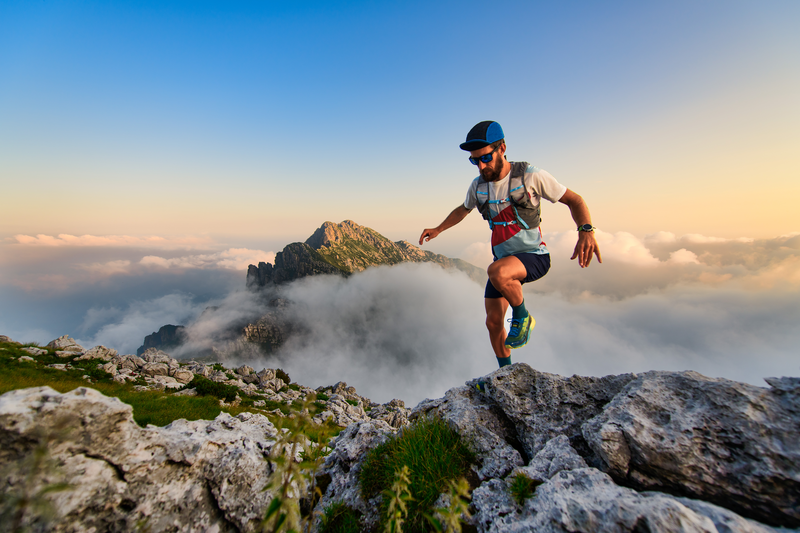
95% of researchers rate our articles as excellent or good
Learn more about the work of our research integrity team to safeguard the quality of each article we publish.
Find out more
SYSTEMATIC REVIEW article
Front. Genet. , 28 February 2024
Sec. Cancer Genetics and Oncogenomics
Volume 15 - 2024 | https://doi.org/10.3389/fgene.2024.1302527
Lung cancer is a crucial global issue, with more than one million deaths annually. While smoking is considered the main etiology of the disease, several genetic variants are associated with it. Alterations in vitamin D pathway genes have also been studied in regards to lung cancer, but the findings have been inconclusive. We here present a systematic review and meta-analysis of seven genes in this pathway: CYP2R1, CYP27B1, CYP24A1, CYP3A4, CYP3A5, GC, and VDR. Four databases (PubMed, Scopus, Cochrane Library, and Web of Science (WOS) databases) were searched. From these, 16 eligible case–control studies comprising 6,206 lung cancer cases and 7,272 health controls were obtained. These studies were subjected to comprehensive data extraction and quality scoring, and the pooled odds ratio with a 95% confidence interval was calculated to estimate the effect of each variant along with heterogeneity analysis and a risk of bias assessment. Our meta-analysis revealed an association between CYP3A4 (rs2740574) and lung cancer in the allelic, heterozygous, and dominant models. In addition, both VDR (Fok1: rs2228570) and VDR (Cdx-2: rs11568820) displayed a protective role in lung cancer development in the heterozygous and dominant models. Furthermore, VDR (Taq1: rs731236) showed a decreased risk of lung cancer in the allelic, homozygous, and recessive models. Similarly, VDR (BsmI: rs1544410) had a positive effect on lung cancer risk when subjected to allelic and recessive models. Our meta-analysis revealed the lack of association of CYP2R1 (rs10741657), CYP27B1 (rs3782130), CYP27B1 (rs10877012), CYP24A1 (rs6068816), CYP24A1 (rs4809960), CYP3A5 (rs776746), GC (rs7041), GC (rs4588), and VDR (ApaI: rs7975232) with lung cancer. Our work revealed that CYP3A4 (rs2740574) can represent an independent risk factor for lung cancer. This conclusion can aid better personalized medicine for lung cancer management, while further assessment for genetic variants of CYP3A4, CYP27B1, CYP24A1, GC, and VDR is still required to address more robust evidence.
Lung cancer is the most robust lethal carcinoma worldwide, accounting for 1.76 million deaths annually (Bade and Dela Cruz, 2020). Small-cell lung cancer (SCLC) and non-small-cell lung cancer (NSCLC) are two primary histological subtypes of lung cancer. NSCLC accounts for 80%–85% of lung cancer cases and includes adenocarcinoma, squamous cell carcinoma, and large-cell carcinoma (Ben et al., 2021). Smoking has been identified as the leading factor contributing to the incidence of lung cancer. Other risk factors include viral infections, exposure to heavy metals, radiation, asbestos, and air pollution (Li et al., 2019). However, these etiologies rarely account for lung cancer, suggesting that genetic factors play a significant role in its incidence (Zhu et al., 2021). Genome-wide association studies (GWASs) in populations from Europe and Asia have conclusively proven the relationship between genetic polymorphisms and the risk of developing lung cancer (Zhou et al., 2020). Therefore, the identification of vulnerable genes and high-risk populations is one of the main goals of lung cancer research for achieving early prevention and treatment (Wei et al., 2021).
Vitamin D is a seco-steroidal prohormone which is synthesized and metabolized by a series of reactions catalyzed by several enzymes. First, pro-vitamin D, absorbed from food or produced in the skin after exposure to sunlight, is converted by the vitamin D 25-hydroxylase enzyme CYP2R1 in the liver (Janoušek et al., 2022). A summary of this process is shown in Figure 1. The 25(OH) D produced is then converted into 1,25-dihydroxyvitamin D [1.25(OH)2D3] by the 25-hydroxyvitamin D-1α-hydroxylase enzyme that is encoded by the CYP27B1 gene in the kidney. Additionally, 25(OH)D3 can also be converted into 24.25(OH)2D3 by hydroxylation at C-24 through the mitochondrial inner membrane enzyme 1,25-dihydroxyvitamin D (3) 24-hydroxylase encoded by the CYP24A1 gene (Christakos et al., 2015).
FIGURE 1. Genes involved in the synthesis and metabolism of vitamin D. UV light, ultraviolet light; GC, group-specific component; VDR, vitamin D receptor.
Cytochrome P450 3A4 is a microsomal enzyme encoded by the CYP3A4 gene that plays a plethora of roles in the xenobiotic transformation process of many drugs and endogenous substances. It is involved in the conversion of vitamin D metabolites into their corresponding inactive molecules, such as 4β,25-OH-D3. Additionally, it activates 23R- and 24S-mediated conversion of 1α,25(OH)2D3 into inactive 1α,23R,25(OH)2D3 and 1α,24S,25(OH)2D3 (Jones et al., 2014). Thus, CYP3A4 was extensively studied in the metabolism of vitamin D (Wang et al., 2012; Piotrowska et al., 2019; Kasarla et al., 2022a). Nevertheless, vitamin D inactivation is mainly dependent on CYP3A4, and the cytochrome P450 3A5 enzyme encoded by the CYP3A5 gene catalyzes 23- or 24-hydroxylation of 1,25-(OH)2D3 (Xu et al., 2006; Klyushova et al., 2022).
