- 1Institute of Medicine, Chung Shan Medical University, Taichung, Taiwan
- 2Department of Ophthalmology, Jen-Ai Hospital, Taichung, Taiwan
- 3Department of Ophthalmology, Changhua Christian Hospital, Changhua, Taiwan
- 4Department of Post-Baccalaureate Medicine, College of Medicine, National Chung Hsing University, Taichung, Taiwan
- 5Department of Ophthalmology, Chang Gung Memorial Hospital, Taoyuan, Taiwan
- 6College of Medicine, Chang Gung University, Taoyuan, Taiwan
- 7Department of Ophthalmology, Chang Gung Memorial Hospital, Xiamen Branch, Xiamen, China
- 8Graduate Institute of Biomedical Engineering, Chang Gung University, Taoyuan, Taiwan
- 9Department of Ophthalmology, Chang Gung Memorial Hospital at Linkou, Taoyuan, Taiwan
- 10Nobel Eye Institute, Taipei, Taiwan
- 11Department of Medical Research, Chung Shan Medical University Hospital, Taichung, Taiwan
Stromal cell-derived factor-1 (SDF-1) and its receptor CXC chemokine 4 (CXCR4) have been demonstrated to play critical roles in diabetic retinopathy (DR). This study investigated whether single-nucleotide polymorphisms (SNPs) of SDF-1 and its receptor CXCR4 are correlated with diabetic retinopathy (DR). Three SDF-1 SNPs, namely, rs1801157 (G/A), rs2297630 (G/A), and rs266085 (T/C), and two CXCR4 SNPs, namely, rs2228014 (C/T) and rs6430612 (C/T), were chosen and genotyped via the TaqMan allelic discrimination for 454 non-DR subjects and 276 DR individuals. Our results revealed that subjects carrying SDF-1 SNP rs2297630 GA (AOR: 2.962, 95% CI: 1.279-6.861, p = 0.011) and SDF-1 SNP rs2297630 GA + AA (AOR: 3.095, 95% CI: 1.394-6.872, p = 0.006) had significantly higher risk in the non-proliferative diabetic retinopathy (NPDR) groups than in the non-DR group. Further analyses using the datasets from the Genotype-Tissue Expression (GTEx) Portal revealed that SDF-1 SNP rs2297630 GA and AA genotypic variants have higher SDF-1 expression than the GG wild-type alleles (p = 0.000016). In conclusion, our findings revealed that SDF-1 SNP rs2297630 was associated with NPDR.
Introduction
Diabetic retinopathy (DR) is a specific microvascular complication in diabetes mellitus (DM) (Lin et al., 2021; Teo et al., 2021). Its global prevalence was estimated to be one-fifth among individuals with diabetes (Teo et al., 2021). The abnormalities of DR include microaneurysm, retinal hemorrhage, venous beading, exudates, cotton wool spots, intraretinal microvascular abnormalities, and neovascularization of the retina. Based on the presence of retinal neovascularization, DR could be divided into non-proliferative diabetic retinopathy (NPDR) and proliferative retinopathy (PDR). To treat visual-threatening DR, treatment options include laser photocoagulation, intravitreal injection of anti-vascular endothelial growth factors (anti-VEGF) or steroids, and vitrectomy. Without adequate treatment, DR may progress rapidly and cause visual impairment (Bakri et al., 2022).
There are some factors that affect the development and progression of DR. A higher glycated hemoglobin (HbA1c) level, a longer duration of diabetes mellitus, hypertension, and dyslipidemia are associated with diabetic retinopathy (Cheung et al., 2010; Lin et al., 2021). Moreover, genetic factors play an essential role in DR, and genetic studies show a racial variation in DR’s incidence (Simó-Servat et al., 2013; Cho and Sobrin, 2014; Mankoč Ramuš et al., 2021; Chuang et al., 2022a; Chuang et al., 2022b; Lee et al., 2022). Genome-wide analysis of DNA methylation in patients with type 1 diabetes mellitus found that this is associated with PDR (Agardh et al., 2015). According to the understanding of the pathogenesis of DR and PDR, several candidate genes were thought to be related to DR and PDR (Petrovic, 2013). The screening of single-nucleotide polymorphisms (SNPs) is useful to find the genetic risk factors for DR.
Stromal cell-derived factor-1 (SDF-1) is a chemokine, and CXC chemokine 4 (CXCR4) is the receptor of SDF-1 (Chang et al., 2009; Teng et al., 2009; Sadri et al., 2022). SDF-1 is expressed by the stromal cells and was initially isolated from the murine bone marrow (Guleng et al., 2005; Dar et al., 2006; Jin et al., 2006). In the human retina, the location of SDF-1 is mainly at the inner photoreceptor matrix and retinal pigment epithelial (RPE) cells, and the location of CXCR4 is mainly in the inner segment of photoreceptors (Bhutto et al., 2006). A higher level of vitreous SDF-1 was found in patients with proliferative diabetic retinopathy (Butler et al., 2005; Keles et al., 2021). Intravitreal injection of triamcinolone in patients with diabetic retinopathy decreased the level of vitreous SDF-1 (Butler et al., 2005). In human serum, the SDF-1 level was higher in patients with at least severe non-proliferative diabetic retinopathy than in patients with less severe diabetic retinopathy (Meleth et al., 2005). In one in vitro study, treatment with high glucose on human retinal vascular endothelial cells (hRVECs) increased the protein levels of SDF-1 and CXCR4, and the CXCR4 antagonist inhibited the expression levels of angiogenesis-associated proteins in hRVECs (Wu et al., 2019). Moreover, the polymorphism of the SDF-1 and CXCR4 gene are already known to be associated with the development of DR (Djuric et al., 2010; Gharibi et al., 2022). A previous study reported that homozygous carriers of SDF-1 3′A genotype are associated with the risk of PDR (Djuric et al., 2010). However, Gharibi et al. indicated no association among SDF-1 SNP rs1801157 and CXCR4 SNP rs2228014 polymorphisms and the risk of PDR in Iran population (Gharibi et al., 2022). Despite several studies having investigated the functional role of SDF-1 and CXCR4 SNPs in DR, the relationships between SDF-1 and CXCR4 SNPs and DR in the Taiwanese population have not been explored. The aim of this study was to investigate the potential association between the genetic polymorphisms of SDF-1 and CXCR4 and PDR in the Taiwanese population with type 2 DM.
Materials and methods
Ethical declarations
The procedures of this study adhered to the declaration of Helsinki in 1964 and its amendments. Our study was approved by the Institutional Review Boards of Chung Shan Medical University Hospital (project identification code: CS1-20048). Signed written informed consents were signed by all the participants of this study.
Subject selection
This prospective case–control study was conducted in Chung Shan Medical University Hospital. A total of 730 individuals with diabetes mellitus from the department of endocrinology were enrolled in this study. According to the records of the ophthalmic department, the participants were divided into three categories by the Early Treatment Diabetic Retinopathy Study (ETDRS) diabetic retinopathy grading scale: the non-DR group, the NPDR group, and the PDR group, in which were 454, 165, and 111 participants, respectively (Kataoka et al., 2023). DR was regarded as at least one of the subsequent findings: microaneurysm, dot- or flame-shape intraretinal hemorrhage, venous beading, hard exudate, cotton-wool spot, or intraretinal microvascular abnormality. The PDR was defined as one of the following findings: neovascularization of the optic disc or the retina, neovascular glaucoma, vitreous hemorrhage, or tractional retinal detachment. The non-DR group, which was considered the control group, included individuals with diabetes mellitus without DR.
