- 1Department of Plant Protection, Faculty of Agriculture, Bolu Abant Izzet Baysal University, Bolu, Türkiye
- 2Department of Field Crops, Faculty of Agriculture, Bolu Abant Izzet Baysal University, Bolu, Türkiye
- 3Laboratory of Crop Production, Department of Applied Biosciences, Kyungpook National University, Daegu, Republic of Korea
- 4Faculty of Agricultural Sciences and Technologies, Sivas University of Science and Technology, Sivas, Türkiye
- 5Department of Plant Resources and Environment, Jeju National University, Jeju, Republic of Korea
Accurate and early diagnosis of bean common mosaic virus (BCMV) in Phaseolus vulgaris tissues is critical since the pathogen can spread easily and have long-term detrimental effects on bean production. The use of resistant varieties is a key factor in the management activities of BCMV. The study reported here describes the development and application of a novel SYBR Green-based quantitative real-time PCR (qRT-PCR) assay targeting the coat protein gene to determine the host sensitivity to the specific NL-4 strain of BCMV. The technique showed high specificity, validated by melting curve analysis, without cross-reaction. Further, the symptoms development of twenty advanced common bean genotypes after mechanical BCMV-NL-4 infection was evaluated and compared. The results showed that common bean genotypes exhibit varying levels of host susceptibility to this BCMV strain. The YLV-14 and BRS-22 genotypes were determined as the most resistant and susceptible genotypes, respectively, in terms of aggressiveness of symptoms. The accumulation of BCMV was analyzed in the resistant and susceptible genotypes 3, 6, and 9 days following the inoculation by the newly developed qRT-PCR. The mean cycle threshold (Ct) values showed that the viral titer was significantly lower in YLV-14, which was evident in both root and leaf 3 days after the inoculation. The qRT-PCR thus facilitated an accurate, specific, and feasible assessment of BCMV accumulation in bean tissues even in low virus titers, allowing novel clues in selecting resistant genotypes in the early stages of infection, which is critical for disease management. To the best of our knowledge, this is the first study of a successfully performed qRT-PCR to estimate BCMV quantification.
1 Introduction
Plant viruses are obligate intracellular pathogens that inhabit the symplast of their hosts solely to accomplish their replication process, including transcription/translation, encapsidation, and transportation to the new host’s cells. The viral accumulation in the various parts of a host plant is crucial for developing symptoms and persistence of the virus in the plant (Hipper et al., 2013). The interaction between viruses and their host plants has intrigued researchers working on breeding programs to improve resistant varieties. Developing new resistant varieties against viral pathogens in plant breeding programs is one of the most important approaches to coping with new environmental challenges lived in agriculture and making the world more liveable and self-sufficient (Borrelli et al., 2018). This phenomenon is that increasing disease resistance in plants plays a significant role in regulating crop production to meet the demand of the increasing global population.
Legumes, known as an important food source in human nutrition on a global scale, have been infected by more than 168 plant viruses from 16 families (Chatzivassiliou, 2021). Bean common mosaic virus (BCMV), a member of Potyvirus, is one of the most important viral pathogens of common bean and has an economic impact on crop production worldwide. The pathogen is monopartite flexuous rod-shaped with a positive sense ssRNA genome of about 10 kb size, transmitted by seeds and aphids (El-Sawy et al., 2014). The infection rate of BCMV by seed transmission may vary between 15 and 30% depending on host genotype, viral strain, infection time, and cultivating conditions (Morales, 1989; Arlı-Sökmen et al., 2016). Virus-free seeds are recommended for the management of BCMV; however, the use of resistant varieties is considered to be the most effective and practical control method (Drijfhout, 1978; Hall, 1994; Miklas et al., 2000). Bean resistance to BCMV is managed by the dominant I gene and six different recessive genes (bc-u, bc-1/bc-12, bc-2/bc-22, and bc-3) (Drijfhout, 1978; Kelly et al., 1995).
Research on recent developments in the understanding of host fitness for resistance is still ongoing (Soler-Garzón et al., 2021). Based on the pathogenicity (P0, P1, P12, P2, P22) and differential bean host reaction, BCMV isolates were classified into 7 pathogroups (PGs), including PGI-II-IV-V-VI-VII and VIII (Drijfhout, 1978; Feng et al., 2014; Feng et al., 2015; Kyrychenko et al., 2019). Due to the necessity of using resistant varieties, plant breeders need to investigate sources of BCMV resistance in response to the challenges of common bean susceptibility. Several studies have been conducted to evaluate the existence of disease-resistant genotypes (Mukeshimana et al., 2005; Bello et al., 2014; Boersma et al., 2014; Yeken et al., 2018; Palacıoğlu et al., 2021).
The detection of BCMV in different plant tissues was carried out with several serological and molecular methods, including Double Antibody Sandwich Enzyme-Linked Immunosorbent Assay (DAS-ELISA) (Vetten et al., 1992), reverse transcription-polymerase chain reaction (RT-PCR) (Xu and Hampton, 1996), and sequencing analysis (Arlı-Sökmen et al., 2016). Effective and highly sensitive approaches have been improved in recent years due to the giant breakthroughs in biotechnology and molecular virology (Yadav et al., 2016). One of these advances is quantitative real-time PCR (qRT-PCR), which is increasingly being used to develop sensitive, accurate, and reliable detection in plant virology (Poojari et al., 2016; Malandraki et al., 2017). The qRT-PCR is based on displaying and calculating the increasing fluorescent radiation (cycle threshold, Ct) based on the amplification of the target region at each cycle (Boonham et al., 2014), showing that low Ct levels indicate higher virus titre and high Ct less viral load, respectively. The qRT-PCR assay has many advantages due to its simplicity, requiring short hands-on time, and automated amplified capacity of detected samples (Espy et al., 2006). The diagnosis of various plant viruses has been accomplished by several studies based on qRT-PCR assays (Mumford et al., 2000; Picó et al., 2005; López et al., 2006; Ling et al., 2007; Hongyun et al., 2008; Ruiz-Ruiz et al., 2009a; Debreczeni et al., 2011; Ferriol et al., 2011; Sharma and Dasgupta, 2012; Herrera-Vásquez et al., 2015; MacKenzie et al., 2015). In addition to diagnostic studies, some significant progress have also been performed on qRT-PCR assays to detect mixed plant viruses infections (Mortimer-Jones et al., 2009; Abrahamian et al., 2013), in cross-protection studies (Ruiz-Ruiz et al., 2009b; Hanssen et al., 2010), in determining virus-vector association and epidemic analysis (Olmos et al., 2005; Rotenberg et al., 2009; Ferriol et al., 2013; Debreczeni et al., 2014), in the correlation between virus accumulations and plant resistance (Gil-Salas et al., 2009; Galipienso et al., 2013; Soler et al., 2015), and in the discrimination mutations caused by aggressive strains (Carrasco et al., 2007; Peña et al., 2014).
Virus quantification is the process of quantifying the amount of viral load in a given volume to calculate the virus concentration. Quantification of a virus enables to detection of its absolute accumulation rather than the presence/absence of the pathogen, so that protective measures can be taken at an early stage (Rubio et al., 2020). Quantification studies conducted by real-time PCR are generally carried out using a hydrolysis probe (TaqMan) or SYBR Green fluorescence dye (Kokane et al., 2021). Comparatively, SYBR Green-based real-time PCR is a cheaper reporter system than a probe due to binding to the small channel of double-stranded DNA and emits fluorescence associated with the quantification of amplified products existing in the target (Levin, 2004; Kokane et al., 2021). Several methods have been developed to quantify many organisms infecting different hosts using qRT-PCR (Santhosh et al., 2007; Bhullar et al., 2014; Mahmoudian et al., 2011; Bayraktar et al., 2016; Özer and Bayraktar, 2019; Olveira et al., 2021). Recently, identification and quantification studies by qRT-PCR were carried out by Chung et al. (2021) on patients infected with SARS-COV-2 (COVID-19). Several plant viruses have also been quantified using the qRT-PCR approach, including cucumber vein yellowing virus (CVYV) (Picó et al., 2005), barley yellow dwarf virus (BYDV) (Jarosová and Kundu, 2010), wheat yellow mosaic virus (WYMV) (Liu et al., 2013), different grapevine viruses (Fajardo et al., 2017), tomato spotted wilt virus (TSWV) (Soler et al., 2015), and cassava brown streak disease (CBSD) (Shirima et al., 2017). However, no study is available in the literature for the detection and quantification of BCMV in host tissues based on qRT-PCR assay.
