- 1Department of Gynecology and Obstetrics, The Fourth Affiliated Hospital, Zhejiang Provincial Clinical Research Center for Obstetrics and Gynecology, Zhejiang University School of Medicine, Yiwu, Zhejiang, China
- 2Reproductive Medicine Center, School of Medicine, The Fourth Affiliated Hospital, Zhejiang University, Yiwu, Zhejiang, China
RNA modifications are mostly dynamically reversible post-transcriptional modifications, of which m6A is the most prevalent in eukaryotic mRNAs. A growing number of studies indicate that RNA modification can finely tune gene expression and modulate RNA metabolic homeostasis, which in turn affects the self-renewal, proliferation, apoptosis, migration, and invasion of tumor cells. Endometrial carcinoma (EC) is the most common gynecologic tumor in developed countries. Although it can be diagnosed early in the onset and have a preferable prognosis, some cases might develop and become metastatic or recurrent, with a worse prognosis. Fortunately, immunotherapy and targeted therapy are promising methods of treating endometrial cancer patients. Gene modifications may also contribute to these treatments, as is especially the case with recent developments of new targeted therapeutic genes and diagnostic biomarkers for EC, even though current findings on the relationship between RNA modification and EC are still very limited, especially m6A. For example, what is the elaborate mechanism by which RNA modification affects EC progression? Taking m6A modification as an example, what is the conversion mode of methylation and demethylation for RNAs, and how to achieve selective recognition of specific RNA? Understanding how they cope with various stimuli as part of in vivo and in vitro biological development, disease or tumor occurrence and development, and other processes is valuable and RNA modifications provide a distinctive insight into genetic information. The roles of these processes in coping with various stimuli, biological development, disease, or tumor development in vivo and in vitro are self-evident and may become a new direction for cancer in the future. In this review, we summarize the category, characteristics, and therapeutic precis of RNA modification, m6A in particular, with the purpose of seeking the systematic regulation axis related to RNA modification to provide a better solution for the treatment of EC.
1 Introduction
It is commonly believed that RNA is the vector that transmits genetic information from DNA to the machinery that synthesizes proteins. Aside from translation, RNA has a hand in many other essential biological processes such as genetic controlling, protein synthesis, degradation, and even catalysis of chemical reactions, which largely rely on intricate RNA modification and structure (Liu and Pan, 2015; Roundtree et al., 2017). In non-coding RNA species such as ribosomal RNA (rRNA) and transfer RNA (tRNA), chemical modifications have long been observed. In addition to mRNA and long non-coding RNAs (lncRNAs), an increasing number of RNA modifications have also been characterized. The rapid development of epigenomics research is manifested in the identification of RNA modifications and the in-depth exploration of the mechanism. It has been reported that more than 170 chemical modifications have been discovered in RNA, thus establishing a new layer of gene expression regulation referred to as the “epitranscriptome” (Barbieri and Kouzarides, 2020). Among the three major types of malignant tumors in the female reproductive system, endometrial cancer (Ec) is one of the most prevalent types in developed countries and the incidence rate is growing quickly (Siegel et al., 2023). Active surgery (Hysterectomy and bilateral salpingo-oophorectomy) are the mainstay treatments for EC, followed by adjuvant treatment based on histology and stage. Early diagnosis and treatment of EC are associated with better prognosis, but recurrent or primary metastatic ones are difficult to treat and have shorter median overall survival (OS) (Inoue et al., 2021). As of now, there are no endorsed focused treatments available for EC. It is crucial to gain more insight into epigenetic mechanisms to create alternative therapies for EC. Fortunately, a variety of methods and advanced detection tools are available to identify and increase the recognition of ribonucleoside modifications, both at a whole genome scale and specific nucleotide resolution, consequently, aside from their role in biology, RNA modifications can be used to improve RNA-based therapies, which are dramatically favorable for diagnosis and treatment for EC. We believe that in the near future, RNA therapy due to RNA modification will bring better opportunities for endometrial cancer patients.
2 Overview of RNA modification
RNA modifications were first detected in highly abundant “infrastructural” RNA (such as rRNAs, tRNAs, snoRNAs, and snRNAs), and were viewed as irreversible decorations that contributed to the structural stability of RNA previously. However, many studies have confirmed that RNA modifications are reversible and that in recent years this has been executed with dynamic modulation of RNA transcription, splicing, localization, decay, and RNA-RBP pattern (Jonkhout et al., 2017; Jiang et al., 2021; Schaefer, 2021). We collected sets of identified RNA modifications that were categorized by their reference nucleotide (G, Adenosine, U, and Cytosine) (Figure 1A). Taking a panoramic view of the situation, RNA modifications, which were highlighted and circled in black, have been established as being associated with diseases (Jonkhout et al., 2017). As for the modifications in RNA of specific categories, this study introduces the principal modifications that occur in mRNA, rRNA, tRNA, LncRNA, circRNA, and Sno/microRNA, respectively (Figure 1B). This illustration indicates that the majority of RNA modifications were mapped to tRNAs, such as Gm, Cm, Um, and yW, with fewer forms identified in mRNA or other RNAs. The dominant type of RNA modification was N1-methyladenosine (m1A), which preferentially enriched in GC content per se (Li et al., 2016; Dominissini et al., 2016), 5-methylcytidine (m5C) and was similar to 5 mC in DNA (Squires et al., 2012). The abundance of N6-methyladenosine (m6A) was identified to be 0.1%–0.4% of total adenosine residues and was widespread in mRNA (Dominissini et al., 2012; Meyer et al., 2012), inosine (I) which represented the site-specific conversion of adenosine to inosine (A-to-I) mostly in precursor mRNAs (Levanon et al., 2004). Pseudouridine (Ψ) was the most abundantly modification in RNA (Schwartz et al., 2014; Li et al., 2015). N7-methylguanosine (m7G) showed a robust bias installed at the 5′ cap of mRNA during transcription initiation (Zhang et al., 2019). N4-acetylcytidine was focused solely on cytidine mainly within coding sequences (CDS) (Arango et al., 2018) and N6,2′-O-dimethyladenosine (m6A.m.) was mapped to 2′-hydroxyl position of the ribose sugar near m7G (Mauer et al., 2017).
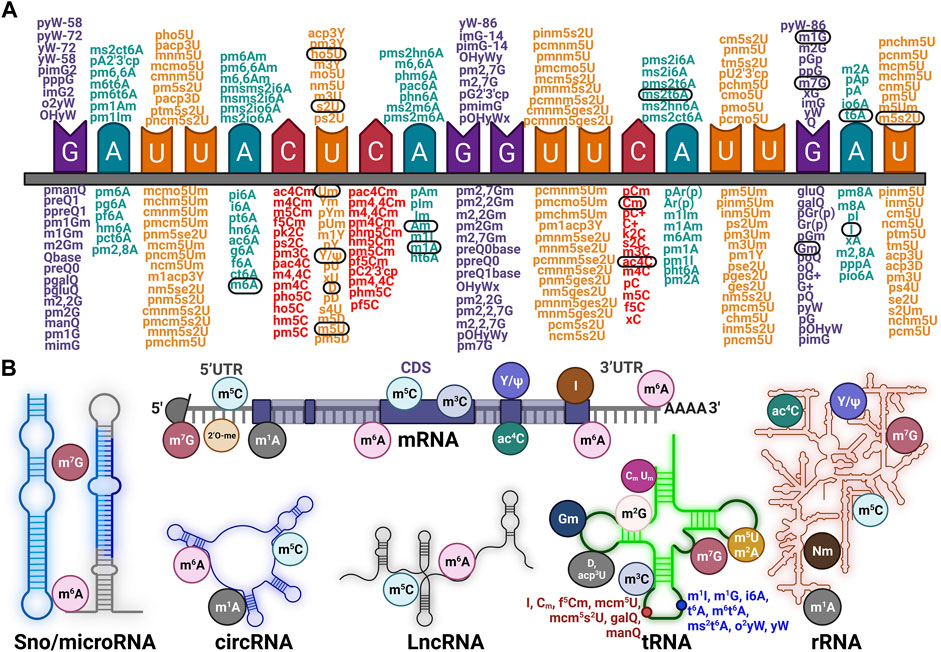
FIGURE 1. Exhaustive profile for various categories of RNA modifications (A), The authenticated types of RNA modifications according to different single nucleotide base (G- Guanine, C- Cytosine, A- Adenine, U- Uracil) and the conserved ones across species were circled in black. (B), Some kinds of familiar and specific RNA modifications occurred in distinct RNAs like Sno/microRNA, circRNA, LncRNA, tRNA, and rRNA, respectively.
The disruption of gene expression patterns controlled by epigenetics can lead to autoimmune diseases, infection, cancers (Wang et al., 2022), inflammatory, autoimmune diseases and a variety of other diseases (Cui et al., 2022)and m6A methylation plays an important role in both hypertrophic and ischemic heart disease (Kumari et al., 2022), kidney diseases (Ni et al., 2023), such as renal cell carcinoma, acute kidney injury and chronic kidney disease, osteosarcoma (Wu et al., 2022) and other different types of cancers. In a variety of cancers, m6A was found to play different roles, the same regulator has different functions in different cancers, and even different cell lines of the same cancer, and the phenotypes of regulator interventions with similar functions in the same cancer are also different.
Collectively, there have been extensive studies about the modifications of RNA during recent years, with modified nucleotides detected in abundant cellular RNAs and the specifically modified bases might exert various effects in RNA metabolism consisting of structure formation, dynamic stability, splicing, transportation, cellular localization, and translatability (Roundtree et al., 2017). In the next few years, research will likely provide a more systematic and precise atlas for RNA modification and the pathways of diseases.
