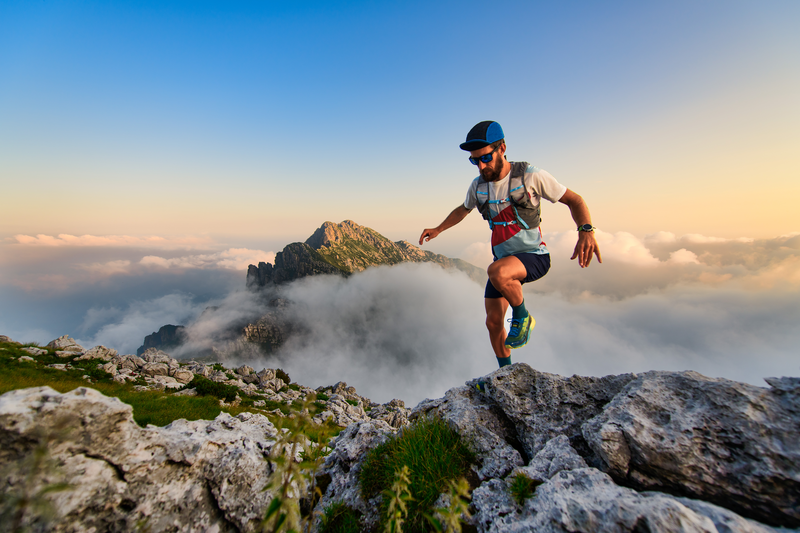
95% of researchers rate our articles as excellent or good
Learn more about the work of our research integrity team to safeguard the quality of each article we publish.
Find out more
CASE REPORT article
Front. Genet. , 08 January 2024
Sec. Human and Medical Genomics
Volume 14 - 2023 | https://doi.org/10.3389/fgene.2023.1292085
This article is part of the Research Topic Diagnosis and Identification of Novel Disorders and Ultra-Rare Disorders in Science and Clinical Routine View all 5 articles
Branchiootic syndrome (BOS) is a rare, autosomal dominant syndrome characterized by malformations of the ear associated with hearing loss, second branchial arch anomalies, and the absence of renal anomalies. Herein, we report the case of an 8-year-old male patient with BOS. The proband also experiences mixed conductive and sensorineural hearing loss in the right ear, and severe-to-profound sensorineural hearing loss in the left ear. Preauricular pits, branchial fistulae, and cochlear hypoplasia were present bilaterally. Type III cup-shaped ear, and external auditory canal stenosis were detected in the right ear. Lateral semicircular canal-vestibule dysplasia was detected in the left ear. Moreover, the patient had unilateral secretory otitis media (SOM) in the right ear and bilateral vestibular hypofunction (VH), which has not been reported in previous studies. The patient’s hearing on the right side was restored to nearly normal after myringotomy. Whole exome sequencing identified a novel frameshift mutation in EYA1 (NM_000503.6): c.1697_1698delinT [p.(Lys566IlefsTer73)] in the proband, which was defined a “pathogenic” mutation according to American College of Medical Genetics and Genomics guidelines. This is the first report of a child presenting with BOS, SOM and VH, which expands the known clinical manifestations of this syndrome. We also observed a novel EYA1 gene mutation in this patient with BOS, which enriches the mutation map and provides a reference for genetic diagnosis of this syndrome.
Branchiootorenal syndrome (BOR1, MIM# 113650) is an autosomal dominant disorder characterized by branchiogenic malformations, various degrees of hearing loss, and renal involvement (Zheng et al., 2021). Branchiootic syndrome (BOS1, MIM# 602588) is distinguished by normal renal anatomy and function. The incidence of this disorder is approximately 1 in 40,000 live births, and accounts for about 2% of children with severe deafness (Li et al., 2018).
