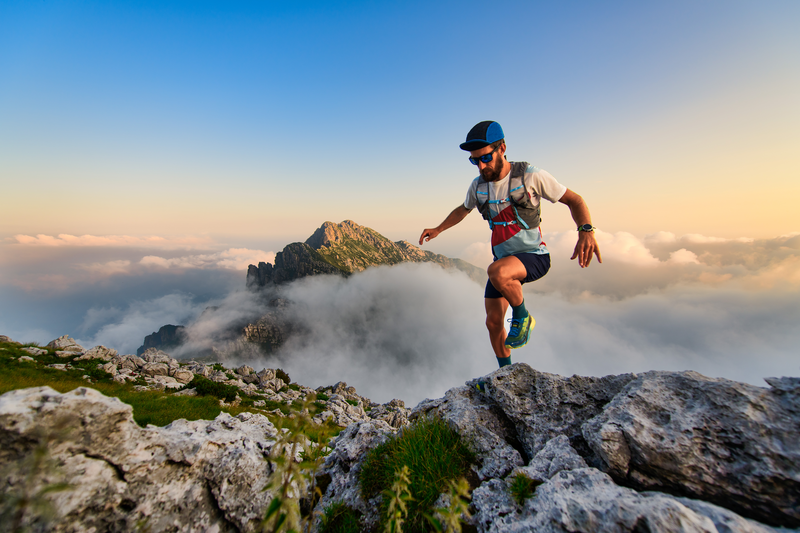
94% of researchers rate our articles as excellent or good
Learn more about the work of our research integrity team to safeguard the quality of each article we publish.
Find out more
REVIEW article
Front. Genet. , 25 July 2023
Sec. Cancer Genetics and Oncogenomics
Volume 14 - 2023 | https://doi.org/10.3389/fgene.2023.1234645
This article is part of the Research Topic Advance in Translational Genomics Based Biomarkers for Cancer Management: Discovery and Application View all 6 articles
Background: Gastric cancer (GC) is one of the most common malignancies, with a low 5-year survival rate. However, if diagnosed at an early stage, it can be cured by endoscopic treatment and has a good prognosis. While gastrointestinal X-ray and upper endoscopy are used as national GC screening methods in some GC high-risk countries, such as Japan and Korea, their radiation exposure, invasiveness, and high cost suggest that they are not the optimal tools for early detection of GC in many countries. Therefore, a cost-effective, and highly accurate method for GC early detection is urgently needed in clinical settings. DNA methylation plays a key role in cancer progression and metastasis and has been demonstrated as a promising marker for cancer early detection.
Aims and methods: This review provides a comprehensive overview of the current status of DNA methylation markers associated with GC, the assays developed for GC early detection, challenges in methylation marker discovery and application, and the future prospects of utilizing methylation markers for early detection of GC. Through our analysis, we found that the currently reported DNA methylation markers related to GC are mainly in the early discovery stage. Most of them have only been evaluated in tissue samples. The majority of non-invasive assays developed based on blood lack standardized sampling protocols, pre-analytical procedures, and multicenter validation, and they exhibit insufficient sensitivity for early-stage GC detection. Meanwhile, the reported GC DNA methylation markers are generally considered pan-cancer markers.
Conclusion: Therefore, future endeavors should focus on identifying additional methylation markers specific to GC and establishing non-invasive diagnostic assays that rely on these markers. These assays should undergo multicenter, large-scale prospective validation in diverse populations.
Gastric cancer (GC) is a major global health concern, it caused 1,089,103 new cases and 768,793 new deaths in 2020, ranking fifth for incidence and fourth for mortality among all cancer types globally (Sung et al., 2021). However, more than 60.0% GC new cases were found in Eastern Asian countries, such as China, Japan, Korea and Mongolia (Ning et al., 2022; Sekiguchi et al., 2022). Although the GC incidence was remarkable decreasing during the past several decades with the improvement of medical treatments and public health strategies (Sekiguchi et al., 2022; Song et al., 2022), the 5-year survival rate for GC still relatively low (about 30.0%–40.0%) in most countries (Sekiguchi et al., 2022). In contrast, 2 GC high-risk countries, Japan and Korea, reported a high 5-year survival rate for GC of 60.0%–70.0%, which was due to the long-term national GC screening programs, and many of these cases were found at early stage (Hamashima, 2020; Sekiguchi et al., 2022). In Japan and Korea, the GC screening strategies included gastrointestinal X-ray and upper endoscopy detection. However, because upper endoscopy is an invasive method with several side effects and a low compliance rate, and gastrointestinal X-ray suffer the risk of radiation exposure, they were not the best choice for primary screening of GC. Other non-invasive methods, such as the CA72-4, PGI/II and the ABC method (combination assay of Helicobacter pylori and serum pepsinogen), have insufficient sensitivity and specificity (Yamaguchi et al., 2016; Xu Y. et al., 2021).
DNA methylation is the most widely studied epigenetic modification which plays a significant role in cancer progression and metastasis (Davalos and Esteller, 2022). Many of DNA methylation occur early in tumorigenesis, which allows DNA methylation-based markers to be suitable for early detection of cancer (Locke et al., 2019; Zhao et al., 2019; Zhao et al., 2020). Because DNA methylation can also be detected in body fluids such as blood, stool, urine, and cerebrospinal fluid (Liu et al., 2020; Rahat et al., 2020), it is more stable, sensitive and specific than other cell free nucleic acid markers (miRNA, lncRNA or mRNA), indicating that DNA methylation is a promising non-invasive marker for cancer early detection (Jamshidi et al., 2022). During the past decade, several commercially available DNA methylation-based assays have been developed and approved by the United States Food and Drug Administration (FDA) and Chinese National Medical Products Administration (NMPAfor clinical application. For example, the plasma SPET9 methylation test (Epi proColon) (Church et al., 2014), and the stool multiple-target assay (Cologuard), which contained two methylation markers (Imperiale et al., 2014), were successfully used for colorectal cancer (CRC) screening (Wolf et al., 2018). Moreover, many studies have proven that DNA methylation markers are more sensitive and specific than traditional blood protein markers (Young et al., 2016; Cai et al., 2021; Lin et al., 2021). Promoter CpG island hypermethylation and tumor suppressor genes inactivated in gastric carcinogenesis has also been frequently observed (Patel et al., 2017), thus DNA methylation can also be a potential marker for GC early detection. Recent advances in translational genomics and analytics, drives numerous potential DNA methylation markers have come to light in clinics.
This review provides a comprehensive overview of the DNA methylation changes in GC, and summarized the achievements, challenges and possible further direction for DNA methylation as a potential tool for GC early detection.
In this review, we performed an extensive search and analysis of previously published DNA methylation markers to evaluate their effectiveness in the detection of GC across different sample types (Table 1; Table 2; Table 3). According to our analysis, current research on DNA methylation in GC primarily focuses on tissue, gastric washes/juice, blood (plasma or serum) and stool samples. Tissue samples are primarily employed for the initial identification of methylation markers, whereas blood or stool samples are commonly used for the subsequent validation of identified markers. Regarding the sample types used in the previous studies, we found that plasma was the most commonly used sample type for DNA methylation analysis, with 26 studies utilizing this sample type. However, in 6 of these studies, plasma volumes were ≤1mL, which may have impacted the accuracy and sensitivity of the analysis. In contrast, 3.5 mL of plasma was used in 7 studies. Serum was used in 13 studies, and the volume of serum used was ≤0.5 mL in 53.8% of these studies.