3 Capital RNA modifications associated with cancer hallmarks
The dynamic and elaborate manipulation of reversible RNA modification such as m6A, was previously predominantly dependent on methyltransferases and demethylases. The regulators associated with RNA modification were divided into “Writer (catalyze to effectively induce RNA modification)”, “Erasers (remove the modification in RNA)”, and “Readers/binders (accurately recognize and bind to RNA modification site)”, which could widely influence the steady state and fate of RNAs (Zaccara et al., 2019; Cui et al., 2022). What RNA modification represents in terms of functional and evolutionary significance is still not exhaustive, but it may notably indicate the crossroads between epigenetic regulation and disease (mostly cancer) (Liu and Pan, 2015; Esteve-Puig et al., 2020; Jiang et al., 2021). After this section, we will expound on the influence of the largely dominant RNA modifications (m6A, m5C, and m7G) as examples of tumor progression regarding cancer hallmarks as guidelines.
Cancer conceptualization aims to distill complex phenotypic and genotypic diversity into a minimally structured set of principles, with the hallmarks continually updated. The latest hallmarks of cancer include avoiding immune destruction, tumor-promoting inflammation, deregulating cellular energetics, sustained proliferative signaling, genome instability, and mutation, enabling replicative immortality, inducing angiogenesis, activating invasion and metastasis, resisting cell death (Hanahan, 2022; Pavlova et al., 2022). Even though a great deal of RNA modifications are catalyzed by enzymes and the fact that the order in which the reactions take place is unknown, the types and roles of RNA-modifying proteins (RMPs) involved in m6A, m5C, and m7G (Figure 2) are summarized below.
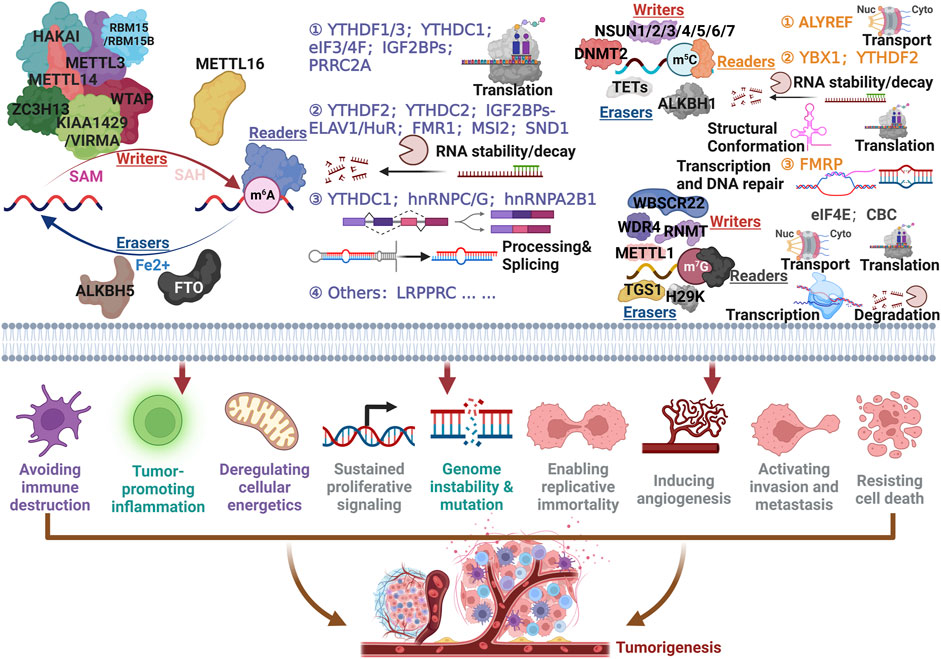
FIGURE 2. Capital RNA modifications associated with cancer hallmarks. To exemplify the prominent effect of RNA modifications, m6A, m5C, and m7G were designated on account of elucidating accurately the detailed mechanism for modulating tumorigenesis.
First of all, m6A has been well-studied for its role in cancer, and the deposition in RNA are mainly mediated by a methyltransferase (i.e., Writers) complex, including METTL3, METTL14, WTAP, KIAA1459/VIRMA, ZC3H13, HAKAI and RBM15/15B, or METTL16 under existence of SAM. The overwhelming majority of m6A sites occurred at a consensus motif “DRACH” (D = A, G or U; R = A or G; H = A, C or U) (Garcias Morales and Reyes, 2021). Demethylases called “Erasers” contained FTO and ALKBH5, which could effectively remove m6A from RNAs (Roundtree et al., 2017; Esteve-Puig et al., 2020). As regards “Readers”, the wide variety of categories endowed quite different fates for RNA. YTHDF1/3 (Lan et al., 2021; Wiener and Schwartz, 2021), YTHDC1 (Garcias Morales and Reyes, 2021), eIF3/4F (Volpon et al., 2016; Wiener and Schwartz, 2021), IGF2BPs (Huang et al., 2018), and PRRC2A (Wu et al., 2019; Tan et al., 2023) tend to influence the translation efficiency of RNAs. YTHDF2 (Roundtree et al., 2017), YTHDC2 (Roundtree et al., 2017), IGF2BPs-ELAV1/HuR (Huang et al., 2018), FMR1 (Yang et al., 2022; Zhang et al., 2022), MSI2 (Zhu et al., 2022), and SND1 (Baquero-Perez et al., 2019) exerted indispensable roles by accurately dominating the stability and decay of RNAs. Additionally, YTHDC1 (Esteve-Puig et al., 2020), hnRNPC/G (changing the conformation to offer the occupancy motif) (Liu et al., 2017; Zhou et al., 2019) and hnRNPA2B1 (Alarcon et al., 2015) (mainly mediated microRNA mature) significantly contributed to RNA (including mRNA, LncRNA, circRNA and microRNA) processing and splicing. The up-to-date annotated reader, LRPPRC was involved in monitoring translation and the mechanism remains further exploration (Arguello et al., 2017; Wang H. et al., 2023).
When it comes to m5C modification, NSUN1-7 and DNMT2 are ‘Writers’ that have been used to catalyze the methylation that occurred at cytosine, conversely, TETs and ALKBH1 were responsible for the removal of m5C (Nombela et al., 2021; Cui et al., 2022). ALYREF could interact with methylated mRNAs to transport them from nuclei to cytoplasm (Yang et al., 2017). YBX1 and YTHDF2 monitored m5C modified RNAs decay or stability, structural conformation, and translation (Chen et al., 2019; Yang et al., 2019; Wang et al., 2023; Liu et al., 2023). FMRP could assist TET1 to facilitate transcription and DNA repair (Yang et al., 2022).
WBSCR22, WDR4, RNMT, and METTL1 have been shown to be “Writers”, while TGS1 and H29K are “Erasers” for m7G modification (Jonkhout et al., 2017; Liu et al., 2020). Studies have also outlined that eIF4E and CBC affect RNA transcription, transport, translation, and degradation (Izumi et al., 2014; Volpon et al., 2016). Briefly, there is still much to learn about the mechanisms and functions of RNA modification biology, which are just the tip of the iceberg in the study of epigenomes and epitranscriptomes. In the future, oncology therapies may benefit from epitranscriptomic anticancer drugs. It is precisely because RNA modification, especially m6A, plays an indispensable role in the proliferation, invasion, and metastasis of tumor cells and drug resistance, and has great potential for clinical application.
4 Therapeutic precis
Treatment for tumors generally comprises surgery, hormonal therapy, Chemo/Target therapy, immunotherapy, radiotherapy, viruses/bacteria (Yanagi et al., 2022), and more recently personalized therapy (Beck et al., 2021; Inoue et al., 2021). The first-line treatment for low-grade hormone receptor-positive metastatic endometrial cancer includes platinum-based chemotherapy and hormonal therapy, and there is no standard follow-up treatment. As a result, new treatment strategies have emerged. Clinical studies of single or combined treatment for endometrial cancer with PARP inhibitors, as well as PD-1 and PD-L1 inhibitors have been reported, which may bring new perspectives to the treatment of metastatic or recurrent endometrial cancer (Karpel et al., 2023; Rowlands et al., 2023).
Apart from surgery, hormonal therapy, and radiotherapy, we introduced Chemo/Target therapy and immunotherapy associated with m6A. Above all, we collected the small molecule inhibitors that targeted m6A related proteins, which included (Figure 3):
1 m6A modification, which could be inhibited by neplanocin A (NPC), Cycloleucine, and 3-Deazaadenosine (Cayir, 2022);
2 METTL3, which was effectively repressed by STM2457 (Yankova et al., 2021) and UZH1a (Yanagi et al., 2022);
3 S-Adenosylhomocysteine (SAH), Antiviral agent 23/24 and STM2120 (Yankova et al., 2021) was identified to inhibit the activity of METTL3/14 complex;
4 FB23 (Huang et al., 2019), Rhein, Meclofenamic acid (MA) (Huang et al., 2015), and Entacapone sodium salt could effectively repress FTO;
5 ALKBH5, whose potential inhibitor was IOX1 (Li et al., 2016);
6 DC-Y3 and DC-Y13-27 were for YTHDF2 (Wang et al., 2023);
7 SND1 was corresponding to Thymidine 3′,5′-disphosphate (Jariwala et al., 2017);
8 IGF2BP2, which was restrained by CWI1-2 (Weng et al., 2022);
9 MSI2, whose inhibitor was Romidepsin (FK228) (Cayir, 2022; Zhu et al., 2022).
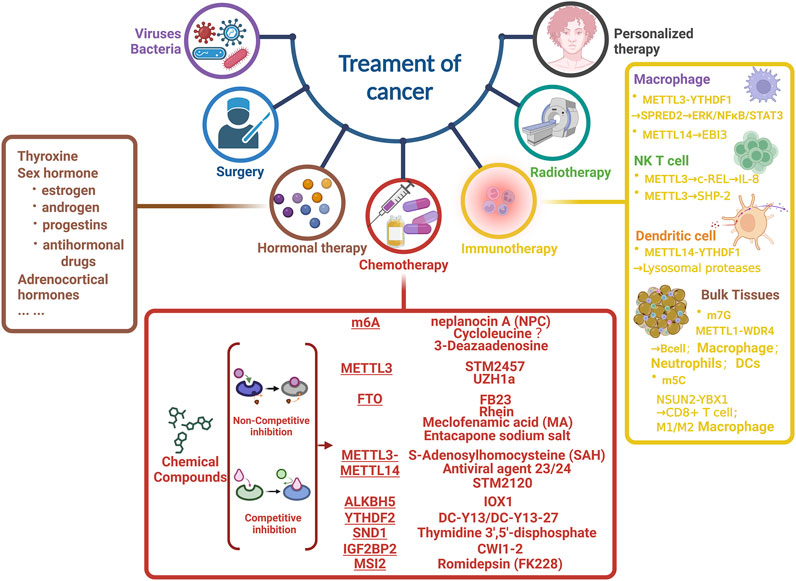
FIGURE 3. Overview of strategies for m6A related cancer therapy. Focusing on chemotherapy and immunotherapy, we enumerated the main compounds/inhibitors and targets of m6A related protein.
