- 1National Key Laboratory for Swine Genetic Improvement and Production Technology, Ministry of Science and Technology of China, Jiangxi Agricultural University, Nanchang, China
Introduction: Muscle glycolytic potential (GP) is a key factor affecting multiple meat quality traits. It is calculated based on the contents of residual glycogen and glucose (RG), glucose-6-phosphate (G6P), and lactate (LAT) contents in muscle. However, the genetic mechanism of glycolytic metabolism in skeletal muscle of pigs remains poorly understood. With a history of more than 400 years and some unique characteristics, the Erhualian pig is called the “giant panda” (very precious) in the world’s pig species by Chinese animal husbandry.
Methods: Here, we performed a genome-wide association study (GWAS) using 1.4M single nucleotide polymorphisms (SNPs) chips for longissimus RG, G6P, LAT, and GP levels in 301 purebred Erhualian pigs.
Results: We found that the average GP value of Erhualian was unusually low (68.09 μmol/g), but the variation was large (10.4–112.7 μmol/g). The SNP-based heritability estimates for the four traits ranged from 0.16–0.32. In total, our GWAS revealed 31 quantitative trait loci (QTLs), including eight for RG, nine for G6P, nine for LAT, five for GP. Of these loci, eight were genome-wide significant (p < 3.8 × 10−7), and six loci were common to two or three traits. Multiple promising candidate genes such as FTO, MINPP1, RIPOR2, SCL8A3, LIFR and SRGAP1 were identified. The genotype combinations of the five GP-associated SNPs also showed significant effect on other meat quality traits.
Discussion: These results not only provide insights into the genetic architecture of GP related traits in Erhualian, but also are useful for pig breeding programs involving this breed.
Introduction
Meat quality significantly influences consumers’ preference and purchasing behavior, thus improving meat quality has been being a major goal of pig breeding (Khanal et al., 2019; Zhang et al., 2019). In recent decades, the meat quality of commercial lean pigs has been improved to a certain extent by removing some known unfavorable alleles for meat quality such as PRKAG3 (200Q) (Milan et al., 2000) and RYR1 (615C) alleles (Fujii et al., 1991); however, their meat quality is still cannot meet the demands of discerning consumers (Bonneau and Lebret, 2010). A prominent problem of meat quality of commercial pigs is the high incidence (10%–30%) of inferior meat, including pale, soft, exudative (PSE) meat and dark, firm, dry (DFD) meat, which causes huge economic losses to pig industry and pork processing industry (Lewis et al., 1987; Van der Wal et al., 1988; Shen et al., 2006; Gajana et al., 2013; Gonzalez-Rivas et al., 2020). In contrast, PSE and DFD meat are rarely found in Chinese native pigs, making them valuable genetic resources for cultivating high-quality pig synthetic lines (Chen et al., 2013; Ma et al., 2013; Liu et al., 2015; Wu et al., 2020).
The degree of post-mortem pH decline in porcine skeletal muscle is an important factor accounting for the occurrence of inferior meat, as it significantly affects meat color development, water-holding capacity and tenderness (Bee et al., 2007; Scheffler and Gerrard, 2007; Popp et al., 2015). The ultimate pH value of PSE and DFD meat is usually lower than 5.5 and greater than 6.0, respectively. It is well known that muscle pH value and other pork quality attributes depend on the content of glycogen of muscle at slaughter, as well as the rate and extent of lactate accumulation during 24 h post-slaughter glycolysis metabolism (Sayre et al., 1963; Hamilton et al., 2003). Based on that, muscle glycolytic potential (GP), defined as the potential of lactate production during post-mortem glycolysis, has been proposed as a predictor for the extent of pH decline (Maribo et al., 1999; Scheffler and Gerrard, 2007).
To date, two major genes have been identified to strongly influence GP phenotype and other meat quality traits in commercial pigs. PRKAG3 is the first causal gene proved to affect GP level, and its R200Q mutation significantly increases muscle glycogen content by up to ∼70%, consequently causing acidic meat in Hampshire pigs (Milan et al., 2000). In addition, it was found in Duroc and its hybrid pigs that a splice mutation (g.8283C > A) in the PHKG1 gene caused a 43% increase in GP and a 20% decrease in drip loss of pork (Ma et al., 2014). These two gene mutations with large effects on GP-related traits are all derived from European commercial pig breeds but are rarely present in Chinese native pigs. However, few studies have systematically investigated the genetic architecture of GP-related traits in Chinese native pigs.
The Chinese Erhualian pig is famous for its high prolificacy (Li et al., 2017). Its meat quality is also good and superior to European commercial pig breeds in color, pH value, water-holding capacity, marbling, intramuscular fat, tenderness, muscle fiber diameter, taste and flavor (Chen et al., 2013). Intriguingly, our recent study demonstrated that compared with Chinese Bama Xiang and Laiwu pigs, Erhualian pigs had a higher muscle ultimate pH value (pHu), a smaller extent of pH decline (pHd) from post-mortem 45 min to 24 h, and a greater variation in the two pH index (Huang et al., 2020). The variation in muscle pH value of Erhualian may be at least partially due to the variation in GP level or the concentrations of its components, including residual glycogen and glucose (RG), glucose-6-phosphate (G6P) and lactate (LAT). To verify this speculation, we herein first assessed the variation of GP-related traits in 301 Erhualian pigs, and then evaluated the impact of GP on pH values. Furthermore, to reveal the genetic architecture of the GP phenotypes of Erhualian pigs, we performed a genome-wide association study (GWAS) using high-density 1.4M single nucleotide polymorphisms (SNPs) chip data from the population.
Materials and methods
Animals and sampling
The Erhualian population was established as described previously (Liu et al., 2015). Briefly, the population consists of 160 barrows (castrated males) and 141 gilts, which were produced from 11 boars and 51 dams. These piglets were all born in Jiaoxi and transferred to Nanchang when they were 2–3 months old. Then they were raised under uniform conditions and slaughtered in 11 batches in the same commercial abattoir when they were about 300 days old. At post-mortem 30 min, 2–3 g muscle samples were collected from the longissimus thoracis (LT) and then stored at −80°C until assay.
Phenotype measurement and correlation analysis
The contents of residual glycogen and glucose (RG), glucose-6-phosphate (G6P) and lactate (LAT) in LT muscle were determined using the glycogen assay kits (E2GN-100) BioAssay Systems, the glucose-6-phosphate assay kits (EG6P-100) BioAssay Systems and the lactic acid assay kits (A019-2) from the Nanjing Jiancheng Bioengineering Institute, respectively. GP were calculated as the sum of: 2 × (RG + G6P) + Lactate (Monin and Sellier, 1985) and expressed as μmol of lactic acid equivalent per g of fresh muscle. In addition, pH values of LT muscle was measured twice on each sample at 45 min (pHi) and 24 h (pHu) after slaughter using a Delta 320 pH meter, and their mean values were calculated separately. The difference between pHi and pHu was taken as pH decline (pHd). Drip loss was assayed using an EZ-Drip Loss method. Three color parameters L*, a* and b* on the surface cuts of LT were objectively evaluated with a CM-2600d/2500d Minolta Chroma meter (Liu et al., 2015). The correlations between the GP related traits and the pH traits were evaluated using Pearson correlation analysis.