Molecular studies have been performed in patients with BOR/BOS, which revealed the most known causative genes were EYA1 (BOR1, MIM# 113650, BOS1, MIM# 602588), SIX1 (BOS3, MIM# 608389), and SIX5 (BOR2, MIM# 610896). Of these genes, EYA1 variants—including frameshifts, and partial duplications—account for the majority of diagnosed patients (Dantas et al., 2015; Wang et al., 2018). EYA1 is a transcription factor important in developing several tissues, including the branchial arches, kidney, eyes, and ear. However, no significant relationship has been shown between the nature of the mutations and the clinical features associated with BOR/BOS. Whole-exome sequencing (WES) is an efficient strategy to identify the coding sequences of a genome, in which 85% of pathogenic mutations are located, and has shown significant advantages in diagnosing genetic disorders (Rabbani et al., 2014; Yang et al., 2015).
Herein, we report a Chinese child with BOS with a novel pathogenic mutation in the EYA1 gene and secretory otitis media (SOM) and vestibular hypofunction (VH), which has not been previously reported. WES and Sanger sequencing was used to analyze the proband’s DNA sample. We conducted this study to expand both the mutational spectrum and clinical characteristics of BOS.
An 8-year-old boy presented to The Second Xiangya Hospital of Central South University, China with bilateral hearing loss, preauricular pits, and branchial fistulae, along with an external ear anomaly in the right ear (Figure 1 B1-B4). The patient also had SOM in the right ear, which caused ear fullness and further mixed hearing loss. After extensive audiological and radiological evaluations, the patient, who has no past medical history, was diagnosed with BOS. He and his family then enrolled in our study to undergo further evaluations (Figures 1A–Ⅱ:2).
FIGURE 1. Clinical manifestations and audiological evaluation. (A) Pedigree of family. (B) The proband had type III cup-shaped right ear (red arrow (B1)), external auditory canal stenosis, bilateral preauricular pits (white arrows (B1–B2)), and bilateral branchial fistulae (white arrows (B3–B4)). (C) Pure-tone audiometry revealed mixed hearing loss in the right ear (average air conduction threshold, 59 dB; average bone conduction threshold, 26 dB (C1)), and severe-to-profound sensorineural hearing loss in the left ear (C2). (D) Acoustic immittance test showed type “C” tympanogram in the right ear that indicates secretory otitis media (D1), and type “A” tympanogram in the left ear (D2).
Micro-otoscopy and pure-tone audiometry (PTA) were used to evaluate hearing levels (Figure 1 C1-C2). High-resolution computed tomography (HRCT) of the temporal bone was performed to determine the middle and inner ear morphologies (Figure 2). Renal examinations, including renal ultrasound, renal function tests, and urinalysis were performed to screen for renal abnormalities. He subsequently underwent myringotomy with grommet insertion at otolaryngology ward of the Second Xiangya Hospital. Three months after surgery, an objective audiometry comprised of acoustic immittance, distortion product otoacoustic emission, auditory brainstem response (ABR), and auditory steady-state response were conducted. Vestibular function and vestibular evoked myogenic potential (VEMP) were also assessed.
FIGURE 2. HRCT of proband (A) Cochlear hypoplasia in the right ear (arrow). (B) Lateral semicircular canal-vestibule dysplasia in the left ear (arrow). (C) Cochlear hypoplasia with less than two turns in the left ear. (D) Soft tissue shadow in the right middle ear (red arrow). Cochlear hypoplasia on both sides (white arrows). (E) Lateral semicircular canal-vestibule dysplasia in the left ear (arrow). (A–C) Coronal view; (D,E) Axial view. HRCT, high-resolution computed tomography.
Peripheral blood samples were collected from the proband and his family members. Genomic DNA was extracted using a DNA extraction kit (Agilent Technologies), and the DNA quality of the samples was controlled using a NanoDrop 2000 Spectrophotometer (ThermoFisher Scientific). Purified DNA was quantified using Qubit (ThermoFisher Scientific).
The SureSelect Human All Exon V6 capture system (Agilent Technologies) was used for library construction and capture. The sequencing data were obtained in FASTQ format by high-throughput sequencing using the 2 × 150 bp double-terminal sequencing mode.