The methods used for analyzing DNA methylation markers included methylation-specific PCR (MSP), nested MSP, quantitative methylation-specific PCR (qMSP), Sanger sequencing, combined restriction digital PCR (CORD), methylation-sensitive melt curve analysis (MS-MCA), pyrosequencing and bisulfite sequencing (Table 1; Table 2; Table 3). Our analysis revealed that MSP was the most commonly used technology in tissue and plasma/serum samples. However, the reported frequency of MSP has significantly decreased in the past 5 years. Similarly, most reports on pyrosequencing for GC methylation analysis were published 5 years ago and mainly focused on tissue and gastric wash samples. In contrast, qMSP has emerged as a more sensitive and convenient method, and it has been increasingly used for plasma/serum analysis in recent years. While the bisulfite sequencing, especially the target bisulfite sequencing is a commonly used method for pan-cancer early detection in recent years (Table 3).
A total of 59 DNA methylation markers were evaluated individually in 41 studies, and 13 out of the 59 markers (P16, RNF180, RUNX3, SEPT9, E-cadherin, Reprimo, ELMO1, TFPI2, RASSF1A, SFRP2, RPRM, ZIC1, and DAPK) were reported at least 2 times. Among these markers, 37 out of them were analyzed in blood samples (plasma or serum), and the repeatedly evaluated markers were also assessed in blood samples at least once. In addition to blood samples, this review also included markers evaluated in tissue and gastric washes/juice. For instance, Sox17 (Oishi et al., 2012) and BARHL2 (Yamamoto et al., 2016) were exclusively evaluated in gastric washes, and 15 markers (CDO1, DCLK1, SFRP1, ZNF331, ZSCAN18, FLT3, LINC00643, JAM2, BHLHE22, RIMS1,GUSBP5, ZNF3, CHFR, NMDAR2B, and RECK) were solely evaluated in tissue samples (fresh frozen tissue or FFPE). The remaining six markers (MINT25, RORA, PRDM5, MLF1, ADAM2, and GDNF) were evaluated simultaneously in both tissue and gastric wash samples (Table 1).
The most frequently evaluated methylated marker for GC early detection was P16, which was assessed in 7 studies across various sample types, including tissue, serum, or plasma (Lee et al., 2002; Kanyama et al., 2003; Ichikawa et al., 2004; Koike et al., 2004; Hou et al., 2005; Abbaszadegan et al., 2008; Saliminejad et al., 2020). Nonetheless, the sensitivity of P16 in blood samples was relatively low in these studies (Lee et al., 2002; Ichikawa et al., 2004; Saliminejad et al., 2020). Apart from P16, RNF180, and RUNX3 were the most frequently investigated methylation markers, each mentioned in 5 studies (Table 1). RNF180 was studied in various research conditions, including marker discovery to multiple center applications (Cheung et al., 2012; Zhang et al., 2014; Cao et al., 2020; Xu J. et al., 2021). Cheung et al. (2012) first reported the feasibility of using RNF180 methylation as an early detection marker for GC in 0.8 mL plasma, showing a sensitivity of 56.3% and specificity of 100.0%. Three studies using 3.5 mL plasma showed that RNF180 methylation had sensitivities ranging from 32.4% to 71.7%, with specificities of 59.4%–88.4% (Cao et al., 2020; Xu J. et al., 2021; Zhao et al., 2022a). RUNX3 also were explored both in tissue and blood samples, its sensitivities in blood range from 29.2% to 58.3%, with the specificities of 79.2%–100.0% (Sakakura et al., 2009; Lin et al., 2017; Hideura et al., 2020; Saliminejad et al., 2020). The three studies examining SEPT9 analyzed it under identical research conditions, yielding sensitivities of 28.4%–48.3% and specificities of 86.9%–98.2% (Cao et al., 2020; Xu J. et al., 2021; Zhao et al., 2022b). Reprimo showed 62.0%–95.3% sensitivities and 90.3%–100.0% specificities in plasma for GC detection (Bernal et al., 2008), and also exhibited 94.3% sensitivity and 92.9% specificity in 1 mL serum (Wang et al., 2016), indicated that it is a promising marker for early detection of GC. E-cadherin has exhibited 100.0% specificity in previous studies conducted on three serum cohorts, but its sensitivity remains relatively low, ranging from 22.2% to 57.4% (Lee et al., 2002; Ichikawa et al., 2004; Koike et al., 2004). Pimson et al. (2016) found that methylated RASSF1A and PCDH10 have high sensitivities (83.2% and 94.1%) and specificities (94.6% and 97.0%) in 0.2 mL plasma, while another study demonstrated that RASSF1A had only 33.3% sensitivity with a specificity of 100.0% in 2 mL plasma (Saliminejad et al., 2020). The ZIC1 was evaluated not only in GC samples but also in samples of gastric precancerous lesions, such as intraepithelial neoplasia (IN) and intestinal metaplasia (IM) (Chen et al., 2015; Lin et al., 2017). Other methylation markers such as SFRP2 (Miao et al., 2020), RPRM (Saliminejad et al., 2020), OSR2 (Li et al., 2016), PPFIA3 (Li et al., 2016), ZNF582 (Peng et al., 2022) and C9orf50 (Li et al., 2022) showed sensitivities higher than 50.0% with specificities higher than 80.0% in plasma, indicating their potential as non-invasive tools for GC early detection.
For early-stage GC detection, most methylation markers lack of the performance evaluation in stage I GC (Table 1). RNF180 and SEPT9 both showed sensitivities less than 20.0% in stage I GC (Xu J. et al., 2021). Among the markers evaluated in stage I GC, C9orf50 had sensitivities ranging from 21.4% to 44.4% (Li et al., 2022), while P16, E-cadherin and SFRP2 showed the relatively higher sensitivities ≥50.0% (Lee et al., 2002; Miao et al., 2020). Only one study containing methylated KCNQ5, C9orf50, and CLIP4 was evaluated in multiple cohorts and showed good reproducibility in three plasma cohorts (Li et al., 2022).
Single DNA methylation markers for GC detection often exhibit insufficient sensitivity, particularly for early-stage cancer, due to tumor heterogeneity and individual differences. Developing methylation panels using multiple DNA methylation markers is an effective strategy to improve sensitivity and has been successfully applied in detection of CRC (Zhao et al., 2019; Zhao et al., 2020) and lung cancer (LC) (Zhang et al., 2017; Wei et al., 2021). In the past decade, several methylation markers were evaluated in panels for GC early detection, with all studies using blood samples, including 10 plasma and three serum sample cohorts (Table 2). The SEPT9 and RNF180 combination was the first and only non-invasive panel approved by NMPA for plasma GC detection in 2020. A previous study indicated that the combination of SEPT9 and RNF180 improved sensitivities from 28.4%–32.4%–40.5% with a specificity of 85.3% (Cao et al., 2020). Another study using a combination of SEPT9, RNF180, and CA72-4, achieved 33.3% and 68.6% sensitivities for stage I and overall stage GC, with a specificity of 85.1% (Xu J. et al., 2021). Combining RNF180 with other markers, such as DAPK1 and SFRP2, showed a high sensitivity of 87.7% but a lower specificity of 38.1% (Zhang et al., 2014).