Medical data and samples collection
We collected the medical records of these patients, including their age, age of onset of DM, gender, duration of diabetes, HbA1c, insulin treatment, body mass index, blood pressure, renal function, and lipid profiles. All participants received venous blood drawing for the investigation of SDF-1 and CXCR4 polymorphisms (Tee et al., 2012). The blood samples were stored in ethylenediaminetetraacetic acid-containing tubes and were then centrifuged and preserved in the laboratory refrigerator at −80 degrees Celsius. If the genomes of the blood samples were degraded before the analyses, they would be excluded from our study.
DNA obtain and definition of SDF-1 and CXCR SNP with Real-Time PCR
Three SDF-1 SNPs [rs1801157 (G/A), rs2297630 (G/A), and rs266085 (T/C)] and two CXCR4 SNPs [rs2228014 (C/T) and rs6430612 (C/T)] were chosen for genetic analysis because of their tendency of malignancy, coronary disease, or hematological disease (Runmin et al., 2018; Li et al., 2020; Lin et al., 2022; Mohamed et al., 2022). Following the manufacturer’s instructions for DNA isolation, the genome DNA was extracted from the leukocytes in the blood samples by the QIAamp DNA kits (Qiagen, Valencia, Valencia, CA, United States) (Hsiao et al., 2010; Su et al., 2018). Then, the samples were stored in the refrigerator at around −20 degrees Celsius. The SDF-1 and CXCR4 SNPs were sequenced by the ABI StepOne Real-Time PCR System (Applied Biosystems, Foster City, California). We analyzed the findings of the SDF-1 and CXCR4 genetics polymorphism via SDS version 3.0 software (Applied Biosystems, Foster City, CA, United States).
Statistical analysis
In our study, we used SAS version 9.4 (SAS Institute Inc, NC, United States) for analysis. We used descriptive analysis containing mean value, standard deviation (SD), and percentage to reveal the clinical and laboratory characteristics of the non-DR group, NPDR group, and PDR group. The independent t-test was used to compare the differences in both the clinical and laboratory data between the non-DR group and the NPDR group and between the non-DR group and the PDR group, respectively. Then, we used multiple logistic regression models after controlling for age, the duration of diabetes, HbA1c, insulin treatment, serum creatinine levels, glomerular filtration rate, and HDL cholesterol levels to estimate the adjusted odds ratio (AOR) and corresponding 95% confidence intervals (CI) of the six SNP distributions between the non-DR group and the DR group. We further searched the data that were related to the SNPs in a public database, the Genotype-Tissue Expression (GTEx) Portal, to analyze the genotype–phenotype correlation (https://gtexportal.org/home/). A p value of less than 0.05 was defined as statistically significant.
Results
Clinical manifestation of study participants
Table 1 summarizes the clinical and laboratory characteristics among the non-DR, NPDR, and PDR groups. The mean age was 60.22 ± 11.22 years old in the non-DR group, 62.29 ± 10.76 years old in the NPDR group, and 63.09 ± 10.70 years old in the PDR group. The NPDR group and PDR group had a longer duration of DM, higher HbA1c level, higher percentage of insulin treatment, higher serum creatinine, and lower glomerular filtration rate compared to the non-DR group (all p < 0.05). The NPDR group revealed a lower HDL cholesterol level compared to the non-DR group (p = 0.014).
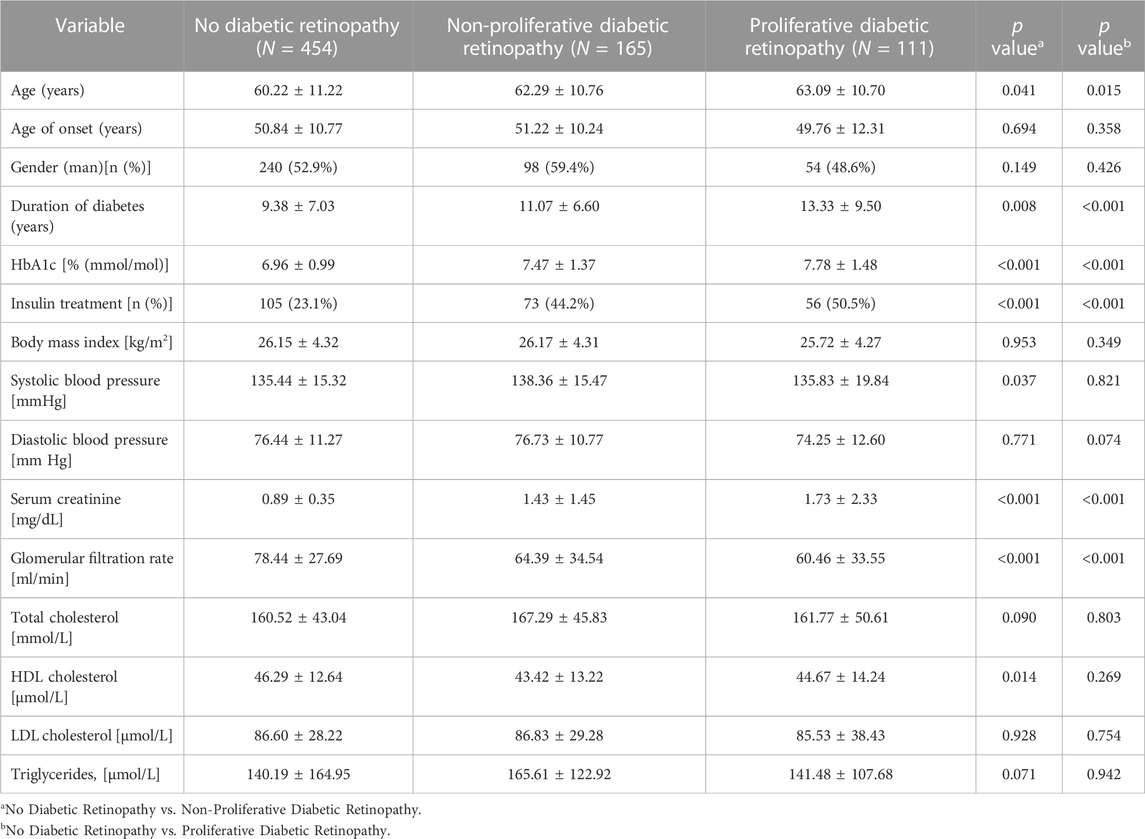
TABLE 1. Clinical and laboratory characteristics of patients with diabetic retinopathy and no diabetic retinopathy.