After quality control, the FASTQ data were aligned to hg38 (GCF_000001405.26) from the National Center for Biotechnology Information (https://www.ncbi.nlm.nih.gov) by using Burrows-Wheeler Aligner 6. GATK software (Broad Institute) was used to determine single nucleotide variants (SNVs) and small fragment insertion-deletion mutations (InDels). Data filtering was completed using the dbSNP, ExAC03, HapMap, and 1,000 Genomes databases, and sites with a mutation frequency of less than 1% were retained. The mutation site with the highest priority was defined as “First1” and was considered as the candidate gene. Finally, we used Phenolyzer software (http://phenolyzer.wglab.org/) to predict gene pathogenicity of the candidate gene in BOS.
Candidate mutation was validated using Sanger sequencing to verify the DNA sequence variants detected by WES. Primers were designed for the target region using Primer3 online software (http://bioinfo.ut.ee/primer3-0.4.0/). The forward and reverse primers were F: 5-CTGCACATATTCATCACGTTTCACA-3; and R: 5-CACTAGGAAAAGAAAGCTGTTTTGAGAG-3, respectively. After purification with shrimp alkaline phosphatase and exonuclease I (Agilent Technologies), the PCR products were sequenced on an ABI 3730XL DNA Analyzer (ThermoFisher Scientific). The sequence reads were analyzed using the PolyPhred software (https://droog.gs.washington.edu/polyphred/).
Medical history and physical examination of the family revealed that only the child with BOS presented with preauricular pits, branchial fistulae, type III cup-shaped ear, external auditory canal stenosis, inner ear anomaly, and hearing loss (Figures 1, 2). This was the first time that hearing loss was diagnosed in the patient, and no previous ear related intervention had been performed. The proband and his family members had no history of using ototoxic drug or noise exposure. The preoperative assessment results of the proband were as follows; pure-tone audiometry showed mixed hearing loss in the right ear, where the average air and bone conduction thresholds were 59 and 26 dB, respectively, and severe-to-profound sensorineural hearing loss in the left ear (Figure 1 C1-C2). Acoustic immittance test showed type “A” tympanogram in the left ear and type “C” tympanogram in the right ear that indicated SOM (Figure 1 D1-D2). Acoustic reflex thresholds could not be obtained in both ears through 500–4,000 Hz. Videonystagmography revealed normal ocular, positional, Dix-Hallpike and roll tests, ruling out a diagnosis of benign paroxysmal positional vertigo. The caloric test revealed bilateral hyporeflexia with reflexes of 2.6 deg/s and 5.0 deg/s after cool water stimulation, and 5.5 deg/s and 4.5 deg/s after warm water stimulation, on the right and left sides, respectively. VEMP were absent after stimulation on both sides. In the right inner ear, HRCT of the temporal bone indicated soft tissue shadow in the right middle ear and cochlear hypoplasia. In the left inner ear, HRCT revealed cochlear hypoplasia and lateral semicircular canal-vestibule (Figure 2). Abdominal color doppler ultrasound, and routine urine and blood biochemical profiles showed no abnormalities. The proband underwent myringotomy with grommet insertion in the right ear, during which viscous fluid accumulation was visible in the middle ear. Consequently, the postoperative ABR was 30 dBnHL in the right ear, indicating a significant improvement compared to the preoperative value.
After quality assessment of the raw data and comparative analysis with reference sequence (hg38), we obtained the following quality assessment data. On average, we generated >12 G of total data per sample. The average ratio of the base Q30 was >91%, and the average mean coverage sequencing depth for the intended target was 106X. When measured at 20X depth, 97% of the target was covered. These results suggest that the data captured by WES is adequate for reliably detecting DNA variants for further analysis.
Mutation sites were classified according to their position relative to the gene regions (Figure 3A1) and functional positions (Figure 3A2). Based on the software analysis results, we got the top 10 genes. The EYA1 gene has the highest score (Figure 3B).
FIGURE 3. The WES results of the family with BOS. (A1) Distribution ratio of SNVs/InDels sites in different gene regions, and (A2) of different functional types. (B) The histogram of the top 10 genes. The EYA1 gene has the highest score. (C) A sequencing chromatography of the reverse complementary strand of the frameshift variant in the proband. The red arrow indicates a change in base position, and the 73rd codon, followed by lysine (Lys566), has become a stop codon. WES, whole-exome sequencing; BOS, branchiootic syndrome; SNVs, single nucleotide variants; InDels, insertion-deletion mutations.