The combination of P16, E-cadherin, and RARb in a panel resulted in a significant improvement in sensitivity compared to using a single marker. However, the sensitivity of the panel remained relatively low (Koike et al., 2004). In contrast, the combination of FAM5C and MYLK demonstrated an area under the curve (AUC) of 0.84 for GC detection, indicating its potential as a non-invasive blood-based method for early detection of GC (Chen et al., 2012). One panel including Reprimo and hMLH1 identified 84.0% of GC cases with a specificity of 96.7%. This panel could also be expanded to detect gastric dysplasia and IM with sensitivities of 76.0% and 34.0%, respectively (Liu and Yang, 2015). Shin et al. (2016) reported a methylation panel involving four new markers, PYCARD, APAF1, MINT1, and BRCA1, with a sensitivity and specificity of 97.6% and 66.3%. Two other studies used low volume blood samples and included three methylation markers by using MSP, showing an increasing trend of sensitivity but a decline in specificity when compared to single markers (Li et al., 2016; Lin et al., 2017). Anderson et al. (2018) developed and validated a novel panel including methylated ELMO1, C13orf18, and ZNF569, which demonstrated a promising sensitivity of 86.0% and a specificity of 95.0%. Ren et al. (2022) used methylated CpG tandem amplification and sequencing (MCTA-Seq) to develop a panel with 153 cfDNA methylation markers, which could detect 44.0% of stage I GC in plasma. Li et al. (2022) integrated KCNQ5, C9orf50 and CLIP4 in a single tube qMSP panel, and evaluated its performance in three cohorts, finding that it had about 10.0%–30.0% in increase of sensitivity compared with single markers. Ruan et al. (2023) also developed a qMSP panel included seven methylation markers, and validated in two independent cohorts, achieving sensitivities and specificities of 80.0%–82.0% and 65.0%–69.0%, respectively.
Except for methylation panels for single cancer type early detection, detecting multiple cancer types together in one panel, called a pan-cancer test, is a new strategy for reducing cancer morbidity and mortality (Duffy et al., 2021; Jamshidi et al., 2022). Seven pan-cancer tests based on methylation markers have been summarized in this review, which have been applied to at least two cancer types, including GC (Table 3). Four of these tests were developed using bisulfite sequencing, all of which demonstrated high specificities higher than 95.0% (Chen et al., 2020; Kandimalla et al., 2021; Klein et al., 2021; Yang et al., 2023). Moreover, most of the bisulfite sequencing based pan-cancer tests can identify tissue of origin (TOO) (Table 3). As for qMSP-based method, Peng et al. (2022) developed a panel by combined using ZNF582, ELMO1, and TFPI2, which can detect GC, esophageal cancer (EC) and esophagogastric junction cancer (EJC) together, and achieved 67.9% and 71.0% sensitivities for GC and all cancer types, respectively, with a specificity of 90.0%. Ma Y. et al. (2022) reported a six methylation markers panel for detection of EC, GC and CRC in plasma, it showed 69.9% and 76.6% for GC and all cancer types with a specificity of 89.2%. In addition to blood, stool was also utilized as a sample type for detection of the pan-cancer in gastrointestinal tract. For example, methylation RASSF2 and SFRP2 were combined using for detection of CRC and GC in 0.1 g stool samples (Nagasaka et al., 2009), and Ma et al used 3 g stool as a sampling type for simultaneous detection of CRC and GC, and it could detect 67.5% GC with a specificity of 97.8% (Ma L. et al., 2022).
Indeed, DNA methylation-based cancer early detection tests face several challenges that need to be addressed before they can be widely adopted in clinical settings (Figure 1). One of the major challenges is the complexity of the DNA methylation detection process. The process often involves bisulfite treatment, PCR amplification, and sequencing or other detection methods. These steps can introduce errors or biases into the results, which can affect the accuracy and reproducibility of the test. In addition to the technical challenges, there are several other factors that can impact the performance of DNA methylation-based cancer early detection tests. For example, the quality of the sample is critical, and the performance of the test can be affected by the pre-analytical conditions, such as the time and temperature of sample storage and transportation. Moreover, the accuracy of the test can be influenced by the selection of the target CpG sites and the panel design. It is important to ensure that the selected CpG sites are informative and specific for the target cancer types, and that the panel design is optimized for sensitivity and specificity. Another critical factor is the quality of the enrolled subjects and the selection criteria. DNA methylation-based cancer early detection tests may have limited sensitivity in early-stage cancers, and false positive results may occur in some cases. Therefore, it is important to carefully select the enrolled subjects based on their clinical and pathological characteristics, such as age, gender, tumor stage, and histological type, to minimize the risk of false positives or false negatives. Lastly, the quality of the operators and the analytical methods used for the test are also important factors that can affect the performance and reproducibility of the test. Therefore, it is crucial to establish standard operating procedures and quality control measures to ensure that the test results are accurate and reliable.
FIGURE 1. The challenges and future of DNA methylation markers for early detection of GC. PPV, positive predictive value; NPV, negative predictive value. Created with MedPeer (www.medpeer.cn).
As mentioned in this review, the samples involved in GC DNA methylation tests include fresh frozen tissue, FFPE, plasma, serum, gastric washes/juice, and stool (Figure 1). However, fresh frozen tissue, FFPE, and gastric washes/juice are invasive sample types and therefore not suitable for large-scale screening or early diagnosis of GC. Tissue samples are more appropriate for discovering DNA methylation markers rather than early detection. In comparison, the methylation level of each marker is consistently higher in tissues than in plasma and serum, which due to the proportion of circulating tumor DNA (ctDNA) in the blood is much lower than that of tumor DNA in the tissues (Abbosh et al., 2018). Therefore, when we translate the markers that from discovery stage in GC tissues to blood-based assay development, a significant decrease in sensitivities might be observed in blood samples (Table 1).
Blood is a convenient, non-invasive, and high-throughput processable sample, that is, easily accessible. Plasma and serum are two main sample types for blood ctDNA analysis, as indicated by the studies summarized in this review (Table 1). Previous head-to-head studies have shown that plasma is a preferable sample type for ctDNA analysis because the ctDNA fraction in serum is lower than that in plasma, while the background and large DNA fragments are higher in serum (Lee et al., 2020; Pittella-Silva et al., 2020). Therefore, we suggest plasma as the ideal non-invasive sample type for GC early detection, and recent studies on GC early diagnosis have also confirmed that plasma is a superior sample type (Table 1; Table 2; Table 3). However, an important issue to address is the significant variation in the volume of plasma samples used in different studies (Table 1), which can have a significant impact on the performance of DNA methylation tests and affect subsequent marker selection and replication by other researchers. Furthermore, the standardized pre-analytical procedure must be considered before the clinical applications (Figure 1) (Kerachian et al., 2021). For example, blood drawn by EDTA tubes should be processed within 4–6 h after collection if storage at room temperature (Meddeb et al., 2019), and stored at 4°C in EDTA tubes for up to 24 h (Kerachian et al., 2021). During the plasma fraction separation procedure, the brake function of the centrifuge must be turned off to prevent disruption of the cell layer (Kerachian et al., 2021), and centrifuging the blood twice is recommended (Volckmar et al., 2018). The plasma should be processed for cfDNA isolation within 24 h if stored at 4°C, and for long-term storage at −20 or −80°C (Kerachian et al., 2021).
Stool is an easily accessible and non-invasive sample type that can be conveniently collected at home (Qian, 2017), making it an ideal sample for early detection of gastrointestinal cancers. However, DNA derived from the stomach has a longer residence time in the digestive tract, making it more susceptible to degradation by nucleases and gastric acid present in the gastrointestinal tract (Olson et al., 2005; Liu et al., 2015). As a result, the proportion of DNA originating from the stomach in stool samples is relatively lower compared to fresh DNA derived from the colon. Consequently, the use of stool samples for detecting GC often exhibits significantly lower sensitivity compared to the detection of CRC (Table 3).
