636. doi:10.3389/fonc.2022.992636
Keywords: medullary thyroid carcinoma, RET, splice site variant, thyroid, endocrine
Citation: Saeed-Vafa D, Chatzopoulos K, Hernandez-Prera J, Cano P, Saller JJ, Hallanger Johnson JE, McIver B and Boyle TA (2024) RET splice site variants in medullary thyroid carcinoma. Front. Genet. 15:1377158. doi: 10.3389/fgene.2024.1377158
Received: 26 January 2024; Accepted: 08 March 2024;
Published: 19 March 2024.
Edited by:
Parmanand Malvi, University of Alabama at Birmingham, United StatesReviewed by:
Frederique Savagner, INSERM U1048 Institut des Maladies Métaboliques et Cardiovasculaires, FranceWenting Tang, Sun Yat-sen University Cancer Center (SYSUCC), China
Rengyun Liu, The First Affiliated Hospital of Sun Yat-sen University, China
Copyright © 2024 Saeed-Vafa, Chatzopoulos, Hernandez-Prera, Cano, Saller, Hallanger Johnson, McIver and Boyle. This is an open-access article distributed under the terms of the Creative Commons Attribution License (CC BY). The use, distribution or reproduction in other forums is permitted, provided the original author(s) and the copyright owner(s) are credited and that the original publication in this journal is cited, in accordance with accepted academic practice. No use, distribution or reproduction is permitted which does not comply with these terms.
*Correspondence: Daryoush Saeed-Vafa, Daryoush.Saeed-Vafa@moffitt.org