- 1College of Animal Science, Xinjiang Agricultural University, Urumqi, China
- 2Adsen Biotechnology Co., Ltd., Urumchi, China
The aim was to investigate the relationship between polymorphisms of gene mutation loci and reproductive traits in local sheep breeds (Duolang Sheep) and introduced sheep breeds (Suffolk, Hu Sheep) in Xinjiang to provide new molecular markers for the selection and breeding of high fecundity sheep. The expression pattern of typing successful genes in sheep tissues was investigated by RT-qPCR technology, providing primary data for subsequent verification of gene function. The 26 mutation loci of WWC2, ARHGEF9, SLK, GAB3, and FSHR genes were typed using KASP. Association analyses were performed using SPSS 25.0, and the typing results showed that five genes with six loci, WWC2 (g.14962207 C>T), ARHGEF9 (g.48271079 C>A), SLK (g.27107842 T>C, g.27108855 G>A), GAB3 (g.86134602 G>A), and FSHR (g.80789180 T>G) were successfully typed. The results of the association analyses showed that WWC2 (g.14962207 C>T), SLK (g.27108855 G>A), ARHGEF9 (g.48271079 C>A), and FSHR (g.80789180 T>G) caused significant or extremely significant effects on the litter size in Duolang, Suffolk and Hu Sheep populations. The expression distribution pattern of the five genes in 12 sheep reproduction-related tissues was examined by RT-qPCR. The results showed that the expression of the SLK gene in the uterus, the FSHR gene in the ovary, and the ARHGEF9 gene in hypothalamic-pituitary-gonadal axis-related tissues were significantly higher than in the tissues of other parts of the sheep. WWC2 and GAB3 genes were highly expressed both in reproductive organs and visceral tissues. In summary, the WWC2 (g.14962207 C>T), SLK (g.27108855 G>A), ARHGEF9 (g.48271079 C>A), and FSHR (g.80789180 T>G) loci can be used as potential molecular markers for detecting differences in reproductive performance in sheep. Due to variations in typing results, the SLK (g.27107842 T>C) and GAB3 (g.86134602 G>A) loci need further validation.
1 Introduction
China, as the country with the most significant amount of sheep rearing, slaughtering, and mutton output, meat sheep farming has gradually transitioned from traditional farmer farming to large-scale and industrialized agriculture, but because most of the meat sheep breeds farmed in China are mainly single lamb, with low fecundity, which increases the cost of production and the expansion of the farming scale is slow, which seriously restricts the rapid development of the meat sheep industry in China (Wang et al., 2018). As one of its most important economic traits, the reproductive performance of sheep directly determines the cost and efficiency of sheep farming production. Increasing the litter size of sheep parity is the most direct way to improve production efficiency, which is of great economic significance. Litter size is a quantitative trait that is controlled by multiple genes. Because of the complexity of the factors affecting the lambing trait and the low heritability of this trait, the genetic mechanism has not been thoroughly investigated, and the complex genetic mechanism of the lambing trait also makes the cycle of traditional selection methods to improve it long (Tao et al., 2020). Marker-assisted selection has the advantages of high efficiency, accuracy, and maneuverability, which can solve inefficiency problems and improve breeding efficiency.
The WWC2 gene is a member of the WWC family of proteins. The WWC proteins and cytoplasmic core kinase cascade work together to regulate Hippo signaling, a pathway that is critical for early embryonic development (Nishioka et al., 2009; Wicklow et al., 2014; Posfai et al., 2017). Impaired transduction inhibits apoptosis and enhances cell proliferation (Meng et al., 2016; Bae and Luo, 2018; Ma et al., 2019). The SLK gene is a member of the Ste20 family of non-receptor serine/threonine protein kinases involved in cell proliferation, migration, and terminal differentiation processes (Davis, 1993; Fanger et al., 1997). SLK genes are classified as GCK-related kinases (Kuramochi et al., 1997) and are commonly expressed in adult tissues and cell lines and developing mouse embryos (Sabourin and Rudnicki, 1999; Zhang et al., 2002). Recent studies have identified an association between the ARHGEF9 gene and seasonal estrus-related genes such as DIO2 and DIO3 in the thyroid (TH) axis (Fan et al., 2022). The ARHGEF9 gene encodes a Rho-like GTPase involved in energy metabolism (Ibaraki et al., 2018), which may be a key candidate gene affecting seasonal estrus in sheep. GAB3 gene deficient mice exhibit impaired uterine NK cell dilation associated with abnormal spiral artery remodeling and increased basal molting trophoblast invasion, leading to stillbirth, retained placenta, maternal hemorrhage, and undelivered placenta of full-term fetuses, which limits trophoblast invasion during pregnancy (Sliz et al., 2019). Follicle-stimulating hormone (FSH) is secreted by the anterior pituitary gland and plays a crucial role in reproduction (Gharib et al., 1990), it is essential for follicular growth, development, differentiation, and triggering follicular maturation and ovulation.
Molecular marker screening is an integral part of molecular breeding. SNP markers are the third generation of molecular markers and are currently dominant. KASP (Kompetitive allele specific polymerase chain reaction) technology is now an essential tool for genotyping. Originally named competitive allele specific PCR (KASP) by its developers, the method is a fluorescence detection-based variant of AS-PCR applicable to amplification results (Alvarez-Fernandez et al., 2021). KASP is primarily used in a wide range of genomic DNA samples for SNPs and locus-specific InDels for precise double allele determination for genotyping. Compared with other SNP detection methods, KASP technology has higher throughput, faster speed, lower cost, and more accurate results (Semagn et al., 2014; Landoulsi et al., 2017). Wang et al. (2022) used KASP genotyping to analyze the correlation between PPP2R5C and SLC39A5 polymorphisms and litter size in black goats on Yunshang. Kusza et al. (2018) studied 48 SNPs affecting milk production traits in goats using KASP technology. Talebi et al. (2018) analyzed the correlation between single nucleotide polymorphisms in significant prolificacy genes and litter size in Mehraban sheep. These studies validated the reliability of KASP results.