Genotyping and quality control
Genomic DNA was extracted from pig ear tissue using standard phenol/chloroform extraction. In order to meet the genotyping requirements, all DNA samples were standardized to a final concentration of 50 ng/μL and quality controlled. Totally, 301 Erhualian pigs were genotyped using the 1.4M SNP Beadchips, which is a customized Affymetrix Axiom chip. The SNPs on the chip were found from whole genome sequence data of 150 Chinese indigenous pigs and 38 International commercial pigs, and were evenly distributed across the pig genome (Gong et al., 2019). PLINK v1.9 was used for quality control of the SNP chip data (Purcell et al., 2007). SNPs with a call rate ≥ 0.90 or a minor allele frequency (MAF) ≥ 0.05, and samples with a call rate greater than 95%, were retained. Consequently, 732,609 SNPs and all individuals were kept for GWAS analysis.
Genome-wide association analyses for GP related traits
We used linear regression models to adjust GP related traits for sex and slaughter batch, and then applied Genome-wide Efficient Mixed-Model Association (GEMMA) method for genetic association analyses and SNP-based heritability estimation, which were conducted using the “−lmm 1” and “−gk 1” commands of GEMMA software (Zhou and Stephens, 2012). GEMMA examined the associations of SNPs with phenotypic values under the following linear mixed model:
where Y is a vector of residual phenotypic values that were corrected for fixed effects (sex and slaughter batch) using lm function in R program. S is the incidence vector for a, and a is the additive genetic effect of the SNP under test; u is a vector of random polygenic effects that is assumed to follow a multivariate normal distribution MVN(0, G
Bonferroni correction method was used to set the genome-wide significant (0.05/N) and suggestive (1/N) association thresholds, where N is the number of independent association tests or SNPs (Lander and Kruglyak, 1995; Yang et al., 2005). Considering that SNP clusters in high linkage disequilibrium (LD) may cause overestimate of N and significance thresholds, we first pruned the full SNP set (732,609 SNPs that passed quality control) to 130,404 independent SNPs (r2 < 0.3) by the command “indep-pairwise 50 5 0.3” in PLINK v1.9. Therefore, a SNP was considered to be genome-wide significance at p < 3.8 × 10−7 (0.05/130,404), and to be suggestive significance at p < 7.6 × 10−6 (1/130,404). The impact of population stratification was estimated by the quantile-quantile (Q-Q) plot and genotype data PCA analysis (Pearson and Manolio, 2008). The phenotypic variances explained by the significant SNPs were estimated by (Vreduce - Vfull)/Vreduce, where Vfull and Vreduce are residual variances of models for association analysis with and without the SNP term, respectively. Haplotype block or LD analysis was performed for the chromosomal regions with multiple significant SNPs clustered around the peak SNP. Haplotype blocks were identified using the HAPLOVIEW v4.2 software with default settings (Barrett et al., 2005).
Results
Descriptive and correlation statistics of GP related traits
The descriptive statistical results of four GP-related traits (RG, G6P, LAT and GP) in the longissimus muscle of 301 Erhualian pigs were given in Table 1. Our data showed that the average value of GP in Erhualian was 68.09 μmol/g (Table 1). The coefficient of variation of GP related traits was relatively large (0.30–0.55). Moreover, these traits had low to moderate heritability (0.16–0.32).
Correlation analysis results showed that the contents of RG, G6P and LAT were not only positively correlated with each other, but also had a strong and positive correlation with the GP content (r ≥ 0.61, p < 0.001; Figure 1). Since changes in muscle glucose metabolism may lead to changes in its pH values, we estimated the correlations between the four GP-related traits and pH index. As expected, our data indicates that higher RG and GP contents tended to cause a greater post-mortem pH decline (pHd) and a lower ultimate pH (pHu) value (r ≤ −0.79, p < 0.001; Figure 1), which is consistent with other studies (Hamilton et al., 2003; Luo et al., 2017).
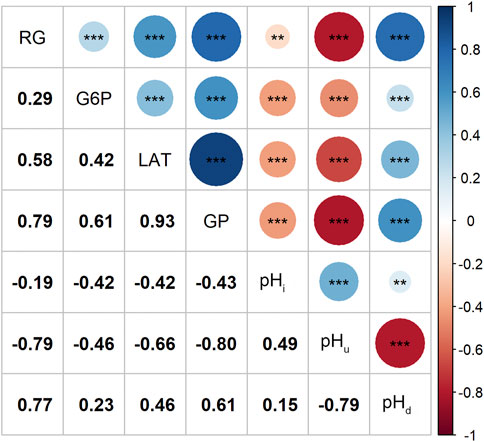
FIGURE 1. Magnitudes of correlations between the four GP related traits and the 3 pH related traits, **: p < 0.01, ***: p < 0.001.
Summary of GWAS results
The population stratification was evaluated using Q-Q plots and PCA plots (Supplementary Figure S1, S2). The inflation factors (λ) for four traits were between 1.018 and 1.119 and PCA analysis showed that the population was relatively clustered, which suggests that our population had no obvious stratification. Through GWAS analysis, we totally identified 31 quantitative trait loci (QTLs) for the four GP related traits (Table 2; Figure 2), including eight for RG, nine for G6P, nine for LAT, five for GP. Notably, six out of the 31 QTLs showed significant association with more than one trait, including the QTLs with lead SNPs rs331183308 for RG and G6P, rs321246758 for RG and GP, rs318410870 and rs318442172 for RG, LAT and GP, and rs697205060 and rs345106152 for LAT and GP. Perhaps because LAT content accounted for the major fraction of GP at 45 min post-mortem (43.41/68.09 ≈ 63.8%; Table 1), they shared four common GWAS peak SNPs (Table 2).