The most commonly used analytical method for analyzing DNA methylation is the bisulfite-treat-based method, which includes MSP, qMSP, bisulfite Sanger sequencing, bisulfite next-generation sequencing (NGS), and pyrosequencing (Figure 1) (Kurdyukov and Bullock, 2016). The entire process consists of three steps: 1) DNA isolation from specimens; 2) DNA bisulfite treatment and purification; 3) converted DNA analysis. The efficiencies of the DNA isolation kit and the DNA bisulfite treatment kit are crucial factors that affect the performance of DNA methylation analysis (Cox et al., 2022). Some studies have compared the most commonly used commercial cfDNA isolation kits and bisulfite conversion kits and have observed significant differences in cfDNA recovery efficiency and bisulfite conversion efficiency (Sorber et al., 2017; Worm Ørntoft et al., 2017). Hence, carefully selecting a suitable and highly efficient kit is necessary during DNA methylation assay development and application. Among the DNA methylation analytical methods, MSP is an economical and traditional method that has been widely used in various sample types (Ramalho-Carvalho et al., 2018), but its low resolution, low-throughput, and potential for cross-contamination limit its application in liquid biopsy (Mao and Chou, 2010). qMSP, a modified method combining MSP and qPCR, can detect several DNA methylation markers simultaneously in one tube with high resolution and avoid cross-contamination from gel analysis (Sigalotti et al., 2019). Currently, several qMSP-based non-invasive cancer early detection tests have approved by FDA and NMPA due to their cost-effectiveness and convenience (Imperiale et al., 2014; Potter et al., 2014; Wu et al., 2016; Wang et al., 2020). Bisulfite NGS as a high-throughput analytical method was used for DNA methylation markers discovery and large-panel development (Luo et al., 2020), and its wider coverage of DNA markers can avoid false positives. However, the data summarized in this review suggest that the NGS-based GC early detection panels do not offer a significant advantage compared to qMSP-based panels (Table 2; Table 3), and the high-cost and complex operation process also limit their application.
In the field of GC early detection, the current methylation markers being used are predominantly pan-cancer markers rather than GC-specific markers. For example, SEPT9 is approved by the FDA and NMPA as a plasma marker for CRC detection (Potter et al., 2014; Wu et al., 2016), while SFRP2 (Li et al., 2019), KCNQ5 (Jensen et al., 2019), C9orf50 (Jensen et al., 2019), CLIP4 (Jensen et al., 2019) and TFPI2 (Hibi et al., 2011b) were found to be positive in CRC plasma. Similarly, ZNF582 (Huang et al., 2017) and ZNF569 (Salta et al., 2020) showed high methylation in esophageal cancers, and RASSF1A has been approved by NMPA as a lung cancer early detection marker (Wei et al., 2021). Meanwhile, the above data in GC samples all came from case-control study, and have not been evaluated in the real-world asymptomatic population. While the cfDNA in blood is derived from organs throughout the body, thus the blood-based GC early detection may result in numerous “false positives” in real-world application if using the pan-cancer methylation markers. To address the issue of insufficient specificity for current methods, a pan-cancer panel may be utilized, but it also raises concerns regarding the next-step examination in case of a positive result. On the bright side, many methylation markers are more likely to exhibit positivity in the digestive system while being relatively specific in other organs. Therefore, the optimal solution for GC early detection in the future may be the target digestive system pan-cancer test.
Compared to DNA methylation in CRC early detection (Nikolaou et al., 2018; Worm Ørntoft, 2018; Nassar et al., 2021), the recent milestones of methylation-based GC early detection are still in a relatively early stage, and the majority of the GC methylation studies were focused on the Asian region, especially in China and Japan (Table 1). In the future, the researchers should pay more attention to the discovery of specific markers and improve the early-stage GC sensitivity, such as through enrichment of the short-length ctDNA in plasma (Mouliere et al., 2018). Meanwhile, the cost and the payer condition (individual or medical insurance) are two important factors that must be concerned when developing and applying the GC DNA methylation test (Figure 1). In developing countries and rural regions, a qMSP-based test may be the first choice, but in developed countries and urban areas, NGS-based methods can also be an alternative solution. Furthermore, automating pre-analytical procedures will provide more consistent and reproducible detection results and reduce costs in the future (Figure 1). Finally, multiplex DNA methylation detection based on blood sample might the optimal method for early detection of GC in the future, which can avoid individual biases by combining multiple markers and improve sensitivity for early-stage cancer detection. Therefore, we provide a potential standardized flowchart for plasma DNA methylation analysis in Figure 2 based on our experience and literature.
In conclusion, DNA methylation as a robust and sensitive marker has also been widely studied in GC samples, numerous potential DNA methylation markers have already been identified in GC, and some of them have been developed as commercial kits. But the lack of early-stage GC sensitivity and specificity should be improved in the future, and the standardized sampling, pre-analytical, cfDNA isolation and conversion procedures must be considered in development of the assay. With the rapid development of new technology and the discovery of more methylation markers, it is expected that DNA methylation will become a cost-effective and non-invasive tool for GC early detection in the near future.
Writing—original draft, YX and YD; writing—review and editing, CH; supervision, BP and ZW; approval of final manuscript, YX, CH, and YD. All authors contributed to the article and approved the submitted version.
This work was supported by Suzhou Science and Technology Development Plan Project (Grant No. SKJY2021011) and Key Technologies R & D Program for Social Development of Kunshan (Grant No. KS2228).
The authors declare that the research was conducted in the absence of any commercial or financial relationships that could be construed as a potential conflict of interest.
All claims expressed in this article are solely those of the authors and do not necessarily represent those of their affiliated organizations, or those of the publisher, the editors and the reviewers. Any product that may be evaluated in this article, or claim that may be made by its manufacturer, is not guaranteed or endorsed by the publisher.
Abbaszadegan, M. R., Moaven, O., Sima, H. R., Ghafarzadegan, K., A'rabi, A., Forghani, M. N., et al. (2008). p16 promoter hypermethylation: a useful serum marker for early detection of gastric cancer. World J. Gastroenterology 14, 2055–2060. doi:10.3748/wjg.14.2055
Abbosh, C., Birkbak, N. J., and Swanton, C. (2018). Early stage NSCLC - challenges to implementing ctDNA-based screening and MRD detection. Nat. Rev. Clin. Oncol. 15, 577–586. doi:10.1038/s41571-018-0058-3
Alarcón, M. A., Olivares, W., Córdova-Delgado, M., Muñoz-Medel, M., de Mayo, T., Carrasco-Aviño, G., et al. (2020). The reprimo-like gene is an epigenetic-mediated tumor suppressor and a candidate biomarker for the non-invasive detection of gastric cancer. Int. J. Mol. Sci. 21, 9472. doi:10.3390/ijms21249472