The present study aimed to; i) identify resistance sources of BCMV NL-4 (PGVII) strain in the genetic germplasm using pathogenicity tests, ii) develop an SYBR Green-based qRT-PCR assay targeting the coat protein (CP) sequence of a BCMV NL-4 strain to assess the accumulation of the virus in various plant tissues, and iii) evaluate this new technique for selection resistant genotypes at early stages of viral infection. The result of the study would help breeders who are improving bean resistance and virologists trying to understand the host-pathogen interactions.
2 Materials and Methods
2.1 Virus source and plant material
The isolate of BCMV described as NL-4 strain was maintained in the common bean cultivar Kantar-05. The BCMV infection on the host plant was confirmed by conventional RT-PCR and partially sequenced through the coat protein gene as described in Traykova et al. (2008). Twenty common bean genotypes (Supplementary Table S1), which were previously characterized in terms of morphologically and agronomically according to IPGRI (International Plant Genetic Resources Institute) and EU-CPVO (European Union Community Plant Variety Office), were used in the study for pathogenicity tests. The cultivars Kantar-05 and Yakutiye-98, provided by the Research Institutes of the Republic of Turkey Ministry of Agriculture and Forestry, were included in the experiment as susceptible and resistant check lines for BCMV NL-4 strain, respectively (Sökmen et al., 2012).
2.2 Virus inoculation, experimental design, and evaluation of disease severity
All genotypes were planted in 20 cm pots containing a sterilized mixture of soil, perlite, and sand (1:1:1, v:v) and maintained in a growth chamber under a 16:8 h L:D photoperiod at 25°C and 70% humidity. The mechanic inoculation was carried out with 3 replications, following full deployment of the cotyledon leaves of 10-day-old plants, of which the first trifoliate leaves were observed. A total of 0.3 g of an infected leaf sample was ground in a mortar with a pestle with 2 ml of PBS (4°C) and then used to mechanical inoculate leaves dusted with carborundum powder. Inoculated plants were inspected daily for symptoms during the experiment. After 25 days post-inoculation (pi), symptoms on common bean genotypes were assessed using a 0–3 scale: 0 = no symptoms, 1 = mild foliar symptoms, 2 = moderate foliar symptoms, and 3 = severe distortion and malformation, which was developed by Odu et al. (2004) for Potyviruses. Two genotypes were selected as the most susceptible and resistant after the pathogenicity assay for further studies.
2.3 Preparing hoagland solution and sampling plant material for qRT-PCR analyses
Seeds of two genotypes selected by the pathogenicity assay were surface sterilized in a 0.5% NaOCl solution for 2 min and placed on two layers of filter paper in 100 × 15 mm plastic Petri dishes containing 5 ml of sterile distilled water. After 4 days, pre-germinated seeds were transferred to a pot filled with Hoagland nutrient solution (Epstein, 1972). Both genotypes were inoculated with the virus isolate and kept in a growth room under the conditions described above. The nutrient solution was refreshed in 48 h periodically. Samples of fully developed first trifoliate leaves and roots were collected from inoculated and control plants at 3-, 6-, and 9-days pi and immediately transferred into liquid nitrogen. The samples were kept at −132°C for long-term storage after RNA isolation. A detailed schematic representation of experimental design and sampling is given in Figure 1.
2.4 Primer design for qRT-PCR
The coat protein sequences of the BCMV NL-4 isolate used in all biological experiments were obtained according to Traykova et al. (2008) and deposited in GenBank under an accession number (OL741709). When designing primers, the 362 bp length coat protein sequence of BCMV NL-4 was used as input data for the NCBI primer design tool (https://www.ncbi.nlm.nih.gov/tools/primer-blast/). The tool-generated primers (Supplementary Table S2) were manually selected based on amplicon size, melting temperature, position, GC content, and annealing temperatures. The results were checked on the Beacon Designer (http://free.premierbiosoft.com) and mFold (http://www.idtdna.com/Scitools/Applications/mFold/) platforms. Comparative controls were also attempted using the BLASTn on NCBI primer design tool to check the specificity of the primers with other related common bean infecting Potyvirus, bean common mosaic necrosis virus (BCMNV), and probable hosts of BCMV to reduce the cross-reactivity due to sequence similarities.
2.5 RNA extraction, DNase treatment, and cDNA synthesis
Total RNA was extracted from 150 mg of infected leaves and roots using NucleoZOL RNA extraction solution (MACHEREY-NAGEL GmbH & Co., Dueren, Germany) according to the manufacturer’s instructions. A total of 8 µg RNA was treated with DNase I (RNase-free, supplied with MnCl2) (Thermo Fischer Scientific, Waltham, MA, United States) according to the manufacturer’s instructions. Complementary DNA (cDNA) was synthesized from 2 µg of total RNA using Thermo Scientific RevertAid First Strand cDNA Synthesis Kit (Thermo Fischer Scientific, Waltham, MA, United States) in a final volume of 20 µL based on the manufacturer’s instructions with the addition of oligo (dT)18 primer that anneals selectively to the poly (A) tail of viral RNA. Diluted cDNA samples were stored at −20°C for further quantification studies. Nucleic acid measurements were conducted with a DS-11 FX + series spectrophotometer (Denovix Inc., Wilmington, DE, United States).
2.6 SYBR green qRT-PCR and primer efficiency
The qRT-PCR was performed in the CFX Connect Real-Time PCR System (Bio-Rad, Hercules, CA, United States) using a 25 μL reaction mix, including 12.5 μL of RealQ Plus 2x Master Mix Green without ROX (Ampliqon, Odense, Denmark), 0.75 μL each of primers (10 μM), 1 μL of cDNA, and 10 μL of RNase-free ddH2O. The reaction was carried out under the following conditions: one cycle at 95°C for 15 min for activation of the TEMPase hot-start enzyme, followed by 40 cycles of 95°C for 15 s, 61°C for 45 s, and 72°C for 20 s. The Ct values were calculated by the CFX Maestro Software (Bio-Rad, Hercules, CA, United States). The samples were subjected to melting curve analysis by heating the samples from 65 to 95°C following the final cycle of the PCR to detect specific and non-specific PCR products. Each sample was examined using three technical duplicates, and all plates contained virus-infected positive (with Kantar-05 and Yakutiye), healthy plant RNA, and no template samples as controls. The five-fold cDNA dilution series was used to determine the primer efficiency and standard curves. The qRT-PCR experiment was performed according to the conditions described above.
2.7 Data analyses
The analysis of variance (ANOVA) was analysed for disease severity for all genotypes and CT values of resistant and sensitive genotypes at different time points and followed by Least Significant Test at p ≤ 0.01 with SAS Statistical Software (SAS Institute Inc., Cary, NC, United States). Then, the data was transferred to GraphPad Prism version 6.04 for Windows (GraphPad Software, San Diego, CA, United States) and visualized as graphics.