In this experiment, Duolang, Hu, and Suffolk Sheep were used as research subjects, and five reproductive performance-related genes (WWC2, SLK, ARHGEF9, GAB3, and FSHR) were obtained by using the results of the team’s previous litter size trait GWAS analysis, based on the existing WWC2, SLK, ARHGEF9, GAB3, and FSHR in Ensembl and UCSC databases, we used KASP technology to type the mutation loci, to investigate the relationship between the polymorphisms of WWC2, SLK, ARHGEF9, GAB3, and FSHR loci and the reproductive performance in different sheep populations, and to screen the key loci affecting the reproductive performance of sheep. At the same time, the expression and distribution patterns of WWC2, SLK, ARHGEF9, GAB3, and FSHR genes in 12 different reproduction-related tissues of sheep breeds in Xinjiang were investigated, which provided the preliminary primary data for further validation of gene functions. Due to different living environments, the reproductive performance of sheep varies from breed, and there are also differences within the breed. The main genes that cause differences in sheep litter size are also different. Therefore, the objectives of this study were to screen for new candidate genes and molecular markers that are most likely to affect sheep reproductive traits.
2 Materials and methods
2.1 Sample collection
The study was conducted on 300 Duolang sheep (lambing one or more lambs in a single litter), 167 Hu sheep (lambing one or more lambs in a single litter), and 100 Suffolk (lambing one or more lambs in a single litter). The Duolang sheep were from the sheep farms of Xinjiang Maigaiti Daolang Sunshine Agricultural and Animal Husbandry Science and Technology Co., Ltd. The Hu sheep were from the sheep farms of Hami Jiankun Farming Co. The Suffolk sheep were from the sheep farms of Manasi Rifa. The blood samples were collected from three breeds of sheep, and one blood sample was collected from the jugular vein of each sheep, put into vacuum blood collection tubes containing anticoagulant factor (EDTA K2), and stored at −20°C storage backup.
Taking sheep in the Xinjiang region as the test object, three healthy ewes with the same parity were selected, requiring the same feeding environment, basically the same or similar body weight, and prohibited from eating for 24 h before slaughtering and determination. In this test, the heart, liver, spleen, lungs, kidneys, uterine horns, uterus, ovary, hypothalamus, pituitary gland, brain, and cerebellum were taken as tissue samples quickly after slaughtering the sheep. Three copies were taken from each sample and then put into the liquid nitrogen immediately afterward. The subsequent determination and analysis could be done by extracting the RNA from the tissue samples.
2.2 Phenotype data collection
According to the sheep’s lambing pattern, contact the corresponding sheep farms and record the lambing data of sheep in different parity (Supplementary Table S1).
2.3 Blood DNA, tissue RNA extraction and detection
DNA was extracted from sheep blood samples (300 Duolang, 167 Hu, and 100 Suffolk sheep) using the blood/cell/tissue genomic DNA extraction kit (TIANGEN, Beijing, China), and the DNA quality was detected by 1.5% agarose gel electrophoresis. Tissue RNA was extracted using the Biomarker Cell/Tissue Total RNA Isolation Kit (Biomarker Technologies, Beijing, China). Multiskan GO (Thermo Fisher Scientific, Wilmington, MA, United States) determined RNA’s purity, and the RNA quality was detected by 1.5% agarose gel electrophoresis. The cDNA was synthesized using the TIANGEN Fastking cDNA First Strand Synthesis Kit (TIANGEN, Beijing, China).
2.4 KASP typing test
The SNP information of five genes (WWC2, SLK, ARHGEF9, GAB3, FSHR) was searched using Ensembl (https://useast.ensembl.org/index.html) and UCSC website (https://genome-asia.ucsc.edu/index.html). The 26 missense mutation sites in the five genes were selected for KASP typing, and the primers were designed using the DNAMAN 8.0 software. Biomarker Technologies Co., Ltd. (Beijing, China) synthesized the primers. Mutation sites and primers information (Table 1).
2.5 RT-qPCR
The genes that were successfully typed by KASP (WWC2, ARHGEF9, SLK, GAB3, and FSHR) were selected for RT-qPCR test, and 12 different reproduction-related tissues of sheep in Xinjiang region (heart, liver, spleen, lungs, kidneys, uterine horns, uterus, ovary, hypothalamus, pituitary gland, brain, cerebellum) were used as templates for the cDNA. The β-actin gene was used as an internal reference, and three replicates were performed for each sample. The sequences of the target genes were found using the NCBI online website (https://www.ncbi.nlm.nih.gov/) and compared in the UCSC Sheep Genome Database (https://genome-asia.ucsc.edu/cgi-bin/hgGateway) to identify the exon locations. The NCBI online website (https://www.ncbi.nlm.nih.gov/tools/primer-blast/index.cgi?LINK_LOC=BlastHome) was utilized to design six genes (WWC2, ARHGEF9, SLK, GAB3, and FSHR) for the Primers were synthesized by Xinjiang Youkang Biotechnology Co., Ltd. (Urumqi, China). Gene sequence information (Table 2).
2.6 Data analysis
(1) After successful typing of loci using the KASP typing technique, the collected production data and experimental data were statistically processed using an Excel sheet [Genotype frequency, Allele frequency, Expected heterozygosity (He), Effective allele numbers (Ne), Observed homozygosity (Ho), Polymorphic information content (PIC)]. Hardy-Weinberg equilibrium (HWE) was analyzed using the PowerMarker V3.25 chi-square test method (Liu and Muse, 2005). The relationship between genetic polymorphisms and litter size of Duolang, Suffolk, and Hu sheep was statistically analyzed using one-way ANOVA and t-test in SPSS 25.0, and the litter size was expressed as “mean ± standard deviation.”