As for immunotherapy, ablation of METTL3 could effectively impair the YTHDF1 mediated translation of SPRED2 and increase M1/M2 like macrophage, as well as regulatory T cell infiltration into tumours (Yin et al., 2021). Furthermore, METTL3 played a pivotal role in suppressing papillary thyroid carcinoma (PTC) carcinogenesis by synergizing the c-Rel and RelA inactivated nuclear factor B (NF-B) pathways in collaboration with YTHDF2 (He et al., 2021).
Similar to m6A, potential inhibitors of m5C modification indicate that they have an inhibitory effect on tumor cell proliferation with high levels of m5C modification (Fu et al., 2014; Kawarada et al., 2017). Drugs that inhibit m5C modification mainly inhibit m5C modification by acting on m5C regulators, that is, promoting m5C demethylases (Erasers) or inhibiting m5C methyltransferases (Writers) NOP2, NSUN2⁃746, DNMT1, DNMT2 (TRD⁃MT1), DNMT3A and DNMT3B and m5C methylation-binding proteins (Readers) ALYREF, YBX1 and RAD52. Proof-of-concept studies have shown that dysregulated m5C regulators targeted by small molecule inhibitors have the potential for cancer treatment (Garcia-Vilchez et al., 2019; Chen et al., 2020). To date, no m5C inhibitors have been developed (Song et al., 2022). This provides a basis for further research and expansion of the application of these drugs in anti-tumor therapy.
When it comes to the therapeutic precis based on the m6A modification, the effect of m6A on cancer is reflected in the regulation of cancer-related gene expression. There is growing evidence that m6A plays a dual role in cancer (He et al., 2019). On the one hand, m6A regulates the expression of oncogenes or tumor suppressor genes, thereby affecting tumor progression. On the other hand, m6A levels and the expression and activity of m6A enzymes can be regulated, thus affecting the role of m6A in cancer. How m6A influences cancer progression by regulating target genes depends on three factors: 1) whether the target gene acts as a tumor promoter or as a tumor suppressor; 2) abnormal levels of m6A in cancer (depending on changes in expression or activity of “writer” or “erase”); 3) Regulation of target mRNA after methylation (determined by “reader”).
Given the important role of m6A regulatory proteins in a variety of diseases, small molecule inhibitors or agonists that target dysregulated m6A regulatory proteins may be promising candidates for disease treatment, particularly different types of cancer therapies. However, therapeutics targeting m6A modification in cancer are still in their infancy. Future research directions include, but are not limited to, clinical validation of small molecule drugs in cancer patients with abnormal RNA m6A modified protein expression. Therefore, the development of safer and more effective small-molecule inhibitors or agonists of m6A regulatory proteins will help promote the development of RNA-based precision medicine in the future. These scientific findings will contribute to our understanding of the relationship between RNA modifications (m6A, m5C, and m7G) and tumor microenvironment plasticity.
5 Systematic profiles of m6A modulation in endometrial cancer
We systematically summarized the landscape of m6A modification associated regulation pathway in EC (Figure 4). In terms of mechanism, SLERT, as a capable scaffold, could effectively recruit METTL3 and enhance the interaction between the “writer complex” and BDNF mRNA. Accordingly, m6A modified BDNF mRNA was recognized by IGF2BP1 and stabilized to bind TRKB facilitating EC cell metastasis (Tian et al., 2023). This indicates that it could be used as a novel marker to suggest metastasis of endometrial cancer.
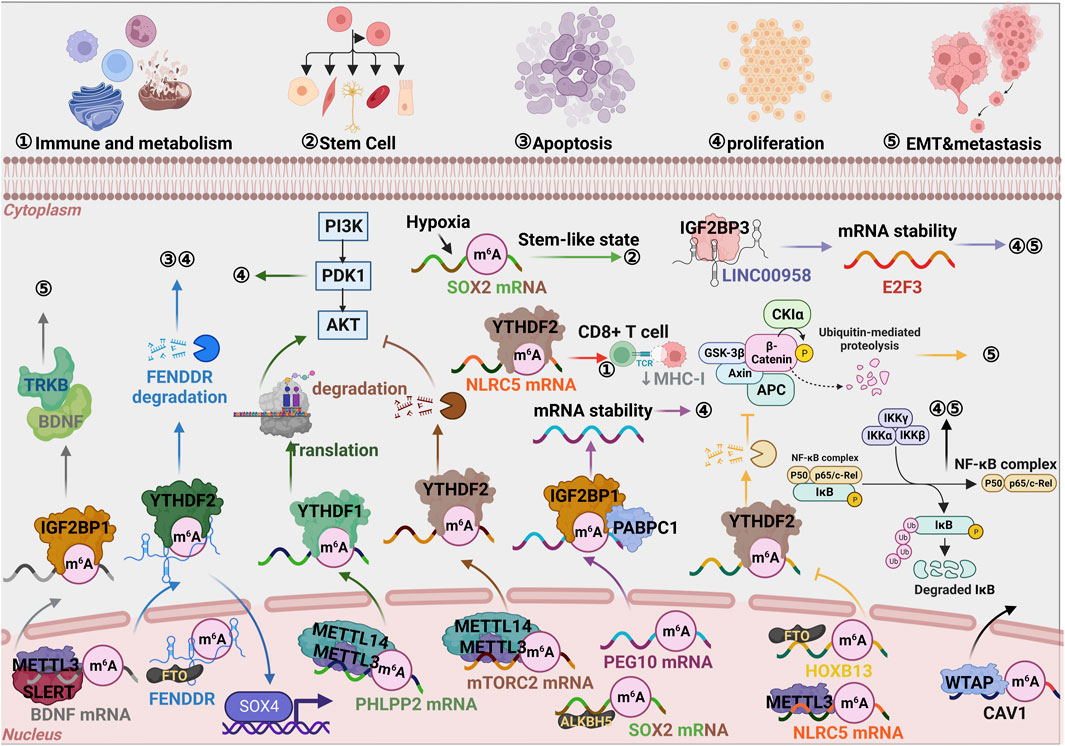
FIGURE 4. Graphic illustration of m6A monitoring pathway in EC. The diversified modulation axis mediated by core proteins in m6A modification, such as Writers, Erases, and Readers, which ultimately lead to cell proliferation, apoptosis, migration/metastasis, or immune/metabolism during tumor progression.
In practice, studies showed that upregulated METTL3 was an independent factor in promoting the progression of endometrioid epithelial ovarian cancer (EEOC) through modulating FZD10 and EIF3C, etc (Ma et al., 2020). It was found that overexpression of METTL3 inhibited the proliferation and migration of EC cells and promoted the proliferation of CD8+ T cells in the coculture system using EC and CD8+ T cells, mechanistically, downregulated METTL3 protects NLRC5 from degradation through m6A modification and YTHDF2 dependent inhibition (Zhan et al., 2023). The conclusion indicated that m6A had a cancer-suppressing effect in EC and would be a novel target for RNA therapy.
LncRNA FENDRR was downregulated and demethylated by FTO in EC cancerous tissues and YTHDF2 was involved in the degradation of FENDRR by m6A recognition, and finally, the expression of SOX4 was elevated to promote cell proliferation (Shen et al., 2021). In addition to m6A modification, LINC00958 also assisted IGF2BP3, significantly enhancing the RNA stability of E2F3, ultimately accelerating EC progression (Wang et al., 2022). Some exciting research in this area described that approximately 70% of endometrial tumors showed reduced m6A methylation, possibly caused by either METTL14 mutation or reduced METTL3 expression. One mechanism was focused on the decreased PHLPP2’s negative regulatory function and the increased mTORC2’s positive regulatory function, as YTHDF1 and YTHDF2 recognized respectively followed by a reduction of m6A methylation (Liu et al., 2018). The model of RNA degradation based on m6A modification, dependent on YTHDF2, was universally accepted. YTHDF2 could not recognize m6A modification as a result of demethylation of HOXB13 mRNA mediated by FTO. With the attenuation of the reduced HOXB13 mRNA, the Wnt signaling pathway was activated, resulting in EC transfer (Zhang et al., 2021a). The upregulated YTHDF2 could restrain EC progression by accelerating IRS1 degradation and inhibiting IRS1/AKT signaling axis (Hong et al., 2021). This would imply that YTHDF2 plays a role in demethylation to promote tumor malignancy, meaning it could be used as a new target for EC therapy like m6A.