3 Results
3.1 Host suitability
Various symptoms, including dwarfing, wrinkling, and mosaic, were observed on common bean genotypes, confirming BCMV infection (Figures 2A–C). The BRS-22 genotype showed the first symptoms caused by the viral inoculation at 14 days pi, and severe mosaic symptoms on this genotype were observed at 25 days pi (Figure 2D). However, no viral symptoms were observed in the genotype YLV-14 due to BCMV infection (Figure 2E). Mild foliar symptoms occurred on 7 genotypes, while moderate foliar and wrinkling symptoms were observed on 11 genotypes. All genotypes were symptomatic (except for YLV-14), and the results of statistical analyses also showed that the disease severity level of YLV-14 and BRS-22 were similar to those recorded for resistance (Yakutiye-98) and susceptible (Kantar-05) check lines, respectively. The classification of common bean genotypes and incidence of symptom types were presented in Table 1. Therefore, BRS-22 and YLV-14 were found to be the most susceptible and resistant genotypes against BCMV NL-4 based on pathogenicity tests and selected for quantification studies.
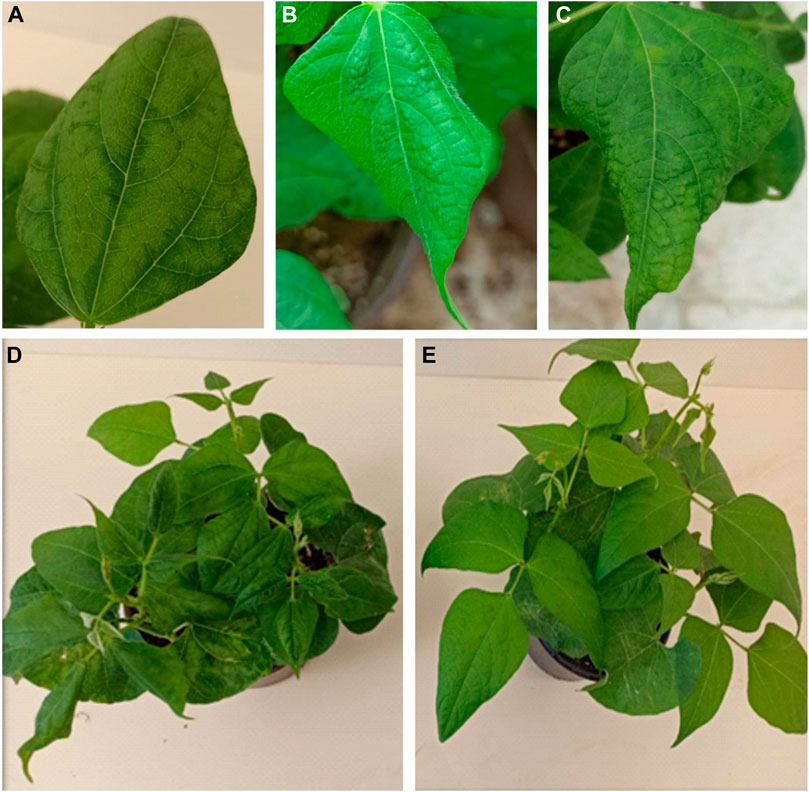
FIGURE 2. Different types of symptoms observed on common bean leaves 25 days pi BCMV NL-4. (A): mosaic symptom, (B,C): wrinkling symptoms with mosaic, (D): dwarfing with severe distortion and malformation on BRS-22, (E): no visible symptoms on YLV-14.
3.2 SYBR green qRT-PCR and primer efficiency
The primer set CPF02F (5′-ATCGGATCGAGCAAGAGAAGC-3′) and CPF02R (5′-GTCCCTTGCAGTGTGCCTTT-3′) showed a single amplicon (Figure 3A) with a sharp melt curve and were selected for thorough testing in the present study. The primers amplified a 133 bp product from the tissues from BCMV inoculated plants but not from uninoculated controls plants. No primer dimers were detected in the melting curve analysis (Figure 3B). Melting curve analysis showed that the tested primers amplified a single peak with a distinct melting point of 82°C in case of the presence of BCMV RNA (Figure 3C). The amplified products were confirmed by agarose gel electrophoresis (Figure 3D). The efficiency of the primer set was calculated from the standard curve slopes using the CFX Maestro Software and visualized using GraphPad Prism. The cDNA dilution series indicated high efficiency (0.93) and high linearity with R2 > 0.99 (Figure 4).
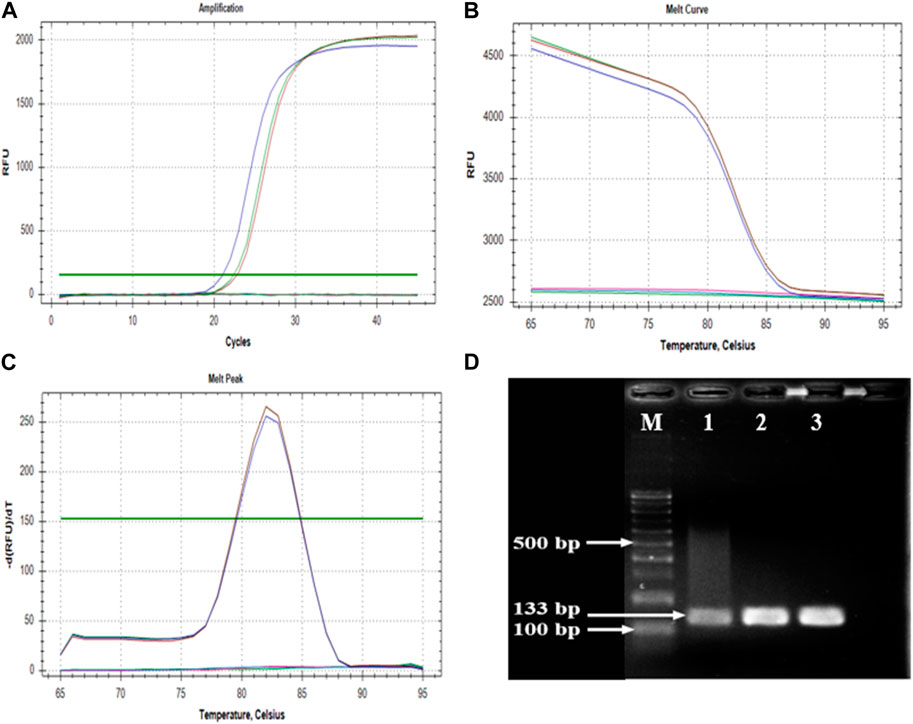
FIGURE 3. Parameters of amplification and melting analyses. (A); sharp amplification peak confirms the positivity, (B,C); melting curve results indicates the specificity, (D); agarose gel photo of an amplified 133 bp product. M: GeneRuler 100 bp DNA Ladder (Thermo Fischer Scientific, Waltham, MA, United States), 1, 2, and 3: cDNAs of BRS-22 genotype in three replicate.
3.3 Determination of viral load by qRT-PCR
Trifoliate leaves and roots of BCMV inoculated plants were sampled to find out the viral accumulation at different time points from BRS-22 and YLV-14 genotypes. The significant differences among Ct values were observed between genotypes at each time point regardless of tissue. The variation in Ct values of root tissue of susceptible and resistant genotypes was significant according to the ANOVA results (F = 123.69; LSD0.01 = 2.75) (Figure 5). The level of Ct values of the amplicon determining BCMV at 3-, 6-, and 9-days pi was found as 23.21, 21.47, and 23.47 for root tissues of BRS-22, respectively. Besides, the level of Ct values was determined as 35.28 at 3 days, 34.88 at 6 days, and 36.44 at 9 days for root tissues of YLV-14. However, there was no significant variation among Ct values at different time points for each genotype. The Ct values of BCMV-infected susceptible and resistant genotype’s roots were separated and placed in the same groups according to the LSD0.01 test. Generally, the Ct values of the susceptible genotype at different time points were lower than the resistant genotype’s Ct values. These results revealed that the accumulation of BCMV NL-4 strain in the root tissues was considerably higher in BRS-22 than in YLV-14.