Additionally, 1,25-dihydroxyvitamin D (3) 24-hydroxylase encoded by the CYP24A1 gene is considered a factor in the vitamin D transformation process and participates in the degradation process of both 25-OH-D3 and 1,25-(OH)2D3 into 24-hydroxylated products in a tissue-dependent manner (Jones et al., 2012; Wang et al., 2013). In fact, it has been suggested that alterations in the vitamin D metabolism result mainly from the induction of hepatic P450 enzymes, including CYP3A4 and CYP24A1 (Wang et al., 2013). Furthermore, in the case of vitamin D toxicity, the liver microsomal enzymes CYP3A4 and CYP3A5 and the extrarenal enzyme CYP24A1 promote the hydroxylation of vitamin D3 into inactive metabolites (Klyushova et al., 2022; Kasarla et al., 2022b). Vitamin D-binding proteins encoded by the GC gene transport the active metabolite 1.25- (OH)2D3 to target tissues where it can bind to the vitamin D receptor gene VDR and regulate physiological genes (Kasarla et al., 2022b).
Vitamin D is involved in a variety of cellular processes such as proliferation, differentiation, metastasis, angiogenesis, and apoptosis (Wei et al., 2018). Vitamin D levels have been linked to the risk of developing several cancers, including breast (Kim and Je, 2014), colorectal (Garland and Gorham, 2017), and prostate (Gilbert et al., 2011). Additionally, a previous meta-analysis has reported an inverse association between serum vitamin D levels and lung cancer risk (Zhang et al., 2015a). Furthermore, it has been found that vitamin D could suppress the metastatic growth of lung cancer cells in animal models (Nakagawa et al., 2005; Zhang et al., 2015b). Therefore, results from previous reports have revealed the potentially preventive role of vitamin D against lung cancer.
Genetic variations in vitamin D genes are potential modulators of their enzymatic functions, levels of expression, and subsequent roles in the susceptibility, progression, and prognosis of lung cancer (Pineda Lancheros et al., 2021; Pineda Lancheros et al., 2022). CYP2R1 gene polymorphisms are linked to an increased risk of NSCLC mortality, particularly in elderly NSCLC patients who have not received treatment. Furthermore, the prognosis of NSCLC may be impacted by several genetic variants connected to the Vitamin D pathway (Kong et al., 2020). Furthermore, the activity of CYP27B1 has been found to be attenuated among some small cell and non-small cell cancer cell lines (Hansdottir et al., 2008). CYP3A4 and CYP3A5 activities as part of liver machinery P450 protein content in the liver account for the metabolism of more than 50% of all drugs and exogenous carcinogens (Ingelman-Sundberg et al., 2007). CYP3A4 and CYP3A5 affect the activation of benzo [a]pyrene (B [a]P), N9-nitrosonornicotine (NNN), aflatoxin B1, stergmatocystin, alpha-hydroxytamoxifen, and procarcinogens present in tobacco smoke (Islam et al., 2014). Altered levels of these toxins are thought to be involved in lung cancer pathogenesis. Increased VDR expression in lung cancer has been correlated with improved survival (Salama et al., 2022). Genetic variants within the VDR gene might potentially influence the binding of 1,25(OH)2D and subsequently alter vitamin D levels and lung cancer pathogenesis (Haznadar et al., 2018). Nevertheless, despite research into the association between vitamin D gene pathway variants and lung cancer, much is still unknown due to a lack of conclusive findings. So this work extensively investigated the genetic variations in the vitamin D pathway, specifically the polymorphisms of CYP2R1, CYP27B1, CYP24A1, CYP3A4, CYP3A5, GC, and VDR genes, to determine their connection with increased risk of developing lung cancer.
We conducted a systematic review and meta-analysis in accordance with meta-analysis of observational studies in epidemiology (MOOSE) guidelines (Stroup et al., 2000). The study results were reported following Preferred Reporting Items for Systematic Reviews and Meta-Analyses protocols (PRISMA-2020) (Page et al., 2021). A comprehensive literature search of PubMed, Scopus, the Cochrane Library, and Web of Science (WOS) databases to 1 January 2023 was conducted by two authors independently for all relevant articles on the effects of the CYP2R1, CYP27B1, CYP24A1, CYP3A4, CYP3A5, GC, and VDR polymorphisms on lung cancer risk using the following search strategy: (“lung” or “pulmonary”) and (“cancer,” “tumor,” “neoplasm,” or “carcinoma”) and (“polymorphism,” “polymorphic,” “variation,” “variant,” “mutant,” “mutation,” “SNP,” “genotypic,” “genotype,” “allelic,” or “allele”) and (“CYP24A1,” “cytochrome P450 family 24 subfamily A member 1,” “P450-CC24,” “CYP24,” “CP24,” “23-OHase,” “rs6068816,” “rs4809957,” “vitamin D-binding protein,” “vitamin D binding protein,” “Gc-MAF,” “GC,” “DBP,” “VDBP,” “rs7041,” “rs4588,” “CYP2R1,” “25-hydroxylase,” “cytochrome P450 2R1,” “CYP27B1,” “25 hydroxyvitamin D3 1 alpha hydroxylase,” “25-hydroxycholecalciferol 1-hydroxylase,” “calcidiol 1 monooxygenase,” “calcidiol 1-monooxygenase,” “25-hydroxyvitamin D 1-alpha-hydroxylase,” “25 hydroxyvitamin D 1 alpha hydroxylase,” “25-hydroxyvitamin D2 1-hydroxylase,” “1-hydroxylase, 25-hydroxyvitamin D2,” “25 hydroxyvitamin D2 1 hydroxylase,” “cytochrome P-450 CYP27B1,” “cytochrome P 450 CYP27B1,” “25-hydroxycholecalciferol-1-hydroxylase,” “25 hydroxyvitamin D3-1-alpha hydroxylase,” “25-hydroxyvitamin D3 1 alpha-hydroxylase,” “EC 1.14.15.18,” “CYP3A5,” “cytochrome P450, family 3, subfamily A, polypeptide 5,” “cytochrome P450-PCN3,” “cytochrome P450 3A5,” “CYP3A4,” “cytochrome P450 family 3 subfamily A member 4,” “CYP3A3” “cytochrome P450, family 3, subfamily A, polypeptide 4,” “cholesterol 25-hydroxylase,” “albendazole sulfoxidase,” “cytochrome P450 3A4,” “cytochrome P450 3A3,” “nifedipine oxidase,” “EC 1.14.14.1,” “CYPIIIA4,” “taurochenodeoxycholate 6-alpha-hydroxylase,” “glucocorticoid-inducible P450,” “EC 1.14.14.55,” “P450PCN1,” “CYP3A,” “NF-25,” “VDDR3,” “CP33,” “CP34,” “HLP,” “vitamin D receptor,” “vitamin D3 receptor,” “calcitriol receptor,” “1,25-dihydroxyvitamin D3 receptor,” “VDR,” “FokI,” “BsmI,” “ApaI,” or “TaqI”). References cited in recruited articles were manually searched for additional eligible studies. When multiple publications reported the same or overlapping data, we only recruited the publication with complete data or the largest population.