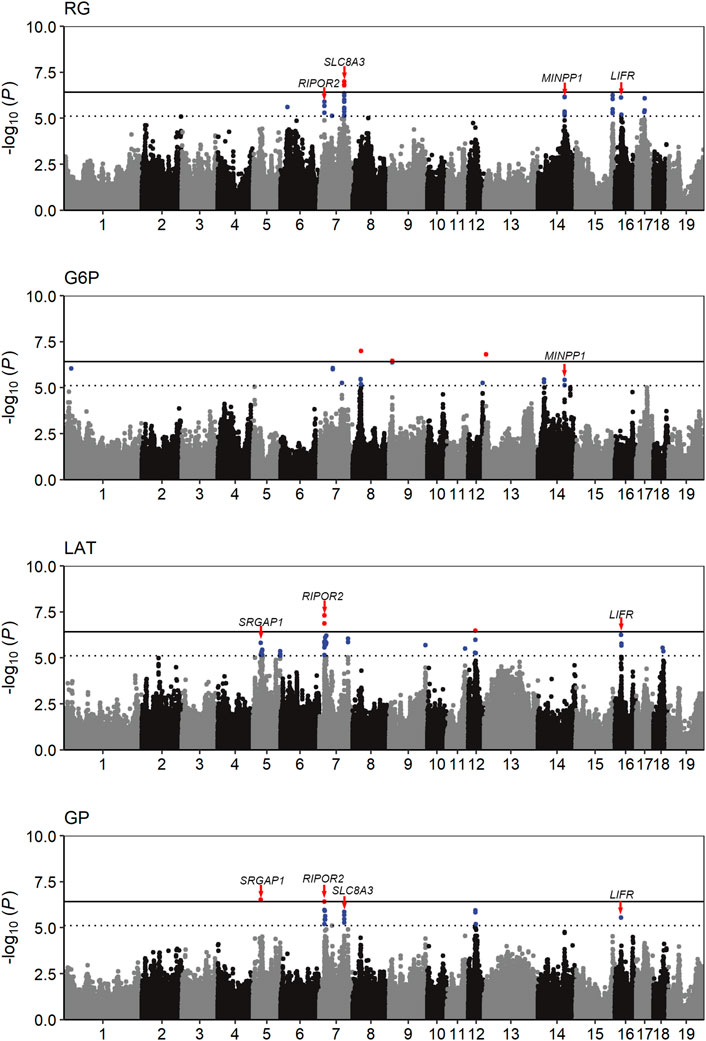
FIGURE 2. Manhattan plots of the GWAS result for the four GP related traits. The solid and dotted lines represent the genome-wide significance threshold and the suggestive significance threshold, respectively. The candidate genes close to five pleiotropic loci were identified.
QTLs for RG trait
The RG level in muscle at post-mortem 45 min is weakly associated with pHi (r = −0.19, p < 0.01) but strongly associated with pHd (r = 0.77, p < 0.01; Figure 1), suggesting that it plays an important role in promoting the subsequent decline of pH in meat. In the Erhualian pigs, a total of eight QTLs associated with RG were identified, which individually explained 3.6%–9.2% of the phenotypic variance. Among them, only the QTL with peak SNP rs321246758 (p = 9.86 × 10−8), which is located in the intron of SLC8A3 gene on Sus scrofa chromosome 7 (SSC7), exceeded the genome-wide significance threthold (Table 2). This QTL region for RG overlapped with the QTL region for GP, in which the peak SNPs rs321246758 for RG and the peak SNP rs332409349 for GP was in moderate LD (r2 = 0.63) (Supplementary Figure S3A), and a common SNP rs322439157 significantly associated with both traits was found, suggesting the existence of a pleiotropic locus in the SLC8A3–SYNJ2BP gene region (93.78–93.95 Mb) on SSC7 (Supplementary Figure S3C). The minor allele of rs322439157 were correlated with decreased levels of both RG and GP (Supplementary Figure S3B). In addition to rs321246758, three other GWAS signals appeared in the genes’ introns, including rs338058884, rs80841859, and rs318410870 located in the introns of FTO, TFAP2D and RIPOR2, respectively.
QTLs for G6P
The conversion of glucose to G6P is the first rate-limiting step in the glycolysis pathway. We detected nine QTLs significantly associated with G6P, including three genome-wide significant QTLs (p < 3.8 × 10−7) with the peak SNPs rs320783325, rs330779127 and rs333704759 on SSC8, 9 and 13, respectively. Among them, the rs320783325, an intron variant in LIMCH1 gene, had the most significant association with G6P (p = 9.81 × 10−8). In addition, we found that on SSC14, the GWAS peak SNP rs337801210 for G6P was located 92.7 kb proximal to the GWAS peak SNP rs331183308 for RG (Table 2). Although a weak LD (r2 = 0.21) were observed between rs337801210 and rs331183308, they and nine other significant SNPs (2 for G6P and seven for RG) linked to them were concentrated in the 99.52–99.64 Mb region, which only contains the MINPP1 gene. Moreover, the minor alleles of rs337801210 and rs331183308 were both associated with increased phenotypic values (Table 2). Thus, there may be a pleiotropic QTL in the MINPP1 region that affects both G6P and RG.
QTLs for LAT
Under anaerobic conditions, the end product of glycolysis in muscle cells is lactic acid. Our GWAS also revealed nine QTLs significantly associated with LAT (Table 2; Figure 2). Of them, two reached genome-wide significance: one on SSC7 with peak SNP rs318410870 (p = 4.86 × 10−8), and another on SSC12 with the peak SNP rs345106152 (p = 3.27 × 10−7). Moreover, these two peak SNPs were also significantly with GP. Notably, the pleiotropic locus rs345106152 explained the largest portion of phenotypic variance for LAT (10%) and GP (12.2%) (Table 2).
QTLs for GP
In this study, two genome-wide significant QTLs and three suggestive QTLs were found to affect the GP trait. These five QTLs for GP were also significantly associated with at least one of the three GP components (RG, G6P and LAT). The GWAS peak SNPs rs697205060 (p = 3.06 × 10−7) and rs318410870 (p = 3.77 × 10−7) represented the genome-wide significant QTLs on SSC5 and SSC7, respectively. For rs697205060, an intron variant of SRGAP1, its minor allele was associated with increased concentrations of GP and LAT, while the minor allele at rs318410870, an intron variant of the RIPOR2 gene, was associated with reduced concentrations of GP, RG and LAT (Table 2; Figures 3A–C). Furthermore, the LD analysis showed that the peak SNP rs318410870 and other three SNPs (including the second significant SNP) were all located in a 3-kb LD block in the RIPOR2 gene (Figure 3D). In addition, on SSC16, the SNP rs318442172 near the LIFR gene was also detected to be significantly associated with GP, RG and LAT.
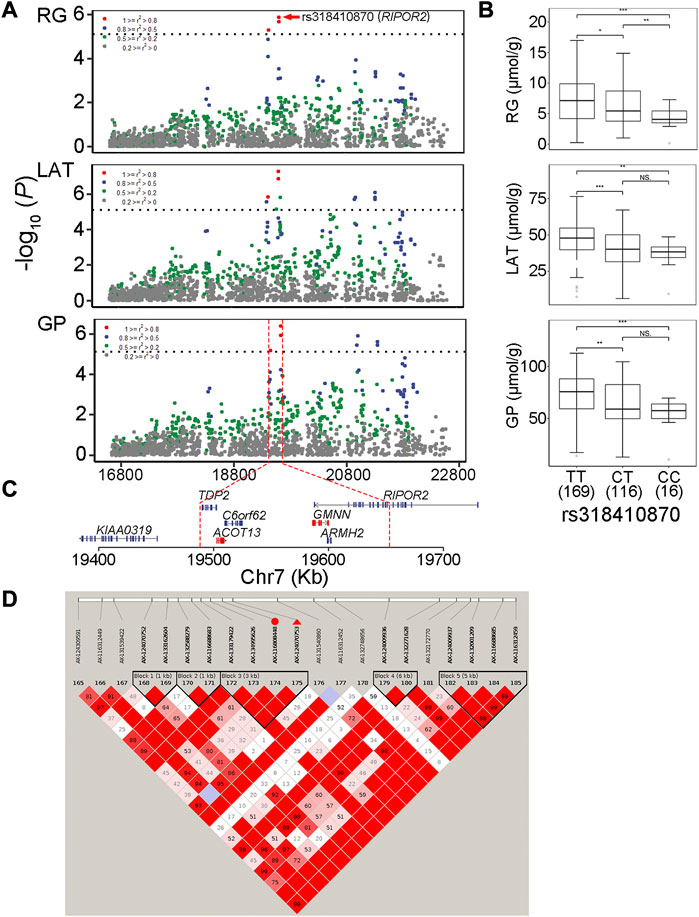
FIGURE 3. The GWAS peak SNP rs318410870 in RIPOR2 gene with pleiotropic effect on residual glycogen and glucose (RG), lactate (LAT) and glycolytic potential (GP) levels in muscle (A) Regional association plots of the QTL region centered by rs318410870 (in red dots). Different colors stand for degrees of linkage disequilibrium (r2) between corresponding SNPs and the peak SNP (B) Boxplots showing the difference in phenotypic values between the three genotypes of the SNP rs318410870 (C) The physical locations of genes surrounding the significant SNPs (D) Haplotype blocks on the QTL region containing rs318410870 indicated with red triangle and its one neighboring significant SNP indicated with red dot associated with the three traits.