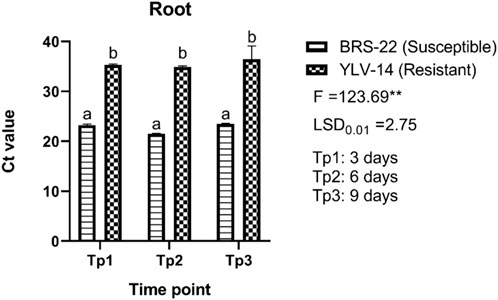
FIGURE 5. The levels of accumulation of BCMV by qRT-PCR in the roots of BRS-22 and YLV-14 genotypes at different time points. Levels not connected by the same letter are significantly different Ct values between genotypes based on LSD test at the 1% level.
For leaf tissues of genotypes, there were also found significant differences among Ct values, (F = 2224.19; LSD0.01 = 0.59) (Figure 6). The Ct values ranged from 17.19 (BRS-22, 9 days pi) to 32.32 (YLV-14, 9 days pi) for leaf tissues. The level of Ct values for leaf tissues of BRS-22 gradually and significantly decreased at each time point during BCMV infection, which indicates a sharp increase in virus accumulation. No significant variations among Ct values for leaf tissues were recorded at different time points for YLV-14 after inoculation. All Ct values obtained from leaf tissues of YLV-14 at different time points were placed statistically in the same group. Here, these variations between the Ct values of susceptible and resistant genotypes for BCMV accumulation were consistent with the result of the pathogenicity test.
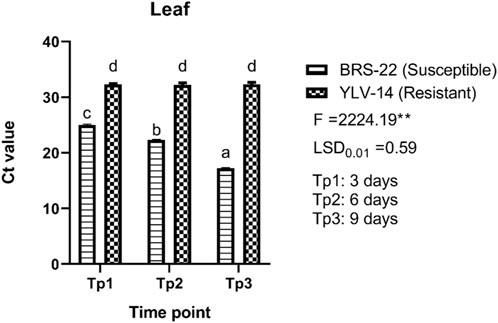
FIGURE 6. The levels of accumulation of BCMV by qRT-PCR in the leaves of BRS-22 and YLV-14 genotypes at different time points. Levels not connected by the same letter are significantly different Ct values between genotypes based on LSD test at the 1% level.
4 Discussion
Sustainable agriculture and crops are affected by abiotic and biotic stresses, including viruses, that cause millions of dollars’ worth of yield losses every year (Mumford et al., 2016; Yılmaz et al., 2021). Identifying plants infected with new viruses and the increase of virus population in agricultural areas or a host are major factors in controlling plant viruses. Disease management strategies such as cultivating resistance varieties, controlling plant material transfer, and pursuing new variants are generally preferable strategies to reduce the harms caused by plant-pathogenic viruses (Anderson et al., 2004; Jones, 2009; Elena et al., 2014).
BCMV is one of the most prevalent viruses infecting common beans and a variety of other legume hosts (Morales, 2006). The yield reduction caused by BCMV might reach up to 100% (Damayanti et al., 2008; Saqib et al., 2010; Singh and Schwartz, 2010; Verma and Gupta, 2010; Li et al., 2014). The use of resistant cultivars to the pathogen plays an active role in reducing the virus’s damages. In the present study, twenty common bean genotypes and two check lines were inoculated with the BCMV NL-4 strain to understand the host suitability against the pathogen. Inoculated common bean genotypes exhibited various symptoms, including wrinkling, mosaic, and dwarfing with severe distortion and malformation changing on a scale from mild foliar to intense mosaic. Several types of symptoms in literature have been reported on BCMV infected common beans, such as mosaic, leaf malformation, stunting (Drijhout et al., 1978), vein clearing, mottling, leaf curling, and chlorosis (Morales and Bos, 1988; Kılıç et al., 2020). The symptoms observed in this study on BCMV-infected genotypes were in accordance with the symptom types previously reported in the studies mentioned above. In addition, this paper has figured out the current position of 20 advanced common bean genotypes demonstrating resistance and susceptibility to BCMV, which were conducted in line with previous studies to assess the presence of BCMV resistance in different genotypes (Mukeshimana et al., 2005; Bello et al., 2014; Boersma et al., 2014; Yeken et al., 2018; Palacıoğlu et al., 2021). The promising genotype YLV-14 resistant to BCMV NL-4 strain can also be evaluated in various classical and molecular breeding studies such as crossing, QTLs, MAS, GWAS, and functional genomics to accelerate the crop improvement. To the best of our knowledge, there was no developed scale related to BCMV symptom severity on host plants. Here, we adapted for the first time a 0–3 scale developed for Yam mosaic virus (YMV) by Odu et al. (2004) to successfully classify the symptom severity of BCMV. Mangeni et al. (2020) also used this scale in determining the disease severity of BCMNV, another Potyvirus disease of common bean, and these results are consistent with this study reported here.
Plant RNA viruses have the indisputable potential for genetic variety, fast development, and adaptability to new environmental conditions (Abadkhah et al., 2020). BCMV, a typical member of Potyviruses, has all the features of the genus, such as high mutation ability, recombination, and formation of various strains (Gibbs et al., 2020). Previous studies identified different pathogroups and strains in BCMV classification (Feng et al., 2015). When the nucleotide sequences of all strains and pathogroups of BCMV in the GenBank database were downloaded and aligned, it was considered that it would be difficult to design an SYBR Green-based real-time PCR primer sets that would enable the amplification of all strains due to the limited conserved genomic regions. Therefore, we used a BCMV NL-4 isolate, whose CP sequence information is available in GenBank (OL741709), to stimulate bean-BCMV interaction and investigate viral accumulation in root and leaf tissues of susceptible and resistant genotypes.
Identifying viruses at the molecular level is unquestionably important in plant virology. A huge number of papers have focused on the design, development, and validation of PCR-derived techniques, with a particular emphasis on real-time PCR in recent years (Olveria et al., 2021). Several studies have been reported to detect BCMV using different techniques such as DAS-ELISA, RT-PCR, and sequencing (Vetten et al., 1992; Xu and Hampton, 1996; Arlı-Sökmen et al., 2016). However, no publications are available for developing a qRT-PCR assay to detect and quantify BCMV in common bean. Here, we established an SYBR Green-based qRT-PCR methodology for BCMV-NL-4 detection and quantification for the first time. This novel developed an assay for BCMV detection has simplified viral quantification, which is increasingly gaining importance as a basic supplement in research, especially early detection of the pathogen. The developed qRT-PCR assay provided various advantages over traditional RT-PCR assays, including increased sensitivity, specificity, and quantitative quantification, as well as ease of standardization for the evaluation of these genotypes. The results that related to the specificity, sensitivity, and quantification in BCMV detection were in accordance with the previous qRT-PCR assays developed for other plant viruses (Mumford et al., 2000; Picó et al., 2005; López et al., 2006; Ling et al., 2007; Hongyun et al., 2008; Ruiz-Ruiz et al., 2009a; Debreczeni et al., 2011; Ferriol et al., 2011; Sharma and Dasgupta, 2012; Herrera-Vásquez et al., 2015; MacKenzie et al., 2015). The designing of primers is a key factor for a sequence-based detection system, and the CP gene is a good target for viral detection due to its specificity (Ghosh et al., 2020). The qRT-PCR assay established in this study amplified a 133 bp fragment of CP of BCMV-NL4 that was used to quantify BCMV in leaves and roots at different time points. We use SYBR Green technology based on binding the fluorescent dye to dsDNA since it is easy to use and relatively cost-benefit than TaqMan technology based on exonuclease activity of Taq polymerase enzyme and dual-labelled oligonucleotide (Mahmoudian et al., 2011). The primers utilized in the assay effectively amplified the target gene, and the results showed that SYBR Green-based qRT-PCR is a fast and sensitive way of directly detecting and quantifying BCMV from leaves and roots. The newly designed primer set targeting the CP of BCMV NL-4 allowed the detection of BCMV with satisfactory E, R2, and slope values, which are reliable with the established standards (Broeders et al., 2014), verifying the accuracy and linear response of the experiments over a wide range of dilutions and implying the absence of PCR inhibitors.