(2) Relative quantitative analysis was performed using the 2−ΔΔCt method (Livak and Schmittgen, 2001) with Excel software, and the quantitative data were visualized by GraphPad Prism 8.0 software.
3 Result
3.1 KASP typing results of 6 loci in 3 populations
KASP typing was performed on 26 missense mutation sites of five genes (WWC2, ARHGEF9, SLK, GAB3, and FSHR). Finally, six loci of 5 genes (WWC2, ARHGEF9, SLK, GAB3, and FSHR) were successfully typed (Figure 1).
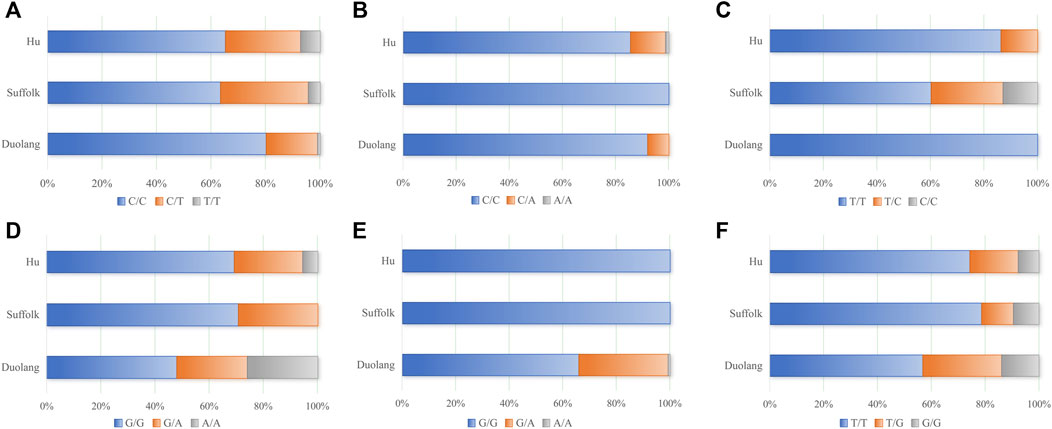
Figure 1. (A) Genotyping results of WWC2 gene (g.14962207 C>T), (B) Genotyping results of ARHGEF9 gene (g.48271079 C>A), (C) Genotyping results of SLK gene (g.27107842 T>C), (D) Genotyping results of SLK gene (g.27108855 G>A), (E) Genotyping results of GAB3 gene (g.86134602 G>A), (F) Genotyping results of FSHR gene (g.80789180 T>G).
3.2 Genetic diversity analysis of WWC2, SLK, ARHGEF9, GAB3, and FSHR genes
3.2.1 Genetic diversity analysis of the WWC2 locus in three sheep breeds
The WWC2 gene g.14962207 C>T locus was polymorphic in all three sheep populations, and the wild-type genotype frequency was higher than the heterozygous and mutant genotype frequencies in all three populations. The WWC2 gene was in Hardy-Weinberg equilibrium (p > 0.05) in the Duolang and Suffolk Sheep populations and unbalanced (p < 0.05) in the Hu Sheep populations. The g.14962207 C>T locus of the WWC2 gene showed low polymorphism (PIC<0.25) in the Duolang sheep populations and moderate polymorphism (0.25<PIC<0.5) in the Suffolk and Hu sheep populations (Table 3).
3.2.2 Genetic diversity analysis of different loci of the SLK gene in three sheep breeds
The SLK gene g.27107842 T>C locus was polymorphic in Suffolk and Hu Sheep populations, and the SLK gene g.27108855 G>A locus was polymorphic in all three sheep populations. The wild-type genotype frequency was higher than the heterozygous and mutant genotype frequencies. The SLK gene g.27107842 T>C and g.27108855 G>A loci were in Hardy-Weinberg equilibrium (p > 0.05) in Suffolk and Hu Sheep populations and were unbalanced (p < 0.05) in Duolang Sheep populations. The g.27107842 T>C locus of the SLK gene in the Hu sheep populations showed low polymorphism (PIC<0.25) and moderate polymorphism (0.25<PIC<0.5) in the Suffolk sheep populations. SLK gene g.27108855 G>A locus showed moderate polymorphism (0.25<PIC<0.5) in both Duolang and Hu sheep populations and low polymorphism (PIC<0.25) in the Suffolk sheep populations (Table 4).
3.2.3 Genetic diversity analysis of the ARHGEF9 locus in three sheep breeds
The g.48271079 C>A locus of the ARHGEF9 gene was polymorphic in Duolang and Hu Sheep populations and the frequencies of the wild-type genotypes were higher than the frequencies of heterozygous and mutant-pure genotypes. The ARHGEF9 gene was in Hardy-Weinberg equilibrium in the Duolang and Hu Sheep populations (p > 0.05). The g.48271079 C>A locus showed low polymorphism (PIC<0.25) in both the Duolang and Hu Sheep populations (Table 5).
3.2.4 Genetic diversity analysis of the GAB3 locus in three sheep breeds
The GAB3 gene g.86134602 G>A locus was polymorphic in the Duolang Sheep population, and the frequency of the wild-type genotype was higher than the heterozygous and mutant genotypes. The GAB3 gene g.86134602 G>A locus was not in Hardy-Weinberg equilibrium (p < 0.05) in the Duolang Sheep populations. The GAB3 gene g.86134602 G>A locus showed low polymorphism (PIC<0.25) in Duolang Sheep populations (Table 6).
3.2.5 Genetic diversity analysis of different loci of the FSHR gene in three sheep breeds
The FSHR gene g.80789180 T>G locus was polymorphic in all three populations, and the wild-type genotype frequency was higher than the heterozygous and mutant genotype frequency in all three populations. The g.80789180 T>G locus of the FSHR gene was in Hardy-Weinberg equilibrium in all three populations (p > 0.05). The g.80789180 T>G locus showed low polymorphism (PIC<0.25) in the Suffolk and Hu Sheep populations and moderate polymorphism (0.25<PIC<0.5) in the Duolang Sheep populations (Table 7).