Bioinformatic analysis of the proband’s WES revealed a heterozygous mutation in. EYA1 (NM_000503.6): c.1697_1698delinT [p.(Lys566IlefsTer73)] at exon 17, where the 566th amino acid changed from lysine to isoleucine. This variant was not found in dbSNP, ExAC03, HapMap, HGMD, or 1,000 Genomes databases and did not exist in the average population. To verify the WES results, we performed Sanger sequencing on all family members and observed that only the proband had the EYA1 gene c.1697_1698delinsT mutation (Figure 3C), whereas other healthy family members had no mutation at this site.
BOS is a rare autosomal dominant disorder with high genotype heterogeneity (Chen et al., 2019). It is expressed differently in various individuals, and currently, there is no evidence of genotype-phenotype correlations in patient with BOS (Unzaki et al., 2018). In 2004, Chang et al. (2004) proposed a widely accepted diagnostic criteria for BOR/BOS using the major and minor criteria. The major criteria include branchial arch anomalies, hearing loss, preauricular pits, and renal anomalies; minor criteria include external, middle, and inner ear anomalies, preauricular tags, facial asymmetry, and palatal abnormalities (Table 1). Patients without a family history are diagnosed with BOR if they meet three or more of the above major criteria or two major and at least two minor criteria (Chang et al., 2004). A previous study reported the frequency of major criteria in BOR as follows: hearing loss 95.4%, preauricular pits 87%, branchial arch anomalies 86.5%, and renal anomalies 58.3% (Li et al., 2020). From a clinical standpoint, hearing loss is the most common and constant feature of the EYA1 gene mutations and is detected in more than 90% of affected individuals (Chang et al., 2004). Treatment of BOS is mainly symptomatic, including the surgical removal of branchial arch fistulae and cochlear implants (Men et al., 2020).
The proband in this study had known BOS clinical presentations such as bilateral preauricular pits, bilateral cervical branchial arch fistulae, hearing loss, right external ear, and bilateral inner ear anomalies. In addition to the above manifestations, the patient had SOM and bilateral VH, phenotypes not previously reported in other studies. While SOM in an 8-year-old child is not rare, SOM in the proband occurred on the same side as the external ear anomaly. This indicates that BOS may affect the Eustachian tube and ear ventilation, which can lead to inflammation. The proband also has VH, which has not previously been associated with BOS. The most common etiology of bilateral VH is gentamicin ototoxicity, followed by other rare entities, such as autoimmune inner ear disease, meningitis, Meniere’s disease and vestibular neuritis; these features were not present in the proband (Hain et al., 2018). HRCT indicated lateral semicircular canal-vestibule dysplasia in the proband’s left ear (Figures 2B, E), which may have caused VH. This finding warrants more attention to the vestibular functions in BOS patients in the future.
Nowadays, WES can sensitively detect sequence variants. In this study, we identified a novel EYA1 frameshift mutation c.1697_1698delinsT [p.(Lys566IlefsTer73)] using read-depth analysis of WES data in this family with BOS and verified by Sanger sequencing in the proband but not in unaffected family members. The EYA1 gene, located on chromosome 8q13.3, is the human homolog of the Drosophila EYA1 gene, which is essential for eye development in this species (Orten et al., 2008). The EYA1 protein is also important for the ear, branchial arches, and kidney development. Animal model studies have shown the EYA1 homozygous-deficient mice lack ears and kidneys, and the EYA1 heterozygous-deficient mice present phenotypes resembling BOR (Xu et al., 1999; Xu et al., 2002). The known pathogenic genes of BOR/BOS include EYA1, SIX1, and SIX5. The EYA1 gene mutations account for approximately 40% of BOR cases, while SIX5 and SIX1 genes account for 5% and less than 1%, respectively (Shah et al., 2020). To date, more than 240 pathological mutations in the EYA1 gene have been reported. In China, EYA1 c.466C>T [p.(Gln156Ter)], and EYA1 c.1735delG [p.(Asp579 fs)] were the first variants of EYA1 identified in 2012 (Wang et al., 2012). With the development of next-generation sequencing technology, an increasing number of novel EYA1 mutations have been reported in Chinese patients with BOS/BOR (Table 2). Han et al. (2021) recruited 7 members of a Chinese family, 4 of whom affected with BOS. EYA1 c.1627C>T [p.(Gln543Ter)] was identified as a novel mutation and pathogenic cause of BOS. Similarly, Xing et al. (2020) also collected blood samples from 6 members of a Chinese family, 4 of whom affected by BOS, and found a novel frameshift variant, c.1075_1077delinsAT [p.(Gly359 fs)] in the EYA1 gene. These results suggest that the BOR/BOS criteria are potential indications for molecular studies to diagnose EYA1-associated syndromes (Chen et al., 2019; Li et al., 2020).