The following inclusion criteria were used to identify the eligible studies for our meta-analysis: 1) case–control studies evaluated the potential association between the CYP2R1, CYP27B1, CYP24A1, CYP3A4, CYP3A5, GC, or VDR polymorphisms and lung risk; 2) studies with sufficient data to calculate an odds ratio (OR) with 95% confidence interval (95% CI); 3) original full-text articles. Otherwise, studies were excluded if they were 1) review articles, meta-analyses, letters, case reports, or articles with abstract only; 2) articles without controls; 3) non-human studies; 4) had duplicated or republished data.
Two authors independently reviewed and extracted the following baseline information for all included studies: first author’s name, year of publication, country of origin, geographical distribution, source of control [hospital-based (HB) or population-based (PB)], mean/median age of cases and controls, sample size of cases and control, classification of lung cancer, genotyping method, allelic/genotype frequencies of cases and controls, matching criteria, and quality control of genotyping. Potential conflicts were resolved by discussion with two other authors to reach a final consensus. Each study was given quality scores based on certain criteria (Supplementary Table S1) (Xu et al., 2015).
First, the allelic and genotypic frequencies of eligible studies were extracted and calculated from the selected records. HWE within cancer-free controls was calculated by the chi-squared method with a p-value >0.05 representing equilibrium between subjects (Elsalahaty et al., 2023). Next, the crude odds ratios (ORs) and their 95% confidence intervals (CI) were processed to assess the association of included variants with the risk of lung cancer. Different genetic association models were performed, including the allelic, homozygous, heterozygous, dominant, and recessive models (Elshazli et al., 2018). Heterogeneity testing amongst eligible reports was performed using the Q-statistic test along with the I2 index, with a p-value for the Q-test ≤0.10 and I2 index >50%, which signified strong heterogeneity between studies; hence, the random-effects model was chosen (Higgins et al., 2003; El Awady et al., 2022). Publication bias was evaluated using Egger’s regression method and Begg’s funnel plot to ensure a symmetric fashion; the p-value <0.05 was considered significant. We conducted a sensitivity analysis by excluding any included case–control study at a time and then recalculated the significance of the results to detect whether our outcomes were significantly impacted by the presence of each individual study. The present meta-analysis was performed using Comprehensive Meta-analysis version 3.0 (Brüggemann and Rajguru, 2022).
Depending on the keyword-based searching on databases, we acquired 1,769 records from the electronic databases (Figure 2). We removed 103 records due to duplication. The remaining 1,666 were subjected to title and abstract screening, thus excluding 60 records. The 1,606 records were independently screened by two authors, leading to 15 being selected for their eligibility as well as one record obtained from the references of included articles (Figure 2). The research in the 16 articles was performed in ten countries: China (Kong et al., 2015; Wu et al., 2016; Qu et al., 2019; Xiong et al., 2020; Jia et al., 2020), Germany (Dally et al., 2004; Timofeeva et al., 2009), Turkey (Dogan et al., 2009; Çiçek et al., 2017), Norway (Zienolddiny et al., 2008), Bangladesh (Islam et al., 2014), Tunisia (Kaabachi et al., 2014), Thailand (Maneechay et al., 2015), Poland (Gromowski et al., 2017), United States of America (Haznadar et al., 2018), and Spain (Pineda Lancheros et al., 2022). The 16 case–control studies included 6,206 lung cancer cases along with 7,272 healthy controls. The main characteristics of the included studies are presented in Table 1. Additionally, comprehensive data extraction of genotypic and allelic frequencies, along with quality scores for the 16 studies, is summarized in Supplementary Table S2.
FIGURE 2. Preferred Reporting Items for Systematic Reviews and Meta-Analyses protocols (PRISMA) flow diagram of the search strategy.
All eligible variants for meta-analysis (≥2 records) were subjected to a five-heredity-model-pooled meta-analysis (Duan et al., 2020) to assess their association with lung cancer. Significant gene variants are depicted in Table 2. Our work addressed statistical significance regarding CYP24A1 (rs4809957) with risk of lung cancer under a homozygous model [AA versus GG, OR = 1.788, 95% CI = 1.172–2.727, p-value = 0.007] (Table 2; Supplementary Figure S1A). However, it revealed a protective impact under the heterozygous model [OR = 0.751, 95% CI = 0.599–0.942 [p-value = 0.013] (Table 2, Supplementary Figure S1B).
TABLE 2. Meta-analysis of the association of CYP24A1 (rs4809957), CYP3A4 (rs2740574), VDR (Fok1: rs2228570), VDR (Cdx-2: rs11568820), VDR (Taq1: rs731236), and VDR (BsmI: rs1544410) with lung cancer.
Regarding CYP3A4 (rs2740574), the meta-analysis addressed the risk association with lung cancer in the allelic [OR = 1.269, 95% CI 1.053–1.530, p-value = 0.012], heterozygous [OR = 1.316, 95% CI 1.043–1.661, p-value = 0.021], and dominant models [OR = 1.322, 95% CI 1.054–1.658, p-value = 0.016] (Figures 3A 3B,C).
FIGURE 3. Association between CYP3A4 (rs2740574) and lung cancer risk under (A) allelic, (B) heterozygous, and (C) dominant models. Association between VDR (Fok1: rs2228570) and decreased lung cancer risk under (D) heterozygous and (E) dominant models. Association between VDR (Cdx-2: rs11568820) and decreased lung cancer risk under (F) heterozygous and (G) dominant models. Association between VDR (Taq1: rs731236) and decreased lung cancer risk under (H) allelic, (I) homozygous, and (J) recessive models. Association between VDR (BsmI: rs1544410) and decreased lung cancer risk under (K) allelic and (L) recessive models.