3.3 Association analysis between polymorphic loci of genes and litter size in three breeds of sheep
Polymorphisms in the WWC2 gene g.14962207 C>T locus were significantly associated with the litter size in Duolang Sheep, the litter size of the wild-type CC genotype individuals was significantly higher than the heterozygous CT genotype individuals (p < 0.05). The litter size of the wild-type CC genotype individuals was significantly higher than the heterozygous CT genotype individuals (p < 0.01) and significantly higher than the mutant pure TT genotype individuals (p < 0.05) in the Suffolk Sheep populations. The SLK gene g.27107842 T>C locus in Suffolk Sheep populations, the litter size of mutant-pure CC genotype individuals was significantly higher than the wild-type TT genotype individuals and heterozygous TC genotype individuals (p < 0.01). The litter size of wild-type TT genotype individuals was significantly higher than the heterozygous TC genotype individuals in the Hu Sheep populations (p < 0.01). The SLK gene g.27108855 G>A locus in Duolang Sheep populations, the litter size of mutant-pure AA genotype individuals was significantly higher than the wild-type GG genotype individuals and heterozygous GA genotype individuals (p < 0.05), and the litter size of wild-type GG genotype individuals was significantly higher than the heterozygous GA genotype individuals in the Suffolk and Hu Sheep populations (p < 0.01). The ARHGEF9 gene g.48271079 C>A locus in Hu Sheep populations, the litter size of heterozygous CA genotype individuals was significantly higher than the wild-type CC genotype individuals (p < 0.01). The GAB3 gene g.86134602 G>A locus in Duolang Sheep populations, the litter size of mutant-pure AA genotype individuals and heterozygous GA genotype individuals was significantly higher than the wild-type GG genotype individuals (p < 0.01). The FSHR gene g.80789180 T>G locus in Duolang and Suffolk Sheep populations, the litter size of mutant-pure GG genotype individuals was significantly higher than the wild-type TT genotype individuals (p < 0.01) (Table 8).
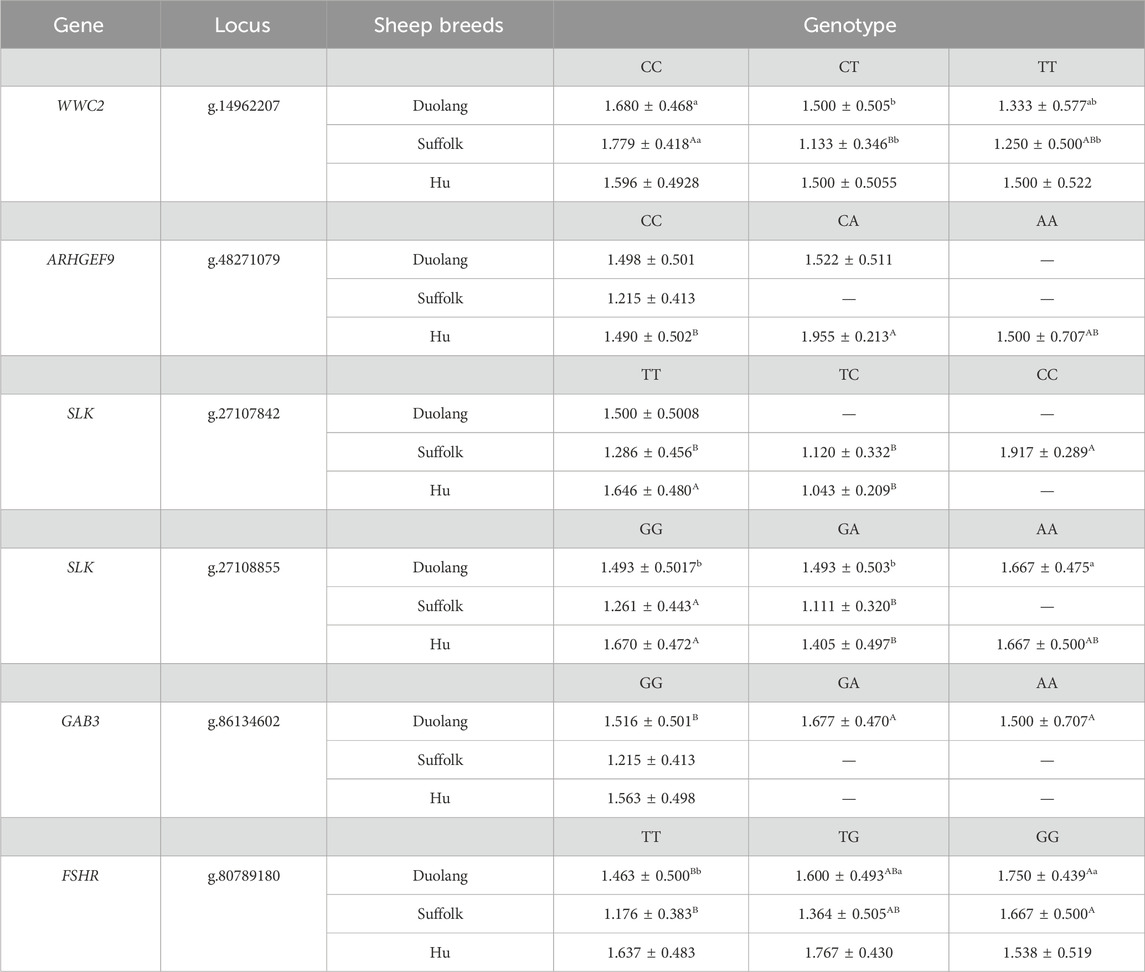
Table 8. Association analysis between different genotypes and average litter size, different uppercase letters of the shoulder mark are highly significant (p < 0.01), different lowercase shoulder tags are significantly different (p < 0.05), and the same letter is not significantly different (p > 0.05), the same as below.