It is often time-consuming and difficult to discover resistance genes, establishing long-period pathogenicity tests, especially when the BCMV mosaic strains employed as a screening inoculum belong to pathogenicity groups other than those identified by Drijfhout (1978) and Feng et al. (2018). In the current study, it is considered that the accumulation of the pathogen in the initial stage of infection can provide valuable information for the selection of cultivars. Based on this data, we imagined that the severity of symptoms exhibited by infected plants might be related to changes in genomic regions of pathogens associated with replication, virus movement, and processes that control the level of virus accumulation in infected plants. Significant differences were found between Ct values obtained at different time points in root and leaf tissue of susceptible and resistant genotypes. Mainly, the Ct values were found to be higher in the resistant genotype than the susceptible genotype in both tissues. The presence of the pathogen in the root 3 days pi in both susceptible and resistant cultivars undoubtedly indicates that the pathogen causes systemic infection. Agrios (2005) stated that when a virus infects a plant, it takes 2–5 days or more for the virus to travel out of an infected leaf, and once in the phloem, the virus spreads quickly toward developing areas (apical meristems) or other food-utilizing sections of the plant, such as tubers and rhizomes. The Ct values obtained from both roots and leaves confirm active viral movement in the plant in accordance with Agrios (2005), showing the pathogen is moving in the phloem. Some papers have been reported indicating the viral movement is triggered by viral determinants of phloem viruses. Following the symptom onset and viral accumulation, Roberts et al. (2007) proved a close link between cauliflower mosaic virus (CaMV) infection and patterns of photoassimilate distribution in sink organs, indicating the virus movement. Similarly, melon necrotic spot virus (MNSV) first moves from the cotyledons to the roots via the external phloem before moving to the shoot apex via the internal phloem (Gosalvez-Bernal et al., 2008). In contrast, some host determinants promote or restrict virus movement in the phloem. Host factors, in addition to viral components, can be recruited to aid virus phloem movement. Cellular proteins may be involved in the creation of viral complexes and can promote the effective transport of such complexes, as well as acting as stabilizing proteins or as protective agents against plant defence systems (Hipper et al., 2013).
The qRT-PCR results of this study revealed that BRS-22 had more accumulation of BCMV NL-4 strain than YLV-14 at different time points in both tissues. This scenario is thought to be generated by the plant’s natural defensive system or by the features of the variety, as stated in Hipper et al. (2013). For CVYV in cucumber, real-time PCR yielded repeatable findings of susceptible and resistant landraces, and the quantity of target viral load detected in genotypes was significantly varied, demonstrating variances in viral accumulation linked to their different levels of resistance (Picó et al., 2005). Jiang et al. (2013) measured the viral load of HTNV (Hantaan virus) by using an SYBR Green I-based one-step qRT-PCR technique and observed low viral titres in samples with high Ct values. Campos et al. (2019) reported that qRT-PCR based on SYBR Green methodology produced high Ct values in low titres of olive viruses. Similarly, de Freitas-Vanzo et al. (2021) used the qRT-PCR to quantify BGMV titre in host plants and found variations in symptom expression and BGMV titre between cultivars and lineages at 10 and 25 pi and the bean cultivar “Tangará” as reported in YLV-14 in this research, had a higher Ct value but showed less symptom severity than the other cultivars. Similar studies have also been carried out to investigate viral load that indicated the resistance and susceptibility of many hosts against various plant viruses, including BYDV, WYMV, TSWV, CBSD, and various grapevine viruses, which results were in agreement with the outcomes reported in this study (Gil salas et al., 2009; Jarošová and Kundu, 2010; Liu et al., 2013; Soler et al., 2015; Fajardo et al., 2017; Shirima et al., 2017). Based on this data, the established assay provides a reliable quantitative tool for accumulating BCMV in the tissues of susceptible and resistant genotypes at various time periods.
In summary, the developed qRT-PCR provided a high degree of reliability, sensitivity, and reproducibility for the prediction of virus accumulation in infected susceptible and resistant cultivars as an effective tool for early detection of diseases, enabling to predict of the genotypes in the early stage of infection. This novel assay may detect viral load differences between highly susceptible and non-symptomatic genotypes and may have utility for plant breeding systems. The results of the study will provide new insights into host-pathogen interactions. In addition, this method will contribute significantly to detection methods for BCMV in different research areas and will provide a reference for future studies.
Data availability statement
The original contributions presented in the study are included in the article/Supplementary Material, further inquiries can be directed to the corresponding authors.
Author contributions
AÇ, OE, and MZY: Conceptualization, data curation, formal analysis, writing- original draft preparation. VÇ: Invesigation, formal analysis, supervision. FSB, YSC, and GÖ: Validation, writing—review and editing, visualization, FSB, YSC, and YK: funding acquisition. All authors read and approved the manuscript.
Funding
This research was funded by Bolu Abant Izzet Baysal University (Project ID: 2022.10.06.1550) and Basic Science Research Program supported this research through the National Research Foundation of Korea (NRF), funded by the Ministry of Education (2019R1A6A1A11052070).
Acknowledgments
We would like to thank the Scientific and Technological Research Council of Turkey (TUBITAK; Project ID: 115R042) and the Research Institutes of the Republic of Turkey Ministry of Agriculture and Forestry for supplying plant materials used in this study. The authors also thank Prof. Miray ARLI SÖKMEN for supplying BCMV NL-4 isolate.
Conflict of interest
The authors declare that the research was conducted in the absence of any commercial or financial relationships that could be construed as a potential conflict of interest.
Publisher’s note
All claims expressed in this article are solely those of the authors and do not necessarily represent those of their affiliated organizations, or those of the publisher, the editors and the reviewers. Any product that may be evaluated in this article, or claim that may be made by its manufacturer, is not guaranteed or endorsed by the publisher.
Supplementary material
The Supplementary Material for this article can be found online at: https://www.frontiersin.org/articles/10.3389/fgene.2023.1136794/full#supplementary-material
References
Abadkhah, M., Hajizadeh, M., and Koolivand, D. (2020). Global population genetic structure of Bean common mosaic virus. Arch. Phytopathol. 53 (5-6), 266–281. doi:10.1080/03235408.2020.1743525
Abrahamian, P., Seblani, R., Sobh, H., and Abou-Jawdah, Y. (2013). Detection and quantitation of two cucurbit criniviruses in mixed infection by real-time RT-PCR. J. Virol. Methods 193 (2), 320–326. doi:10.1016/j.jviromet.2013.06.004
Anderson, P. K., Cunningham, A. A., Patel, N. G., Morales, F. J., Epstein, P. R., and Daszak, P. (2004). Emerging infectious diseases of plants: Pathogen pollution, climate change and agrotechnology drivers. Trends Ecol. Evol. 19 (10), 535–544. doi:10.1016/j.tree.2004.07.021
Arli-Sokmen, M., Deligoz, I., and Kutluk-Yilmaz, N. D. (2016). Characterization of Bean common mosaic virus and Bean common mosaic necrosis virus isolates in common bean growing areas in Turkey. Eur. J. Plant Pathol. 146 (1), 1–16. doi:10.1007/s10658-016-0886-x
Bayraktar, H., Özer, G., Aydoğan, A., and Palacıoğlu, G. (2016). Determination of Ascochyta blight disease in chickpea using real-time PCR. J. Plant Dis. Prot. 123 (3), 109–117. doi:10.1007/s41348-016-0017-0
Bello, M. H., Moghaddam, S. M., Massoudi, M., McClean, P. E., Cregan, P. B., and Miklas, P. N. (2014). Application of in silico bulked segregant analysis for rapid development of markers linked to Bean common mosaic virus resistance in common bean. BMC Genomics 15 (1), 903. doi:10.1186/1471-2164-15-903
Bhullar, S. S., Chandak, N. H., Purohit, H. J., Taori, G. M., Daginawala, H. F., and Kashyap, R. S. (2014). Determination of viral load by quantitative real-time PCR in herpes simplex encephalitis patients. Intervirology 57 (1), 1–7.