Effects of multiple pleiotropic loci combination on meat quality traits
Next, we asked what would happen to other meat quality traits if we selected the five loci that affect GP and other components (including rs697205060, rs332409349, rs318410870, rs345106152, and rs318442172) in breeding. To this end, we first identified the genome combination types of these five SNPs in the Erhualian population. 73 genotype combinations were found in this population (Supplementary Table S1). According to the number of alleles (called allele2) that increase GP values at the five peak SNPs in each individual’s genotype combination, we divided all individuals into three groups: group A with 0–2 alleles2, group B with three to seven alleles2, and group C with 8–10 alleles2. Analysis of variance showed that there were significant differences in GP, pHi, pHu, pHd, drip loss, redness (a*) and yellowness (b*) of meat between the three groups, but no significant differences in lightness (L*). Moreover, the GP, pHd, drip loss, a* and b* values tended to increase in the genotype combination groups with more allele2, while their corresponding pHi and pHu showed a significant downward trend (Figure 4).
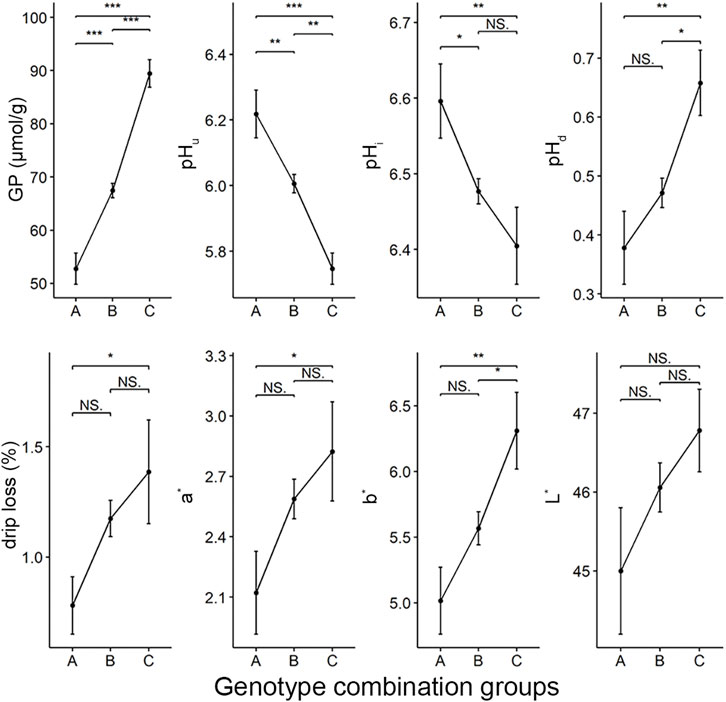
FIGURE 4. The differences in meat quality traits between three groups of genotype combinations of five GWAS peak SNPs for GP. The color parameters L*, a*, b* indicate the lightness, redness and yellowness of the meat, respectively. There were 31, 234 and 30 individuals in group A, B and C respectively.
Discussion
Many studies have investigated the variation of muscle GP level and its correlation with meat quality in Western commercial pig purebreds, crossbreds and Western Chinese hybrid pigs (van Laack and Kauffman, 1999; Bee et al., 2006; Duan et al., 2009; Conte et al., 2021), but such studies have been rarely conducted in Chinese indigenous pig breeds. In this study, the post-mortem muscle glycolytic phenotype of Erhualian pigs was characterized for the first time. We found that the average GP value of Erhualian was 68.09 μmol/g, which is only nearly half of the values reported by D’Astous-page et al. (D'Astous-Pagé et al., 2017) for Duroc (161.06 μmol/g), Landrace (164.27 μmol/g) and Yorkshire (159.27 μmol/g) and the value reported by Duan et al. (Duan et al., 2009) for a White Duroc × Erhualian F2 pigs (136.66 μmol/g). In addition, we showed that the RG and GP levels were highly negatively (r ≤ −0.79, p < 0.001) correlated with pHu, which is consistent with the notion that higher glycogen or GP at slaughter leads to greater pHu decline (Scheffler and Gerrard, 2007). Given the significant effect of GP on pH decline in muscle, it can be inferred that the lower glycogen and glucose contents in the muscle of Erhualian before slaughter may be the main reason why its meat pHu value was higher than that of Western commercial pig breeds (6.01 vs. ∼5.5) (Huang et al., 2020). In addition, the four GP-related traits of the Erhualian pigs showed considerable variation (CV = 30%–55%; Table 1), which may largely explain the wide variation of some meat quality traits related to GP in this population, such as the CV (63.4%) of pHd value (Huang et al., 2020).
Numerous QTLs for meat quality traits have been identified in various F2 pig crosses between Chinese and Western outbred lines or in Western commercial hybrid pigs, but few QTLs have been detected in founder lines, which to some extent hinder the development of pure-breeding and cross-breeding. To our best knowledge, this is the first study to explore genetic loci for GP-related traits in a Chinese local pig breed (Erhualian). Unlike most pig GWAS using 50 K or 60 K SNP chips, this study used 1.4 M SNP chips, which improved the statistical power and QTL mapping resolution. In fact, we not only detected 31 QTLs (8 loci per trait on average), but also found QTLs in some regions with small LD.
By comparing with the QTLs for GP deposited in pig QTL database (pigQTLdb), we found that all QTLs detected in this study, except for two, had not been previously reported. On SSC7, the GWAS peak locus (rs332736034) we identified for LAT at 109.12 Mb is close to the QTL for the same trait detected in a Meishan × Pietrain F2 family (Reiner et al., 2002). Similarly, on SSC15, the significant region associated with RG of Erhualian overlapped with the QTL interval found in a Berkshire × Yorkshire F2 intercross (Ciobanu et al., 2001). Thus, the alleles at the two loci may be segregating rather than fixed in Erhualian pigs.