VDR (Fok1: rs2228570) indicated a protective impact within the heterozygous model [OR = 0.858, 95% CI = 0.744–0.988, p-value = 0.034] (Figure 3D), and similar protective findings were found in the dominant model [OR = 0.862, 95% CI = 0.755–0.986, p-value = 0.030] (Figure 3E). VDR (Cdx-2: rs11568820) was found to be associated with protection from lung cancer under the heterozygous model [OR = 0.818, 95% CI = 0.683–978, p-value = 0.028] (Figure 3F), along with a positive impact of the variant under the dominant model [OR = 0.807, 95% CI = 0.676–0.962, p-value = 0.017] (Figure 3G).
VDR (Taq1: rs731236) exercised a protective role through our pooled analysis among allelic [OR = 0.89, 95% CI 0.804–0.986, p-value = 0.025], homozygous [OR = 0.776, 95% CI 0.618–0.976, p-value = 0.030], and recessive models [OR = 0.795, 95% CI 0.643–0.984, p-value = 0.035] (Figures 3H–J).
VDR (BsmI: rs1544410) reduced lung cancer risk in the allelic model [OR = 0.724, 95% CI, p-value], and a similar impact was noticed in the recessive model [OR = 0.684, 95% CI, p-value = 0.043] (Figure 3K and 3L) (Table 2).
Conversely, this meta-analysis revealed the lack of association of CYP2R1 (rs10741657), CYP27B1 (rs3782130), CYP27B1 (rs10877012), CYP24A1 (rs6068816), CYP24A1 (rs4809960), CYP3A5 (rs776746), GC (rs7041), GC (rs4588), and VDR (ApaI: rs7975232) with lung cancer [p-value >0.05] (Supplementary Table S3).
The random-effects model was employed for the analysis to nullify the heterogeneity among studies under the recessive model for CYP24A1 (rs4809957) and CYP27B1 (rs10877012) (Table 2 and Supplementary Table S3. CYP24A1 (rs6068816), CYP24A1 (rs4809960), and GC (rs7041) were subjected to the random-effects model for all genetic models (Supplementary Table S3), as was VDR (BsmI: rs1544410). CYP3A5 (rs776746) in both homozygous and recessive models was subjected to the random-effects model (Supplementary Table S3). VDR (ApaI: rs7975232) heterogeneity was observed in allelic, homozygous, and recessive models (Supplementary Table S3). Lastly, VDR (Cdx-2: rs11568820) and VDR (Taq1: rs731236) showed significant heterogeneity within the recessive and heterozygous models, respectively (Table 2).
Sensitivity analysis was subsequently executed to evaluate whether our results were substantially affected by one individual study. Each time, we removed one included study to recalculate the significance of the results, and the obtained results showed that the ORs were not significantly changed. The graphs of Begg’s funnel were symmetrically executed, guaranteeing the absence of publication bias among the pooled studies in our meta-analysis (Supplementary Figure S2). Additionally, Egger’s regression test showed a p-value of >0.05 within included studies under all genetic models, addressing the lack of publication bias across our present study.
As depicted in Table 3, we subjected data of one-study variants to five heredity models to assess their association with lung cancer. Of the CYP24A1-included variants, CYP24A1 (rs2585439) indicated significance with increased risk for lung cancer under allelic, homozygous, and recessive models [p-value <0.05]. Interestingly, three variants of CYP24A1 showed an increased susceptibility to lung cancer within all genetic models, including rs2762937, rs2762940 and, rs2209314 [p-value <0.05]. Conversely, CYP24A1 (rs6022993), CYP24A1 (rs8120563), and CYP24A1 (rs6068816) revealed a protective role for lung cancer in all genetic models [p-value <0.05]. CYP24A1 (rs8120563) was correlated with increased lung cancer risk in allelic, heterozygous, and dominant models [p-value <0.05], while CYP24A1 (rs2181874) was associated with lung cancer under the allelic model. Lastly, CYP24A1 (rs6022999) indicated significance with lung cancer susceptibility under allelic and homozygous models (Table 3).
TABLE 3. Genetic association models of one-study variants with the risk of lung cancer within the included studies.
Regarding the CY3A4 gene, CYP3A4 (rs4646440) indicated substantial significance with the risk of lung cancer within all genetic models [p-value <0.05]. CYP3A4 (rs4646437) revealed a decreased risk under allelic, heterozygous, dominant, and recessive models [p-value <0.05] (Table 3).
VDR (rs4237855) and VDR (rs2107301) showed a significant risk for lung cancer in homozygous and heterozygous models, respectively. VDR (rs2239184), VDR (rs7967152), and VDR (rs4760733) were correlated with lung cancer susceptibility among heterozygous and dominant models [p-value <0.05] (Table 3). Both VDR (rs10875693) and VDR (rs7974708) showed a risk for lung cancer within allelic, heterozygous, and dominant genetic models [p-value <0.05]. VDR (rs6580642) had a protective impact within the heterozygous model (Table 3).
Lung cancer is a critical problem for human health and is associated with a high rate of mortality. Therefore, its rapid and personalized management is an urgent priority. The well-established roles of vitamin D and genetic variants in the pathogenesis of several types of cancer, including lung cancer, are multifactorial and involve the alteration of transcription, translation, expression, and protein function (Shaw, 2013; Wang et al., 2017; Elsalahaty et al., 2023). Vitamin D has been attributed a favorable role in pulmonary inflammation, and its alteration was thought to be linked to lung cancer progression by modulating the tumor microenvironment and immune function (Prietl et al., 2013). Furthermore, vitamin D metabolites have been found to possess anticancer potency in preclinical models of lung cancer (Shaurova et al., 2018).
While numerous clinical trials have investigated the association of vitamin D gene variants with lung cancer, their findings were not conclusive and were not comprehensively analyzed through different genetic models. Additionally, the most recent studies have not been included. To the best of our knowledge, this is the first systematic review to extract all available data of vitamin D gene variants and subject them to genetic analyses. Our meta-analysis evidence is based on a sum of 6,206 lung cancer cases together with 7,272 healthy controls included in 16 case–control studies among seven genes of the vitamin D pathway (CYP2R1, CYP27B1, CYP24A1, CYP3A4, CYP3A5, GC, and VDR) to assess their association with lung cancer.