Anderson, B. W., Suh, Y. S., Choi, B., Lee, H. J., Yab, T. C., Taylor, W. R., et al. (2018). Detection of gastric cancer with novel methylated DNA markers: Discovery, tissue validation, and pilot testing in plasma. Clin. cancer Res. official J. Am. Assoc. Cancer Res. 24, 5724–5734. doi:10.1158/1078-0432.Ccr-17-3364
Bernal, C., Aguayo, F., Villarroel, C., Vargas, M., Díaz, I., Ossandon, F. J., et al. (2008). Reprimo as a potential biomarker for early detection in gastric cancer. Clin. cancer Res. official J. Am. Assoc. Cancer Res. 14, 6264–6269. doi:10.1158/1078-0432.Ccr-07-4522
Cai, G., Cai, M., Feng, Z., Liu, R., Liang, L., Zhou, P., et al. (2021). A multilocus blood-based assay targeting circulating tumor DNA methylation enables early detection and early relapse prediction of colorectal cancer. Gastroenterology 161, 2053–2056.e2. e2052. doi:10.1053/j.gastro.2021.08.054
Cao, C. Q., Chang, L., and Wu, Q. (2020). Circulating methylated Septin 9 and ring finger protein 180 for noninvasive diagnosis of early gastric cancer. Transl. Cancer Res. 9, 7012–7021. doi:10.21037/tcr-20-1330
Chen, L., Su, L., Li, J., Zheng, Y., Yu, B., Yu, Y., et al. (2012). Hypermethylated FAM5C and MYLK in serum as diagnosis and pre-warning markers for gastric cancer. Dis. markers 32, 195–202. doi:10.3233/dma-2011-0877
Chen, X., Lin, Z., Xue, M., Si, J., and Chen, S. (2015). Zic1 promoter hypermethylation in plasma DNA is a potential biomarker for gastric cancer and intraepithelial neoplasia. PloS one 10, e0133906. doi:10.1371/journal.pone.0133906
Chen, X., Gole, J., Gore, A., He, Q., Lu, M., Min, J., et al. (2020). Non-invasive early detection of cancer four years before conventional diagnosis using a blood test. Nat. Commun. 11, 3475. doi:10.1038/s41467-020-17316-z
Cheung, K. F., Lam, C. N., Wu, K., Ng, E., Chong, W. W. S., Cheng, A. S. L., et al. (2012). Characterization of the gene structure, functional significance, and clinical application of RNF180, a novel gene in gastric cancer. Cancer 118, 947–959. doi:10.1002/cncr.26189
Church, T. R., Wandell, M., Lofton-Day, C., Mongin, S. J., Burger, M., Payne, S. R., et al. (2014). Prospective evaluation of methylated SEPT9 in plasma for detection of asymptomatic colorectal cancer. Gut 63, 317–325. doi:10.1136/gutjnl-2012-304149
Cox, D. R., Wong, B. K. L., Lee, E., Testro, A., Muralidharan, V., Dobrovic, A., et al. (2022). Evaluating DNA recovery efficiency following bisulphite modification from plasma samples submitted for cell-free DNA methylation analysis. Epigenetics 17, 1956–1960. doi:10.1080/15592294.2022.2091821
Davalos, V., and Esteller, M. (2022). Cancer epigenetics in clinical practice. CA a cancer J. Clin. 73, 376–424. doi:10.3322/caac.21765
Du, Y. Y., Dai, D. Q., and Yang, Z. (2010). Role of RECK methylation in gastric cancer and its clinical significance. World J. gastroenterology 16, 904–908. doi:10.3748/wjg.v16.i7.904
Duffy, M. J., Diamandis, E. P., and Crown, J. (2021). Circulating tumor DNA (ctDNA) as a pan-cancer screening test: Is it finally on the horizon? Clin. Chem. Laboratory Med. 59, 1353–1361. doi:10.1515/cclm-2021-0171
Haghverdi, M. K., and Moslemi, E. (2018). Expression rate and PAX5 gene methylation in the blood of people suffering from gastric cancer. Open access Macedonian J. Med. Sci. 6, 1571–1576. doi:10.3889/oamjms.2018.269
Hamashima, C. (2020). The burden of gastric cancer. Ann. Transl. Med. 8, 734. doi:10.21037/atm.2020.03.166
Hibi, K., Goto, T., Shirahata, A., Saito, M., Kigawa, G., Nemoto, H., et al. (2011a). Detection of TFPI2 methylation in the serum of gastric cancer patients. Anticancer Res. 31, 3835–3838.
Hibi, K., Goto, T., Shirahata, A., Saito, M., Kigawa, G., Nemoto, H., et al. (2011b). Detection of TFPI2 methylation in the serum of colorectal cancer patients. Cancer Lett. 311, 96–100. doi:10.1016/j.canlet.2011.07.006
Hideura, E., Suehiro, Y., Nishikawa, J., Shuto, T., Fujimura, H., Ito, S., et al. (2020). Blood free-circulating DNA testing of methylated RUNX3 is useful for diagnosing early gastric cancer. Cancers 12, 789. doi:10.3390/cancers12040789
Hou, P., Ji, M. J., Shen, J. Y., and Lu, Z. H. (2005). Detection of p16 hypermethylation in gastric carcinomas using a seminested methylation-specific PCR. Biochem. Genet. 43, 1–9. doi:10.1007/s10528-005-1062-8
Hu, S. L., Kong, X. Y., Cheng, Z. D., Sun, Y. B., Shen, G., Xu, W. P., et al. (2010). Promoter methylation of p16, Runx3, DAPK and CHFR genes is frequent in gastric carcinoma. Tumori 96, 726–733. doi:10.1177/030089161009600515
Huang, J., Wang, G., Tang, J., Zhuang, W., Wang, L. P., Liou, Y. L., et al. (2017). DNA methylation status of PAX1 and ZNF582 in esophageal squamous cell carcinoma. Int. J. Environ. Res. public health 14, 216. doi:10.3390/ijerph14020216
Ichikawa, D., Koike, H., Ikoma, H., Ikoma, D., Tani, N., Otsuji, E., et al. (2004). Detection of aberrant methylation as a tumor marker in serum of patients with gastric cancer. Anticancer Res. 24, 2477–2481.
Imperiale, T. F., Ransohoff, D. F., Itzkowitz, S. H., Levin, T. R., Lavin, P., Lidgard, G. P., et al. (2014). Multitarget stool DNA testing for colorectal-cancer screening. N. Engl. J. Med. 370, 1287–1297. doi:10.1056/NEJMoa1311194
Jamshidi, A., Liu, M. C., Klein, E. A., Venn, O., Hubbell, E., Beausang, J. F., et al. (2022). Evaluation of cell-free DNA approaches for multi-cancer early detection. Cancer Cell. 40, 1537–1549.e12. e1512. doi:10.1016/j.ccell.2022.10.022
Jensen, S., Øgaard, N., Ørntoft, M. W., Rasmussen, M. H., Bramsen, J. B., Kristensen, H., et al. (2019). Novel DNA methylation biomarkers show high sensitivity and specificity for blood-based detection of colorectal cancer-a clinical biomarker discovery and validation study. Clin. epigenetics 11, 158. doi:10.1186/s13148-019-0757-3
Kandimalla, R., Xu, J., Link, A., Matsuyama, T., Yamamura, K., Parker, M. I., et al. (2021). EpiPanGI dx: A cell-free DNA methylation fingerprint for the early detection of gastrointestinal cancers. Clin. cancer Res. official J. Am. Assoc. Cancer Res. 27, 6135–6144. doi:10.1158/1078-0432.Ccr-21-1982
Kanyama, Y., Hibi, K., Nakayama, H., Kodera, Y., Ito, K., Akiyama, S., et al. (2003). Detection of p16 promoter hypermethylation in serum of gastric cancer patients. Cancer Sci. 94, 418–420. doi:10.1111/j.1349-7006.2003.tb01457.x
Kerachian, M. A., Azghandi, M., Mozaffari-Jovin, S., and Thierry, A. R. (2021). Guidelines for pre-analytical conditions for assessing the methylation of circulating cell-free DNA. Clin. epigenetics 13, 193. doi:10.1186/s13148-021-01182-7
Klein, E. A., Richards, D., Cohn, A., Tummala, M., Lapham, R., Cosgrove, D., et al. (2021). Clinical validation of a targeted methylation-based multi-cancer early detection test using an independent validation set. Ann. Oncol. official J. Eur. Soc. Med. Oncol. 32, 1167–1177. doi:10.1016/j.annonc.2021.05.806
Koike, H., Ichikawa, D., Ikoma, H., Otsuji, E., Kitamura, K., and Yamagishi, H. (2004). Comparison of methylation-specific polymerase chain reaction (MSP) with reverse transcriptase-polymerase chain reaction (RT-PCR) in peripheral blood of gastric cancer patients. J. Surg. Oncol. 87, 182–186. doi:10.1002/jso.20106
Kurdyukov, S., and Bullock, M. (2016). DNA methylation analysis: Choosing the right method. Biology 5, 3. doi:10.3390/biology5010003
Lee, T. L., Leung, W. K., Chan, M. W., Ng, E., Tong, J. H. M., Lo, K. W., et al. (2002). Detection of gene promoter hypermethylation in the tumor and serum of patients with gastric carcinoma. Clin. cancer Res. official J. Am. Assoc. Cancer Res. 8, 1761–1766.