Excitingly, ß-estradiol (E2)/estrogen could remarkably induce FTO expression and transfer to nuclei, activate PI3K/AKT and MAPK signal pathways, and participate in enhancing proliferation and invasion of EC through modulating CyclinD1 and MMP2/9 level (Zhang et al., 2012). The high level of ALKBH5, which was another demethylase, under hypoxic conditions facilitated SOX2 mRNA expression by reducing m6A in endometrial cancer stem cells (ECSCs) (Chen et al., 2020). In addition, ALKBH5 enhanced the stability of its mRNA by emethylating IGF1R, thus promoting the proliferation and invasion of EC (Pu et al., 2020). Furthermore, there were many studies on the mechanism by which RNA stability depended on m6A modification. When IGF2BP1 was bound to the m6A site in its 3′UTR and recruited PABPC1 to perform its primary function, the patrol-expressed gene 10 (PEG10) mRNA was stable (Zhang et al., 2021b). Another study also found that oncogenic WTAP enhanced EC proliferation and invasiveness through the caveolin-1(CAV1)/nuclear factor-κB (NF-κB) axis, indicating that CAV1 was identified as a new target for WTAP (Li et al., 2021). Furthermore, many studies have been devoted to the mechanism of RNA stability, which was dependent on m6A modification. Based on the above, the biomarkers that inhibit m6a demethylation (inhibit the “eraser”) or promote m6A methylation (promote the “reader”) play a protective role in EC and could be used as targeted therapeutic targets.
6 Network resources
Using bioinformatics analysis, the researchers investigated m6A modifications and their associated genes as potential biomarkers for endometrial cancer. A vital function is played by m6A methylation regulators in the development of endometrial cancer. Age, grade, and risk score were independent risk factors, and a high expression of FTO was associated with poor overall survival, according to both univariate and multivariate Cox regression analyses (Zhang and Yang, 2021). Using the TIMER algorithm to analyze the clinical, sequencing, and copy number variation (CNV) data in The Cancer Genome Atlas (TCGA), which were correlated with m6A regulators, a positive correlation was found between immune cell infiltration and METTL14, ZC3H13, and YTHDC1 level (Jian Ma and Ma, 2021), meanwhile, YTHDC2 performed the same important function in immune infiltration as a prospective biomarker for diagnosis and prognosis (Zhang et al., 2021). Moreover, a potentially useful biomarker for EC prognosis is m7G-related mRNAs. These mRNAs regulated cell cycle progression accompanied by immune cell infiltration, which might lead to UCEC progression (Zhao et al., 2022). Likewise, the findings indicated that the constituents of hazard models relying on the lncRNAs associated with m5C, m7G, or m6A could function as significant intermediaries of the immune milieu and promising prognosis biomarkers with therapeutic response in UCEC (Gu et al., 2022; Sun et al., 2022; Chen et al., 2023; Zhou et al., 2023). Wang et al. outline new insights into CNVs/SNVs in m6A regulatory genes which were associated with a negative impact on patient survival of EC and ultimately found that three genes, IGF2BP3, KIAA1429, and IGF2BP1, were effective predictors of EC outcomes (Wang et al., 2020). Similarly, Chen et al. selected the risk signature of 8-m6A regulators as the potential predictive prognostic value for EC (Miao et al., 2021).
It is possible to study the epitranscriptome by functionally using RNA modification detection methods and tools. In the wake of the advancement of high-throughput sequencing technologies applied for transcriptome-wide mapping, a number of RNA modification databases have emerged. This is an exciting new research area that encourages additional exploration into the mechanisms and roles of these altered ribonucleotides. Here, we introduced some databases constructed for RNA modification in Table 1, including annotation, site prediction, and functional analysis database. All three primary phylogenetic domains (archaea, bacteria, and eukaryotes) are represented in the RNA Modification Database (RNAMDB) (Cantara et al., 2011). MODOMICS is the most comprehensive source of RNA modification pathways, containing information about the chemical structure of modified nucleosides, their localization in RNA sequences, the pathways of their biosynthesis, and enzymes involved in their synthesis integrated into the model (Boccaletto et al., 2022). Using them, we propose a few suggestions regarding RNA modification databases, with the hope of extending the depth of research in this area.
Despite advancements in treatment, EC’s incidence and mortality rates continue to rise. A new biomarker and therapeutic target for EC were highlighted in the current study. Future research needs to identify RNA modification regulators and develop a prognostic gene signatures in EC, which are critically important to elucidating cancer pathogenesis and progression.
7 Discussion and prospect
At present, more than 150 RNA methylation modifications have been identified as eukaryotic post-transcriptional regulatory markers, and m6A has been well-studied for its role in cancers and the deposition in RNA has been mainly mediated by methyltransferase. With the deepening of research, the role of RNA modifications, especially m6A, in the process of cancer development has attracted more and more attention, but the mechanism of RNA methylation modifications in tumor development needs to be further elucidated. In our review, the types and the roles of RNA methylation modifications in EC are summarized, and the challenges and future directions of RNA methylation modifications in tumor research examined. To facilitate the molecular consideration of further diagnosis and treatment of endometrial cancer, the Cancer Genome Atlas (TCGA) divides endometrial cancer into four different types, including the: 1) DNA polymerase ε (POLE) super mutant type, which had a very high mutation load and a good prognosis; 2) the MicroSatellite Instability (MSI) type (Hrzenjak et al., 2006), with a high mutation load and moderate prognosis; 3) the copy-number low (CNL) type, with low mutation load and moderate prognosis; and 4) the copy-number high (CNH) type, which has a relatively low mutation amount and a poor prognosis. This new classification of endometrial cancer not only provides sufficient prognostic information but also produces subsets of biologically defined ones that may exhibit different responses to specific drugs. For example, POLE hypermutation and deficient mismatch repair (dMMR) endometrial cancers may be more sensitive to PD-1/PD-L1 inhibitor-based immunotherapy because they are associated with high mutational burden and significant immune infiltration. Mismatch repair (MMR) refers to the function of genetic mismatch repair. The MMR gene can express the corresponding MMR protein after transcription and translation, and any loss of expression of MMR protein can cause a base mismatch in the DNA replication process to lose repair function and cause accumulation, resulting in MSI. MSI is divided into microsatellite instability-high (MSI-H), microsatellite instability-low (MSI-L), and stable (MSS). MMR is divided into dMMR and Mismatch Repair Full Function (pMMR). dMMR presents as MSI-H and pMMR as MSI-L or MS-S. Second, CNH endometrial carcinoma is characterized by alterations in the p53 pathway, which was associated with an increased incidence of homologous recombination deficiency (HRD), and in general, HRD tumors might respond to PARP inhibitors (Xiong et al., 2006; Zhou et al., 2007; Tao and Freudenheim, 2010; Zhang et al., 2012). Since RNA can be detected in serum or plasma in vivo, it can be used for early diagnosis, assessment of efficient treatment, and prognosis prediction of EC. It is also expected to provide new ideas and new targets for the pathogenesis and therapy of EC (Pavlova et al., 2022; Xu et al., 2022). Over the past few years, it has been possible to identify the chemical basis and multiple functions of m6A RNA methylation due to the availability of highly specific antibodies and the availability of high-throughput sequencing techniques. With the rapid development of m6A crosslinking-immunoprecipitation and RNA-seq technology, m6A has been shown to be involved in the development of a variety of malignancies. This will enable the targeting of m6A-related enzymes or m6A-dependent pathways, providing an important scientific basis for the targeted treatment of human cancer with m6A.
Although the role of m6A in cancer has gradually been revealed, many challenges remain. Firstly, the mechanism of m6A regulators in tumors is largely unknown, such as the role and mechanism of “Readers” in cancer in m6A methylation modification is still a big gap; Secondly, although many studies have shown that m6A-related regulators and pathways could be used as new targets in cancer treatment, there is a lack of certain clinical practice, and m6A can affect the expression of genes in many aspects. That is to say, its side effects cannot be ignored. The coming mission will aim to deeply explore the molecular mechanism of “Writers”, “Erasers” and “Readers” in m6A modification involved in the regulation of tumorigenesis, as well as evaluate the correlation between m6A and cancer in combination with clinical data. Therefore, strengthening our understanding of tumor malignant transformation, ultimately, will be conducive to seeking and designing novel prospective targets for cancer therapy soon.
Author contributions
ZJ organized the database, completed the drawing of the chart, wrote the first draft of the manuscript, Funding acquisition. JS maked significant changes of the version, YyH, YZ, XW, and YpH wrote sections of the manuscript. All authors contributed to the article and approved the submitted version.
Funding
This study was supported by the National Natural Science Foundation of China (No. 82171613).
Conflict of interest
The authors declare that the research was conducted in the absence of any commercial or financial relationships that could be construed as a potential conflict of interest.
Publisher’s note
All claims expressed in this article are solely those of the authors and do not necessarily represent those of their affiliated organizations, or those of the publisher, the editors and the reviewers. Any product that may be evaluated in this article, or claim that may be made by its manufacturer, is not guaranteed or endorsed by the publisher.