Our analysis showed a significant association of CYP24A1 (rs4809957) with an increased risk of lung cancer in the homozygous model and a protective impact within the heterozygous comparison. However, this analysis is limited because of its dependence on data from two available studies. Altered levels of toxins manipulated through the CYP450 machinery, including CYP3A4 and CYP3A5, are thought to participate in the pathogenesis of lung cancer. Our analysis revealed that CYP3A4 (rs2740574) has a significant association with increased lung cancer risk, while CYP3A5 (rs776746) was not correlated with lung cancer risk.
Vitamin D-binding protein encoded by the GC gene is the main transport protein for 25(OH)D and vitamin D metabolites (Speeckaert et al., 2006; Anic et al., 2014). The potential implementation of GC in diseases is thought to be linked to the altered expression of 25(OH)D, actin scavenging, and immunomodulatory roles in macrophage activation and neutrophil chemotaxis (Chun, 2012). Our analysis could not reject the null hypothesis regarding GC (rs7041) and GC (rs4588), and no correlation was observed with the risk of lung cancer in all genetic models. These findings were consistent with those of a previous meta-analysis (Duan et al., 2020).
VDR is tightly linked to vitamin D levels attributed to the modulation of the risk of lung cancer due to the antiproliferative impact of vitamin D. VDR (Apa1: rs7975232) did not reveal a significant impact on lung cancer susceptibility. The same findings were confirmed in a recent meta-analysis (Li et al., 2019; Duan et al., 2020). However, VDR (Fok1: rs2228570) and VDR (Cdx-2: rs11568820) showed significant protection in heterozygous and dominant models. Similarly, Li et al. (2019) obtained a protective impact of Cdx-2: rs11568820 in heterozygous and dominant models relying on two reports. In addition, VDR (Taq1: rs731236) indicated a significant association with a decreased risk of lung cancer in allelic, homozygous, and recessive models. Lastly, VDR (BsmI: rs1544410) indicated protection against lung cancer in the allelic and recessive models.
In contrast, CYP2R1 (rs10741657), CYP27B (rs3782130), CYP27B1 (rs10877012), and CYP24A1 (rs6068816) were not found to be associated with lung cancer. In agreement with Zhu et al. (2019), they did not show a clear association between CYP27B1 (rs10877012) and cancer risk.
Our comprehensive systematic review and data extraction for all included variants, gathered data for one-study variants that were subjected to genetic models. As a result, CYP24A1 (rs2762937), CYP24A1 (rs2762940), and CYP24A1 (rs2209314) showed a correlation with lung cancer susceptibility. In contrast, CYP24A1 (rs6022993), CYP24A1 (rs8120563), and CYP24A1 (rs6068816) revealed a protective role for lung cancer in all genetic models. Our evidence-based study suggests that CYP3A4 (rs2740574) contributes to the development of lung cancer by modulating vitamin D metabolism. It could therefore represent an independent risk factor for developing lung cancer. Genetic testing for this variant in lung cancer patients may be a useful tool for addressing which patients are characterized by higher aggressive characteristics and poorer outcomes, allowing clinicians to choose more personalized and robust treatments early in the course of the disease when treatment is most likely to be effective.
This systematic review and meta-analysis addressed the correlation of the CYP3A4 (rs2740574) variant with lung cancer risk. Conversely, VDR (Fok1: rs2228570) and VDR (Cdx-2: rs11568820, Taq1: rs731236, and BsmI: rs1544410) showed protective effects. However, CYP2R1 (rs10741657), CYP27B1 (rs3782130), CYP27B1 (rs10877012), CYP24A1 (rs6068816), CYP24A1 (rs4809960), CYP3A5 (rs776746), GC (rs7041), GC (rs4588), and VDR (ApaI: rs7975232) did not show any significant association with lung cancer. Further assessment of vitamin D pathway genes with lung cancer is required, especially for CYP3A4, CYP27B1, CYP24A1, GC, and VDR, among different ethnicities, to generalize these associations.
The original contributions presented in the study are included in the article/Supplementary Material; further inquiries can be directed to the corresponding author.
ME: conceptualization, data curation, formal analysis, investigation, methodology, project administration, resources, software, supervision, validation, writing–original draft, and writing–review and editing. SA: data curation, formal analysis, investigation, methodology, project administration, validation, visualization, writing–original draft, and writing–review and editing. AB: data curation, formal analysis, investigation, methodology, software, validation, writing–original draft, and writing–review and editing. KE-T: conceptualization, data curation, investigation, methodology, software, writing–review and editing, funding acquisition, resources, and visualization. ME-S: data curation, formal analysis, investigation, methodology, software, and writing–review and editing. EY: conceptualization, data curation, formal analysis, investigation, methodology, project administration, software, writing–original draft, and writing–review and editing.
The author(s) declare financial support was received for the research, authorship, and/or publication of this article. This review was funded by the Abu Dhabi Award for Research Excellence, Department of Education and Knowledge (Grant #: 21S105) to KE-T, and by UAEU Program for Advanced Research (UPAR; Grant #: 12S169) to KE-T.
The authors wholeheartedly thank Associate Prof. Rami M. Elshazli for his clarification of some ambiguity they encountered in their work.
The authors declare that the research was conducted in the absence of any commercial or financial relationships that could be construed as a potential conflict of interest.
All claims expressed in this article are solely those of the authors and do not necessarily represent those of their affiliated organizations, or those of the publisher, the editors, and the reviewers. Any product that may be evaluated in this article, or claim that may be made by its manufacturer, is not guaranteed or endorsed by the publisher.