SDF-1 and CXCR4 SNPs distribution among different DR populations
In the comparison of the SDF-1 and CXCR4 SNPs between the non-DR and the DR group, the distribution frequency of the SDF-1 and CXCR4 SNPs was similar to that of wild type (all p > 0.05) (Table 2). Moreover, the distribution frequencies of the SDF-1 and CXCR4 SNPs between the non-DR, NPDR, and PDR groups were similar to that of the wild type (all p > 0.05) (Table 3; Table 4). Between the non-DR and the NPDR groups in the younger group (≤60 years), the results revealed that subjects carrying the SDF-1 SNP rs2297630 GA (AOR: 2.962, 95% CI: 1.279-6.861, p = 0.011) and SDF-1 SNP rs2297630 GA + AA (AOR: 3.095, 95% CI: 1.394-6.872, p = 0.006) had a significantly higher risk in the NPDR group than in the non-DR group (Table 5).
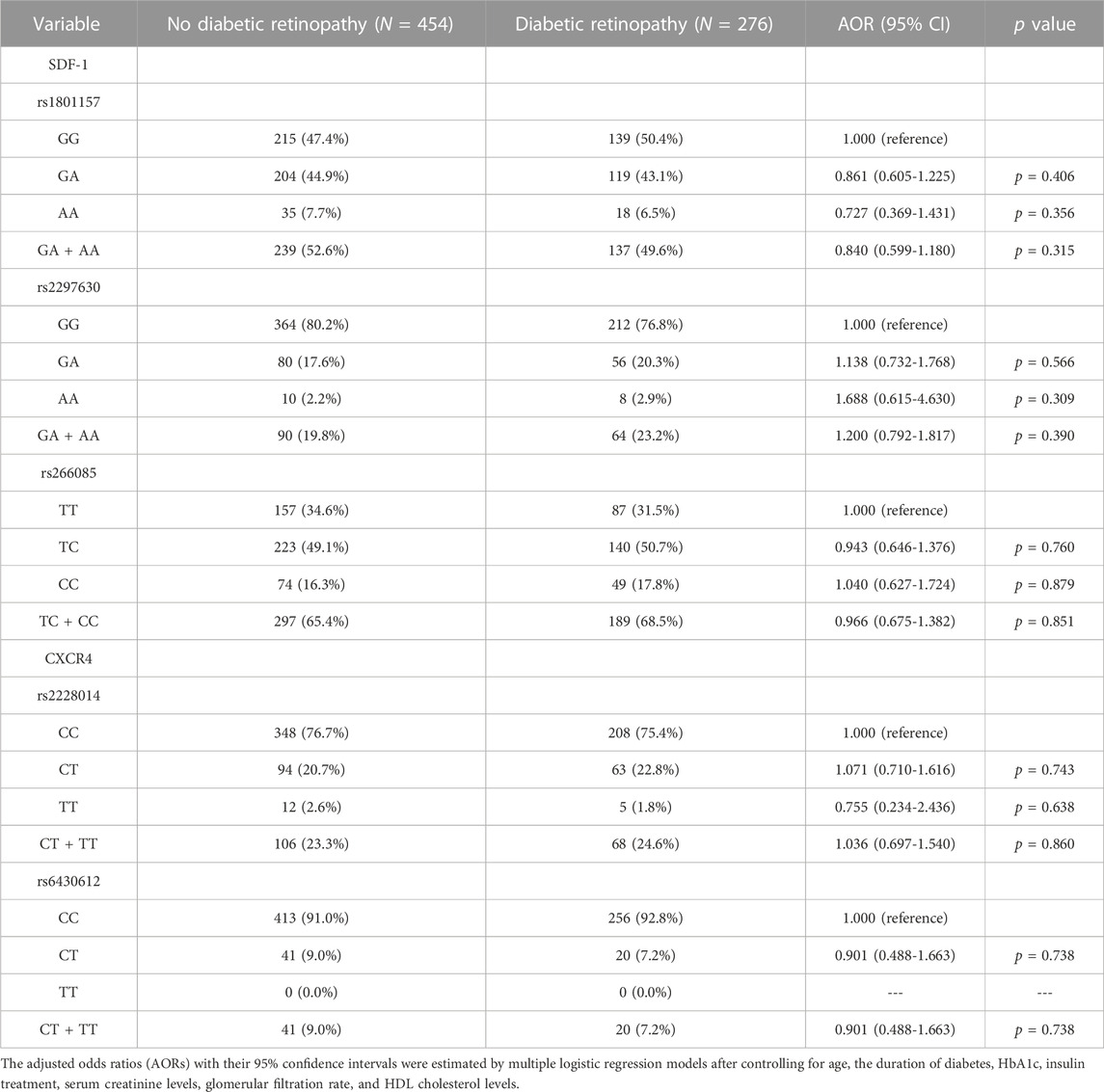
TABLE 2. Odds ratios (ORs) and 95% confidence intervals (CIs) of diabetic retinopathy associated with SDF-1α/CXCR4 axis genotypic frequencies.
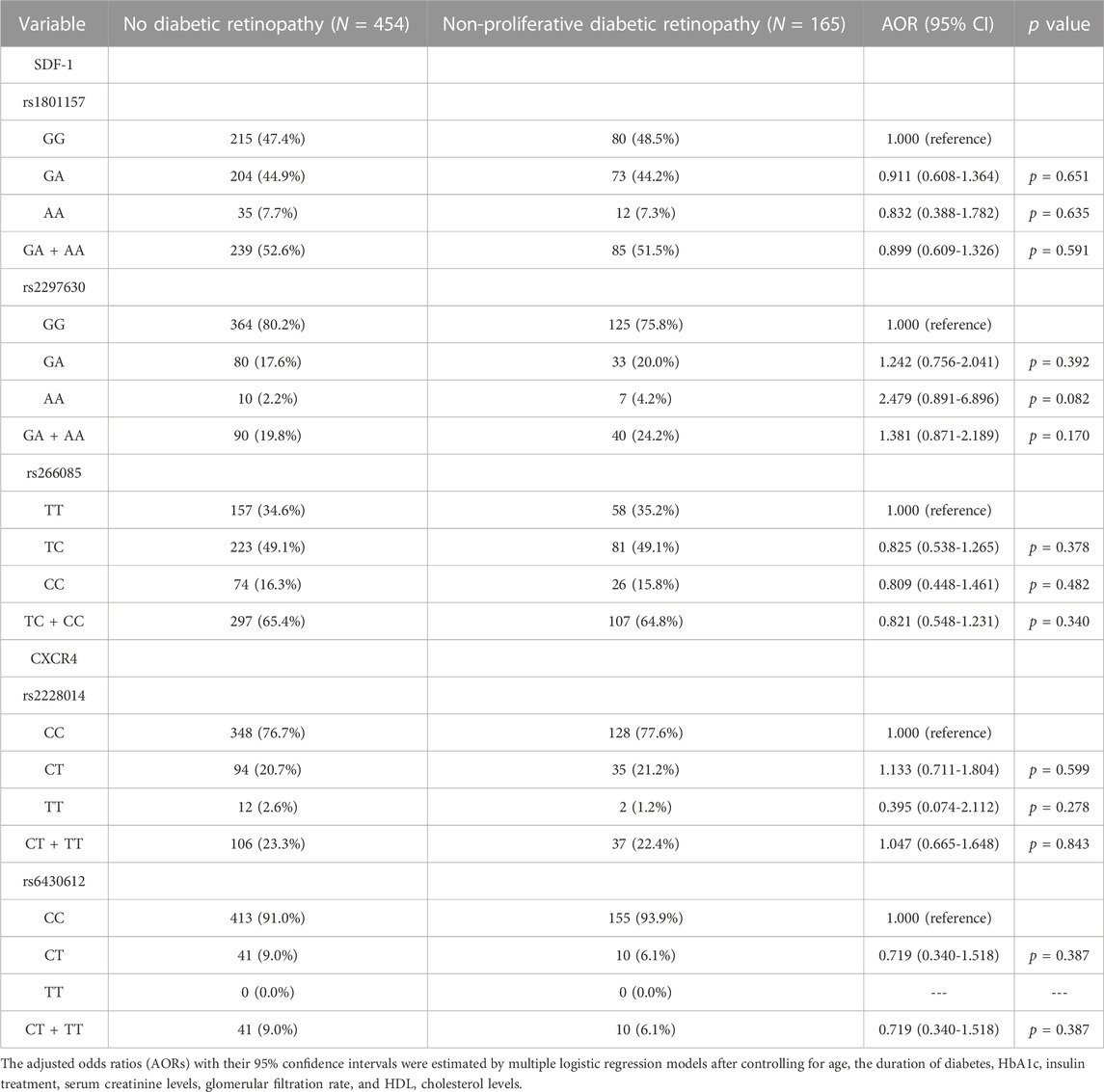
TABLE 3. Odds ratios (ORs) and 95% confidence intervals (CIs) of non-proliferative diabetic retinopathy associated with SDF-1α/CXCR4 axis genotypic frequencies.