References
Alarcon, C. R., Goodarzi, H., Lee, H., Liu, X., Tavazoie, S., and Tavazoie, S. F. (2015). HNRNPA2B1 is a mediator of m(6)a-dependent nuclear RNA processing events. Cell 162, 1299–1308. doi:10.1016/j.cell.2015.08.011
Arango, D., Sturgill, D., Alhusaini, N., Dillman, A. A., Sweet, T. J., Hanson, G., et al. (2018). Acetylation of cytidine in mRNA promotes translation efficiency. Cell 175, 1872–1886. doi:10.1016/j.cell.2018.10.030
Arguello, A. E., DeLiberto, A. N., and Kleiner, R. E. (2017). RNA chemical proteomics reveals the N(6)-methyladenosine (m(6)A)-Regulated protein-RNA interactome. J. Am. Chem. Soc. 139, 17249–17252. doi:10.1021/jacs.7b09213
Bai, W., Yang, W., Wang, W., Wang, Y., Liu, C., Jiang, Q., et al. (2017). Ged: A manually curated comprehensive resource for epigenetic modification of gametogenesis. Brief. Bioinform 18, 98–104. doi:10.1093/bib/bbw007
Bao, X., Zhang, Y., Li, H., Teng, Y., Ma, L., Chen, Z., et al. (2023). RM2Target: A comprehensive database for targets of writers, erasers and readers of RNA modifications. Nucleic Acids Res. 51, D269–D279. doi:10.1093/nar/gkac945
Baquero-Perez, B., Antanaviciute, A., Yonchev, I. D., Carr, I. M., Wilson, S. A., and Whitehouse, A. (2019). The Tudor SND1 protein is an m(6)A RNA reader essential for replication of Kaposi's sarcoma-associated herpesvirus. Elife 8, e47261. doi:10.7554/eLife.47261
Barbieri, I., and Kouzarides, T. (2020). Role of RNA modifications in cancer. Nat. Rev. Cancer 20, 303–322. doi:10.1038/s41568-020-0253-2
Beck, J. D., Reidenbach, D., Salomon, N., Sahin, U., Türeci, Ö., Vormehr, M., et al. (2021). mRNA therapeutics in cancer immunotherapy. Mol. Cancer 20, 69. doi:10.1186/s12943-021-01348-0
Becquey, L., Angel, E., and Tahi, F. (2021). RNANet: an automatically built dual-source dataset integrating homologous sequences and RNA structures. Bioinformatics 37, 1218–1224. doi:10.1093/bioinformatics/btaa944
Boccaletto, P., Stefaniak, F., Ray, A., Cappannini, A., Mukherjee, S., Purta, E., et al. (2022). Modomics: A database of RNA modification pathways. 2021 update. Nucleic Acids Res. 50, D231–D235. doi:10.1093/nar/gkab1083
Cantara, W. A., Crain, P. F., Rozenski, J., McCloskey, J. A., Harris, K. A., Zhang, X., et al. (2011). The RNA modification database, RNAMDB: 2011 update. Nucleic Acids Res. 39, D195–D201. doi:10.1093/nar/gkq1028
Cayir, A. (2022). RNA modifications as emerging therapeutic targets. Wiley Interdiscip. Rev. RNA 13, e1702. doi:10.1002/wrna.1702
Chandrashekar, D. S., Karthikeyan, S. K., Korla, P. K., Patel, H., Shovon, A. R., Athar, M., et al. (2022). Ualcan: an update to the integrated cancer data analysis platform. Neoplasia 25, 18–27. doi:10.1016/j.neo.2022.01.001
Chen, G., Liu, B., Yin, S., Li, S., Guo, Y., Wang, M., et al. (2020b). Hypoxia induces an endometrial cancer stem-like cell phenotype via HIF-dependent demethylation of SOX2 mRNA. Oncogenesis 9, 81. doi:10.1038/s41389-020-00265-z
Chen, H., Yang, H., Zhu, X., Yadav, T., Ouyang, J., Truesdell, S. S., et al. (2020a). m(5)C modification of mRNA serves a DNA damage code to promote homologous recombination. Nat. Commun. 11, 2834. doi:10.1038/s41467-020-16722-7
Chen, J., Luo, X., Wang, G., Zhang, J., and Zhang, Y. (2023). Analysis of m(6)A methylation patterns and tumor microenvironment in endometrial cancer. Gene 852, 147052. doi:10.1016/j.gene.2022.147052
Chen, K., Song, B., Tang, Y., Wei, Z., Xu, Q., Su, J., et al. (2021). RMDisease: A database of genetic variants that affect RNA modifications, with implications for epitranscriptome pathogenesis. Nucleic Acids Res. 49, D1396–D1404. doi:10.1093/nar/gkaa790
Chen, K., Wei, Z., Zhang, Q., Wu, X., Rong, R., Lu, Z., et al. (2019b). Whistle: A high-accuracy map of the human N6-methyladenosine (m6A) epitranscriptome predicted using a machine learning approach. Nucleic Acids Res. 47, e41. doi:10.1093/nar/gkz074
Chen, X., Sun, B. F., Yang, Y., Han, Y. N., Yuan, X., Li, A., et al. (2019a). 5-methylcytosine promotes pathogenesis of bladder cancer through stabilizing mRNAs. Nat. Cell Biol. 21, 978–990. doi:10.1038/s41556-019-0361-y
Cui, L., Ma, R., Cai, J., Guo, C., Chen, Z., Yao, L., et al. (2022). RNA modifications: importance in immune cell biology and related diseases. Signal Transduct. Target Ther. 7, 334. doi:10.1038/s41392-022-01175-9
Dai, E., Yu, X., Zhang, Y., Meng, F., Wang, S., Liu, X., et al. (2014). EpimiR: A database of curated mutual regulation between miRNAs and epigenetic modifications. Database (Oxford) 2014, bau023. doi:10.1093/database/bau023
Dassi, E., Re, A., Leo, S., Tebaldi, T., Pasini, L., Peroni, D., et al. (2014). Aura 2: empowering discovery of post-transcriptional networks. Transl. (Austin) 2, e27738. doi:10.4161/trla.27738
Deng, S., Zhang, H., Zhu, K., Li, X., Ye, Y., Li, R., et al. (2021). M6A2Target: A comprehensive database for targets of m6A writers, erasers and readers. Brief. Bioinform 22, bbaa055. doi:10.1093/bib/bbaa055
Dominissini, D., Moshitch-Moshkovitz, S., Schwartz, S., Salmon-Divon, M., Ungar, L., Osenberg, S., et al. (2012). Topology of the human and mouse m6A RNA methylomes revealed by m6A-seq. Nature 485, 201–206. doi:10.1038/nature11112
Dominissini, D., Nachtergaele, S., Moshitch-Moshkovitz, S., Peer, E., Kol, N., Ben-Haim, M. S., et al. (2016). The dynamic N(1)-methyladenosine methylome in eukaryotic messenger RNA. Nature 530, 441–446. doi:10.1038/nature16998
Esteve-Puig, R., Bueno-Costa, A., and Esteller, M. (2020). Writers, readers and erasers of RNA modifications in cancer. Cancer Lett. 474, 127–137. doi:10.1016/j.canlet.2020.01.021
Fu, L., Guerrero, C. R., Zhong, N., Amato, N. J., Liu, Y., Liu, S., et al. (2014). Tet-mediated formation of 5-hydroxymethylcytosine in RNA. J. Am. Chem. Soc. 136, 11582–11585. doi:10.1021/ja505305z
Garcia-Vilchez, R., Sevilla, A., and Blanco, S. (2019). Post-transcriptional regulation by cytosine-5 methylation of RNA. Biochim. Biophys. Acta Gene Regul. Mech. 1862, 240–252. doi:10.1016/j.bbagrm.2018.12.003
Garcias Morales, D., and Reyes, J. L. (2021). A birds'-eye view of the activity and specificity of the mRNA m(6) A methyltransferase complex. Wiley Interdiscip. Rev. RNA 12, e1618. doi:10.1002/wrna.1618
Gu, W. X., Chen, Y., and Wang, W. (2022). Immune infiltrates of m5C RNA methylation-related LncRNAs in uterine corpus endometrial carcinoma. J. Oncol. 2022, 1531474. doi:10.1155/2022/1531474
Hanahan, D. (2022). Hallmarks of cancer: new dimensions. Cancer Discov. 12, 31–46. doi:10.1158/2159-8290.CD-21-1059
He, J., Zhou, M., Yin, J., Wan, J., Chu, J., Jia, J., et al. (2021). METTL3 restrains papillary thyroid cancer progression via m(6)A/c-Rel/IL-8-mediated neutrophil infiltration. Mol. Ther. 29, 1821–1837. doi:10.1016/j.ymthe.2021.01.019
He, L., Li, H., Wu, A., Peng, Y., Shu, G., and Yin, G. (2019). Functions of N6-methyladenosine and its role in cancer. Mol. Cancer 18, 176. doi:10.1186/s12943-019-1109-9
Hong, L., Pu, X., Gan, H., Weng, L., and Zheng, Q. (2021). YTHDF2 inhibit the tumorigenicity of endometrial cancer via downregulating the expression of IRS1 methylated with m6A. A. J. Cancer 12, 3809–3818. doi:10.7150/jca.54527
Hrzenjak, A., Moinfar, F., Kremser, M. L., Strohmeier, B., Staber, P. B., Zatloukal, K., et al. (2006). Valproate inhibition of histone deacetylase 2 affects differentiation and decreases proliferation of endometrial stromal sarcoma cells. Mol. Cancer Ther. 5, 2203–2210. doi:10.1158/1535-7163.MCT-05-0480
Hu, B., Yang, Y. T., Huang, Y., Zhu, Y., and Lu, Z. J. (2017). Postar: A platform for exploring post-transcriptional regulation coordinated by RNA-binding proteins. Nucleic Acids Res. 45, D104–D114. doi:10.1093/nar/gkw888
Huang, H., Weng, H., Sun, W., Qin, X., Shi, H., Wu, H., et al. (2018). Recognition of RNA N(6)-methyladenosine by IGF2BP proteins enhances mRNA stability and translation. Nat. Cell Biol. 20, 285–295. doi:10.1038/s41556-018-0045-z
Huang, Y., Su, R., Sheng, Y., Dong, L., Dong, Z., Xu, H., et al. (2019). Small-molecule targeting of oncogenic FTO demethylase in acute myeloid leukemia. Cancer Cell 35, 677–691. doi:10.1016/j.ccell.2019.03.006