The Supplementary Material for this article can be found online at: https://www.frontiersin.org/articles/10.3389/fgene.2024.1302527/full#supplementary-material
Anic, G. M., Weinstein, S. J., Mondul, A. M., Männistö, S., and Albanes, D. (2014). Serum vitamin D, vitamin D binding protein, and lung cancer survival. Lung Cancer 86 (3), 297–303. doi:10.1016/j.lungcan.2014.10.008
Bade, B. C., and Dela Cruz, C. S. (2020). Lung cancer 2020: epidemiology, etiology, and prevention. Clin. Chest Med. 41 (1), 1–24. doi:10.1016/j.ccm.2019.10.001
Ben, X., Tian, D., Liang, J., Wu, M., Xie, F., Zheng, J., et al. (2021). APOBEC3B deletion polymorphism and lung cancer risk in the southern Chinese population. Ann. Transl. Med. 9 (8), 656. doi:10.21037/atm-21-989
Brüggemann, P., and Rajguru, K. (2022). Comprehensive Meta-Analysis (CMA) 3.0: a software review. J. Mark. Anal. 10 (4), 425–429. doi:10.1057/s41270-022-00184-5
Christakos, S., Dhawan, P., Verstuyf, A., Verlinden, L., and Carmeliet, G. (2015). Vitamin D: metabolism, molecular mechanism of action, and pleiotropic effects. Physiol. Rev. 96 (1), 365–408. doi:10.1152/physrev.00014.2015
Chun, R. F. (2012). New perspectives on the vitamin D binding protein. Cell Biochem. Funct. 30 (6), 445–456. doi:10.1002/cbf.2835
Çiçek, H., Güleken, N., Öztuzcu, S., and Sevinç, A. (2017). Vitamin D receptor polymorphisms and related biochemical parameters in various cancer species. Param. Var. cancer species 42 (2), 161–168. doi:10.1515/tjb-2016-0215
Dally, H., Bartsch, H., Jäger, B., Edler, L., Schmezer, P., Spiegelhalder, B., et al. (2004). Genotype relationships in the CYP3A locus in Caucasians. Cancer Lett. 207 (1), 95–99. doi:10.1016/j.canlet.2003.12.011
Dogan, I., Onen, H. I., Yurdakul, A. S., Konac, E., Ozturk, C., Varol, A., et al. (2009). Polymorphisms in the vitamin D receptor gene and risk of lung cancer. Med. Sci. Monit. 15 (8), Br232–42.
Duan, G.-q., Zheng, X., Li, W.-k., Zhang, W., Li, Z., and Tan, W. (2020). The association between VDR and GC polymorphisms and lung cancer risk: a systematic review and meta-analysis. Genet. Test. Mol. Biomarkers 24 (5), 285–295. doi:10.1089/gtmb.2019.0187
El Awady, A. A., Elshazli, R. M., El Awady, A. A., Elgaml, A., Khalifa, A. K., and Settin, A. (2022). Association of CTLA4 c.49A > G (rs231775; p.Thr17Ala) gene variant with the risk of hepatocellular carcinoma and gastric cancer: a meta-analysis and meta-regression. Meta Gene 31, 100999. doi:10.1016/j.mgene.2021.100999
Elsalahaty, M. I., Salama, A. F., Diab, T., Ghazy, M., Toraih, E., and Elshazli, R. M. (2023). Unleash multifunctional role of miRNA biogenesis gene variants (XPO5*rs34324334 and RAN*rs14035) with susceptibility to hepatocellular carcinoma. J. Personalized Med. 13, 959. doi:10.3390/jpm13060959
Elshazli, R. M., Salman, D. O., Kamel, M. M., Toraih, E. A., and Fawzy, M. S. (2018). Genetic polymorphisms of IL-17A rs2275913, rs3748067 and IL-17F rs763780 in gastric cancer risk: evidence from 8124 cases and 9873 controls. Mol. Biol. Rep. 45 (5), 1421–1444. doi:10.1007/s11033-018-4202-z
Garland, C. F., and Gorham, E. D. (2017). Dose-response of serum 25-hydroxyvitamin D in association with risk of colorectal cancer: a meta-analysis. J. steroid Biochem. Mol. Biol. 168, 1–8. doi:10.1016/j.jsbmb.2016.12.003
Gilbert, R., Martin, R. M., Beynon, R., Harris, R., Savovic, J., Zuccolo, L., et al. (2011). Associations of circulating and dietary vitamin D with prostate cancer risk: a systematic review and dose-response meta-analysis. Cancer causes control CCC 22 (3), 319–340. doi:10.1007/s10552-010-9706-3
Gromowski, T., Gapska, P., Scott, R. J., Kąklewski, K., Marciniak, W., Durda, K., et al. (2017). Serum 25(OH)D concentration, common variants of the VDR gene and lung cancer occurrence. Int. J. Cancer 141 (2), 336–341. doi:10.1002/ijc.30740
Hansdottir, S., Monick, M. M., Hinde, S. L., Lovan, N., Look, D. C., and Hunninghake, G. W. (2008). Respiratory epithelial cells convert inactive vitamin D to its active form: potential effects on host defense. J. Immunol. 181 (10), 7090–7099. doi:10.4049/jimmunol.181.10.7090
Haznadar, M., Krausz, K. W., Margono, E., Diehl, C. M., Bowman, E. D., Manna, S. K., et al. (2018). Inverse association of vitamin D(3) levels with lung cancer mediated by genetic variation. Cancer Med. 7 (6), 2764–2775. doi:10.1002/cam4.1444
Higgins, J. P. T., Thompson, S. G., Deeks, J. J., and Altman, D. G. (2003). Measuring inconsistency in meta-analyses. Br. Med. J. 327 (7414), 557–560. doi:10.1136/bmj.327.7414.557
Ingelman-Sundberg, M., Sim, S. C., Gomez, A., and Rodriguez-Antona, C. (2007). Influence of cytochrome P450 polymorphisms on drug therapies: pharmacogenetic, pharmacoepigenetic and clinical aspects. Pharmacol. Ther. 116 (3), 496–526. doi:10.1016/j.pharmthera.2007.09.004
Islam, M. S., Mostofa, A. G., Ahmed, M. U., Bin Sayeed, M. S., Hassan, M. R., and Hasnat, A.Association of CYP3A4 (2014). Association of CYP3A4, CYP3A5 polymorphisms with lung cancer risk in Bangladeshi population. Tumour Biol. 35 (2), 1671–1678. doi:10.1007/s13277-013-1230-0