In the regions around the GWAS peaks, we screened a number of candidate genes, providing new clues for understanding the genetic mechanism of glycolytic phenotypes. For the identified pleiotropic loci, five strong candidate genes were proposed based on their functional annotations. The GWAS signal (rs331183308) for RG and G6P found on SSC14 was adjacent to the MINPP1 gene. The protein encoded by MINPP1 is multiple inositol polyphosphate phosphatase, which can remove 3-phosphate from inositol phosphate substrates and convert 2,3 bisphosphoglycerate (2,3-BPG) to 2-phosphoglycerate that is part of the glycolytic pathway (Cho et al., 2008). The related pathways of MINPP1 include Rapoport-Luebering glycolytic shunt and inositol phosphate metabolism (Cho et al., 2008). Thus, MINPP1 could be regarded as strong candidate genes for RG and G6P. In addition, the peak locus rs321246758 in the intron of SLC8A3 on SSC7 accounted 8.7% of the phenotypic variance in RG. The SLC8A3 gene encodes a member of the sodium/calcium exchanger integral membrane protein family. Calcium plays a crucial role in muscle contraction and energy metabolism (Berridge et al., 2003), and SLC8A3 contributes to Ca2+ transport during excitation-contraction coupling in muscle. Its loss leads to muscle necrosis and abnormal Ca2+ homeostasis (Michel et al., 2014). Therefore, SLC8A3 is a good candidate for RG.
The two GWAS peaks rs318410870 on SSC7 and rs318442172 on SSC16 were common to RG, LAT and GP. The SNP rs318410870 is an intronic variant of the RIPOR2 gene. RIPOR2 can inhibit the activity of small GTPase RhoA and regulate myoblast differentiation (Yoon et al., 2007). RhoA plays an important role in actin cytoskeleton reorganization through RhoA-ROCKS signaling pathway (Ridley and Hall, 1992). Khue Ha Minh Duong et al. indicated that RhoA mediates glucose transport by regulating the vesicle trafficking machinery in an Akt-independent manner (Duong and Chun, 2019), and David Wu et al. also showed that activation of RhoA induces celluar glycolysis through translocation of glucose transporter GLUT3, which provides energy for cell contraction (Wu et al., 2021). Therefore, RIPOR2 may influence the process of glucose metabolism by inhibiting RhoA. Another GWAS locus affecting three traits, rs318442172, is an intronic variant in LIFR gene. LIFR protein, a member of the type I cytokine receptor family, binds to a converter subunit gp130 to form a receptor complex which mediates the role of the leukemia inhibitory factor (LIF) in cellular differentiation and proliferation (Hunt and White, 2016). Jessica C Hogan et al. showed that LIF regulates the expression of genes involved in lipid synthesis and has an impact on insulin-stimulated glucose uptake (Hogan and Stephens, 2005). In addition, Suhu Liu et al. found that LIFR can regulates the expression level of glucose transporter GLUT1, and the reduced expression level of LIFR leads to an increase in GLUT1 expression level, glycolysis and mitochondrial respiration (Liu et al., 2021). Thus, LIFR and RIPOR2 could be regarded as candidate genes for the GP-related traits.
Two QTLs with the peak SNPs rs697205060 and rs345106152 were detected to affect both GP and LAT. The SNP rs697205060 is located in the intron of the SRGAP1 gene, which encodes a GTPase activator for RhoA and Cdc42. As mentioned above, RhoA plays an important role in glucose transport (Ridley and Hall, 1992; Duong and Chun, 2019; Wu et al., 2021). Therefore, SRGAP1 may influence the process of glucose metabolism by activating RhoA, and could be regarded as a promising candidate gene for the GP-related traits.
In addition to the pleiotropic loci, several possible candidate genes for the QTL associated with a single trait were also recognized. For example, the FTO gene containing the significant SNP rs338058884 for RG is a promising candidate. Melina Claussnitzer et al. found that the SNP rs1421085 in FTO gene disrupts a conserved motif for the ARID5B repressor, which leads to derepression of a potent preadipocyte enhancer and an increase in lipid storage with the increasing expression of IRX3 and IRX5 (Claussnitzer et al., 2015). Moreover, FTO’s related pathways include Glucose/Energy Metabolism and many studies have shown that FTO is closely related to glucose metabolism (Maia-Landim et al., 2018; Yang et al., 2019; Krüger et al., 2020; De Soysa et al., 2021). So, FTO is likely involved in regulating muscle glycogen content. On SSC17, the coding gene closest to the peak SNP rs322341359 for RG is FOXA2 that encodes Forkhead Box A2. As a transcription activator, FOXA2 has a role in regulation of the expression of genes responsible for glucose homeostasis. Ping Wang et al. showed that FOXA2 restrains the proliferation of liver progenitor cells by decreasing PI3K/Akt/HK2-mediated glycolysis (Wang et al., 2020). Jennifer N Dines et al. reported that aberrant glucose homeostasis occurs in an individual with a missense variant in FOXA2 (Dines et al., 2019). Therefore, FOXA2 are likely related to the SSC17 QTL effect on RG.
The most significant GWAS signal (rs320783325 with p = 9.81 × 10−8) for G6P was present in the LIMCH1 gene. This gene encodes calponin homology domains-containing protein 1 which enables myosin II head/neck binding activity. The absence of LIMCH1 can affects the formation of actin stress fibers as well as the stability of focal adhesions, which are basic structures to ensure benign contraction and expansion in skeletal muscle (Lin et al., 2017). Fiuza-luces et al. showed that there was a significant relationship between LIMCH1 expression and adaptation of skeletal muscle to endurance training, independent of muscle glycogen availability (Fiuza-Luces et al., 2018). However, it is not ruled out that LIMCH1 alters the rate of glycolysis when it participates in stress pathways regulated by exercise, which warrants further investigation.
It is very important to identify genetic loci affecting GP phenotypes for genetic improvement of meat quality traits, as demonstrated by the application of mutations in PRKAG3 and PHKG1, two known major genes in pig breeding (Galve et al., 2013). In this study, we identified five GP-associated peak SNPs, with minor allele frequencies of 0.127–0.434 (Table 2), indicating that there is sufficient room for selection of these loci in Erhualian pigs. More importantly, we observed that the genotype combinations of these five loci significantly affected not only GP, but also pH, color and water-holding capacity of meat (Figure 4). Therefore, the results provide a reference for improving meat quality uniformity and breeding efficiency of Erhualian pigs by multi-marker assistant selection.
Conclusion
We found that the GP content in the skeletal muscle of Erhualian pigs was low and had a wide range of phenotypic variation, which may play a role in the formation of specific meat quality traits of this breed. Further, our GWAS analysisin the Erhualian population identified 31 loci significantly associated with the GP related traits, including 29 novel loci. Among them, six loci exhibited pleiotropic effects on these GP related traits. SCL8A3, MINPP1, SRGAP1, RIPOR2, and LIFR near the pleiotropic QTL peaks are highlighted as novel candidate genes related to glucose metabolism in muscle, which are worthy of further study. We also demonstrate the potential application of GP-associated SNPs in improving a variety of meat quality traits. Therefore, this study not only advances our understanding of the genetic architecture of GP related traits but also provide genetic markers for improving the pork quality of Erhualian.