3.4 Distribution pattern of differential gene expression in tissues
The expression distribution pattern of five genes (WWC2, SLK, ARHGEF9, GAB3, and FSHR) in 12 tissue samples (heart, liver, spleen, lung, kidney, uterine horn, uterus, ovary, hypothalamus, pituitary gland, brain, cerebellum) of sheep was examined by RT-qPCR. The RT-qPCR results are shown in Figure 2. Five genes (WWC2, SLK, ARHGEF9, GAB3, and FSHR) were expressed in all parts of the sheep’s tissues. The expression of the SLK gene in the uterus, the FSHR gene in the ovary, and the ARHGEF9 gene in pituitary and hypothalamus tissues were significantly higher than other parts of sheep’s tissues. The RT-qPCR results showed that SLK, FSHR, and ARHGEF9 genes were mainly explicitly expressed in reproductive organs such as the uterus and ovary and tissues related to the hypothalamic-pituitary-gonadal axis and were involved in reproductive effects.
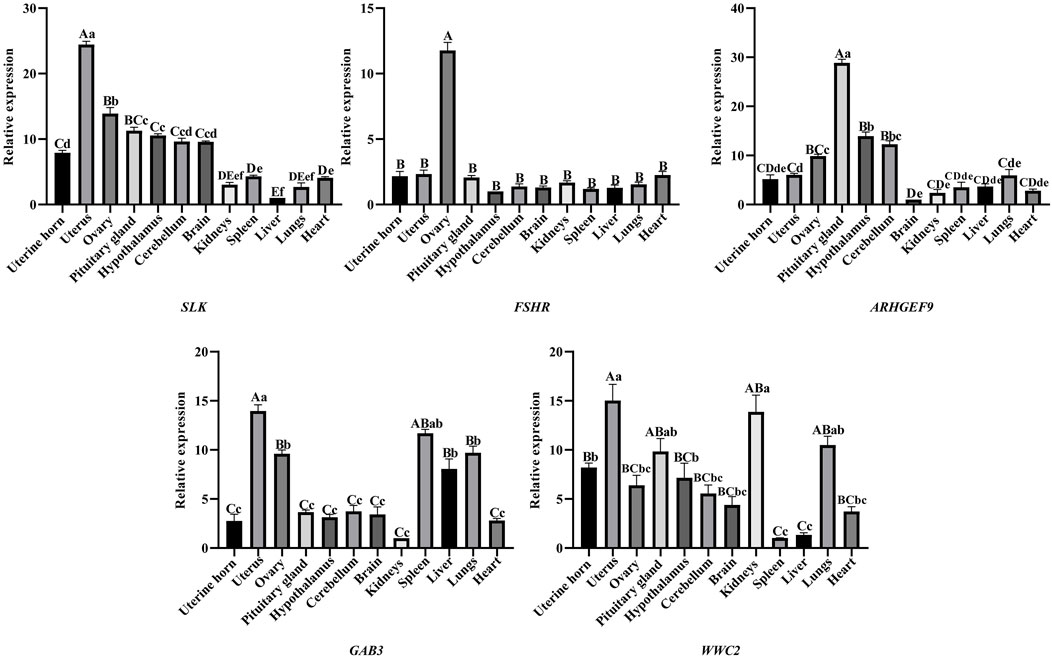
Figure 2. SLK, FSHR, ARHGEF9, GAB3, WWC2 gene expression in different organizations, different capital letters indicate highly significant between groups (p < 0.01), different lowercase letters indicate significantly different between groups (p < 0.05), and the same letters indicate non-significant different between groups (p > 0.05).
4 Discussion
4.1 Association of WWC2 gene polymorphisms with reproductive performance
Previous studies have shown that the WWC2 gene is strongly associated with reproductive performance. Hermann et al. (2021) found that WWC2 gene deletion resulted in early embryonic death in mice, and WWC2 knockout (KO) embryos exhibited growth retardation, disrupted placental development, impaired vascularization, and finally embryonic death, demonstrating that the WWC2 gene has a significant impact on cell fate determination, cell metabolism, and angiogenesis. In the present study, the relationship between genotype and litter size showed that wild-type CC genotypes in the g.14962207 C>T locus of the WWC2 gene were significantly correlated with litter size. The litter size of individuals with heterozygous and mutant-pure genotypes was significantly reduced, suggesting that mutations at this locus reduce litter size to a certain extent in the Duolang and Suffolk Sheep. Dysregulated expression of the WWC2 gene has also been found to be particularly associated with cell-autonomous defects in embryonic cell number and abnormal cell division, typified by an imbalance in chromatin segregation of daughter cells, and during meiotic maturation of mouse oocytes, dysregulation of WWC2 impedes progression of the fertilizability stage of meiosis II mid-stage arrest, leading to spindle defects and Aurora-A kinase (AURKA) activation failure (Virnicchi et al., 2020).
4.2 Association of SLK gene polymorphisms with reproductive performance
Ste20 kinase is an important signaling molecule intensively studied in yeast as a regulator of the mitogen-activated protein kinase (MAPK)-dependent pathway in controlling the mating response (Zhao et al., 1995). We obtained the SLK gene through previous screening. We found that its expression in reproductive organs such as the uterus and ovary was significantly higher than in visceral tissues such as the heart, liver, and spleen. It was hypothesized that this gene was closely related to reproductive performance in sheep. Studies have shown that the SLK gene is commonly expressed in adult tissues, cell lines, and developing mouse embryos (Sabourin and Rudnicki, 1999; Zhang et al., 2002). Song et al. (2023) found that SLK is highly expressed in mouse oocyte meiosis with upregulated phosphorylation modifications. SLK has also been shown to be required for cell cycle progression from G2 to mitosis (G2/M) by activating the polo-like kinase homolog (PLK1), an essential kinase that regulates cell cycle trafficking, and cell cycle protein-dependent kinases, and that SLK depletion results in the inability to downregulate cell cycle protein A and early G2 arrest (Ellinger-Ziegelbauer et al., 2000; O'Reilly et al., 2005). Pryce et al. (2017) found that SLK plays a critical role in embryonic development and that knockdown of the SLK gene results in embryonic lethality. Consistent with this, the SLK gene trap allele (SLK-LacZ) that results in the truncation of the ATH structural domain SLK was lethal to purebred mouse embryos (Al-Zahrani et al., 2014).