Huang, Y., Yan, J., Li, Q., Li, J., Gong, S., Zhou, H., et al. (2015). Meclofenamic acid selectively inhibits FTO demethylation of m6A over ALKBH5. Nucleic Acids Res. 43, 373–384. doi:10.1093/nar/gku1276
Inoue, F., Sone, K., Toyohara, Y., Takahashi, Y., Kukita, A., Hara, A., et al. (2021). Targeting epigenetic regulators for endometrial cancer therapy: its molecular biology and potential clinical applications. Int. J. Mol. Sci. 22, 2305. doi:10.3390/ijms22052305
Izumi, H., McCloskey, A., Shinmyozu, K., and Ohno, M. (2014). p54nrb/NonO and PSF promote U snRNA nuclear export by accelerating its export complex assembly. Nucleic Acids Res. 42, 3998–4007. doi:10.1093/nar/gkt1365
Jariwala, N., Rajasekaran, D., Mendoza, R. G., Shen, X. N., Siddiq, A., Akiel, M. A., et al. (2017). Oncogenic role of SND1 in development and progression of hepatocellular carcinoma. Cancer Res. 77, 3306–3316. doi:10.1158/0008-5472.CAN-17-0298
Jian Ma, D. Y., and Ma, X.-X. (2021). Immune infiltration-related N6-methyladenosine RNA methylation regulators influence the malignancy and prognosis of endometrial cancer. AGING 13, 16287–16315. doi:10.18632/aging.203157
Jiang, W., Qu, Y., Yang, Q., Ma, X., Meng, Q., Xu, J., et al. (2019). D-Lnc: A comprehensive database and analytical platform to dissect the modification of drugs on lncRNA expression. RNA Biol. 16, 1586–1591. doi:10.1080/15476286.2019.1649584
Jiang, X., Liu, B., Nie, Z., Duan, L., Xiong, Q., Jin, Z., et al. (2021). The role of m6A modification in the biological functions and diseases. Signal Transduct. Target Ther. 6, 74. doi:10.1038/s41392-020-00450-x
Jonkhout, N., Tran, J., Smith, M. A., Schonrock, N., Mattick, J. S., and Novoa, E. M. (2017). The RNA modification landscape in human disease. RNA 23, 1754–1769. doi:10.1261/rna.063503.117
Karpel, H. C., Slomovitz, B., Coleman, R. L., and Pothuri, B. (2023). Treatment options for molecular subtypes of endometrial cancer in 2023. Curr. Opin. Obstet. Gynecol. 35, 270–278. doi:10.1097/GCO.0000000000000855
Kawarada, L., Suzuki, T., Ohira, T., Hirata, S., Miyauchi, K., and Suzuki, T. (2017). ALKBH1 is an RNA dioxygenase responsible for cytoplasmic and mitochondrial tRNA modifications. Nucleic Acids Res. 45, 7401–7415. doi:10.1093/nar/gkx354
Kumari, R., Ranjan, P., Suleiman, Z. G., Goswami, S. K., Li, J., Prasad, R., et al. (2022). mRNA modifications in cardiovascular biology and disease: with a focus on m6A modification. Cardiovasc Res. 118, 1680–1692. doi:10.1093/cvr/cvab160
Lan, Q., Liu, P. Y., Bell, J. L., Wang, J. Y., Hüttelmaier, S., Zhang, X. D., et al. (2021). The emerging roles of RNA m(6)A methylation and demethylation as critical regulators of tumorigenesis, drug sensitivity, and resistance. Cancer Res. 81, 3431–3440. doi:10.1158/0008-5472.CAN-20-4107
Lei, H. T., Wang, Z. H., Li, B., Sun, Y., Mei, S. Q., Yang, J. H., et al. (2023). tModBase: deciphering the landscape of tRNA modifications and their dynamic changes from epitranscriptome data. Nucleic Acids Res. 51, D315–D327. doi:10.1093/nar/gkac1087
Levanon, E. Y., Eisenberg, E., Yelin, R., Nemzer, S., Hallegger, M., Shemesh, R., et al. (2004). Systematic identification of abundant A-to-I editing sites in the human transcriptome. Nat. Biotechnol. 22, 1001–1005. doi:10.1038/nbt996
Li, F., Kennedy, S., Hajian, T., Gibson, E., Seitova, A., Xu, C., et al. (2016b). A radioactivity-based assay for screening human m6A-RNA methyltransferase, METTL3-METTL14 complex, and demethylase ALKBH5. J. Biomol. Screen 21, 290–297. doi:10.1177/1087057115623264
Li, Q., Wang, C., Dong, W., Su, Y., and Ma, Z. (2021a). WTAP facilitates progression of endometrial cancer via CAV-1/NF-κB axis. Cell Biol. Int. 45, 1269–1277. doi:10.1002/cbin.11570
Li, T., Fu, J., Zeng, Z., Cohen, D., Li, J., Chen, Q., et al. (2020). TIMER2.0 for analysis of tumor-infiltrating immune cells. Nucleic Acids Res. 48, W509–W514. doi:10.1093/nar/gkaa407
Li, X., Xiong, X., Wang, K., Wang, L., Shu, X., Ma, S., et al. (2016a). Transcriptome-wide mapping reveals reversible and dynamic N(1)-methyladenosine methylome. Nat. Chem. Biol. 12, 311–316. doi:10.1038/nchembio.2040
Li, X., Zhu, P., Ma, S., Song, J., Bai, J., Sun, F., et al. (2015). Chemical pulldown reveals dynamic pseudouridylation of the mammalian transcriptome. Nat. Chem. Biol. 11, 592–597. doi:10.1038/nchembio.1836
Li, Y., Li, X., Yang, Y., Li, M., Qian, F., Tang, Z., et al. (2021b). TRlnc: A comprehensive database for human transcriptional regulatory information of lncRNAs. Brief. Bioinform 22, 1929–1939. doi:10.1093/bib/bbaa011
Li, Z., Zhang, Y., Fang, J., Xu, Z., Zhang, H., Mao, M., et al. (2023). NcPath: A novel platform for visualization and enrichment analysis of human non-coding RNA and KEGG signaling pathways. Bioinformatics 39, btac812. doi:10.1093/bioinformatics/btac812
Liu, H., Ma, J., Meng, J., and Zhang, L. (2021). MeT-DB V2.0: elucidating context-specific functions of N6-methyl-adenosine methyltranscriptome. Methods Mol. Biol. 2284, 507–518. doi:10.1007/978-1-0716-1307-8_27
Liu, J., Eckert, M. A., Harada, B. T., Liu, S. M., Lu, Z., Yu, K., et al. (2018). m(6)A mRNA methylation regulates AKT activity to promote the proliferation and tumorigenicity of endometrial cancer. Nat. Cell Biol. 20, 1074–1083. doi:10.1038/s41556-018-0174-4
Liu, K., Xu, P., Lv, J., Ge, H., Yan, Z., Huang, S., et al. (2023). Peritoneal high-fat environment promotes peritoneal metastasis of gastric cancer cells through activation of NSUN2-mediated ORAI2 m5C modification. Oncogene 42, 1980–1993. doi:10.1038/s41388-023-02707-5
Liu, N., and Pan, T. (2015). RNA epigenetics. Transl. Res. 165, 28–35. doi:10.1016/j.trsl.2014.04.003
Liu, N., Zhou, K. I., Parisien, M., Dai, Q., Diatchenko, L., and Pan, T. (2017). N6-methyladenosine alters RNA structure to regulate binding of a low-complexity protein. Nucleic Acids Res. 45, 6051–6063. doi:10.1093/nar/gkx141
Liu, S., Zhu, A., He, C., and Chen, M. (2020b). Repic: A database for exploring the N(6)-methyladenosine methylome. Genome Biol. 21, 100. doi:10.1186/s13059-020-02012-4
Liu, X., Liu, Z., Mao, X., and Li, Q. (2020a). m7GPredictor: an improved machine learning-based model for predicting internal m7G modifications using sequence properties. Anal. Biochem. 609, 113905. doi:10.1016/j.ab.2020.113905
Luo, X., Li, H., Liang, J., Zhao, Q., Xie, Y., Ren, J., et al. (2021). RMVar: an updated database of functional variants involved in RNA modifications. Nucleic Acids Res. 49, D1405–D1412. doi:10.1093/nar/gkaa811
Ma, J., Song, B., Wei, Z., Huang, D., Zhang, Y., Su, J., et al. (2022). m5C-Atlas: a comprehensive database for decoding and annotating the 5-methylcytosine (m5C) epitranscriptome. Nucleic Acids Res. 50, D196–D203. doi:10.1093/nar/gkab1075
Ma, Z., Li, Q., Liu, P., Dong, W., and Zuo, Y. (2020). METTL3 regulates m6A in endometrioid epithelial ovarian cancer independently of METTl14 and WTAP. Cell Biol. Int. 44, 2524–2531. doi:10.1002/cbin.11459
Machnicka, M. A., Dunin-Horkawicz, S., de Crecy-Lagard, V., and Bujnicki, J. M. (2016). tRNAmodpred: A computational method for predicting posttranscriptional modifications in tRNAs. Methods 107, 34–41. doi:10.1016/j.ymeth.2016.03.013
Mansi, L., Tangaro, M. A., Lo Giudice, C., Flati, T., Kopel, E., Schaffer, A. A., et al. (2021). REDIportal: millions of novel A-to-I RNA editing events from thousands of RNAseq experiments. Nucleic Acids Res. 49, D1012–D1019. doi:10.1093/nar/gkaa916
Mauer, J., Luo, X., Blanjoie, A., Jiao, X., Grozhik, A. V., Patil, D. P., et al. (2017). Reversible methylation of m(6)A(m) in the 5' cap controls mRNA stability. Nature 541, 371–375. doi:10.1038/nature21022
Meyer, K. D., Saletore, Y., Zumbo, P., Elemento, O., Mason, C. E., and Jaffrey, S. R. (2012). Comprehensive analysis of mRNA methylation reveals enrichment in 3' UTRs and near stop codons. Cell 149, 1635–1646. doi:10.1016/j.cell.2012.05.003