Data availability statement
The data analyzed in this study is subject to the following licenses/restrictions: Datasets belong to Jiangxi Agricultural University. Requests to access these datasets should be directed to bWFfanVud3VAaG90bWFpbC5jb20=.
Ethics statement
The animal study was reviewed and approved by the ethics committee of Jiangxi Agricultural University. Written informed consent was obtained from the owners for the participation of their animals in this study.
Author contributions
XX performed the experiments, analyzed the data and wrote the article. CH, YH, XZ, and RZ helped with the experimental process and data analysis. HA contributed materials. LH and JM conceived and designed the experiments. All authors reviewed and approved the article.
Funding
This study was supported by the National Natural Science Foundation of China (No 31790413 and 31872339).
Conflict of interest
The authors declare that the research was conducted in the absence of any commercial or financial relationships that could be construed as a potential conflict of interest.
Publisher’s note
All claims expressed in this article are solely those of the authors and do not necessarily represent those of their affiliated organizations, or those of the publisher, the editors and the reviewers. Any product that may be evaluated in this article, or claim that may be made by its manufacturer, is not guaranteed or endorsed by the publisher.
Supplementary material
The Supplementary Material for this article can be found online at: https://www.frontiersin.org/articles/10.3389/fgene.2023.1141411/full#supplementary-material
SUPPLEMENTARY FIGURE S1 | The Q-Q plots for 4 GP-related traits. The x axis represents the expected -log10 (P) and the y axis represents the observed -log10 (P)
SUPPLEMENTARY FIGURE S2 | The PCA plots for the genotype data of Erhuanlian population
SUPPLEMENTARY FIGURE S3 | The pleiotropic QTL for residual glycogen and glucose (RG) and glycolytic potential (GP) in muscle at about 93.78 Mb on SSC7. (A) Regional association plots of the QTL region of 4 Mb centered by the peak SNP (in red dots). Different colors stand for degrees of linkage disequilibrium (r2) between corresponding SNPs and the peak SNP. (B) Boxplots showing the difference in RG and GP values between the three genotypes of the common significant SNP rs322439157. (C) The physical locations of genes surrounding the significant SNPs
References
Barrett, J. C., Fry, B., Maller, J., and Daly, M. J. (2005). Haploview: Analysis and visualization of LD and haplotype maps. Bioinformatics 21 (2), 263–265. doi:10.1093/bioinformatics/bth457
Bee, G., Anderson, A. L., Lonergan, S. M., and Huff-Lonergan, E. (2007). Rate and extent of pH decline affect proteolysis of cytoskeletal proteins and water-holding capacity in pork. Meat Sci. 76 (2), 359–365. doi:10.1016/j.meatsci.2006.12.004
Bee, G., Biolley, C., Guex, G., Herzog, W., Lonergan, S. M., and Huff-Lonergan, E. (2006). Effects of available dietary carbohydrate and preslaughter treatment on glycolytic potential, protein degradation, and quality traits of pig muscles. J. Anim. Sci. 84 (1), 191–203. doi:10.2527/2006.841191x
Berridge, M. J., Bootman, M. D., and Roderick, H. L. (2003). Calcium signalling: Dynamics, homeostasis and remodelling. Nat. Rev. Mol. Cell Biol. 4 (7), 517–529. doi:10.1038/nrm1155
Bonneau, M., and Lebret, B. (2010). Production systems and influence on eating quality of pork. Meat Sci. 84 (2), 293–300. doi:10.1016/j.meatsci.2009.03.013
Chen, J., Huang, R., Li, Q., and Gu, Y. (2013). Research progress of germplasm resources of Erhuface pig and experience in development and utilization. CHINA SWINE IND. 8 (S1), 72–75.
Cho, J., King, J. S., Qian, X., Harwood, A. J., and Shears, S. B. (2008). Dephosphorylation of 2,3-bisphosphoglycerate by MIPP expands the regulatory capacity of the Rapoport-Luebering glycolytic shunt. Proc. Natl. Acad. Sci. U. S. A. 105 (16), 5998–6003. doi:10.1073/pnas.0710980105
Ciobanu, D., Bastiaansen, J., Malek, M., Helm, J., Woollard, J., Plastow, G., et al. (2001). Evidence for new alleles in the protein kinase adenosine monophosphate-activated gamma(3)-subunit gene associated with low glycogen content in pig skeletal muscle and improved meat quality. Genetics 159 (3), 1151–1162. doi:10.1093/genetics/159.3.1151
Claussnitzer, M., Dankel, S. N., Kim, K-H., Quon, G., Meuleman, W., Haugen, C., et al. (2015). FTO obesity variant circuitry and adipocyte browning in humans. N. Engl. J. Med. 373 (10), 895–907. doi:10.1056/NEJMoa1502214
Conte, S., Pomar, C., Paiano, D., Duan, Y., Zhang, P., Lévesque, J., et al. (2021). The effects of feeding finishing pigs of two genders with a high fiber and high fat diet on muscle glycolytic potential at slaughter and meat quality. Meat Sci. 177, 108484. doi:10.1016/j.meatsci.2021.108484
D'Astous-Pagé, J., Gariépy, C., Blouin, R., Cliche, S., Méthot, S., Sullivan, B., et al. (2017). Identification of single nucleotide polymorphisms in carnosine-related genes and effects of genotypes on pork meat quality attributes. Meat Sci. 134, 54–60. doi:10.1016/j.meatsci.2017.07.019
De Soysa, A. K. H., Langaas, M., Jakic, A., Shojaee-Moradie, F., Umpleby, A. M., Grill, V., et al. (2021). The fat mass and obesity-associated (FTO) gene allele rs9939609 and glucose tolerance, hepatic and total insulin sensitivity, in adults with obesity. PloS One 16 (3), e0248247. doi:10.1371/journal.pone.0248247
Dines, J. N., Liu, Y. J., Neufeld-Kaiser, W., Sawyer, T., Ishak, G. E., Tully, H. M., et al. (2019). Expanding phenotype with severe midline brain anomalies and missense variant supports a causal role for FOXA2 in 20p11.2 deletion syndrome. Am. J. Med. Genet. A 179 (9), 1783–1790. doi:10.1002/ajmg.a.61281
Duan, Y. Y., Ma, J. W., Yuan, F., Huang, L. B., Yang, K. X., Xie, J. P., et al. (2009). Genome-wide identification of quantitative trait loci for pork temperature, pH decline, and glycolytic potential in a large-scale White Duroc x Chinese Erhualian resource population. J. Anim. Sci. 87 (1), 9–16. doi:10.2527/jas.2008-1128