4.3 Association of ARHGEF9 gene polymorphisms with reproductive performance
Based on the association analysis results, we found a significant association between the ARHGEF9 gene and sheep’s reproductive performance. We hypothesized that this gene may be one of the critical genes affecting sheep’s reproductive performance. The protein encoded by the ARHGEF9 (guanine nucleotide exchange factor 9) gene is a Rho-like GTPase that switches between an active (GTP-bound) state and an inactive (GDP-bound) state that is important for energy metabolism (Ibaraki et al., 2018). Previous studies have shown that the ARHGEF9 gene is associated with critical genes DIO2, DIO3, TSHB, and TSHR in the thyroid (TH) axis pathway, which may be related to the promotion of estrus (Malpaux et al., 2001; Fan et al., 2022). The thyroid (TH) axis is essential for seasonal reproduction in sheep, and thyrotropin secreted by the nodal section (PT) is the major photoperiod-dependent upstream regulator of (DIO2/DIO3) gene expression. Long sunlight promotes thyrotropin production, increasing type II thyroid hormone deiodinase (DIO2) and inhibiting DIO3 expression, which enhances TH signaling in the MBH. In contrast, short sunlight has the opposite effect. Overall, the TH axis directs seasonal reproduction through thyroid hormone (TH) reciprocal regulation of TH deiodinase (DIO2/DIO3) gene expression in stretch cells in the medial ventricular zone (MBH) of the hypothalamus (Goldman, 2001; Hanon et al., 2008). Our study selected seasonal rutting sheep (Duolang and Suffolk Sheep) and year-round rutting sheep (Hu Sheep) as test subjects. We used the KASP technique to type the mutant locus of the ARHGEF9 gene and then found differences in the typing results of the gene in the three populations. Maybe due to breed or geographical differences, only the wild-type CC genotype was typed in the Suffolk Sheep populations. In the Duolang Sheep populations, two genotypes (wild-type CC and heterozygous CA) were typed. Three genotypes (wild-type CC, heterozygous CA, and mutant-pure AA genotypes) were typed in the Hu Sheep populations. The ARHGEF9 gene did not appear in the mutant-pure individual in the seasonally rutting sheep population. In contrast, the mutant-pure AA genotype individual appeared in the year-round rutting Hu Sheep populations, and we hypothesized that it might be due to this pure mutation that causes year-round estrus in the Hu Sheep populations. Currently, there are fewer studies on the role of the ARHGEF9 gene in sheep reproductive performance. The regulatory mechanism is unclear and its function needs to be further verified.
5 Conclusion
Breeding high fecundity sheep is very important for sheep meat production and molecular marker screening can be used to improve early molecular selection, to make breakthroughs in the development of genes and markers for multiple births in sheep, to improve the efficiency of molecular breeding for high fecundity sheep, and also to improve other low yielding populations to increase the efficiency of meat production in sheep. The present study verified the role of five genes. Among the five selected genes, the WWC2 gene g.14962207 C>T locus, the SLK gene g.27108855 G>A locus, the ARHGEF9 gene g.48271079 C>A locus, and the FSHR gene g.80789180 T>G locus were identified as having significant molecular markers among different genotypes. These four loci can be used to detect the local sheep populations in Xinjiang (Duolang Sheep) and introduced sheep populations (Suffolk and Hu Sheep) in terms of litter size. This finding provides new information to explain sheep fertility’s regulatory mechanisms and identify molecular markers for litter size traits. These molecular markers are essential for the selection of high fecundity sheep breeds.
Data availability statement
The original contributions presented in the study are included in the article/Supplementary Material, further inquiries can be directed to the corresponding authors.
Ethics statement
The animal study was approved by The University of Xinjiang Agricultural University approved the use of its facilities for the study (Animal protocol number: 2017010). The study was conducted in accordance with the local legislation and institutional requirements.
Author contributions
MT: Writing–review and editing, Writing–original draft, Validation, Software, Data curation. ZL: Writing–review and editing, Software, Methodology. ML: Writing–review and editing, Visualization, Validation. HM: Writing–review and editing, Supervision, Methodology. WL: Writing–review and editing, Supervision, Methodology.
Funding
The author(s) declare that financial support was received for the research, authorship, and/or publication of this article. This research was funded by the National 14th Five-Year Plan Key Research and Development Program (2021YFD1600702) and the Autonomous Region Natural Science Foundation Youth Fund Project (2022D01B84).
Acknowledgments
The authors thank the staff who supported the study’s progress, the reviewers, and the editors for their helpful comments and suggestions on this paper.
Conflict of interest
Authors MT and ZL were employed by Adsen Biotechnology Co., Ltd.
The remaining authors declare that the research was conducted in the absence of any commercial or financial relationships that could be construed as a potential conflict of interest.
Publisher’s note
All claims expressed in this article are solely those of the authors and do not necessarily represent those of their affiliated organizations, or those of the publisher, the editors and the reviewers. Any product that may be evaluated in this article, or claim that may be made by its manufacturer, is not guaranteed or endorsed by the publisher.