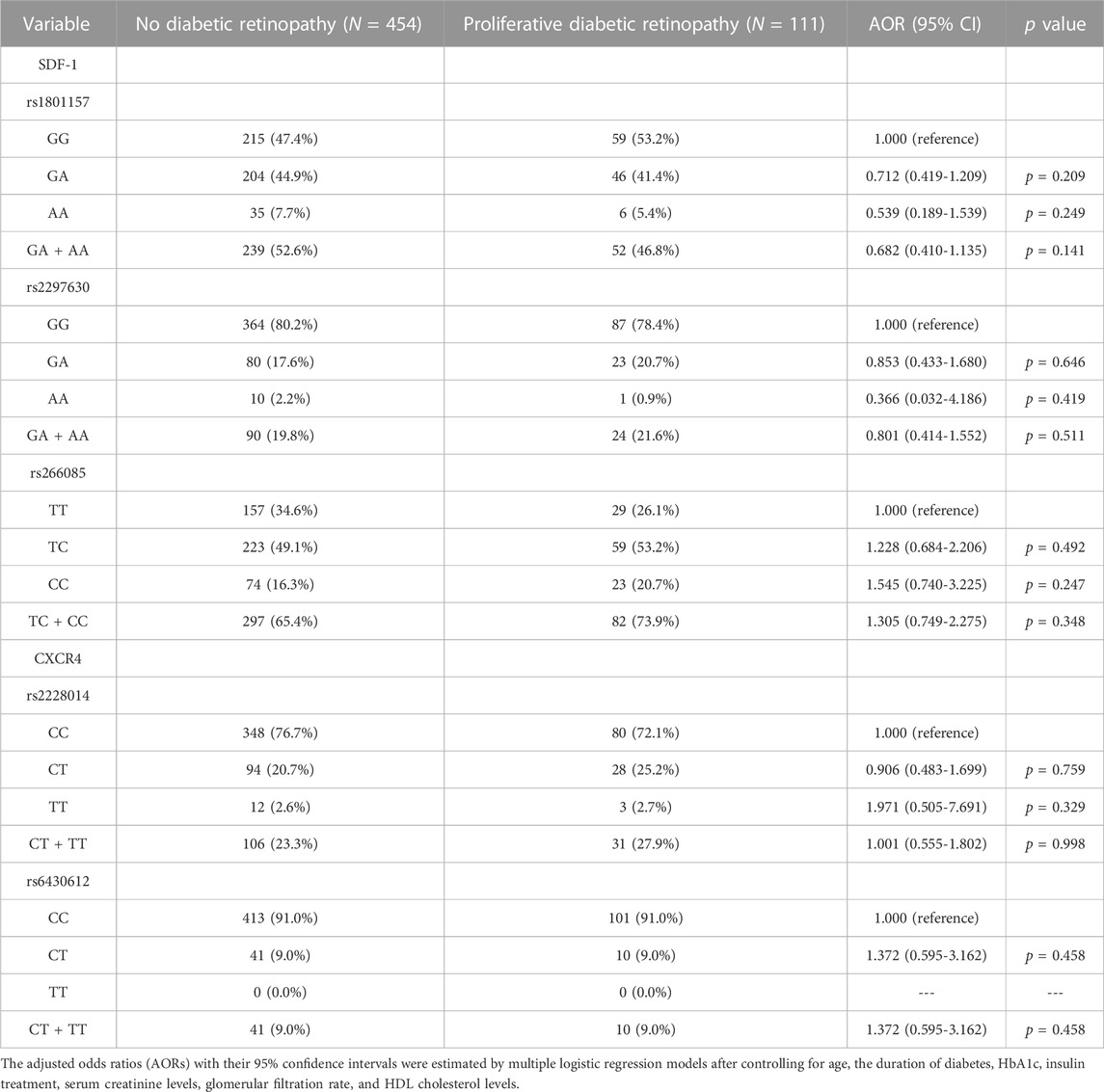
TABLE 4. Odds ratios (ORs) and 95% confidence intervals (CIs) of proliferative diabetic retinopathy associated with SDF-1α/CXCR4 axis genotypic frequencies.