Boersma, J. G., Conner, R. L., Balasubramanian, P. M., Navabi, A., Yu, K., and Hou, A. (2014). Combining resistance to common bacterial blight anthracnose and bean common mosaic virus into Manitoba-adapted dry bean (Phaseolus vulgaris L) cultivars. Can. J. Plant Sci. 94 (2), 405–415. doi:10.4141/cjps2013-281
Boonham, N., Kreuze, J., Winter, S., van der Vlugt, R., Bergervoet, J., Tomlinson, J., et al. (2014). Methods in virus diagnostics: From ELISA to next generation sequencing. Virus Res. 186, 20–31. doi:10.1016/j.virusres.2013.12.007
Borrelli, V. M., Brambilla, V., Rogowsky, P., Marocco, A., and Lanubile, A. (2018). The enhancement of plant disease resistance using CRISPR/Cas9 technology. Front. Plant Sci. 9, 1245. doi:10.3389/fpls.2018.01245
Broeders, S., Huber, I., Grohmann, L., Berben, G., Taverniers, I., Mazzara, M., et al. (2014). Guidelines for validation of qualitative real-time PCR methods. Trends Food Sci. Technol. 37 (2), 115–126. doi:10.1016/j.tifs.2014.03.008
Campos, M. D., Zellama, M. S., Varanda, C., Materatski, P., Peixe, A., Chaouachi, M., et al. (2019). Establishment of a sensitive qPCR methodology for detection of the olive-infecting viruses in Portuguese and Tunisian orchards. Front. Plant Sci. 10, 694. doi:10.3389/fpls.2019.00694
Carrasco, P., Daròs, J. A., Agudelo-Romero, P., and Elena, S. F. (2007). A real-time RT-PCR assay for quantifying the fitness of Tobacco etch virus in competition experiments. J. Virol. Methods 139 (2), 181–188. doi:10.1016/j.jviromet.2006.09.020
Chatzivassiliou, E. K. (2021). An annotated list of legume-infecting viruses in the light of metagenomics. Plants 10 (7), 1413. doi:10.3390/plants10071413
Chung, Y. S., Lee, N. J., Woo, S. H., Kim, J. M., Kim, H. M., Jo, H. J., et al. (2021). Validation of real-time RT-PCR for detection of SARS-CoV-2 in the early stages of the COVID-19 outbreak in the Republic of Korea. Sci. Rep. 11 (1), 14817–14818. doi:10.1038/s41598-021-94196-3
Damayanti, T. A., Susilo, D., Nurlaelah, S., Sartiami, D., Okuno, T., and Mise, K. (2008). First report of Bean common mosaic virus in yam bean [Pachyrhizus erosus (L) Urban] in Indonesia. J. Gen. Plant Pathol. 74 (6), 438–442. doi:10.1007/s10327-008-0122-4
De Freitas-Vanzo, A. T., Da Silva, C. D. C., De Novaes, T. G., Mazzieri Walz, D., Marcelino Guimaraes, F. C., Kuwahara, M. K., et al. (2021). Evaluation of disease severity caused by Bean golden mosaic virus in different bean cultivars. Can. J. Plant Pathol. 43 (1), 172–178. doi:10.1080/07060661.2020.1771618
Debreczeni, D. E., Rubio, L., Aramburu, J., Lopez, C., Galipienso, L., Soler, S., et al. (2014). Transmission of Tomato spotted wilt virus isolates able and unable to overcome tomato or pepper resistance by its vector Frankliniella occidentalis. Ann. Appl. Biol. 164 (2), 182–189. doi:10.1111/aab.12090
Debreczeni, D. E., Ruiz-Ruiz, S., Aramburu, J., Lopez, C., Belliure, B., Galipienso, L., et al. (2011). Detection discrimination and absolute quantitation of Tomato spotted wilt virus isolates using real time RT-PCR with TaqMan® MGB probes. J. Virol. Methods 176 (1-2), 32–37. doi:10.1016/j.jviromet.2011.05.027
Drijfhout, E. (1978). Genetic interaction between Phaseolus vulgaris and Bean common mosaic virus with implications for strain identification and breeding for resistance. Agricultural research report, 98.
Drijfhout, E., Silbernagel, M. J., and Burke, D. W. (1978). Differentiation of strains of bean common mosaic virus. J. Plant Pathol. 84 (1), 13–26. doi:10.1007/bf01978099
El-Sawy, M. A., Mohamed, H. A. E., and Elsharkawy, M. M. (2014). Serological and molecular characterisations of the Egyptian isolate of Bean common mosaic virus. Arch. Phytopathol. 47 (12), 1431–1443. doi:10.1080/03235408.2013.845470
Elena, S. F., Fraile, A., and García-Arenal, F. (2014). Evolution and emergence of plant viruses. Adv. Virus Res 88, 161–191. doi:10.1016/b978-0-12-800098-4.00003-9
Epstein, E. (1972). Mineral nutrition of plants: Principles and perspectives. New York, NY: J. Wiley and Sons, Inc, 68–82.
Espy, M. J., Uhl, J. R., Sloan, L. M., Buckwalter, S. P., Jones, M. F., Vetter, E. A., et al. (2006). Real-time PCR in clinical microbiology: Applications for routine laboratory testing. Clin. Microbiol. Rev. 19 (1), 165–256. doi:10.1128/CMR.19.1.165-256.2006
Fajardo, T. V. M., Vanni, M. F., and Nickel, O. (2017). Absolute quantification of viruses by TaqMan real-time RT-PCR in grapevines. Cienc. Rural. 47 (6). doi:10.1590/0103-8478cr20161063
Feng, X., Myers, J. R., and Karasev, A. (2015). Bean common mosaic virus isolate exhibits a novel pathogenicity profile in common bean overcoming the bc-3 resistance allele coding for the mutated eIF4E translation initiation factor. Phytopathology 105 (11), 1487–1495. doi:10.1094/phyto-04-15-0108-r
Feng, X., Orellana, G. E., Myers, J. R., and Karasev, A. V. (2018). Recessive resistance to Bean common mosaic virus conferred by the bc-1 and bc-2 genes in common bean (Phaseolus vulgaris) affects long-distance movement of the virus. Phytopathology 108 (8), 1011–1018. doi:10.1094/phyto-01-18-0021-r
Feng, X., Poplawsky, A. R., Nikolaeva, O. V., Myers, J. R., and Karasev, A. V. (2014). Recombinants of Bean common mosaic virus (BCMV) and genetic determinants of BCMV involved in overcoming resistance in common bean. J. Phytopathol. 104 (7), 786–793. doi:10.1094/phyto-08-13-0243-r
Ferriol, I., Rubio, L., Pérez-Panadés, J., Carbonell, E. A., Davino, S., and Belliure, B. (2013). Transmissibility of Broad bean wilt virus 1 by aphids; İnfluence of virus accumulation in plants virus genotype and aphid species. Ann. Appl. Biol. 162 (1), 71–79. doi:10.1111/j.1744-7348.2012.00579.x
Ferriol, I., Ruiz-Ruiz, S., and Rubio, L. (2011). Detection and absolute quantitation of Broad bean wilt virus 1 (BBWV-1) and BBWV-2 by real time RT-PCR. J. Virol. Methods 177 (2), 202–205. doi:10.1016/j.jviromet.2011.08.003
Galipienso, L., Janssen, D., Rubio, L., Aramburu, J., and Velasco, L. (2013). Cucumber vein yellowing virus isolate-specific expression of symptoms and viral RNA accumulation in susceptible and resistant cucumber cultivars. Crop Prot. 43, 141–145. doi:10.1016/j.cropro.2012.08.004
Ghosh, D. K., Kokane, A. D., Kokane, S. B., Tenzin, J., Gubyad, M. G., Wangdi, P., et al. (2021). Detection and molecular characterization of ‘C andidatus liberibacter asiaticus’ and citrus tristeza virus associated with citrus decline in Bhutan. Phytopathology 111, 870–881. doi:10.1094/PHYTO-07-20-0266-R
Gibbs, A. J., Hajizadeh, M., Ohshima, K., and Jones, R. A. (2020). The potyviruses: An evolutionary synthesis is emerging. Viruses 12 (2), 132. doi:10.3390/v12020132
Gil-Salas, F. M., Colyer, A., Boonham, N., Cuadrado, I. M., and Janssen, D. (2009). Resistance screening against Cucumber vein yellowing virus using a real-time (Taqman®) RT-PCR assay in cucumber (Cucumis sativus). Crop Prot. 28 (1), 109–112. doi:10.1016/j.cropro.2008.09.005
Gosalvez-Bernal, B., Genoves, A., Navarro, J. A., Pallas, V., and Sanchez-Pina, M. A. (2008). Distribution and pathway for phloem-dependent movement of Melon necrotic spot virus in melon plants. Mol. Plant Pathol. 9 (4), 447–461. doi:10.1111/j.1364-3703.2008.00474.x
Hall, R. (1994). Bean diseases bean pathogens bean disease control compendium of bean diseases. Minnesota, USA: APS press.