Supplementary material
The Supplementary Material for this article can be found online at: https://www.frontiersin.org/articles/10.3389/fgene.2024.1371872/full#supplementary-material
References
Alvarez-Fernandez, A., Bernal, M. J., Fradejas, I., Ramírez, A., Yusuf, N. A., Lanza, M., et al. (2021). KASP: a genotyping method to rapid identification of resistance in Plasmodium falciparum. Malar. J. 20 (1), 16. doi:10.1186/s12936-020-03544-7
Al-Zahrani, K. N., Sekhon, P., Tessier, D. R., Yockell-Lelievre, J., Pryce, B. R., Baron, K. D., et al. (2014). Essential role for the SLK protein kinase in embryo genesis and placental tissue development. Dev. Dynam. 243 (5), 640–651. doi:10.1002/dvdy.24106
Bae, S. J., and Luo, X. (2018). Activation mechanisms of the Hippo kinase signaling cascade. Biosci. Rep. 38 (4), BSR20171469. doi:10.1042/BSR20171469
Davis, R. J. (1993). The mitogen-activated protein kinase signal transduction pathway. J. Biol. Chem. 268 (20), 14553–14556. doi:10.1016/s0021-9258(18)82362-6
Ellinger-Ziegelbauer, H., Karasuyama, H., Yamada, E., Tsujikawa, K., Todokoro, K., and Nishida, E. (2000). Ste20-like kinase (SLK), a regulatory kinase for polo-like kinase (Plk) during the G2/M transition in somatic cells. Genes cells. 5 (6), 491–498. doi:10.1046/j.1365-2443.2000.00337.x
Fan, Y. K., Di, R., Liu, Z. Y., Li, W. T., Zhang, W. F., Zu, M. X., et al. (2022). Validation of key miRNAs and their target genes for seasonal estrus in the pituitary gland of Sunit sheep. Chin. J. Anim. Sci. 58 (12), 109–115. doi:10.19556/j.0258-7033.20211201-02
Fanger, G. R., Gerwins, P., Widmann, C., Jarpe, M. B., and Johnson, G. L. (1997). MEKKs, GCKs, MLKs, PAKs, TAKs, and tpls: upstream regulators of the c-Jun amino-terminal kinases? Curr. Opin. Genet. Dev. 7 (1), 67–74. doi:10.1016/s0959-437x(97)80111-6
Gharib, S. D., Wierman, M. E., Shupnik, M. A., and Chin, W. W. (1990). Molecular biology of the pituitary gonadotropins. Endocr. Rev. 11 (1), 177–199. doi:10.1210/edrv-11-1-177
Goldman, B. D. (2001). Mammalian photoperiodic system: formal properties and neuroendocrine mechanisms of photoperiodic time measurement. J. Biol. Rhythm. 16 (4), 283–301. doi:10.1177/074873001129001980
Hanon, E. A., Lincoln, G. A., Fustin, J. M., Dardente, H., Masson-Pévet, M., Morgan, P. J., et al. (2008). Ancestral TSH mechanism signals summer in a photoperiodic mammal. Curr. Biol. 18 (15), 1147–1152. doi:10.1016/j.cub.2008.06.076
Hermann, A., Wu, G., Nedvetsky, P. I., Brücher, V. C., Egbring, C., Bonse, J., et al. (2021). The Hippo pathway component Wwc2 is a key regulator of embryonic development and angiogenesis in mice. Cell Death Dis. 12 (1), 117. doi:10.1038/s41419-021-03409-0
Ibaraki, K., Mizuno, M., Aoki, H., Niwa, A., Iwamoto, I., Hara, A., et al. (2018). Biochemical and morphological characterization of a guanine nucleotide exchange factor ARHGEF9 in mouse tissues. Acta histochem. Cytoc 51 (3), 119–128. doi:10.1267/ahc.18009
Kuramochi, S., Moriguchi, T., Kuida, K., Endo, J., Semba, K., Nishida, E., et al. (1997). LOK is a novel mouse STE20-like protein kinase that is expressed predominantly in lymphocytes. J. Biol. Chem. 272 (36), 22679–22684. doi:10.1074/jbc.272.36.22679
Kusza, S., Cziszter, L. T., Ilie, D. E., Sauer, M., Padeanu, I., and Gavojdian, D. (2018). Kompetitive Allele Specific PCR (KASP™) genotyping of 48 polymorphisms at different caprine loci in French Alpine and Saanen goat breeds and their association with milk composition. PeerJ 6, e4416. doi:10.7717/peerj.4416
Landoulsi, Z., Benromdhan, S., Djebara, M., Damak, M., Dallali, H., Kefi, R., et al. (2017). Using KASP technique to screen LRRK2 G2019S mutation in a large Tunisian cohort. BMC Med. Genet. 18, 70. doi:10.1186/s12881-017-0432-5
Liu, K., and Muse, S. V. (2005). PowerMarker: an integrated analysis environment for genetic marker analysis. Bioinformatics 21 (9), 2128–2129. doi:10.1093/bioinformatics/bti282
Livak, K. J., and Schmittgen, T. D. (2001). Analysis of relative gene expression data using Real-Time quantitative PCR and the 2−ΔΔCT method. Methods 25 (4), 402–408. doi:10.1006/meth.2001.1262
Ma, S., Meng, Z., Chen, R., and Guan, K. L. (2019). The Hippo pathway: biology and pathophysiology. Annu. Rev. Biochem. 88, 577–604. doi:10.1146/annurev-biochem-013118-111829
Malpaux, B., Migaud, M., Tricoire, H., and Chemineau, P. (2001). Biology of mammalian photoperiodism and the critical role of the pineal gland and melatonin. J. Biol. Rhythm. 16 (4), 336–347. doi:10.1177/074873001129002051
Meng, Z., Moroishi, T., and Guan, K. L. (2016). Mechanisms of Hippo pathway regulation. Gene. Dev. 30 (1), 1–17. doi:10.1101/gad.274027.115
Nishioka, N., Inoue, K. I., Adachi, K., Kiyonari, H., Ota, M., Ralston, A., et al. (2009). The Hippo signaling pathway components Lats and Yap pattern Tead4 activity to distinguish mouse trophectoderm from inner cell mass. Dev. Cell 16 (3), 398–410. doi:10.1016/j.devcel.2009.02.003