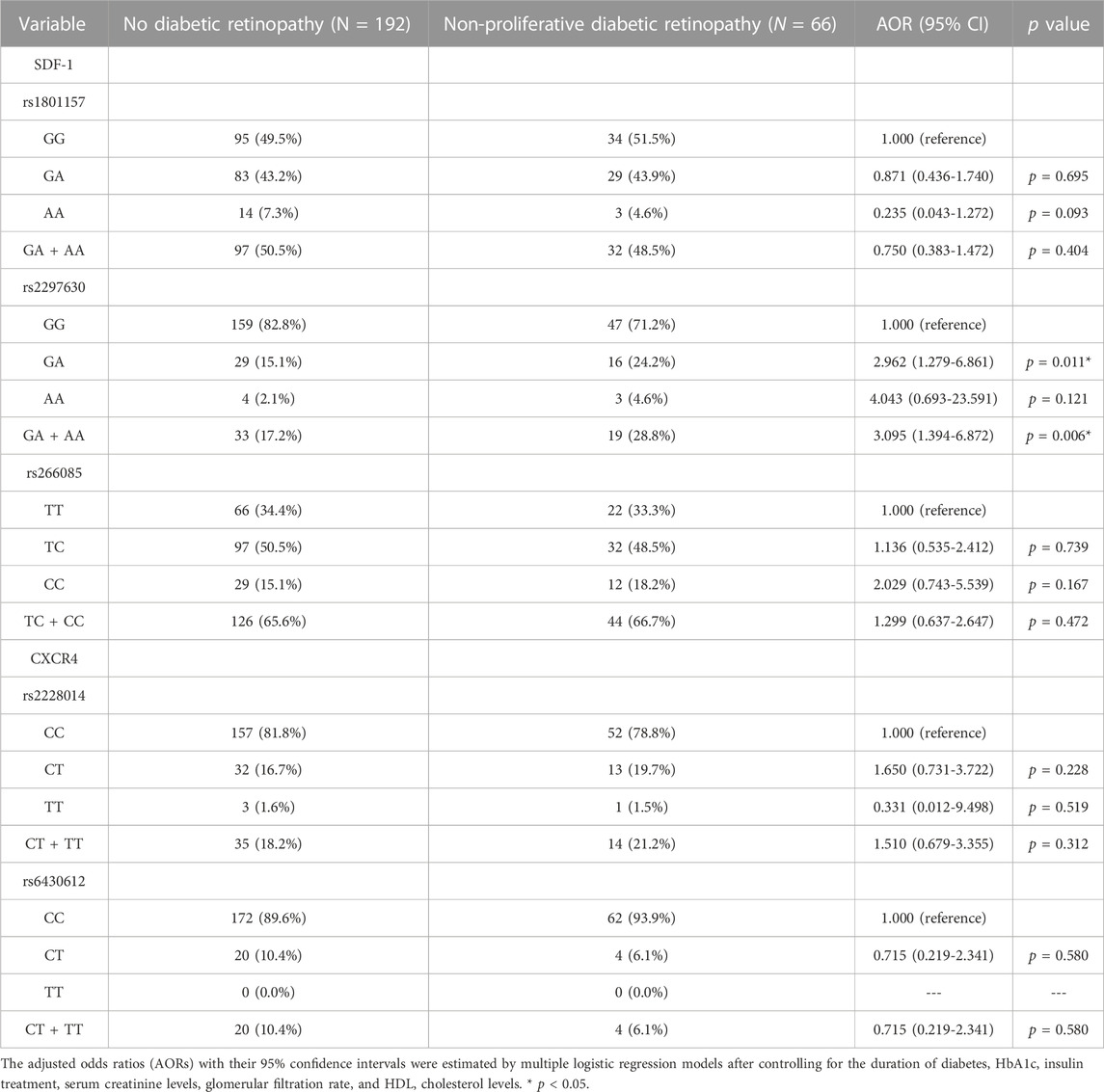
TABLE 5. Odds ratios (ORs) and 95% confidence intervals (CIs) of diabetic retinopathy associated with SDF-1α/CXCR4 axis genotypic frequencies in age ≤60.
The correlations of SDF-1 SNP rs2297630 with SDF-1 expression levels
Since the SDF-1 SNP rs2297630 was found to be associated with the NPDR groups, additional analyses using public datasets were performed to increase its putative functional relevance. By using the Genotype-Tissue Expression (GTEx) Portal, the results revealed that the SDF-1 SNP rs2297630 GA and AA genotypic variants have higher SDF-1 expression than the GG wild-type alleles (p = 0.000016) (Figure 1).
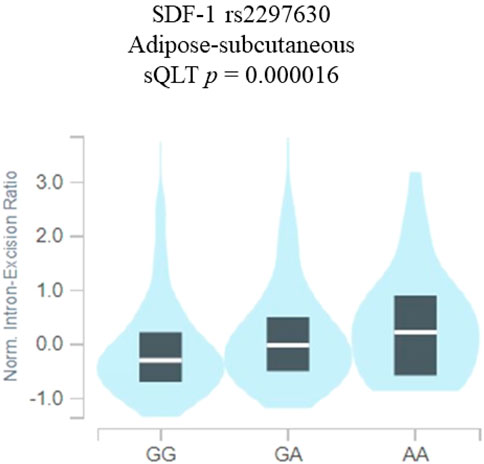
FIGURE 1. SDF-1 SNP rs2297630 regulates the expression of SDF-1. eQTL analysis of SDF-1 SNP rs2297630 in adipose subcutaneous tissues based on data from the GTEx portal. p values are calculated with the linear regression model.
Discussion
Our study demonstrated that rs2297630 of the SDF-1 gene was associated with NPDR. The SDF-1 and CXCR4 signaling pathway was considered to have roles in angiogenesis, tumor growth, embryogenesis, and wound healing (Sadri et al., 2022). A previous study showed that SDF-1 bound to its receptor CXCR4 on the endothelial cells and induced angiogenesis by releasing vascular endothelial growth factors (VEGF) (Mirshahi et al., 2000). VEGF was reported to induce expression of CXCR4 on the endothelial cells (Salcedo et al., 1999). The signaling pathway was also related to breast cancer, prostate cancer, lung cancer, leukemia, and pancreatic cancer (Ueda et al., 2006; Juarez et al., 2007; Nervi et al., 2009; Gao et al., 2010; Shi et al., 2020; Wang et al., 2021; Luo et al., 2022). Many SDF-1 and CXCR4 SNPs were found to be related to different tumors.
The genetic factors, linkage analyses, candidate gene studies, and genome-wide association studies (GWAS) were found to be correlated with DR risk or progression (Cabrera et al., 2020; Forrest et al., 2021). In a genome-wide polygenic risk score study, Forrest et al. established the significant polygenic underpinnings of DR (Forrest et al., 2021). Moreover, in a genome-wide association study in 286 Mexican Americans with type 2 diabetes, Fu et al. reported that CAMK4 (calcium/calmodulin-dependent protein kinase IV) rs2300782 and FMN1 (formin 1) gene SNP rs10519765 were associated with severe diabetic retinopathy (Fu et al., 2010). In a systematic meta-analysis study, Abhary et al. concluded that variations within the aldose reductase gene are highly significantly associated with DR development (Abhary et al., 2009). Therefore, the screening of SNPs is useful to discover the genetic risk factors of DR.
The current study revealed that the distribution frequency of SDF-1 SNP rs2297630 GA + AA is significantly higher in NPDR patients than non-DR patients. The rs2297630 SNP is located in intron 3, +8906 of the SDF-1 gene (Xiao et al., 2008; Ku et al., 2013). Individuals with rs2297630 AA genotype were reported to have higher SDF-1 levels and a lower circulating endothelial progenitor cells number (Xiao et al., 2008). Our results also revealed that the SDF-1 SNP rs2297630 GA and AA genotypes have higher SDF-1 expression than the GG wild-type alleles (Figure 1). Xiao et al. reported that this SDF-1 SNP rs2297630 can affect SDF-1 expression or mRNA splicing to alter SDF-1 expression level (Xiao et al., 2008; Lin et al., 2022). Moreover, the rs2297630 polymorphism was found to be significantly linked to many diseases (Yin et al., 2019; Ji et al., 2023; Wang et al., 2023). The G allele of rs2297630 in SDF-1 was found to be associated with a higher risk of hypertension (Wang et al., 2023). The A allele of SDF-1 SNP rs2297630 was found to be a risk factor for pediatric acute lymphoblastic lymphoma (Ji et al., 2023). Rs2297630 AA genotype was reported to increase the risk of type 2 diabetes mellitus development (Yin et al., 2019). The A allele of rs2297630 was related to the increased production of interleukin 6 (Wang et al., 2019), which was thought to be associated with not only the occurrence of sepsis but also with diabetes mellitus and diabetic retinopathy (Mocan et al., 2006; Chen et al., 2017; Akbari and Hassan-Zadeh, 2018; Yao et al., 2019).