Duong, K. H. M., and Chun, K-H. (2019). Regulation of glucose transport by RhoA in 3T3-L1 adipocytes and L6 myoblasts. Biochem. Biophys. Res. Commun. 519 (4), 880–886. doi:10.1016/j.bbrc.2019.09.083
Fiuza-Luces, C., Santos-Lozano, A., Llavero, F., Campo, R., Nogales-Gadea, G., Díez-Bermejo, J., et al. (2018). Muscle molecular adaptations to endurance exercise training are conditioned by glycogen availability: A proteomics-based analysis in the McArdle mouse model. J. Physiol. 596 (6), 1035–1061. doi:10.1113/JP275292
Fujii, J., Otsu, K., Zorzato, F., de Leon, S., Khanna, V. K., Weiler, J. E., et al. (1991). Identification of a mutation in porcine ryanodine receptor associated with malignant hyperthermia. Science 253 (5018), 448–451. doi:10.1126/science.1862346
Gajana, C. S., Nkukwana, T. T., Marume, U., and Muchenje, V. (2013). Effects of transportation time, distance, stocking density, temperature and lairage time on incidences of pale soft exudative (PSE) and the physico-chemical characteristics of pork. Meat Sci. 95 (3), 520–525. doi:10.1016/j.meatsci.2013.05.028
Galve, A., Burgos, C., Varona, L., Carrodeguas, J. A., Cánovas, Á., and López-Buesa, P. (2013). Allelic frequencies of PRKAG3 in several pig breeds and its technological consequences on a Duroc × Landrace-Large White cross. J. Animal Breed. Genet. = Zeitschrift Fur Tierzuchtung Und Zuchtungsbiologie. 130 (5), 382–393. doi:10.1111/jbg.12042
Gong, H., Xiao, S., Li, W., Huang, T., Huang, X., Yan, G., et al. (2019). Unravelling the genetic loci for growth and carcass traits in Chinese Bamaxiang pigs based on a 1.4 million SNP array. J. Anim. Breed. Genet. 136 (1), 3–14. doi:10.1111/jbg.12365
Gonzalez-Rivas, P. A., Chauhan, S. S., Ha, M., Fegan, N., Dunshea, F. R., and Warner, R. D. (2020). Effects of heat stress on animal physiology, metabolism, and meat quality: A review. Meat Sci. 162, 108025. doi:10.1016/j.meatsci.2019.108025
Hamilton, D. N., Miller, K. D., Ellis, M., McKeith, F. K., and Wilson, E. R. (2003). Relationships between longissimus glycolytic potential and swine growth performance, carcass traits, and pork quality. J. Anim. Sci. 81 (9), 2206–2212. doi:10.2527/2003.8192206x
Hogan, J. C., and Stephens, J. M. (2005). Effects of leukemia inhibitory factor on 3T3-L1 adipocytes. J. Endocrinol. 185 (3), 485–496. doi:10.1677/joe.1.05980
Huang, Y., Zhou, L., Zhang, J., Liu, X., Zhang, Y., Cai, L., et al. (2020). A large-scale comparison of meat quality and intramuscular fatty acid composition among three Chinese indigenous pig breeds. Meat Sci. 168, 108182. doi:10.1016/j.meatsci.2020.108182
Hunt, L. C., and White, J. (2016). The role of leukemia inhibitory factor receptor signaling in skeletal muscle growth, injury and disease. Adv. Exp. Med. Biol. 900, 45–59. doi:10.1007/978-3-319-27511-6_3
Khanal, P., Maltecca, C., Schwab, C., Gray, K., and Tiezzi, F. (2019). Genetic parameters of meat quality, carcass composition, and growth traits in commercial swine. J. Anim. Sci. 97 (9), 3669–3683. doi:10.1093/jas/skz247
Krüger, N., Biwer, L. A., Good, M. E., Ruddiman, C. A., Wolpe, A. G., DeLalio, L. J., et al. (2020). Loss of endothelial FTO antagonizes obesity-induced metabolic and vascular dysfunction. Circ. Res. 126 (2), 232–242. doi:10.1161/CIRCRESAHA.119.315531
Lander, E., and Kruglyak, L. (1995). Genetic dissection of complex traits: Guidelines for interpreting and reporting linkage results. Nat. Genet. 11 (3), 241–247. doi:10.1038/ng1195-241
Lewis, P. K., Yakes, L. Y., Noland, P. R., and Brown, C. J. (1987). The effect of DFD classification and internal cooking temperature on certain pork muscle characteristics. Meat Sci. 21 (2), 137–144. doi:10.1016/0309-1740(87)90026-X
Li, P-H., Ma, X., Zhang, Y-Q., Zhang, Q., and Huang, R-H. (2017). Progress in the physiological and genetic mechanisms underlying the high prolificacy of the Erhualian pig. Yi Chuan 39 (11), 1016–1024. doi:10.16288/j.yczz.17-119
Lin, Y-H., Zhen, Y-Y., Chien, K-Y., Lee, I. C., Lin, W-C., Chen, M-Y., et al. (2017). LIMCH1 regulates nonmuscle myosin-II activity and suppresses cell migration. Mol. Biol. Cell 28 (8), 1054–1065. doi:10.1091/mbc.E15-04-0218
Liu, S., Gandler, H. I., Tošić, I., Ye, D. Q., Giaccone, Z. T., and Frank, D. A. (2021). Mutant KRAS downregulates the receptor for leukemia inhibitory factor (LIF) to enhance a signature of glycolysis in pancreatic cancer and lung cancer. Mol. Cancer Res. 19, 1283–1295. doi:10.1158/1541-7786.MCR-20-0633
Liu, X., Xiong, X., Yang, J., Zhou, L., Yang, B., Ai, H., et al. (2015). Genome-wide association analyses for meat quality traits in Chinese Erhualian pigs and a Western Duroc × (Landrace × Yorkshire) commercial population. Genet. Sel. Evol. 47, 44. doi:10.1186/s12711-015-0120-x
Luo, J., Shen, Y. L., Lei, G. H., Zhu, P. K., Jiang, Z. Y., Bai, L., et al. (2017). Correlation between three glycometabolic-related hormones and muscle glycolysis, as well as meat quality, in three pig breeds. J. Sci. Food Agric. 97 (9), 2706–2713. doi:10.1002/jsfa.8094
Ma, J., Yang, J., Zhou, L., Ren, J., Liu, X., Zhang, H., et al. (2014). A splice mutation in the PHKG1 gene causes high glycogen content and low meat quality in pig skeletal muscle. PLoS Genet. 10 (10), e1004710. doi:10.1371/journal.pgen.1004710
Ma, J., Yang, J., Zhou, L., Zhang, Z., Ma, H., Xie, X., et al. (2013). Genome-wide association study of meat quality traits in a White Duroc×Erhualian F2 intercross and Chinese Sutai pigs. PloS One 8 (5), e64047. doi:10.1371/journal.pone.0064047
Maia-Landim, A., Ramírez, J. M., Lancho, C., Poblador, M. S., and Lancho, J. L. (2018). Long-term effects of Garcinia cambogia/Glucomannan on weight loss in people with obesity, PLIN4, FTO and Trp64Arg polymorphisms. BMC Complement. Altern. Med. 18 (1), 26. doi:10.1186/s12906-018-2099-7
Maribo, H., Støier, S., and Jørgensen, P. F. (1999). Procedure for determination of glycolytic potential in porcine m. longissimus dorsi. Meat Sci. 51 (2), 191–193. doi:10.1016/s0309-1740(98)00130-2