Hanssen, I. M., Gutiérrez-Aguirre, I., Paeleman, A., Goen, K., Wittemans, L., Lievens, B., et al. (2010). Cross-protection or enhanced symptom display in greenhouse tomato co-infected with different Pepino mosaic virus isolates. Plant Pathol. 59 (1), 13–21. doi:10.1111/j.1365-3059.2009.02190.x
Herrera-Vásquez, J. A., Rubio, L., Alfaro-Fernández, A., Debreczeni, D. E., Font-San-Ambrosio, I., Falk, B. W., et al. (2015). Detection and absolute quantitation of Tomato torrado virus (ToTV) by real time RT-PCR. J. Virol. Methods 221, 90–94. doi:10.1016/j.jviromet.2015.04.029
Hipper, C., Brault, V., Ziegler-Graff, V., and Revers, F. (2013). Viral and cellular factors involved in phloem transport of plant viruses. Front. Plant Sci. 4, 154. doi:10.3389/fpls.2013.00154
Hongyun, C., Wenjun, Z., Qinsheng, G., Qing, C., Shiming, L., and Shuifang, Z. (2008). Real time TaqMan RT-PCR assay for the detection of Cucumber green mottle mosaic virus. J. Virol. Methods 149 (2), 326–329. doi:10.1016/j.jviromet.2008.02.006
Jarošová, J., and Kundu, J. K. (2010). Validation of reference genes as internal control for studying viral infections in cereals by quantitative real-time RT-PCR BMC. Plant Biol. 10 (1), 1–9. doi:10.1186/1471-2229-10-146
Jiang, W., Yu, H. T., Zhao, K., Zhang, Y., Du, H., Wang, P. Z., et al. (2013). Quantification of Hantaan virus with a SYBR green I-based one-step qRT-PCR assay. PloS One 8 (11), e81525. doi:10.1371/journal.pone.0081525
Jones, R. A. (2009). Plant virus emergence and evolution: Origins new encounter scenarios factors driving emergence effects of changing world conditions and prospects for control. Virus Res. 141 (2), 113–130. doi:10.1016/j.virusres.2008.07.028
Kelly, J. D., Afanador, L., and Haley, S. D. (1995). Pyramiding genes for resistance to bean common mosaic virus. Euphytica 82 (3), 207–212. doi:10.1007/BF00029562
Kiliç, H. Ç., Kök, H., and Yardimci, N. (2020). Bean common mosaic virus and bean common mosaic necrosis virus infections in bean production areas in the Lakes Region of Turkey. EJOSAT 19, 386–392. doi:10.31590/ejosat.705686
Kokane, A. D., Lawrence, K., Kokane, S. B., Gubyad, M. G., Misra, P., Reddy, M. K., et al. (2021). Development of a SYBR Green-based RT-qPCR assay for the detection of Indian citrus ringspot virus. 3 Biotech. 11 (7), 359–412. doi:10.1007/s13205-021-02903-8
Kyrychenko, A., Shcherbatenko, I., and Mishchenko, L. (2019). BCMV-Ukr: Isolate of bean common mosaic virus revealed in Ukraine. Archives Phytopathology Plant Prot. 52 (11-12), 1005–1017. doi:10.1080/03235408.2019.1688448
Levin, R. E. (2004). The application of real-time PCR to food and agricultural systems a review. Food Biotechnol. 18, 97–133. doi:10.1081/FBT-120030386
Li, Y. Q., Liu, Z. P., Yang, K., Li, Y. S., Zhao, B., Fan, Z. F., et al. (2014). First report of Bean common mosaic virus infecting Azuki bean (Vigna angularis) in China. Plant Dis. 98 (7), 1017. doi:10.1094/PDIS-01-14-0064-PDN
Ling, K. S., Wechter, W. P., and Jordan, R. (2007). Development of a one-step immunocapture real-time TaqMan RT-PCR assay for the broad spectrum detection of Pepino mosaic virus. J. Virol. Methods 144 (1-2), 65–72. doi:10.1016/j.jviromet.2007.03.022
Liu, W., Zhao, X., Zhang, P., Mar, T. T., Liu, Y., Zhang, Z., et al. (2013). A one step real-time RT-PCR assay for the quantitation of Wheat yellow mosaic virus (WYMV). Virol. J. 10 (1), 173–179. doi:10.1186/1743-422X-10-173
López, R., Asensio, C., Guzman, M. M., and Boonham, N. (2006). Development of real-time and conventional RT-PCR assays for the detection of potato yellow vein virus (PYVV). J. Virol. Methods 136 (1-2), 24–29. doi:10.1016/j.jviromet.2006.03.026
MacKenzie, T. D., Nie, X., and Singh, M. (2015). “RT-PCR and real-time RT-PCR methods for the detection of potato virus Y in potato leaves and tubers,” in Plant virology protocols (New York, NY: Humana Press), 13–26.
Mahmoudian, A., Kirkpatrick, N. C., Coppo, M., Lee, S. W., Devlin, J. M., Markham, P. F., et al. (2011). Development of a SYBR Green quantitative polymerase chain reaction assay for rapid detection and quantification of infectious laryngotracheitis virus. Avian Pathol. 40 (3), 237–242. doi:10.1080/03079457.2011.553582
Malandraki, I., Beris, D., Isaioglou, I., Olmos, A., Varveri, C., and Vassilakos, N. (2017). Simultaneous detection of three pome fruit tree viruses by one-step multiplex quantitative RT-PCR. PLoS One 12 (7), e0180877. doi:10.1371/journal.pone.0180877
Mangeni, B. C., Were, H. K., Ndong’a, M., and Mukoye, B. (2020). Incidence and severity of bean common mosaic disease and resistance of popular bean cultivars to the disease in western Kenya. Journal of Phytopathology 168 (9), 501–515.
Miklas, P. N., Larsen, R. C., Riley, R., and Kelly, J. D. (2000). Potential marker-assisted selection for bc-1 2 resistance to bean common mosaic potyvirus in common bean. Euphytica 116 (3), 211–219. doi:10.1023/A:1004006514814
Morales, F. J. (1989). Bean common mosaic: Screening for disease resistance centro internacional de Agricultura tropical. Cali Colombia: CIAT. Publication No 33.
Morales, F. J., and Bos, L. (1988). Bean common mosaic virus AAB descriptions of plant viruses No 337 (No 73 revised).
Morales, F. J. (2006). “Common beans,” in Natural resistance mechanisms of plants to viruses. Editors G. Loebenstein, and J. P. Carr (Netherlands: Springer), 367–382.