O'Reilly, P. G., Wagner, S., Franks, D. J., Cailliau, K., Browaeys, E., Dissous, C., et al. (2005). The ste20-like kinase SLK is required for cell cycle progression through g2. J. Biol. Chem. 280 (51), 42383–42390. doi:10.1074/jbc.M510763200
Posfai, E., Petropoulos, S., de Barros, F. R. O., Schell, J. P., Jurisica, I., Sandberg, R., et al. (2017). Position-and Hippo signaling-dependent plasticity during lineage segregation in the early mouse embryo. Elife 6, e22906. doi:10.7554/eLife.22906
Pryce, B. R., Al-Zahrani, K. N., Dufresne, S., Belkina, N., Labrèche, C., Patino-Lopez, G., et al. (2017). Deletion of the Ste20-like kinase SLK in skeletal muscle results in a progressive myopathy and muscle weakness. Skelet. Muscle 7, 3. doi:10.1186/s13395-016-0119-1
Sabourin, L. A., and Rudnicki, M. A. (1999). Induction of apoptosis by SLK, a Ste20-related kinase. Oncogene 18 (52), 7566–7575. doi:10.1038/sj.onc.1203119
Semagn, K., Babu, R., Hearne, S., and Olsen, M. (2014). Single nucleotide polymorphism genotyping using Kompetitive Allele Specific PCR (KASP): overview of the technology and its application in crop improvement. Mol. Breed. 33, 1–14. doi:10.1007/s11032-013-9917-x
Sliz, A., Locker, K. C. S., Lampe, K., Godarova, A., Plas, D. R., Janssen, E. M., et al. (2019). Gab3 is required for IL-2- and IL-15-induced NK cell expansion and limits trophoblast invasion during pregnancy. Sci. Immunol. 4 (38), eaav3866. doi:10.1126/sciimmunol.aav3866
Song, K., Jiang, X., Xu, X., Chen, Y., Zhang, J., Tian, Y., et al. (2023). Ste20-like kinase activity promotes meiotic resumption and spindle microtubule stability in mouse oocytes. Cell Proliferat 56 (4), e13391. doi:10.1111/cpr.13391
Talebi, R., Ahmadi, A., Afraz, F., Sarry, J., Woloszyn, F., and Fabre, S. (2018). Detection of single nucleotide polymorphisms at major prolificacy genes in the Mehraban sheep and association with litter size. Ann. Anim. Sci. 18 (3), 685–698. doi:10.2478/aoas-2018-0014
Tao, L., He, X. Y., Jiang, Y. T., Lan, R., Li, M., Li, Z. M., et al. (2020). Combined approaches to reveal genes associated with litter size in Yunshang black goats. Anim. Genet. 51 (6), 924–934. doi:10.1111/age.12999
Virnicchi, G., Bora, P., Gahurova, L., Šušor, A., and Bruce, A. W. (2020). Wwc2 is a novel cell division regulator during preimplantation mouse embryo lineage formation and oogenesis. Front. Cell Dev. Biol. 8, 857. doi:10.3389/fcell.2020.00857
Wang, J. Y., Zhou, P., Wang, J., Tang, B., Su, T., Liu, X. R., et al. (2018). ARHGEF9 mutations in epileptic encephalopathy/intellectual disability: toward understanding the mechanism underlying phenotypic variation. Neurogenetics 19 (1), 9–16. doi:10.1007/s10048-017-0528-2
Wang, P., Li, W., Liu, Z., He, X., Lan, R., Liu, Y., et al. (2022). Analysis of the association of two SNPs in the promoter regions of the PPP2R5C and SLC39A5 genes with litter size in yunshang black goats. Animals-Basel 12 (20), 2801. doi:10.3390/ani12202801
Wicklow, E., Blij, S., Frum, T., Hirate, Y., Lang, R. A., Sasaki, H., et al. (2014). HIPPO pathway members restrict SOX2 to the inner cell mass where it promotes ICM fates in the mouse blastocyst. Plos Genet. 10 (10), e1004618. doi:10.1371/journal.pgen.1004618
Zhang, Y. H., Hume, K., Cadonic, R., Thompson, C., Hakim, A., Staines, W., et al. (2002). Expression of the Ste20-like kinase SLK during embryonic development and in the murine adult central nervous system. Dev. Brain Res. 139 (2), 205–215. doi:10.1016/s0165-3806(02)00551-5
Keywords: sheep, fecundity, litter size, KASP, SNP, RT-qPCR
Citation: Tao M, Li Z, Liu M, Ma H and Liu W (2024) Association analysis of polymorphisms in SLK, ARHGEF9, WWC2, GAB3, and FSHR genes with reproductive traits in different sheep breeds. Front. Genet. 15:1371872. doi: 10.3389/fgene.2024.1371872
Received: 17 January 2024; Accepted: 01 April 2024;
Published: 12 April 2024.
Edited by:
Aline Silva Mello Cesar, University of São Paulo, BrazilReviewed by:
Rui Su, Inner Mongolia Agricultural University, ChinaShamik Polley, West Bengal University of Animal and Fishery Sciences, India
Copyright © 2024 Tao, Li, Liu, Ma and Liu. This is an open-access article distributed under the terms of the Creative Commons Attribution License (CC BY). The use, distribution or reproduction in other forums is permitted, provided the original author(s) and the copyright owner(s) are credited and that the original publication in this journal is cited, in accordance with accepted academic practice. No use, distribution or reproduction is permitted which does not comply with these terms.
*Correspondence: Wujun Liu, wujunliu1026@xjau.edu.cn; Haiyu Ma, haiyu_ma@163.com