Michel, L. Y. M., Verkaart, S., Koopman, W. J. H., Willems, P. H. G. M., Hoenderop, J. G. J., and Bindels, R. J. M. (2014). Function and regulation of the Na+-Ca2+ exchanger NCX3 splice variants in brain and skeletal muscle. J. Biol. Chem. 289 (16), 11293–11303. doi:10.1074/jbc.M113.529388
Milan, D., Jeon, J. T., Looft, C., Amarger, V., Robic, A., Thelander, M., et al. (2000). A mutation in PRKAG3 associated with excess glycogen content in pig skeletal muscle. Science 288 (5469), 1248–1251. doi:10.1126/science.288.5469.1248
Monin, G., and Sellier, P. (1985). Pork of low technological quality with a normal rate of muscle pH fall in the immediate post-mortem period: The case of the Hampshire breed. Meat Sci. 13 (1), 49–63. doi:10.1016/S0309-1740(85)80004-8
Pearson, T. A., and Manolio, T. A. (2008). How to interpret a genome-wide association study. JAMA 299 (11), 1335–1344. doi:10.1001/jama.299.11.1335
Popp, J., Wicke, M., Klein, G., and Krischek, C. (2015). The relationship of pork longissimus muscle pH to mitochondrial respiratory activities, meat quality and muscle structure. Animal 9 (2), 356–361. doi:10.1017/S1751731114002365
Purcell, S., Neale, B., Todd-Brown, K., Thomas, L., Ferreira, M. A. R., Bender, D., et al. (2007). Plink: A tool set for whole-genome association and population-based linkage analyses. Am. J. Hum. Genet. 81 (3), 559–575. doi:10.1086/519795
Reiner, G., Heinricy, L., Muller, E., Geldermann, H., and Dzapo, V. (2002). Indications of associations of the porcine FOS proto-oncogene with skeletal muscle fibre traits. Anim. Genet. 33 (1), 49–55. doi:10.1046/j.1365-2052.2002.00805.x
Ridley, A. J., and Hall, A. (1992). The small GTP-binding protein rho regulates the assembly of focal adhesions and actin stress fibers in response to growth factors. Cell 70 (3), 389–399. doi:10.1016/0092-8674(92)90163-7
Sayre, R. N., Briskey, E. J., and Hoekstra, W. G. (1963). Porcine muscle glycogen structure and its association with other muscle properties. Proc. Soc. Exp. Biol. Med. 112, 223–225. doi:10.3181/00379727-112-27999
Scheffler, T. L., and Gerrard, D. E. (2007). Mechanisms controlling pork quality development: The biochemistry controlling postmortem energy metabolism. Meat Sci. 77 (1), 7–16. doi:10.1016/j.meatsci.2007.04.024
Shen, Q. W., Means, W. J., Thompson, S. A., Underwood, K. R., Zhu, M. J., McCormick, R. J., et al. (2006). Pre-slaughter transport, AMP-activated protein kinase, glycolysis, and quality of pork loin. Meat Sci. 74 (2), 388–395. doi:10.1016/j.meatsci.2006.04.007
Van der Wal, P. G., Bolink, A. H., and Merkus, G. S. (1988). Differences in quality characteristics of normal, PSE and DFD pork. Meat Sci. 24 (1), 79–84. doi:10.1016/0309-1740(89)90009-0
van Laack, R. L., and Kauffman, R. G. (1999). Glycolytic potential of red, soft, exudative pork longissimus muscle. J. Anim. Sci. 77 (11), 2971–2973. doi:10.2527/1999.77112971x
Wang, P., Cong, M., Liu, T., Li, Y., Liu, L., Sun, S., et al. (2020). FoxA2 inhibits the proliferation of hepatic progenitor cells by reducing PI3K/Akt/HK2-mediated glycolysis. J. Cell Physiol. 235 (12), 9524–9537. doi:10.1002/jcp.29759
Wu, D., Harrison, D. L., Szasz, T., Yeh, C-F., Shentu, T-P., Meliton, A., et al. (2021). Single-cell metabolic imaging reveals a SLC2A3-dependent glycolytic burst in motile endothelial cells. Nat. Metab. 3 (5), 714–727. doi:10.1038/s42255-021-00390-y
Wu, P., Wang, K., Zhou, J., Chen, D., Yang, X., Jiang, A., et al. (2020). Whole-genome sequencing association analysis reveals the genetic architecture of meat quality traits in Chinese Qingyu pigs. Genome 63 (10), 503–515. doi:10.1139/gen-2019-0227
Yang, Q., Cui, J., Chazaro, I., Cupples, L. A., and Demissie, S. (2005). Power and type I error rate of false discovery rate approaches in genome-wide association studies. BMC Genet. 6 (1), S134. doi:10.1186/1471-2156-6-S1-S134
Yang, Y., Shen, F., Huang, W., Qin, S., Huang, J-T., Sergi, C., et al. (2019). Glucose is involved in the dynamic regulation of m6A in patients with type 2 diabetes. J. Clin. Endocrinol. Metab. 104 (3), 665–673. doi:10.1210/jc.2018-00619
Yoon, S., Molloy, M. J., Wu, M. P., Cowan, D. B., and Gussoni, E. (2007). C6ORF32 is upregulated during muscle cell differentiation and induces the formation of cellular filopodia. Dev. Biol. 301 (1), 70–81. doi:10.1016/j.ydbio.2006.11.002
Zhang, Y., Zhang, J., Gong, H., Cui, L., Zhang, W., Ma, J., et al. (2019). Genetic correlation of fatty acid composition with growth, carcass, fat deposition and meat quality traits based on GWAS data in six pig populations. Meat Sci. 150, 47–55. doi:10.1016/j.meatsci.2018.12.008
Keywords: Erhualian, pig, glycolysis potential, GWAS, candidate gene
Citation: Xie X, Huang C, Huang Y, Zou X, Zhou R, Ai H, Huang L and Ma J (2023) Genetic architecture for skeletal muscle glycolytic potential in Chinese Erhualian pigs revealed by a genome-wide association study using 1.4M SNP array. Front. Genet. 14:1141411. doi: 10.3389/fgene.2023.1141411
Received: 10 January 2023; Accepted: 16 February 2023;
Published: 17 March 2023.
Edited by:
Li Ma, University of Maryland, College Park, United StatesReviewed by:
Wentao Cai, Institute of Animal Sciences, Chinese Academy of Agricultural Sciences, ChinaLiming Hou, Nanjing Agricultural University, China
Copyright © 2023 Xie, Huang, Huang, Zou, Zhou, Ai, Huang and Ma. This is an open-access article distributed under the terms of the Creative Commons Attribution License (CC BY). The use, distribution or reproduction in other forums is permitted, provided the original author(s) and the copyright owner(s) are credited and that the original publication in this journal is cited, in accordance with accepted academic practice. No use, distribution or reproduction is permitted which does not comply with these terms.
*Correspondence: Lusheng Huang, bHVzaGVuZ2h1YW5nQGh0b21haWwuY29t; Junwu Ma, bWFfanVud3VAaG90bWFpbC5jb20=bWp3anhsYWJAanhhdS5lZHUuY24=