Janoušek, J., Pilařová, V., Macáková, K., Nomura, A., Veiga-Matos, J., Silva, D. D. d., et al. (2022). Vitamin D: sources, physiological role, biokinetics, deficiency, therapeutic use, toxicity, and overview of analytical methods for detection of vitamin D and its metabolites. Crit. Rev. Clin. laboratory Sci. 59 (8), 517–554. doi:10.1080/10408363.2022.2070595
Jia, Z., Zhou, W., Zhang, G., Fu, J., Li, D., and Ren, L. (2020). CYP3A4 genetic variants are associated with susceptibility of non-small cell lung cancer in a Shaanxi Han population. Genomics 112 (5), 3465–3472. doi:10.1016/j.ygeno.2020.05.023
Jones, G., Prosser, D. E., and Kaufmann, M. (2012). 25-Hydroxyvitamin D-24-hydroxylase (CYP24A1): its important role in the degradation of vitamin D. Archives Biochem. Biophysics 523 (1), 9–18. doi:10.1016/j.abb.2011.11.003
Jones, G., Prosser, D. E., and Kaufmann, M. (2014). Cytochrome P450-mediated metabolism of vitamin D. J. Lipid Res. 55 (1), 13–31. doi:10.1194/jlr.R031534
Kaabachi, W., Kaabachi, S., Rafrafi, A., Amor, A. B., Tizaoui, K., Haj Sassi, F., et al. (2014). Association of vitamin D receptor FokI and ApaI polymorphisms with lung cancer risk in Tunisian population. Mol. Biol. Rep. 41 (10), 6545–6553. doi:10.1007/s11033-014-3538-2
Kasarla, S. S., Garikapati, V., Kumar, Y., and Dodoala, S. (2022a). Interplay of vitamin D and CYP3A4 polymorphisms in endocrine disorders and cancer. Endocrinol. Metab. Seoul. 37 (3), 392–407. doi:10.3803/EnM.2021.1349
Kasarla, S. S., Garikapati, V., Kumar, Y., and Dodoala, S. (2022b). Interplay of vitamin D and CYP3A4 polymorphisms in endocrine disorders and cancer. Endocrinol. Metabolism 37 (3), 392–407. doi:10.3803/EnM.2021.1349
Kim, Y., and Je, Y. (2014). Vitamin D intake, blood 25 (OH) D levels, and breast cancer risk or mortality: a meta-analysis. Br. J. cancer 110 (11), 2772–2784. doi:10.1038/bjc.2014.175
Klyushova, L. S., Perepechaeva, M. L., and Grishanova, A. Y. (2022). The role of CYP3A in health and disease. Biomedicines 10 (11), 2686. doi:10.3390/biomedicines10112686
Kong, J., Chen, X., Wang, J., Li, J., Xu, F., Gao, S., et al. (2020). Genetic polymorphisms in the vitamin D pathway and non-small cell lung cancer survival. Pathology Oncol. Res. POR 26 (3), 1709–1715. doi:10.1007/s12253-019-00702-4
Kong, J., Xu, F., Qu, J., Wang, Y., Gao, M., Yu, H., et al. (2015). Genetic polymorphisms in the vitamin D pathway in relation to lung cancer risk and survival. Oncotarget 6 (4), 2573–2582. doi:10.18632/oncotarget.2951
Li, M., Liu, X., Liu, N., Yang, T., Shi, P., He, R., et al. (2019). Association between polymorphisms of vitamin D receptor and lung cancer susceptibility: evidence from an updated meta-analysis. J. Cancer 10 (16), 3639–3649. doi:10.7150/jca.33431
Maneechay, W., Boonpipattanapong, T., Kanngurn, S., Puttawibul, P., Geater, S. L., and Sangkhathat, S. (2015). Single nucleotide polymorphisms in the Gc gene for vitamin D binding protein in common cancers in Thailand. Asian Pac J. Cancer Prev. 16 (8), 3339–3344. doi:10.7314/apjcp.2015.16.8.3339
Nakagawa, K., Kawaura, A., Kato, S., Takeda, E., and Okano, T. (2005). 1 alpha,25-Dihydroxyvitamin D(3) is a preventive factor in the metastasis of lung cancer. Carcinogenesis 26 (2), 429–440. doi:10.1093/carcin/bgh332
Page, M. J., McKenzie, J. E., Bossuyt, P. M., Boutron, I., Hoffmann, T. C., Mulrow, C. D., et al. (2021). The PRISMA 2020 statement: an updated guideline for reporting systematic reviews. BMJ 372, n71. doi:10.1136/bmj.n71
Pineda Lancheros, L. E., Pérez Ramírez, C., Sánchez Martín, A., Gálvez Navas, J. M., Martínez Martínez, F., Ramírez Tortosa, M. D. C., et al. (2021). Impact of genetic polymorphisms on the metabolic pathway of vitamin D and survival in non-small cell lung cancer. Nutrients 13 (11), 3783. doi:10.3390/nu13113783
Pineda Lancheros, L. E., Rojo Tolosa, S., Gálvez Navas, J. M., Martínez Martínez, F., Sánchez Martín, A., Jiménez Morales, A., et al. (2022). Effect of single nucleotide polymorphisms in the vitamin D metabolic pathway on susceptibility to non-small-cell lung cancer. Nutrients 14 (21), 4668. doi:10.3390/nu14214668
Piotrowska, A., Wierzbicka, J., Rybarczyk, A., Tuckey, R. C., Slominski, A. T., and Żmijewski, M. A. (2019). Vitamin D and its low calcemic analogs modulate the anticancer properties of cisplatin and dacarbazine in the human melanoma A375 cell line. Int. J. Oncol. 54 (4), 1481–1495. doi:10.3892/ijo.2019.4725
Prietl, B., Treiber, G., Pieber, T. R., and Amrein, K. (2013). Vitamin D and immune function. Nutrients 5 (7), 2502–2521. doi:10.3390/nu5072502
Qu, R., Li, X., Quan, X., Xia, L., Fang, X., Li, H., et al. (2019). Polymorphism in CYP24A1 is associated with lung cancer risk: a case-control study in Chinese female nonsmokers. DNA Cell Biol. 38 (3), 243–249. doi:10.1089/dna.2018.4510
Salama, R. H. M., Faied, S. M. A., Elkholy, M., Abd-Elmawgoud, N. S., Alsanory, T. A., Alsanory, A. A., et al. (2022). Gene expression of programmed cell death ligand-1 (PDL-1) and vitamin D receptor (VDR) with the serum vitamin D3 in lung cancer. Egypt. J. Bronchology 16 (1), 65. doi:10.1186/s43168-022-00168-0
Shaurova, T., Seshadri, M., and Hershberger, P. A. (2018). “Chapter 101 - vitamin D and lung cancer,” in Vitamin D. Editor D. Feldman (Academic Press2018), 875–890.