Lee, J. S., Kim, M., Seong, M. W., Kim, H. S., Lee, Y. K., and Kang, H. J. (2020). Plasma vs. serum in circulating tumor DNA measurement: Characterization by DNA fragment sizing and digital droplet polymerase chain reaction. Clin. Chem. laboratory Med. 58, 527–532. doi:10.1515/cclm-2019-0896
Li, W. H., Zhou, Z. J., Huang, T. H., Guo, K., Chen, W., Wang, Y., et al. (2016). Detection of OSR2, VAV3, and PPFIA3 methylation in the serum of patients with gastric cancer. Dis. markers 2016, 5780538. doi:10.1155/2016/5780538
Li, H., Wang, Z., Zhao, G., Ma, Y., Chen, Y., Xue, Q., et al. (2019). Performance of a MethyLight assay for methylated SFRP2 DNA detection in colorectal cancer tissue and serum. Int. J. Biol. markers 34, 54–59. doi:10.1177/1724600818820536
Li, H., Zhao, G., Guo, Y., Fang, Y., Wang, K., Ma, Y., et al. (2022). Feasibility and reproducibility of a plasma-based multiplex DNA methylation assay for early detection of gastric cancer. Pathology, Res. Pract. 238, 154086. doi:10.1016/j.prp.2022.154086
Lin, Z., Luo, M., Chen, X., He, X., Qian, Y., Lai, S., et al. (2017). Combined detection of plasma ZIC1, HOXD10 and RUNX3 methylation is a promising strategy for early detection of gastric cancer and precancerous lesions. J. Cancer 8, 1038–1044. doi:10.7150/jca.18169
Lin, W. H., Xiao, J., Ye, Z. Y., Wei, D. L., Zhai, X. H., Xu, R. H., et al. (2021). Circulating tumor DNA methylation marker MYO1-G for diagnosis and monitoring of colorectal cancer. Clin. epigenetics 13, 232. doi:10.1186/s13148-021-01216-0
Liu, L., and Yang, X. (2015). Implication of Reprimo and hMLH1 gene methylation in early diagnosis of gastric carcinoma. Int. J. Clin. Exp. pathology 8, 14977–14982.
Liu, J. W., Kim, M. S., Nagpal, J., Yamashita, K., Poeta, L., Chang, X., et al. (2007). Quantitative hypermethylation of NMDAR2B in human gastric cancer. Int. J. cancer 121, 1994–2000. doi:10.1002/ijc.22934
Liu, Y., Zhang, Y., Dong, P., An, R., Xue, C., Ge, Y., et al. (2015). Digestion of nucleic acids starts in the stomach. Sci. Rep. 5, 11936. doi:10.1038/srep11936
Liu, Y., Zhao, G., Miao, J., Li, H., Ma, Y., Liu, X., et al. (2020). Performance comparison between plasma and stool methylated SEPT9 tests for detecting colorectal cancer. Front. Genet. 11, 324. doi:10.3389/fgene.2020.00324
Locke, W. J., Guanzon, D., Ma, C., Liew, Y. J., Duesing, K. R., Fung, K. Y. C., et al. (2019). DNA methylation cancer biomarkers: Translation to the clinic. Front. Genet. 10, 1150. doi:10.3389/fgene.2019.01150
Luo, H., Zhao, Q., Wei, W., Zheng, L., Yi, S., Li, G., et al. (2020). Circulating tumor DNA methylation profiles enable early diagnosis, prognosis prediction, and screening for colorectal cancer. Sci. Transl. Med. 12, eaax7533. doi:10.1126/scitranslmed.aax7533
Ma, Y., Zhao, G., Wang, K., Song, L., Xiong, S., Li, H., et al. (2022a). Clinical performance of a liquid biopsy test based on the detection of multiple DNA methylation biomarkers for early detection of gastrointestinal cancers. J. Clin. Oncol. 40, e16096. doi:10.1200/JCO.2022.40.16_suppl.e16096
Ma, L., Gong, J., Zhao, M., Kong, X., Gao, P., Jiang, Y., et al. (2022b). A novel stool methylation test for the non-invasive screening of gastric and colorectal cancer. Front. Oncol. 12, 860701. doi:10.3389/fonc.2022.860701
Maeda, M., Yamashita, S., Shimazu, T., Iida, N., Takeshima, H., Nakajima, T., et al. (2018). Novel epigenetic markers for gastric cancer risk stratification in individuals after Helicobacter pylori eradication. Gastric cancer official J. Int. Gastric Cancer Assoc. Jpn. Gastric Cancer Assoc. 21, 745–755. doi:10.1007/s10120-018-0803-4
Mao, R., and Chou, L. S. (2010). Methylation analysis by restriction endonuclease digestion and real-time PCR. Clin. Chem. 56, 1050–1052. doi:10.1373/clinchem.2010.146654
Meddeb, R., Pisareva, E., and Thierry, A. R. (2019). Guidelines for the preanalytical conditions for analyzing circulating cell-free DNA. Clin. Chem. 65, 623–633. doi:10.1373/clinchem.2018.298323
Miao, J., Liu, Y., Zhao, G., Liu, X., Ma, Y., Li, H., et al. (2020). Feasibility of plasma-methylated SFRP2 for early detection of gastric cancer. Cancer control J. Moffitt Cancer Cent. 27, 559. doi:10.1177/1073274820922559
Mouliere, F., Chandrananda, D., Piskorz, A. M., Moore, E. K., Morris, J., Ahlborn, L. B., et al. (2018). Enhanced detection of circulating tumor DNA by fragment size analysis. Sci. Transl. Med. 10, eaat4921. doi:10.1126/scitranslmed.aat4921
Muretto, P., Ruzzo, A., Pizzagalli, F., Graziano, F., Maltese, P., Zingaretti, C., et al. (2008). Endogastric capsule for E-cadherin gene (CDH1) promoter hypermethylation assessment in DNA from gastric juice of diffuse gastric cancer patients. Ann. Oncol. official J. Eur. Soc. Med. Oncol. 19, 516–519. doi:10.1093/annonc/mdm493
Nagasaka, T., Tanaka, N., Cullings, H. M., Sun, D. S., Sasamoto, H., Uchida, T., et al. (2009). Analysis of fecal DNA methylation to detect gastrointestinal neoplasia. J. Natl. Cancer Inst. 101, 1244–1258. doi:10.1093/jnci/djp265
Nassar, F. J., Msheik, Z. S., Nasr, R. R., and Temraz, S. N. (2021). Methylated circulating tumor DNA as a biomarker for colorectal cancer diagnosis, prognosis, and prediction. Clin. epigenetics 13, 111. doi:10.1186/s13148-021-01095-5
Nikolaou, S., Qiu, S., Fiorentino, F., Rasheed, S., Tekkis, P., and Kontovounisios, C. (2018). Systematic review of blood diagnostic markers in colorectal cancer. Tech. coloproctology 22, 481–498. doi:10.1007/s10151-018-1820-3
Ning, F. L., Lyu, J., Pei, J. P., Gu, W. J., Zhang, N. N., Cao, S. Y., et al. (2022). The burden and trend of gastric cancer and possible risk factors in five Asian countries from 1990 to 2019. Sci. Rep. 12, 5980. doi:10.1038/s41598-022-10014-4
Oishi, Y., Watanabe, Y., Yoshida, Y., Sato, Y., Hiraishi, T., Oikawa, R., et al. (2012). Hypermethylation of Sox17 gene is useful as a molecular diagnostic application in early gastric cancer. Tumour Biol. J. Int. Soc. Oncodevelopmental Biol. Med. 33, 383–393. doi:10.1007/s13277-011-0278-y
Olson, J., Whitney, D. H., Durkee, K., and Shuber, A. P. (2005). DNA stabilization is critical for maximizing performance of fecal DNA-based colorectal cancer tests. Diagnostic Mol. pathology Am. J. Surg. pathology, part B 14, 183–191. doi:10.1097/01.pas.0000176768.18423.7e