EYA1 is also affected by gene dose effects, and clinical phenotypic heterogeneity may be related to insufficient EYA1 gene dosage. However, gene activity can be recognized only when the number of encoded proteins exceeds a certain threshold. In addition, gene regulation of specific molecules that encode different amounts of gene products explains the wide phenotypic variation in BOS patients between or within families (Wang et al., 2018).
Based on the American College of Medical Genetics and Genomics (ACMG) guidelines, the following three criteria were applied to interpret this EYA1 c.1697_1698delinsT as a pathogenic variant with an important role in EYA1-assoicated conditions. First, it is a frameshift mutation in the EYA1 and causes gene loss-of-function; this is a known disease mechanism (very strong pathogenic criterion; PVS1). The absence of this variation in the proband’s parents suggests that this is a de novo mutation (strong pathogenic criterion; PS2). Finally, since this variant was not found in the Genome Aggregation Database (gnomAD), the rarity of the mutation was recognized (moderate pathogenic criterion; PM2).
This de novo mutation in our proband contributes to an autosomal dominant disorder. According to the law of autosomal dominant inheritance, it is estimated that the offsprings of the proband have a 50% increased risk of inheriting EYA1 pathogenic variants. Therefore, detecting the pathogenic variants for this disease is of great importance, and patients suspected of BOS should be monitored accordingly for associated otologic and second branchial arch anomalies. With the emergence of new sequencing technologies in recent years, many novel pathogenic mutations have been reported, but many unknown pathogenic variants still need to be discovered. The novel EYA1 frameshift mutation discovered in this study can be equip BOS genetic testing in the future for early diagnose of diseases and to assist with the personalized prenatal screening.
We identified a novel heterozygous de novo pathogenic frameshift mutation in the EYA1 gene in a proband with BOS. Previously unreported clinical manifestations of BOS, including bilateral vestibular hypofunction and unilateral secretory otitis media, were observed. Our results expand both the mutational and phenotypic spectra of BOS, suggesting that the mutation of a key amino acid is an etiological factor. Considering the high phenotypic heterogeneity of BOS in patients whose diagnosis is difficult based on clinical manifestations alone, genetic testing for candidate pathogenic gene mutations can successfully complement clinical diagnosis.
The datasets presented in this study can be found in online repositories. The names of the repository/repositories and accession number(s) can be found below: GEO database and the accession number is PRJNA979267.
The studies involving humans were approved by the Ethics Committee of the Second Xiangya Hospital of Central South University. The studies were conducted in accordance with the local legislation and institutional requirements. Written informed consent for participation in this study was provided by the participants’ legal guardians/next of kin. Written informed consent was obtained from the individual(s), and minor(s)’ legal guardian/next of kin, for the publication of any potentially identifiable images or data included in this article.
JH: Writing–original draft, Methodology, Visualization. AM: Writing–review and editing, Visualization. YG: Investigation, Writing–review and editing. JF: Investigation. QY: Investigation. WL: Funding acquisition, Investigation, Writing–review and editing.
The author(s) declare that no financial support was received for the research, authorship, and/or publication of this article.