Shaw, G. (2013). Polymorphism and single nucleotide polymorphisms (SNPs). BJU Int. 112 (5), 664–665. doi:10.1111/bju.12298
Speeckaert, M., Huang, G., Delanghe, J. R., and Taes, Y. E. (2006). Biological and clinical aspects of the vitamin D binding protein (Gc-globulin) and its polymorphism. Clin. Chim. Acta 372 (1-2), 33–42. doi:10.1016/j.cca.2006.03.011
Stroup, D. F., Berlin, J. A., Morton, S. C., Olkin, I., Williamson, G. D., Rennie, D., et al. (2000). Meta-analysis of observational studies in epidemiology: a proposal for reporting. Meta-analysis of Observational Studies in Epidemiology (MOOSE) group. Jama 283 (15), 2008–2012. doi:10.1001/jama.283.15.2008
Timofeeva, M. N., Kropp, S., Sauter, W., Beckmann, L., Rosenberger, A., Illig, T., et al. (2009). CYP450 polymorphisms as risk factors for early-onset lung cancer: gender-specific differences. Carcinogenesis 30 (7), 1161–1169. doi:10.1093/carcin/bgp102
Wang, J., Liu, Q., Yuan, S., Xie, W., Liu, Y., Xiang, Y., et al. (2017). Genetic predisposition to lung cancer: comprehensive literature integration, meta-analysis, and multiple evidence assessment of candidate-gene association studies. Sci. Rep. 7 (1), 8371. doi:10.1038/s41598-017-07737-0
Wang, Z., Lin, Y. S., Zheng, X. E., Senn, T., Hashizume, T., Scian, M., et al. (2012). An inducible cytochrome P450 3A4-dependent vitamin D catabolic pathway. Mol. Pharmacol. 81 (4), 498–509. doi:10.1124/mol.111.076356
Wang, Z., Schuetz, E. G., Xu, Y., and Thummel, K. E. (2013). Interplay between vitamin D and the drug metabolizing enzyme CYP3A4. J. Steroid Biochem. Mol. Biol. 136, 54–58. doi:10.1016/j.jsbmb.2012.09.012
Wei, H., Jing, H., Wei, Q., Wei, G., and Heng, Z. (2018). Associations of the risk of lung cancer with serum 25-hydroxyvitamin D level and dietary vitamin D intake: a dose-response PRISMA meta-analysis. Medicine 97 (37), e12282. doi:10.1097/MD.0000000000012282
Wei, Z., Zhang, S., and Hu, J. (2021). CTLA-4 +49 A/G polymorphism and the risk of lung cancer: a meta-analysis. Zhongguo fei ai za zhi = Chin. J. lung cancer 24 (3), 173–181. doi:10.3779/j.issn.1009-3419.2021.102.09
Wu, X., Cheng, J., and Yang, K. (2016). Vitamin D-related gene polymorphisms, plasma 25-hydroxy-vitamin D, cigarette smoke and non-small cell lung cancer (NSCLC) risk. Int. J. Mol. Sci. 17 (10), 1597. doi:10.3390/ijms17101597
Xiong, Q., Jiao, Y., Yang, P., Liao, Y., Gu, X., Hu, F., et al. (2020). The association study between CYP24A1 gene polymorphisms and risk of liver, lung and gastric cancer in a Chinese population. Pathol. Res. Pract. 216 (12), 153237. doi:10.1016/j.prp.2020.153237
Xu, Q., Liu, J.-w., and Yuan, Y. (2015). Comprehensive assessment of the association between miRNA polymorphisms and gastric cancer risk. Mutat. Research/Reviews Mutat. Res. 763, 148–160. doi:10.1016/j.mrrev.2014.09.004
Xu, Y., Hashizume, T., Shuhart, M. C., Davis, C. L., Nelson, W. L., Sakaki, T., et al. (2006). Intestinal and hepatic CYP3A4 catalyze hydroxylation of 1alpha,25-dihydroxyvitamin D(3): implications for drug-induced osteomalacia. Mol. Pharmacol. 69 (1), 56–65. doi:10.1124/mol.105.017392
Zhang, L., Wang, S., Che, X., and Li, X. (2015a). Vitamin D and lung cancer risk: a comprehensive review and meta-analysis. Int. J. Exp. Cell. physiology, Biochem. Pharmacol. 36 (1), 299–305. doi:10.1159/000374072
Zhang, L., Wang, S., Che, X., and Li, X. (2015b). Vitamin D and lung cancer risk: a comprehensive review and meta-analysis. Cell. Physiology Biochem. 36 (1), 299–305. doi:10.1159/000374072
Zhou, W., Zhu, W., Tong, X., Ming, S., Ding, Y., Li, Y., et al. (2020). CHRNA5 rs16969968 polymorphism is associated with lung cancer risk: a meta-analysis. Clin. Respir. J. 14 (6), 505–513. doi:10.1111/crj.13165
Zhu, K., Xu, A., Xia, W., Li, P., Zhang, B., Jiang, H., et al. (2021). Association between NAT2 polymorphism and lung cancer risk: a systematic review and meta-analysis. Front. Oncol. 11, 567762. doi:10.3389/fonc.2021.567762
Zhu, M., Tan, Z., Luo, Z., Hu, H., Wu, T., Fang, S., et al. (2019). Association of the vitamin D metabolism gene GC and CYP27B1 polymorphisms with cancer susceptibility: a meta-analysis and trial sequential analysis. Biosci. Rep. 39 (9), BSR20190368. doi:10.1042/BSR20190368
Keywords: gene variants, lung cancer, polymorphism, vitamin D, vitamin D receptor
Citation: Elsalahaty MI, Alkafaas SS, Bashir AO, El-Tarabily KA, El-Saadony MT and Yousef EH (2024) Revealing the association between vitamin D metabolic pathway gene variants and lung cancer risk: a systematic review and meta-analysis. Front. Genet. 15:1302527. doi: 10.3389/fgene.2024.1302527
Received: 26 September 2023; Accepted: 05 January 2024;
Published: 28 February 2024.
Edited by:
Nosheen Masood, Fatima Jinnah Women University, PakistanReviewed by:
Nagham Nafiz Hendi, Hamad Bin Khalifa University, QatarCopyright © 2024 Elsalahaty, Alkafaas, Bashir, El-Tarabily, El-Saadony and Yousef. This is an open-access article distributed under the terms of the Creative Commons Attribution License (CC BY). The use, distribution or reproduction in other forums is permitted, provided the original author(s) and the copyright owner(s) are credited and that the original publication in this journal is cited, in accordance with accepted academic practice. No use, distribution or reproduction is permitted which does not comply with these terms.
*Correspondence: Khaled A. El-Tarabily, a3RhcmFiaWx5QHVhZXUuYWMuYWU=
†These authors have contributed equally to this work
Disclaimer: All claims expressed in this article are solely those of the authors and do not necessarily represent those of their affiliated organizations, or those of the publisher, the editors and the reviewers. Any product that may be evaluated in this article or claim that may be made by its manufacturer is not guaranteed or endorsed by the publisher.
Research integrity at Frontiers
Learn more about the work of our research integrity team to safeguard the quality of each article we publish.