Patel, T. N., Roy, S., and Ravi, R. (2017). Gastric cancer and related epigenetic alterations. Ecancermedicalscience 11, 714. doi:10.3332/ecancer.2017.714
Peng, C., Zhao, G., Pei, B., Wang, K., Li, H., Fei, S., et al. (2022). A novel plasma-based methylation panel for upper gastrointestinal cancer early detection. Cancers 14, 5282. doi:10.3390/cancers14215282
Pimson, C., Ekalaksananan, T., Pientong, C., Promthet, S., Putthanachote, N., Suwanrungruang, K., et al. (2016). Aberrant methylation of PCDH10 and RASSF1A genes in blood samples for non-invasive diagnosis and prognostic assessment of gastric cancer. PeerJ 4, e2112. doi:10.7717/peerj.2112
Pittella-Silva, F., Chin, Y. M., Chan, H. T., Nagayama, S., Miyauchi, E., Low, S. K., et al. (2020). Plasma or serum: Which is preferable for mutation detection in liquid biopsy? Clin. Chem. 66, 946–957. doi:10.1093/clinchem/hvaa103
Potter, N. T., Hurban, P., White, M. N., Whitlock, K. D., Lofton-Day, C. E., Tetzner, R., et al. (2014). Validation of a real-time PCR-based qualitative assay for the detection of methylated SEPT9 DNA in human plasma. Clin. Chem. 60, 1183–1191. doi:10.1373/clinchem.2013.221044
Qian, C. N. (2017). At-home cancer screening: A solution for China and other developing countries with a large population and limited number of healthcare practitioners. Chin. J. cancer 36, 68. doi:10.1186/s40880-017-0235-2
Rahat, B., Ali, T., Sapehia, D., Mahajan, A., and Kaur, J. (2020). Circulating cell-free nucleic acids as epigenetic biomarkers in precision medicine. Front. Genet. 11, 844. doi:10.3389/fgene.2020.00844
Ramalho-Carvalho, J., Henrique, R., and Jerónimo, C. (2018). “Methylation-specific PCR,” in DNA methylation protocols. Editor J. Tost (New York, NY: Springer New York), 447–472.
Ren, J., Lu, P., Zhou, X., Liao, Y., Liu, X., Li, J., et al. (2022). Genome-scale methylation analysis of circulating cell-free DNA in gastric cancer patients. Clin. Chem. 68, 354–364. doi:10.1093/clinchem/hvab204
Ruan, W., Lei, X., Sha, W., Li, B., Wang, H., Xia, Y., et al. (2023). A multiplex DNA methylation assay for noninvasive early detection of gastric cancer. J. Clin. Oncol. 41, 300. doi:10.1200/JCO.2023.41.4_suppl.300
Sakakura, C., Hamada, T., Miyagawa, K., Nishio, M., Miyashita, A., Nagata, H., et al. (2009). Quantitative analysis of tumor-derived methylated RUNX3 sequences in the serum of gastric cancer patients. Anticancer Res. 29, 2619–2625.
Saliminejad, K., Soleymani Fard, S., Khorram Khorshid, H. R., Yaghmaie, M., Mahmoodzadeh, H., Mousavi, S. A., et al. (2020). Methylation analysis of P16, RASSF1A, RPRM, and RUNX3 in circulating cell-free DNA for detection of gastric cancer: A validation study. Avicenna J. Med. Biotechnol. 12, 99–106.
Salta, S., Macedo-Silva, C., Miranda-Gonçalves, V., Lopes, N., Gigliano, D., Guimarães, R., et al. (2020). A DNA methylation-based test for esophageal cancer detection. Biomark. Res. 8, 68. doi:10.1186/s40364-020-00248-7
Sekiguchi, M., Oda, I., Matsuda, T., and Saito, Y. (2022). Epidemiological trends and future perspectives of gastric cancer in eastern asia. Digestion 103, 22–28. doi:10.1159/000518483
Shin, D. G., Kim, K., Choi, S., Joo, H. J., Baik, S. H., and Park, M. (2016). A methylation profile of circulating cell free DNA for the early detection of gastric cancer and the effects after surgical resection. J. Clin. Exp. Oncol. 5. doi:10.4172/2324-9110.1000151
Shirahata, A., Sakuraba, K., Kitamura, Y., Yokomizo, K., Gotou, T., Saitou, M., et al. (2012). Detection of vimentin methylation in the serum of patients with gastric cancer. Anticancer Res. 32, 791–794.
Sigalotti, L., Covre, A., Colizzi, F., and Fratta, E. (2019). Quantitative methylation-specific PCR: A simple method for studying epigenetic modifications of cell-free DNA. Methods Mol. Biol. Clift. NJ) 1909, 137–162. doi:10.1007/978-1-4939-8973-7_11
Song, Y., Liu, X., Cheng, W., Li, H., and Zhang, D. (2022). The global, regional and national burden of stomach cancer and its attributable risk factors from 1990 to 2019. Sci. Rep. 12, 11542. doi:10.1038/s41598-022-15839-7
Sorber, L., Zwaenepoel, K., Deschoolmeester, V., Roeyen, G., Lardon, F., Rolfo, C., et al. (2017). A comparison of cell-free DNA isolation kits: Isolation and quantification of cell-free DNA in plasma. J. Mol. diagnostics JMD 19, 162–168. doi:10.1016/j.jmoldx.2016.09.009
Sung, H., Ferlay, J., Siegel, R. L., Laversanne, M., Soerjomataram, I., Jemal, A., et al. (2021). Global cancer statistics 2020: GLOBOCAN estimates of incidence and mortality worldwide for 36 cancers in 185 countries. CA a cancer J. Clin. 71, 209–249. doi:10.3322/caac.21660
Vedeld, H. M., Andresen, K., Eilertsen, I. A., Nesbakken, A., Seruca, R., Gladhaug, I. P., et al. (2015). The novel colorectal cancer biomarkers CDO1, ZSCAN18 and ZNF331 are frequently methylated across gastrointestinal cancers. Int. J. cancer 136, 844–853. doi:10.1002/ijc.29039
Volckmar, A. L., Sültmann, H., Riediger, A., Fioretos, T., Schirmacher, P., Endris, V., et al. (2018). A field guide for cancer diagnostics using cell-free DNA: From principles to practice and clinical applications. Genes., chromosomes cancer 57, 123–139. doi:10.1002/gcc.22517
Wang, H., Zheng, Y., Lai, J., Luo, Q., Ke, H., and Chen, Q. (2016). Methylation-sensitive melt curve analysis of the Reprimo gene methylation in gastric cancer. PloS one 11, e0168635. doi:10.1371/journal.pone.0168635
Wang, J., Liu, S., Wang, H., Zheng, L., Zhou, C., Li, G., et al. (2020). Robust performance of a novel stool DNA test of methylated SDC2 for colorectal cancer detection: A multicenter clinical study. Clin. epigenetics 12, 162. doi:10.1186/s13148-020-00954-x
Watanabe, Y., Kim, H. S., Castoro, R. J., Chung, W., Estecio, M. R. H., Kondo, K., et al. (2009). Sensitive and specific detection of early gastric cancer with DNA methylation analysis of gastric washes. Gastroenterology 136, 2149–2158. doi:10.1053/j.gastro.2009.02.085
Wei, K. L., Chou, J. L., Chen, Y. C., Jin, H., Chuang, Y. M., Wu, C. S., et al. (2019). Methylomics analysis identifies a putative STAT3 target, SPG20, as a noninvasive epigenetic biomarker for early detection of gastric cancer. PloS one 14, e0218338. doi:10.1371/journal.pone.0218338