We are grateful to the patient and his family in our research study. We express our gratitude to everyone on the healthcare team who helped us in conducting this study. We thank Jacqueline Yao for providing valuable comments and helping us revise this manuscript.
The authors declare that the research was conducted in the absence of any commercial or financial relationships that could be construed as a potential conflict of interest.
All claims expressed in this article are solely those of the authors and do not necessarily represent those of their affiliated organizations, or those of the publisher, the editors and the reviewers. Any product that may be evaluated in this article, or claim that may be made by its manufacturer, is not guaranteed or endorsed by the publisher.
The Supplementary Material for this article can be found online at: https://www.frontiersin.org/articles/10.3389/fgene.2023.1292085/full#supplementary-material
Chang, E. H., Menezes, M., Meyer, N. C., Cucci, R. A., Vervoort, V. S., Schwartz, C. E., et al. (2004). Branchio-oto-renal syndrome: the mutation spectrum in EYA1 and its phenotypic consequences. Hum. Mutat. 23 (6), 582–589. doi:10.1002/humu.20048
Chen, P., Liu, H., Lin, Y., Xu, J., Zhu, W., Wu, H., et al. (2019). EYA1 mutations leads to Branchio-Oto syndrome in two Chinese Han deaf families. Int. J. Pediatr. otorhinolaryngology 123, 141–145. doi:10.1016/j.ijporl.2019.05.006
Dantas, V. G., Freitas, E. L., Della-Rosa, V. A., Lezirovitz, K., de Moraes, A. M. S. M., Ramos, S. B., et al. (2015). Novel partial duplication of EYA1 causes branchiootic syndrome in a large Brazilian family. Int. J. audiology 54 (9), 593–598. doi:10.3109/14992027.2015.1030511
Feng, H., Xu, H., Chen, B., Sun, S., Zhai, R., Zeng, B., et al. (2021). Genetic and phenotypic variability in Chinese patients with branchio-oto-renal or branchio-oto syndrome. Front. Genet. 12, 765433. doi:10.3389/fgene.2021.765433
Hain, T. C., Cherchi, M., and Yacovino, D. A. (2018). Bilateral vestibular weakness. Front. neurology 9, 344. doi:10.3389/fneur.2018.00344
Han, R., Xia, Y., Liu, Z., Wu, S., Ye, E., Duan, L., et al. (2021). A mutation of EYA1 gene in a Chinese Han family with Branchio-Oto syndrome. Medicine 100 (25), e24691. doi:10.1097/MD.0000000000024691
Li, G., Shen, Q., Sun, L., Liu, H., An, Y., and Xu, H. (2018). A de novo and novel mutation in the EYA1 gene in a Chinese child with branchio-oto-renal syndrome. Intractable rare Dis. Res. 7 (1), 42–45. doi:10.5582/irdr.2017.01075
Li, H. X., Zhou, P., Tong, M., and Zheng, Y. (2020). Branchial cleft fistula to branchio-oto-renal syndrome: a case report and literature review. J. Int. Med. Res. 48 (7), 300060520926363. doi:10.1177/0300060520926363
Men, M., Li, W., Chen, H., Wu, J., Feng, Y., Guo, H., et al. (2020). Identification of a novel CNV at 8q13 in a family with branchio-oto-renal syndrome and epilepsy. Laryngoscope 130 (2), 526–532. doi:10.1002/lary.27941
Orten, D. J., Fischer, S. M., Sorensen, J. L., Radhakrishna, U., Cremers, C. W. R. J., Marres, H. A. M., et al. (2008). Branchio-oto-renal syndrome (BOR): novel mutations in the EYA1 gene, and a review of the mutational genetics of BOR. Hum. Mutat. 29 (4), 537–544. doi:10.1002/humu.20691
Rabbani, B., Tekin, M., and Mahdieh, N. (2014). The promise of whole-exome sequencing in medical genetics. J. Hum. Genet. 59 (1), 5–15. doi:10.1038/jhg.2013.114