There are some limitations in our study. First, the case numbers in some subgroups were too low to be calculated adequately and may have led to statistical bias. Second, the diagnosis and staging of DR were conducted by different ophthalmologists in our hospital. Although our doctors used the same diagnostic criteria of non-DR, NPDR, and PDR, the clinical judgement may have varied. Third, the longitudinal effect of SDF-1 and CXCR4 SNPs could not be evaluated because of the case–control design of this study.
In conclusion, the distribution frequency of SDF-1 SNP rs2297630 GA + AA is more prevalent in NPDR patients than non-DR patients. Genetic analysis of the SDF-1 polymorphism may be suggested for DR individuals. Larger prospective studies to evaluate the association between the SDF-1 and CXCR4 polymorphism and the treatment outcome are necessary.
Data availability statement
The original contributions presented in the study are included in the article/Supplementary Material, further inquiries can be directed to the corresponding author.
Ethics statement
The studies involving humans were approved by the project identification code: CS1-20048. The studies were conducted in accordance with the local legislation and institutional requirements. The participants provided their written informed consent to participate in this study.
Author contributions
S-YP: Conceptualization, Writing–original draft, Writing–review and editing. C-CC: Formal Analysis, Methodology, Writing–original draft. Y-SH: Supervision, Writing–original draft. C-HY: Supervision, Writing–original draft. C-YL: Formal Analysis, Writing–original draft. S-FY: Conceptualization, Formal Analysis, Writing–original draft, Writing–review and editing.
Funding
The author(s) declare financial support was received for the research, authorship, and/or publication of this article. This research was funded by Jen-Ai Hospital, Taichung, Taiwan, Grant Number JMRPGJM0051.
Conflict of interest
The authors declare that the research was conducted in the absence of any commercial or financial relationships that could be construed as a potential conflict of interest.
Publisher’s note
All claims expressed in this article are solely those of the authors and do not necessarily represent those of their affiliated organizations, or those of the publisher, the editors and the reviewers. Any product that may be evaluated in this article, or claim that may be made by its manufacturer, is not guaranteed or endorsed by the publisher.
References
Abhary, S., Hewitt, A. W., Burdon, K. P., and Craig, J. E. (2009). A systematic meta-analysis of genetic association studies for diabetic retinopathy. Diabetes 58, 2137–2147. doi:10.2337/db09-0059
Agardh, E., Lundstig, A., Perfilyev, A., Volkov, P., Freiburghaus, T., Lindholm, E., et al. (2015). Genome-wide analysis of DNA methylation in subjects with type 1 diabetes identifies epigenetic modifications associated with proliferative diabetic retinopathy. BMC Med. 13, 182. doi:10.1186/s12916-015-0421-5
Akbari, M., and Hassan-Zadeh, V. (2018). IL-6 signalling pathways and the development of type 2 diabetes. Inflammopharmacology 26, 685–698. doi:10.1007/s10787-018-0458-0
Bakri, S. J., Malhotra, M., and Stoilov, I. (2022). Course of diabetic retinopathy in untreated fellow eyes in ride and rise: a post hoc analysis. Ophthalmic Surg. Lasers Imaging Retina 53, 202–207. doi:10.3928/23258160-20220311-03
Bhutto, I. A., Mcleod, D. S., Merges, C., Hasegawa, T., and Lutty, G. A. (2006). Localisation of SDF-1 and its receptor CXCR4 in retina and choroid of aged human eyes and in eyes with age related macular degeneration. Br. J. Ophthalmol. 90, 906–910. doi:10.1136/bjo.2006.090357
Butler, J. M., Guthrie, S. M., Koc, M., Afzal, A., Caballero, S., Brooks, H. L., et al. (2005). SDF-1 is both necessary and sufficient to promote proliferative retinopathy. J. Clin. Invest. 115, 86–93. doi:10.1172/JCI22869
Cabrera, A. P., Mankad, R. N., Marek, L., Das, R., Rangasamy, S., Monickaraj, F., et al. (2020). Genotypes and phenotypes: a search for influential genes in diabetic retinopathy. Int. J. Mol. Sci. 21, 2712. doi:10.3390/ijms21082712
Chang, C. C., Chen, S. C., Hsieh, Y. H., Chen, Y. C., Chen, T. Y., Chu, Y. H., et al. (2009). Stromal cell-derived factor-1 but not its receptor, CXCR4, gene variants increase susceptibility and pathological development of hepatocellular carcinoma. Clin. Chem. Lab. Med. 47, 412–418. doi:10.1515/CCLM.2009.092
Chen, Y. L., Qiao, Y. C., Pan, Y. H., Xu, Y., Huang, Y. C., Wang, Y. H., et al. (2017). Correlation between serum interleukin-6 level and type 1 diabetes mellitus: a systematic review and meta-analysis. Cytokine 94, 14–20. doi:10.1016/j.cyto.2017.01.002
Cheung, N., Mitchell, P., and Wong, T. Y. (2010). Diabetic retinopathy. Lancet 376, 124–136. doi:10.1016/S0140-6736(09)62124-3
Cho, H., and Sobrin, L. (2014). Genetics of diabetic retinopathy. Curr. Diab Rep. 14, 515. doi:10.1007/s11892-014-0515-z
Chuang, C. C., Wang, K., Yang, Y. S., Kornelius, E., Tang, C. H., Lee, C. Y., et al. (2022a). Association of long noncoding RNA HOTAIR polymorphism and the clinical manifestations of diabetic retinopathy. Int. J. Environ. Res. Public Health 19, 14592. doi:10.3390/ijerph192114592
Chuang, C. C., Yang, Y. S., Kornelius, E., Huang, C. N., Hsu, M. Y., Lee, C. Y., et al. (2022b). Impact of long noncoding RNA LINC00673 genetic variants on susceptibility to diabetic retinopathy. Front. Genet. 13, 889530. doi:10.3389/fgene.2022.889530
Dar, A., Kollet, O., and Lapidot, T. (2006). Mutual, reciprocal SDF-1/CXCR4 interactions between hematopoietic and bone marrow stromal cells regulate human stem cell migration and development in NOD/SCID chimeric mice. Exp. Hematol. 34, 967–975. doi:10.1016/j.exphem.2006.04.002