Miao, C., Fang, X., Chen, Y., Zhao, Y., and Guo, Q. (2021). Identification of an eight-m6A RNA methylation regulator prognostic signature of uterine corpus endometrial carcinoma based on bioinformatics analysis. Med. Baltim. 100, e27689. doi:10.1097/MD.0000000000027689
Milanowska, K., Mikolajczak, K., Lukasik, A., Skorupski, M., Balcer, Z., Machnicka, M. A., et al. (2013). RNApathwaysDB-a database of RNA maturation and decay pathways. Nucleic Acids Res. 41, D268–D272. doi:10.1093/nar/gks1052
Nakayama, H., Akiyama, M., Taoka, M., Yamauchi, Y., Nobe, Y., Ishikawa, H., et al. (2009). Ariadne: A database search engine for identification and chemical analysis of RNA using tandem mass spectrometry data. Nucleic Acids Res. 37, e47. doi:10.1093/nar/gkp099
Nassar, L. R., Barber, G. P., Benet-Pagès, A., Casper, J., Clawson, H., Diekhans, M., et al. (2023). The UCSC genome browser database: 2023 update. Nucleic Acids Res. 51, D1188–D1195. doi:10.1093/nar/gkac1072
Ni, W. J., Lu, H., Hou, B. B., Zeng, J., Zhou, H., Ma, N. N., et al. (2023). RNA N(6) -methyladenosine modifications and potential targeted therapeutic strategies in kidney disease. Br. J. Pharmacol. 180, 5–24. doi:10.1111/bph.15968
Nie, F., Feng, P., Song, X., Wu, M., Tang, Q., and Chen, W. (2020). Rnawre: A resource of writers, readers and erasers of RNA modifications. Database (Oxford) 2020, baaa049. doi:10.1093/database/baaa049
Nie, F., Tang, Q., Liu, Y., Qin, H., Liu, S., Wu, M., et al. (2022). Rname: A comprehensive database of RNA modification enzymes. Comput. Struct. Biotechnol. J. 20, 6244–6249. doi:10.1016/j.csbj.2022.11.022
Nombela, P., Miguel-Lopez, B., and Blanco, S. (2021). The role of m(6)A, m(5)C and psi RNA modifications in cancer: novel therapeutic opportunities. Mol. Cancer 20, 18. doi:10.1186/s12943-020-01263-w
Pantano, L., Estivill, X., and Marti, E. (2010). SeqBuster, a bioinformatic tool for the processing and analysis of small RNAs datasets, reveals ubiquitous miRNA modifications in human embryonic cells. Nucleic Acids Res. 38, e34. doi:10.1093/nar/gkp1127
Pavlova, N. N., Zhu, J., and Thompson, C. B. (2022). The hallmarks of cancer metabolism: still emerging. Cell Metab. 34, 355–377. doi:10.1016/j.cmet.2022.01.007
Pu, X., Gu, Z., and Gu, Z. (2020). ALKBH5 regulates IGF1R expression to promote the proliferation and tumorigenicity of endometrial cancer. J. Cancer 11, 5612–5622. doi:10.7150/jca.46097
Roundtree, I. A., Evans, M. E., Pan, T., and He, C. (2017). Dynamic RNA modifications in gene expression regulation. Cell 169, 1187–1200. doi:10.1016/j.cell.2017.05.045
Rowlands, C. E., Folberg, A. M., Beickman, Z. K., Devor, E. J., Leslie, K. K., and Givens, B. E. (2023). Particles and prejudice: nanomedicine approaches to reducing health disparities in endometrial cancer. Small, e2300096. doi:10.1002/smll.202300096
Ryvkin, P., Leung, Y. Y., Silverman, I. M., Childress, M., Valladares, O., Dragomir, I., et al. (2013). Hamr: high-throughput annotation of modified ribonucleotides. RNA 19, 1684–1692. doi:10.1261/rna.036806.112
Schaefer, M. R. (2021). The regulation of RNA modification systems: the next frontier in epitranscriptomics? Genes (Basel) 12, 345. doi:10.3390/genes12030345
Schwartz, S., Bernstein, D. A., Mumbach, M. R., Jovanovic, M., Herbst, R. H., León-Ricardo, B. X., et al. (2014). Transcriptome-wide mapping reveals widespread dynamic-regulated pseudouridylation of ncRNA and mRNA. Cell 159, 148–162. doi:10.1016/j.cell.2014.08.028
Shen, J., Feng, X. P., Hu, R. B., Wang, H., Wang, Y. L., Qian, J. H., et al. (2021). N-methyladenosine reader YTHDF2-mediated long noncoding RNA FENDRR degradation promotes cell proliferation in endometrioid endometrial carcinoma. Lab. Invest. 101, 775–784. doi:10.1038/s41374-021-00543-3
Siegel, R. L., Miller, K. D., Wagle, N. S., and Jemal, A. (2023). Cancer statistics. CA Cancer J. Clin. 73, 17–48. doi:10.3322/caac.21763
Song, B., Huang, D., Zhang, Y., Wei, Z., Su, J., Pedro de Magalhães, J., et al. (2022b). m6A-TSHub: unveiling the context-specific m(6)A methylation and m6A-affecting mutations in 23 human tissues. Genomics Proteomics Bioinforma. doi:10.1016/j.gpb.2022.09.001
Song, B., Tang, Y., Chen, K., Wei, Z., Rong, R., Lu, Z., et al. (2020b). m7GHub: deciphering the location, regulation and pathogenesis of internal mRNA N7-methylguanosine (m7G) sites in human. Bioinformatics 36, 3528–3536. doi:10.1093/bioinformatics/btaa178
Song, B., Tang, Y., Wei, Z., Liu, G., Su, J., Meng, J., et al. (2020a). Piano: A web server for pseudouridine-site (psi) identification and functional annotation. Front. Genet. 11, 88. doi:10.3389/fgene.2020.00088
Song, H., Zhang, J., Liu, B., Xu, J., Cai, B., Yang, H., et al. (2022a). Biological roles of RNA m(5)C modification and its implications in Cancer immunotherapy. Biomark. Res. 10, 15. doi:10.1186/s40364-022-00362-8
Squires, J. E., Patel, H. R., Nousch, M., Sibbritt, T., Humphreys, D. T., Parker, B. J., et al. (2012). Widespread occurrence of 5-methylcytosine in human coding and non-coding RNA. Nucleic Acids Res. 40, 5023–5033. doi:10.1093/nar/gks144
Sun, J., Li, L., Chen, H., Gan, L., and Guo, X. (2022). Identification and validation of an m7G-related lncRNAs signature for prognostic prediction and immune function analysis in endometrial cancer. Genes (Basel) 13, 1301. doi:10.3390/genes13081301
Sun, W. J., Li, J. H., Liu, S., Wu, J., Zhou, H., Qu, L. H., et al. (2016). RMBase: A resource for decoding the landscape of RNA modifications from high-throughput sequencing data. Nucleic Acids Res. 44, D259–D265. doi:10.1093/nar/gkv1036
Tan, X., Zheng, C., Zhuang, Y., Jin, P., and Wang, F. (2023). The m6A reader PRRC2A is essential for meiosis I completion during spermatogenesis. Nat. Commun. 14, 1636. doi:10.1038/s41467-023-37252-y
Tang, Y., Chen, K., Song, B., Ma, J., Wu, X., Xu, Q., et al. (2021). m6A-Atlas: a comprehensive knowledgebase for unraveling the N6-methyladenosine (m6A) epitranscriptome. Nucleic Acids Res. 49, D134–D143. doi:10.1093/nar/gkaa692
Tao, M. H., and Freudenheim, J. L. (2010). DNA methylation in endometrial cancer. Epigenetics 5, 491–498. doi:10.4161/epi.5.6.12431
Tian, J., Cheng, H., Wang, N., and Wang, C. (2023). SLERT, as a novel biomarker, orchestrates endometrial cancer metastasis via regulation of BDNF/TRKB signaling. World J. Surg. Oncol. 21, 27. doi:10.1186/s12957-022-02821-w
Volpon, L., Culjkovic-Kraljacic, B., Osborne, M. J., Ramteke, A., Sun, Q., Niesman, A., et al. (2016). Importin 8 mediates m7G cap-sensitive nuclear import of the eukaryotic translation initiation factor eIF4E. Proc. Natl. Acad. Sci. U. S. A. 113, 5263–5268. doi:10.1073/pnas.1524291113
Wang, C., Kong, F., Ma, J., Miao, J., Su, P., Yang, H., et al. (2022b). IGF2BP3 enhances the mRNA stability of E2F3 by interacting with LINC00958 to promote endometrial carcinoma progression. Cell Death Discov. 8, 279. doi:10.1038/s41420-022-01045-x
Wang, H., Tang, A., Cui, Y., Gong, H., and Li, H. (2023a). LRPPRC facilitates tumor progression and immune evasion through upregulation of m(6)A modification of PD-L1 mRNA in hepatocellular carcinoma. Front. Immunol. 14, 1144774. doi:10.3389/fimmu.2023.1144774
Wang, L., Dou, X., Chen, S., Yu, X., Huang, X., Zhang, L., et al. (2023c). YTHDF2 inhibition potentiates radiotherapy antitumor efficacy. Cancer Cell 41, 1294–1308.e8. doi:10.1016/j.ccell.2023.04.019
Wang, S., Li, H., Lian, Z., and Deng, S. (2022a). The role of RNA modification in HIV-1 infection. Int. J. Mol. Sci. 23, 7571. doi:10.3390/ijms23147571
Wang, Y., Ren, F., Song, Z., Wang, X., and Ma, X. (2020). Multiomics profile and prognostic gene signature of m6A regulators in uterine corpus endometrial carcinoma. J. Cancer 11, 6390–6401. doi:10.7150/jca.46386
Wang, Y., Wei, J., Feng, L., Li, O., Huang, L., Zhou, S., et al. (2023b). Aberrant m5C hypermethylation mediates intrinsic resistance to gefitinib through NSUN2/YBX1/QSOX1 axis in EGFR-mutant non-small-cell lung cancer. Mol. Cancer 22, 81. doi:10.1186/s12943-023-01780-4