Shah, A. M., Krohn, P., Baxi, A. B., Tavares, A. L. P., Sullivan, C. H., Chillakuru, Y. R., et al. (2020). Six1 proteins with human branchio-oto-renal mutations differentially affect cranial gene expression and otic development. Dis. models Mech. 13 (3), dmm043489. doi:10.1242/dmm.043489
Unzaki, A., Morisada, N., Nozu, K., Ye, M. J., Ito, S., Matsunaga, T., et al. (2018). Clinically diverse phenotypes and genotypes of patients with branchio-oto-renal syndrome. J. Hum. Genet. 63 (5), 647–656. doi:10.1038/s10038-018-0429-8
Wang, S. H., Wu, C. C., Lu, Y. C., Lin, Y. H., Su, Y. N., Hwu, W. L., et al. (2012). Mutation screening of the EYA1, SIX1, and SIX5 genes in an East Asian cohort with branchio-oto-renal syndrome. Laryngoscope 122 (5), 1130–1136. doi:10.1002/lary.23217
Wang, Y. G., Sun, S. P., Qiu, Y. L., Xing, Q. H., and Lu, W. (2018). A novel mutation in EYA1 in a Chinese family with Branchio-oto-renal syndrome. BMC Med. Genet. 19 (1), 139. doi:10.1186/s12881-018-0653-2
Xing, Z. K., Wang, S. Y., Xia, X., Ding, W. J., Duan, L., Cui, X., et al. (2020). Targeted next-generation sequencing identifies a novel frameshift EYA1 variant causing branchio-otic syndrome in a Chinese family. Int. J. Pediatr. otorhinolaryngology 138, 110202. doi:10.1016/j.ijporl.2020.110202
Xu, P. X., Adams, J., Peters, H., Brown, M. C., Heaney, S., and Maas, R. (1999). Eya1-deficient mice lack ears and kidneys and show abnormal apoptosis of organ primordia. Nat. Genet. 23 (1), 113–117. doi:10.1038/12722
Xu, P. X., Zheng, W., Laclef, C., Maire, P., Maas, R. L., Peters, H., et al. (2002). Eya1 is required for the morphogenesis of mammalian thymus, parathyroid and thyroid. Dev. Camb. Engl. 129 (13), 3033–3044. doi:10.1242/dev.129.13.3033
Yang, H., Robinson, P. N., and Wang, K. (2015). Phenolyzer: phenotype-based prioritization of candidate genes for human diseases. Nat. Methods 12, 841–843. doi:10.1038/nmeth.3484
Keywords: branchiootic syndrome, EYA1 gene mutation, whole exome sequencing, secretory otitis media, vestibular hypofunction, case report
Citation: He J, Mahmoudi A, Gu Y, Fu J, Yuan Q and Liu W (2024) Case report: A novel mutation in the EYA1 gene in a child with branchiootic syndrome with secretory otitis media and bilateral vestibular hypofunction. Front. Genet. 14:1292085. doi: 10.3389/fgene.2023.1292085
Received: 11 September 2023; Accepted: 13 December 2023;
Published: 08 January 2024.
Edited by:
Jared C. Roach, Institute for Systems Biology (ISB), United StatesReviewed by:
Miguel Angel Alcántara-Ortigoza, National Institute of Pediatrics, MexicoCopyright © 2024 He, Mahmoudi, Gu, Fu, Yuan and Liu. This is an open-access article distributed under the terms of the Creative Commons Attribution License (CC BY). The use, distribution or reproduction in other forums is permitted, provided the original author(s) and the copyright owner(s) are credited and that the original publication in this journal is cited, in accordance with accepted academic practice. No use, distribution or reproduction is permitted which does not comply with these terms.
*Correspondence: Wei Liu, bGl1d2VpMDA3QGNzdS5lZHUuY24=
Disclaimer: All claims expressed in this article are solely those of the authors and do not necessarily represent those of their affiliated organizations, or those of the publisher, the editors and the reviewers. Any product that may be evaluated in this article or claim that may be made by its manufacturer is not guaranteed or endorsed by the publisher.
Research integrity at Frontiers
Learn more about the work of our research integrity team to safeguard the quality of each article we publish.