Djuric, Z., Sharei, V., Rudofsky, G., Morcos, M., Li, H., Hammes, H. P., et al. (2010). Association of homozygous SDF-1 3'A genotype with proliferative diabetic retinopathy. Acta Diabetol. 47, 79–82. doi:10.1007/s00592-009-0119-2
Forrest, I. S., Chaudhary, K., Paranjpe, I., Vy, H. M. T., Marquez-Luna, C., Rocheleau, G., et al. (2021). Genome-wide polygenic risk score for retinopathy of type 2 diabetes. Hum. Mol. Genet. 30, 952–960. doi:10.1093/hmg/ddab067
Fu, Y. P., Hallman, D. M., Gonzalez, V. H., Klein, B. E., Klein, R., Hayes, M. G., et al. (2010). Identification of diabetic retinopathy genes through a genome-wide association study among Mexican-Americans from starr county, Texas. J. Ophthalmol. 2010, 861291. doi:10.1155/2010/861291
Gao, Z., Wang, X., Wu, K., Zhao, Y., and Hu, G. (2010). Pancreatic stellate cells increase the invasion of human pancreatic cancer cells through the stromal cell-derived factor-1/CXCR4 axis. Pancreatology 10, 186–193. doi:10.1159/000236012
Gharibi, S., Aghaei, S., Shahvazian, E., Mahmoodi, M. B., Sahami-Fard, M. H., Yazd, E. F., et al. (2022). Association of CXCL12 and CXCR4 genes polymorphism with proliferative diabetic retinopathy in diabetic patients. J. Shahid Sadoughi Univ. Med. Sci. doi:10.18502/ssu.v30i7.10750
Guleng, B., Tateishi, K., Ohta, M., Kanai, F., Jazag, A., Ijichi, H., et al. (2005). Blockade of the stromal cell-derived factor-1/CXCR4 axis attenuates in vivo tumor growth by inhibiting angiogenesis in a vascular endothelial growth factor-independent manner. Cancer Res. 65, 5864–5871. doi:10.1158/0008-5472.CAN-04-3833
Hsiao, P. C., Chen, M. K., Su, S. C., Ueng, K. C., Chen, Y. C., Hsieh, Y. H., et al. (2010). Hypoxia inducible factor-1alpha gene polymorphism G1790A and its interaction with tobacco and alcohol consumptions increase susceptibility to hepatocellular carcinoma. J. Surg. Oncol. 102, 163–169. doi:10.1002/jso.21539
Ji, H., Li, F., Song, L., Xing, Y., Liu, G., Lu, Y., et al. (2023). Inflammation-related gene polymorphisms associated with childhood acute lymphoblastic leukemia. J. Pediatr. Hematol. Oncol. 45, e9–e13. doi:10.1097/MPH.0000000000002533
Jin, D. K., Shido, K., Kopp, H. G., Petit, I., Shmelkov, S. V., Young, L. M., et al. (2006). Cytokine-mediated deployment of SDF-1 induces revascularization through recruitment of CXCR4+ hemangiocytes. Nat. Med. 12, 557–567. doi:10.1038/nm1400
Juarez, J., Dela Pena, A., Baraz, R., Hewson, J., Khoo, M., Cisterne, A., et al. (2007). CXCR4 antagonists mobilize childhood acute lymphoblastic leukemia cells into the peripheral blood and inhibit engraftment. Leukemia 21, 1249–1257. doi:10.1038/sj.leu.2404684
Kataoka, S. Y., Lois, N., Kawano, S., Kataoka, Y., Inoue, K., and Watanabe, N. (2023). Fenofibrate for diabetic retinopathy. Cochrane Database Syst. Rev. 6, CD013318. doi:10.1002/14651858.CD013318.pub2
Keles, A., Sonmez, K., Erol, Y. O., Ayyildiz, S. N., and Ogus, E. (2021). Vitreous levels of vascular endothelial growth factor, stromal cell-derived factor-1α, and angiopoietin-like protein 2 in patients with active proliferative diabetic retinopathy. Graefes Arch. Clin. Exp. Ophthalmol. 259, 53–60. doi:10.1007/s00417-020-04889-0
Ku, F. C., Tsai, C. R., Der Wang, J., Wang, C. H., Chang, T. K., and Hwang, W. L. (2013). Stromal-derived factor-1 gene variations in pediatric patients with primary immune thrombocytopenia. Eur. J. Haematol. 90, 25–30. doi:10.1111/ejh.12025
Lee, C. M., Yang, Y. S., Kornelius, E., Huang, C. N., Hsu, M. Y., Lee, C. Y., et al. (2022). Association of long non-coding RNA growth arrest-specific 5 genetic variants with diabetic retinopathy. Genes (Basel) 13, 584. doi:10.3390/genes13040584
Li, Z., Song, Y., Xu, Y., Shen, Y., Zhang, N., Yang, M., et al. (2020). Identification of Leukocyte telomere length-related genetic variants contributing to predisposition of Esophageal Squamous Cell Carcinoma. J. Cancer 11, 5025–5031. doi:10.7150/jca.45165
Lin, K. Y., Hsih, W. H., Lin, Y. B., Wen, C. Y., and Chang, T. J. (2021). Update in the epidemiology, risk factors, screening, and treatment of diabetic retinopathy. J. Diabetes Investig. 12, 1322–1325. doi:10.1111/jdi.13480
Lin, S., Zheng, Y., Wang, M., Zhou, L., Zhu, Y., Deng, Y., et al. (2022). Associations of CXCL12 polymorphisms with clinicopathological features in breast cancer: a case-control study. Mol. Biol. Rep. 49, 2255–2263. doi:10.1007/s11033-021-07047-9
Luo, F., Su, Y., Zhang, Z., and Li, J. (2022). Bone marrow mesenchymal stem cells promote the progression of prostate cancer through the SDF-1/CXCR4 axis in vivo and vitro. Clin. Transl. Oncol. 24, 892–901. doi:10.1007/s12094-021-02740-4
Mankoč Ramuš, S., Pungeršek, G., Petrovič, M. G., and Petrovič, D. (2021). The GG genotype of erythropoietin rs1617640 polymorphism affects the risk of proliferative diabetic retinopathy in Slovenian subjects with type 2 diabetes mellitus: enemy or ally? Acta Ophthalmol. 99, e1382–e1389. doi:10.1111/aos.14813
Meleth, A. D., Agron, E., Chan, C. C., Reed, G. F., Arora, K., Byrnes, G., et al. (2005). Serum inflammatory markers in diabetic retinopathy. Invest. Ophthalmol. Vis. Sci. 46, 4295–4301. doi:10.1167/iovs.04-1057
Mirshahi, F., Pourtau, J., Li, H., Muraine, M., Trochon, V., Legrand, E., et al. (2000). SDF-1 activity on microvascular endothelial cells: consequences on angiogenesis in in vitro and in vivo models. Thromb. Res. 99, 587–594. doi:10.1016/s0049-3848(00)00292-9
Mocan, M. C., Kadayifcilar, S., and Eldem, B. (2006). Elevated intravitreal interleukin-6 levels in patients with proliferative diabetic retinopathy. Can. J. Ophthalmol. 41, 747–752. doi:10.3129/i06-070
Mohamed, Y. A., Taha, G. E. M., Ezzat, D. A., Ahmed, A. B., Doudar, N. A., Badawy, O., et al. (2022). Stromal derived factor-1 gene polymorphism in pediatric immune thrombocytopenia. J. Pediatr. Hematol. Oncol. 44, e319–e323. doi:10.1097/MPH.0000000000002342