Wei, B., Wu, F., Xing, W., Sun, H., Yan, C., Zhao, C., et al. (2021). A panel of DNA methylation biomarkers for detection and improving diagnostic efficiency of lung cancer. Sci. Rep. 11, 16782. doi:10.1038/s41598-021-96242-6
Wolf, A. M. D., Fontham, E. T. H., Church, T. R., Flowers, C. R., Guerra, C. E., LaMonte, S. J., et al. (2018). Colorectal cancer screening for average-risk adults: 2018 guideline update from the American cancer society. CA a cancer J. Clin. 68, 250–281. doi:10.3322/caac.21457
Worm Ørntoft, M. B., Jensen, S., Hansen, T. B., Bramsen, J. B., and Andersen, C. L. (2017). Comparative analysis of 12 different kits for bisulfite conversion of circulating cell-free DNA. Epigenetics 12, 626–636. doi:10.1080/15592294.2017.1334024
Worm Ørntoft, M. B. (2018). Review of blood-based colorectal cancer screening: How far are circulating cell-free DNA methylation markers from clinical implementation? Clin. colorectal cancer 17, e415–e433. doi:10.1016/j.clcc.2018.02.012
Wu, D., Zhou, G., Jin, P., Zhu, J., Li, S., Wu, Q., et al. (2016). Detection of colorectal cancer using a simplified SEPT9 gene methylation assay is a reliable method for opportunistic screening. J. Mol. diagnostics JMD 18, 535–545. doi:10.1016/j.jmoldx.2016.02.005
Xu, Y., Zhang, P., Zhang, K., and Huang, C. (2021a). The application of CA72-4 in the diagnosis, prognosis, and treatment of gastric cancer. Biochimica biophysica acta Rev. cancer 1876, 188634. doi:10.1016/j.bbcan.2021.188634
Xu, J., Song, J., Wang, T., Zhu, W., Zuo, L., Wu, J., et al. (2021b). A combination of methylation and protein markers is capable of detecting gastric cancer detection by combined markers. Epigenomics 13, 1557–1570. doi:10.2217/epi-2021-0080
Yamaguchi, Y., Nagata, Y., Hiratsuka, R., Kawase, Y., Tominaga, T., Takeuchi, S., et al. (2016). Gastric cancer screening by combined assay for serum anti-Helicobacter pylori IgG antibody and serum pepsinogen levels-the ABC method. Digestion 93, 13–18. doi:10.1159/000441742
Yamamoto, H., Watanabe, Y., Oikawa, R., Morita, R., Yoshida, Y., Maehata, T., et al. (2016). BARHL2 methylation using gastric wash DNA or gastric juice exosomal DNA is a useful marker for early detection of gastric cancer in an H. Pylori-independent manner. Clin. Transl. gastroenterology 7, e184. doi:10.1038/ctg.2016.40
Yang, Q., Gao, J., Xu, L., Zeng, Z., Sung, J. J. Y., and Yu, J. (2013). Promoter hypermethylation of BCL6B gene is a potential plasma DNA biomarker for gastric cancer. Biomarkers Biochem. Indic. Expo. response susceptibility Chem. 18, 721–725. doi:10.3109/1354750x.2013.853839
Yang, X., He, D., Xiong, Z., Yan, B., Li, Q., Guo, D., et al. (2023). Early detection and localization of major gastrointestinal cancers by combining DNA methylation and fragmentomic signatures generated from a single cfDNA targeted sequencing assay. J. Clin. Oncol. 41, 330. doi:10.1200/JCO.2023.41.4_suppl.330
Young, G. P., Pedersen, S. K., Mansfield, S., Murray, D. H., Baker, R. T., Rabbitt, P., et al. (2016). A cross-sectional study comparing a blood test for methylated BCAT1 and IKZF1 tumor-derived DNA with CEA for detection of recurrent colorectal cancer. Cancer Med. 5, 2763–2772. doi:10.1002/cam4.868
Zhang, X., Zhang, X., Sun, B., Lu, H., Wang, D., Yuan, X., et al. (2014). Detection of aberrant promoter methylation of RNF180, DAPK1 and SFRP2 in plasma DNA of patients with gastric cancer. Oncol. Lett. 8, 1745–1750. doi:10.3892/ol.2014.2410
Zhang, C., Yu, W., Wang, L., Zhao, M., Guo, Q., Lv, S., et al. (2017). DNA methylation analysis of the SHOX2 and RASSF1A panel in bronchoalveolar lavage fluid for lung cancer diagnosis. J. Cancer 8, 3585–3591. doi:10.7150/jca.21368
Zhao, G., Li, H., Yang, Z., Wang, Z., Xu, M., Xiong, S., et al. (2019). Multiplex methylated DNA testing in plasma with high sensitivity and specificity for colorectal cancer screening. Cancer Med. 8, 5619–5628. doi:10.1002/cam4.2475
Zhao, G., Ma, Y., Li, H., Li, S., Zhu, Y., Liu, X., et al. (2020). A novel plasma based early colorectal cancer screening assay base on methylated SDC2 and SFRP2. Clin. chimica acta; Int. J. Clin. Chem. 503, 84–89. doi:10.1016/j.cca.2020.01.010
Zhao, L., Liu, Y., Zhang, S., and Li, M. (2022a). Plasma methylated RNF180 for noninvasive diagnosis of gastric cancer. BioMed Res. Int. 2022, 6548945. doi:10.1155/2022/6548945
Zhao, L., Li, M., Zhang, S., and Liu, Y. (2022b). Plasma-methylated SEPT9 for the noninvasive diagnosis of gastric cancer. J. Clin. Med. 11, 6399. doi:10.3390/jcm11216399
Keywords: sample types, analytical methods, non-invasive, gastric cancer, DNA methylation
Citation: Xue Y, Huang C, Pei B, Wang Z and Dai Y (2023) An overview of DNA methylation markers for early detection of gastric cancer: current status, challenges, and prospects. Front. Genet. 14:1234645. doi: 10.3389/fgene.2023.1234645
Received: 05 June 2023; Accepted: 17 July 2023;
Published: 25 July 2023.
Edited by:
Wenjun Lan, Cold Spring Harbor Laboratory, United StatesReviewed by:
Rui Sun, Nantong University, ChinaCopyright © 2023 Xue, Huang, Pei, Wang and Dai. This is an open-access article distributed under the terms of the Creative Commons Attribution License (CC BY). The use, distribution or reproduction in other forums is permitted, provided the original author(s) and the copyright owner(s) are credited and that the original publication in this journal is cited, in accordance with accepted academic practice. No use, distribution or reproduction is permitted which does not comply with these terms.
*Correspondence: Ying Xue, eHVleWluZzAzMDRAMTI2LmNvbQ==; Yanmiao Dai, ODVfZHltQDE2My5jb20=
†These authors have contributed equally to this work
Disclaimer: All claims expressed in this article are solely those of the authors and do not necessarily represent those of their affiliated organizations, or those of the publisher, the editors and the reviewers. Any product that may be evaluated in this article or claim that may be made by its manufacturer is not guaranteed or endorsed by the publisher.
Research integrity at Frontiers
Learn more about the work of our research integrity team to safeguard the quality of each article we publish.