Weng, H., Huang, F., Yu, Z., Chen, Z., Prince, E., Kang, Y., et al. (2022). The m(6)A reader IGF2BP2 regulates glutamine metabolism and represents a therapeutic target in acute myeloid leukemia. Cancer Cell 40, 1566–1582.e10. doi:10.1016/j.ccell.2022.10.004
Wiener, D., and Schwartz, S. (2021). The epitranscriptome beyond m(6)A. Nat. Rev. Genet. 22, 119–131. doi:10.1038/s41576-020-00295-8
Wu, R., Sun, B., Sun, J. G., Zhang, J., Zhang, T., Li, A., et al. (2019). A novel m(6)A reader Prrc2a controls oligodendroglial specification and myelination. Cell Res. 29, 23–41. doi:10.1038/s41422-018-0113-8
Wu, Y., Wang, Z., Shen, J., Yan, W., Xiang, S., Liu, H., et al. (2022). The role of m6A methylation in osteosarcoma biological processes and its potential clinical value. Hum. Genomics 16, 12. doi:10.1186/s40246-022-00384-1
Xiang, S., Liu, K., Yan, Z., Zhang, Y., and Sun, Z. (2016). RNAMethPre: A web server for the prediction and query of mRNA m6A sites. PLoS One 11, e0162707. doi:10.1371/journal.pone.0162707
Xiong, Y., Dowdy, S. C., Eberhardt, N. L., Podratz, K. C., and Jiang, S. W. (2006). hMLH1 promoter methylation and silencing in primary endometrial cancers are associated with specific alterations in MBDs occupancy and histone modifications. Gynecol. Oncol. 103, 321–328. doi:10.1016/j.ygyno.2006.03.045
Xu, T., Ding, H., Chen, J., Lei, J., Zhao, M., Ji, B., et al. (2022). Research progress of DNA methylation in endometrial cancer. Biomolecules 12, 938. doi:10.3390/biom12070938
Yanagi, Y., Watanabe, T., Hara, Y., Sato, Y., Kimura, H., and Murata, T. (2022). EBV exploits RNA m(6)A modification to promote cell survival and progeny virus production during lytic cycle. Front. Microbiol. 13, 870816. doi:10.3389/fmicb.2022.870816
Yang, H., Wang, Y., Xiang, Y., Yadav, T., Ouyang, J., Phoon, L., et al. (2022). FMRP promotes transcription-coupled homologous recombination via facilitating TET1-mediated m5C RNA modification demethylation. Proc. Natl. Acad. Sci. U. S. A. 119, e2116251119. doi:10.1073/pnas.2116251119
Yang, X., Yang, Y., Sun, B. F., Chen, Y. S., Xu, J. W., Lai, W. Y., et al. (2017). 5-methylcytosine promotes mRNA export - NSUN2 as the methyltransferase and ALYREF as an m(5)C reader. Cell Res. 27, 606–625. doi:10.1038/cr.2017.55
Yang, Y., Wang, L., Han, X., Yang, W. L., Zhang, M., Ma, H. L., et al. (2019). RNA 5-methylcytosine facilitates the maternal-to-zygotic transition by preventing maternal mRNA decay. Mol. Cell 75, 1188–1202. doi:10.1016/j.molcel.2019.06.033
Yankova, E., Blackaby, W., Albertella, M., Rak, J., De Braekeleer, E., Tsagkogeorga, G., et al. (2021). Small-molecule inhibition of METTL3 as a strategy against myeloid leukaemia. Nature 593, 597–601. doi:10.1038/s41586-021-03536-w
Yin, H., Zhang, X., Yang, P., Zhang, X., Peng, Y., Li, D., et al. (2021). RNA m6A methylation orchestrates cancer growth and metastasis via macrophage reprogramming. Nat. Commun. 12, 1394. doi:10.1038/s41467-021-21514-8
Zaccara, S., Ries, R. J., and Jaffrey, S. R. (2019). Reading, writing and erasing mRNA methylation. Nat. Rev. Mol. Cell Biol. 20, 608–624. doi:10.1038/s41580-019-0168-5
Zhan, L., Zhang, J., Zhang, J. H., Liu, X. J., Guo, B., Chen, J. H., et al. (2023). METTL3 facilitates immunosurveillance by inhibiting YTHDF2-mediated NLRC5 mRNA degradation in endometrial cancer. Biomark. Res. 11, 43. doi:10.1186/s40364-023-00479-4
Zhang, C., Guo, C., Li, Y., Ouyang, L., Zhao, Q., and Liu, K. (2021c). The role of YTH domain containing 2 in epigenetic modification and immune infiltration of pan-cancer. J. Cell Mol. Med. 25, 8615–8627. doi:10.1111/jcmm.16818
Zhang, G., Xu, Y., Wang, X., Zhu, Y., Wang, L., Zhang, W., et al. (2022). Dynamic FMR1 granule phase switch instructed by m6A modification contributes to maternal RNA decay. Nat. Commun. 13, 859. doi:10.1038/s41467-022-28547-7
Zhang, L. S., Liu, C., Ma, H., Dai, Q., Sun, H. L., Luo, G., et al. (2019). Transcriptome-wide mapping of internal N(7)-methylguanosine methylome in mammalian mRNA. Mol. Cell 74, 1304–1316. doi:10.1016/j.molcel.2019.03.036
Zhang, L., Wan, Y., Zhang, Z., Jiang, Y., Gu, Z., Ma, X., et al. (2021b). IGF2BP1 overexpression stabilizes PEG10 mRNA in an m6A-dependent manner and promotes endometrial cancer progression. Theranostics 11, 1100–1114. doi:10.7150/thno.49345
Zhang, L., Wan, Y., Zhang, Z., Jiang, Y., Lang, J., Cheng, W., et al. (2021a). FTO demethylates m6A modifications in HOXB13 mRNA and promotes endometrial cancer metastasis by activating the WNT signalling pathway. RNA Biol. 18, 1–14. doi:10.1080/15476286.2020.1841458
Zhang, Y., Jiang, J., Ma, J., Wei, Z., Wang, Y., Song, B., et al. (2023). DirectRMDB: A database of post-transcriptional RNA modifications unveiled from direct RNA sequencing technology. Nucleic Acids Res. 51, D106–D116. doi:10.1093/nar/gkac1061
Zhang, Y., and Yang, Y. (2021). Effects of m6A RNA methylation regulators on endometrial cancer. J. Clin. Lab. Anal. 35, e23942. doi:10.1002/jcla.23942
Zhang, Z., Zhou, D., Lai, Y., Liu, Y., Tao, X., Wang, Q., et al. (2012). Estrogen induces endometrial cancer cell proliferation and invasion by regulating the fat mass and obesity-associated gene via PI3K/AKT and MAPK signaling pathways. Cancer Lett. 319, 89–97. doi:10.1016/j.canlet.2011.12.033
Zhao, J., Zou, J., Jiao, W., Lin, L., Wang, J., and Lin, Z. (2022). Construction of N-7 methylguanine-related mRNA prognostic model in uterine corpus endometrial carcinoma based on multi-omics data and immune-related analysis. Sci. Rep. 12, 18813. doi:10.1038/s41598-022-22879-6
Zheng, L. L., Zhou, K. R., Liu, S., Zhang, D. Y., Wang, Z. L., Chen, Z. R., et al. (2018). dreamBase: DNA modification, RNA regulation and protein binding of expressed pseudogenes in human health and disease. Nucleic Acids Res. 46, D85–D91. doi:10.1093/nar/gkx972
Zhou, D., Wang, H., Bi, F., Xing, J., Gu, Y., Wang, C., et al. (2021). M6ADD: A comprehensive database of m(6)A modifications in diseases. RNA Biol. 18, 2354–2362. doi:10.1080/15476286.2021.1913302
Zhou, K. I., Shi, H., Lyu, R., Wylder, A. C., Matuszek, Ż., Pan, J. N., et al. (2019). Regulation of Co-transcriptional pre-mRNA splicing by m(6)A through the low-complexity protein hnRNPG. Mol. Cell 76, 70–81. doi:10.1016/j.molcel.2019.07.005
Zhou, X. C., Dowdy, S. C., Podratz, K. C., and Jiang, S. W. (2007). Epigenetic considerations for endometrial cancer prevention, diagnosis and treatment. Gynecol. Oncol. 107, 143–153. doi:10.1016/j.ygyno.2007.06.019
Zhou, X., Zhang, H., Duan, Y., Zhu, J., and Dai, H. (2023). m6A-related long noncoding RNAs predict prognosis and indicate therapeutic response in endometrial carcinoma. J. Clin. Lab. Anal. 37, e24813. doi:10.1002/jcla.24813
Zhou, Y., Zeng, P., Li, Y. H., Zhang, Z., and Cui, Q. (2016). Sramp: prediction of mammalian N6-methyladenosine (m6A) sites based on sequence-derived features. Nucleic Acids Res. 44, e91. doi:10.1093/nar/gkw104
Keywords: RNA modification, endometrial cancer, N6-methyladenosine (m6A), 5-methylcytidine (m5c), RNA therapy
Citation: Jin Z, Sheng J, Hu Y, Zhang Y, Wang X and Huang Y (2023) Shining a spotlight on m6A and the vital role of RNA modification in endometrial cancer: a review. Front. Genet. 14:1247309. doi: 10.3389/fgene.2023.1247309
Received: 25 June 2023; Accepted: 19 September 2023;
Published: 11 October 2023.
Edited by:
Jiaqiu Li, Affiliated Hospital of Weifang Medical University, ChinaReviewed by:
Neelum Yousaf Zai, Case Western Reserve University, United StatesSarah K. Azzam, Khalifa University, United Arab Emirates
Copyright © 2023 Jin, Sheng, Hu, Zhang, Wang and Huang. This is an open-access article distributed under the terms of the Creative Commons Attribution License (CC BY). The use, distribution or reproduction in other forums is permitted, provided the original author(s) and the copyright owner(s) are credited and that the original publication in this journal is cited, in accordance with accepted academic practice. No use, distribution or reproduction is permitted which does not comply with these terms.
*Correspondence: Yiping Huang, buddyeva@zju.edu.cn