Nervi, B., Ramirez, P., Rettig, M. P., Uy, G. L., Holt, M. S., Ritchey, J. K., et al. (2009). Chemosensitization of acute myeloid leukemia (AML) following mobilization by the CXCR4 antagonist AMD3100. Blood 113, 6206–6214. doi:10.1182/blood-2008-06-162123
Petrovic, D. (2013). Candidate genes for proliferative diabetic retinopathy. Biomed. Res. Int. 2013, 540416. doi:10.1155/2013/540416
Runmin, G., Jiamei, J., Zhiliang, J., Yonghua, C., Zhizhou, S., Guizhou, T., et al. (2018). Genetic variation of CXCR4 and risk of coronary artery disease: epidemiological study and functional validation of CRISPR/Cas9 system. Oncotarget 9, 14077–14083. doi:10.18632/oncotarget.23491
Sadri, F., Rezaei, Z., and Fereidouni, M. (2022). The significance of the SDF-1/CXCR4 signaling pathway in the normal development. Mol. Biol. Rep. 49, 3307–3320. doi:10.1007/s11033-021-07069-3
Salcedo, R., Wasserman, K., Young, H. A., Grimm, M. C., Howard, O. M., Anver, M. R., et al. (1999). Vascular endothelial growth factor and basic fibroblast growth factor induce expression of CXCR4 on human endothelial cells: in vivo neovascularization induced by stromal-derived factor-1alpha. Am. J. Pathol. 154, 1125–1135. doi:10.1016/s0002-9440(10)65365-5
Shi, Y., Riese, D. J., and Shen, J. (2020). The role of the CXCL12/CXCR4/CXCR7 chemokine Axis in cancer. Front. Pharmacol. 11, 574667. doi:10.3389/fphar.2020.574667
Simó-Servat, O., Hernández, C., and Simó, R. (2013). Genetics in diabetic retinopathy: current concepts and new insights. Curr. Genomics 14, 289–299. doi:10.2174/13892029113149990008
Su, S. C., Hsieh, M. J., Lin, C. W., Chuang, C. Y., Liu, Y. F., Yeh, C. M., et al. (2018). Impact of HOTAIR gene polymorphism and environmental risk on oral cancer. J. Dent. Res. 97, 717–724. doi:10.1177/0022034517749451
Tee, Y. T., Yang, S. F., Wang, P. H., Tsai, H. T., Lin, L. Y., Lee, S. K., et al. (2012). G801A polymorphism of human stromal cell-derived factor 1 gene raises no susceptibility to neoplastic lesions of uterine cervix. Int. J. Gynecol. Cancer 22, 1297–1302. doi:10.1097/IGC.0b013e318265d334
Teng, Y. H., Liu, T. H., Tseng, H. C., Chung, T. T., Yeh, C. M., Li, Y. C., et al. (2009). Contribution of genetic polymorphisms of stromal cell-derived factor-1 and its receptor, CXCR4, to the susceptibility and clinicopathologic development of oral cancer. Head. Neck 31, 1282–1288. doi:10.1002/hed.21094
Teo, Z. L., Tham, Y. C., Yu, M., Chee, M. L., Rim, T. H., Cheung, N., et al. (2021). Global prevalence of diabetic retinopathy and projection of burden through 2045: systematic review and meta-analysis. Ophthalmology 128, 1580–1591. doi:10.1016/j.ophtha.2021.04.027
Ueda, Y., Neel, N. F., Schutyser, E., Raman, D., and Richmond, A. (2006). Deletion of the COOH-terminal domain of CXC chemokine receptor 4 leads to the down-regulation of cell-to-cell contact, enhanced motility and proliferation in breast carcinoma cells. Cancer Res. 66, 5665–5675. doi:10.1158/0008-5472.CAN-05-3579
Wang, S., Wang, M., Peng, H., Tian, Y., Guo, H., Wang, J., et al. (2023). Synergism of cell adhesion regulatory genes and instant air pollutants on blood pressure elevation. Chemosphere 312, 136992. doi:10.1016/j.chemosphere.2022.136992
Wang, X., Zhang, A. Q., Gu, W., Wen, D. L., Lu, H. X., Yang, J. H., et al. (2019). Clinical relevance of single nucleotide polymorphisms in the CXCL1 and CXCL12 genes in patients with major trauma. J. Trauma Acute Care Surg. 86, 440–447. doi:10.1097/TA.0000000000002141
Wang, Y., Lan, W., Xu, M., Song, J., Mao, J., Li, C., et al. (2021). Cancer-associated fibroblast-derived SDF-1 induces epithelial-mesenchymal transition of lung adenocarcinoma via CXCR4/β-catenin/PPARδ signalling. Cell Death Dis. 12, 214. doi:10.1038/s41419-021-03509-x
Wu, D., Jin, L., and Xu, H. (2019). The effects of the CXCR4 antagonist, AMD3465, on human retinal vascular endothelial cells (hRVECs) in a high glucose model of diabetic retinopathy. Med. Sci. Monit. 25, 6946–6954. doi:10.12659/MSM.917186
Xiao, Q., Ye, S., Oberhollenzer, F., Mayr, A., Jahangiri, M., Willeit, J., et al. (2008). SDF1 gene variation is associated with circulating SDF1alpha level and endothelial progenitor cell number: the Bruneck Study. PLoS One 3, e4061. doi:10.1371/journal.pone.0004061
Yao, Y., Li, R., Du, J., Long, L., Li, X., and Luo, N. (2019). Interleukin-6 and diabetic retinopathy: a systematic review and meta-analysis. Curr. Eye Res. 44, 564–574. doi:10.1080/02713683.2019.1570274
Keywords: diabetic retinopathy, SDF-1, CXCR4, single-nucleotide polymorphisms, proliferative diabetic retinopathy
Citation: Peng S-Y, Chuang C-C, Hwang Y-S, Yen C-H, Lee C-Y and Yang S-F (2023) Association of SDF-1 and its receptor CXCR4 polymorphisms on the susceptibility of diabetic retinopathy in the Taiwanese population. Front. Genet. 14:1296773. doi: 10.3389/fgene.2023.1296773
Received: 19 September 2023; Accepted: 13 November 2023;
Published: 23 November 2023.
Edited by:
Shi Song Rong, Massachusetts Eye and Ear Infirmary and Harvard Medical School, United StatesReviewed by:
Daniel Petrovič, University of Ljubljana, SloveniaFatmahan Atalar, Istanbul University, Türkiye
Copyright © 2023 Peng, Chuang, Hwang, Yen, Lee and Yang. This is an open-access article distributed under the terms of the Creative Commons Attribution License (CC BY). The use, distribution or reproduction in other forums is permitted, provided the original author(s) and the copyright owner(s) are credited and that the original publication in this journal is cited, in accordance with accepted academic practice. No use, distribution or reproduction is permitted which does not comply with these terms.
*Correspondence: Shun-Fa Yang, eXNmQGNzbXUuZWR1LnR3