Mortimer-Jones, S. M., Jones, M. G., Jones, R. A., Thomson, G., and Dwyer, G. I. (2009). A single tube quantitative real-time RT-PCR assay that detects four potato viruses simultaneously. J. Virol. Methods 161 (2), 289–296. doi:10.1016/j.jviromet.2009.06.027
Mukeshimana, G., Paneda, A., Rodríguez-Suárez, C., Ferreira, J. J., Giraldez, R., and Kelly, J. D. (2005). Markers linked to the bc-3 gene conditioning resistance to bean common mosaic potyviruses in common bean. Euphytica 144 (3), 291–299. doi:10.1007/s10681-005-7397-8
Mumford, R. A., Macarthur, R., and Boonham, N. (2016). The role and challenges of new diagnostic technology in plant biosecurity. Food Secu 8 (1), 103–109. doi:10.1007/s12571-015-0533-y
Mumford, R. A., Walsh, K., Barker, I., and Boonham, N. (2000). Detection of Potato mop top virus and Tobacco rattle virus using a multiplex real-time fluorescent reverse-transcription polymerase chain reaction assay. Phytopathology 90 (5), 448–453. doi:10.1094/phyto.2000.90.5.448
Odu, B. O., Asiedu, R., Hughes, J. D. A., Shoyinka, S. A., and Oladiran, O. A. (2004). Identification of resistance to Yam mosaic virus (YMV) genus Potyvirus in white Guinea yam (Dioscorea rotundata Poir). Field Crops Res. 89 (1), 97–105. doi:10.1016/j.fcr.2004.01.009
Olmos, A., Bertolini, E., Gil, M., and Cambra, M. (2005). Real-time assay for quantitative detection of non-persistently transmitted Plum pox virus RNA targets in single aphids. J. Virol. Methods 128 (1-2), 151–155. doi:10.1016/j.jviromet.2005.05.011
Olveira, J. G., Souto, S., Bandín, I., and Dopazo, C. P. (2021). Development and validation of a SYBR green real time PCR protocol for detection and quantification of nervous necrosis virus (NNV) using different standards. Animals 11 (4), 1100. doi:10.3390/ani11041100
Özer, G., and Bayraktar, H. (2019). Development of conventional and real-time PCR assays to detect Alternaria burnsii in cumin seed. Gesunde Pflanz. 71 (3), 205–212. doi:10.1007/s10343-019-00466-6
Palacıoğlu, G., Özer, G., Yeken, M. Z., Çiftçi, V., and Bayraktar, H. (2021). Resistance sources and reactions of common bean (Phaseolus vulgaris L) cultivars in Turkey to anthracnose disease. Genet. Resour. Crop Evol. 68, 3373–3381. doi:10.1007/s10722-021-01195-4
Pena, E. J., Ferriol, I., Sambade, A., Buschmann, H., Niehl, A., Elena, S. F., et al. (2014). Experimental virus evolution reveals a role of plant microtubule dynamics and TORTIFOLIA1/SPIRAL2 in RNA trafficking. PLoS One 9 (8), e105364. doi:10.1371/journal.pone.0105364
Picó, B., Sifres, A., and Nuez, F. (2005). Quantitative detection of Cucumber vein yellowing virus in susceptible and partially resistant plants using real-time PCR. J. Virol. Methods 128 (1-2), 14–20. doi:10.1016/j.jviromet.2005.03.010
Poojari, S., Alabi, O. J., Okubara, P. A., and Naidu, R. A. (2016). SYBR® Green-based real-time quantitative reverse-transcription PCR for detection and discrimination of grapevine viruses. J. Virol. Methods 235, 112–118. doi:10.1016/j.jviromet.2016.05.013
Roberts, K., Love, A. J., Laval, V., Laird, J., Tomos, A. D., Hooks, M. A., et al. (2007). Long-distance movement of Cauliflower mosaic virus and host defence responses in Arabidopsis follow a predictable pattern that is determined by the leaf orthostichy. New Phytol. 175 (4), 707–717. doi:10.1111/j.1469-8137.2007.02136.x
Rotenberg, D., Krishna Kumar, N. K., Ullman, D. E., Montero-Astúa, M., Willis, D. K., German, T. L., et al. (2009). Variation in Tomato spotted wilt virus titer in Frankliniella occidentalis and its association with frequency of transmission. Phytopathology 99 (4), 404–410. doi:10.1094/phyto-99-4-0404
Rubio, L., Galipienso, L., and Ferriol, I. (2020). Detection of plant viruses and disease management: Relevance of genetic diversity and evolution. Front. Plant Sci. 11, 1092. doi:10.3389/fpls.2020.01092
Ruiz-Ruiz, S., Ambrós, S., del Carmen Vives, M., Navarro, L., Moreno, P., and Guerri, J. (2009a). Detection and quantitation of Citrus leaf blotch virus by TaqMan real-time RT-PCR. J. Virol. Methods 160 (1-2), 57–62. doi:10.1016/j.jviromet.2009.04.012
Ruiz-Ruiz, S., Moreno, P., Guerri, J., and Ambrós, S. (2009b). Discrimination between mild and severe Citrus tristeza virus isolates with a rapid and highly specific real-time reverse transcription-polymerase chain reaction method using TaqMan LNA probes. Phytopathology 99 (3), 307–315. doi:10.1094/phyto-99-3-0307
Santhosh, S. R., Parida, M. M., Dash, P. K., Pateriya, A., Pattnaik, B., Pradhan, H. K., et al. (2007). Development and evaluation of SYBR Green I-based one-step real-time RT-PCR assay for detection and quantification of Chikungunya virus. J. Clin. Virol. 39 (3), 188–193. doi:10.1016/j.jcv.2007.04.015
Saqib, M., Nouri, S., Cayford, B., Jones, R. A. C., and Jones, M. G. K. (2010). Genome sequences and phylogenetic placement of two isolates of Bean common mosaic virus from Macroptilium atropurpureum in north-west Australia Australasian. Plant Pathol. 39 (2), 184–191. doi:10.1071/AP09080
Sharma, S., and Dasgupta, I. (2012). Development of SYBR Green I based real-time PCR assays for quantitative detection of Rice tungro bacilliform virus and Rice tungro spherical virus. J. Virol. Methods 181 (1), 86–92. doi:10.1016/j.jviromet.2012.01.018
Shirima, R. R., Maeda, D. G., Kanju, E., Ceasar, G., Tibazarwa, F. I., and Legg, J. P. (2017). Absolute quantification of cassava Brown streak virus mRNA by real-time qPCR. J. Virol. Methods 245, 5–13. doi:10.1016/j.jviromet.2017.03.003
Singh, S. P., and Schwartz, H. F. (2010). Breeding common bean for resistance to diseases: A review. Crop Sci. 50 (6), 2199–2223. doi:10.2135/cropsci2009.03.0163
Soler, S., Debreczeni, D. E., Vidal, E., Aramburu, J., López, C., Galipienso, L., et al. (2015). A new Capsicum baccatum accession shows tolerance to wild-type and resistance-breaking isolates of Tomato spotted wilt virus. Ann. Appl. Biol. 167 (3), 343–353. doi:10.1111/aab.12229
Soler-Garzón, A., McClean, P. E., and Miklas, P. N. (2021). Coding mutations in vacuolar protein-sorting 4 AAA+ ATPase endosomal sorting complexes required for transport protein homologs underlie bc-2 and new bc-4 gene conferring resistance to bean common mosaic virus in common bean. Front. Plant Sci. 12, 769247. doi:10.3389/fpls.2021.769247
Traykova, A., Hristova, D., and Ziegler, A. (2008). Diagnostics of Bean common mosaic virus in common bean (Phaseolus vulgaris L) seeds. Plant Sci. 45, 128–135.
Verma, P., and Gupta, U. P. (2010). Immunological detection of bean common mosaic virus in French bean (Phaseolus vulgaris L) leaves. Indian J. Microbiol. 50 (3), 263–265. doi:10.1007/s12088-010-0019-8
Vetten, H. J., Lesemann, D. E., and Maiss, E. (1992). Serotype A and B strains of bean common mosaic virus are two distinct potyviruses in Potyvirus taxonomy. Vienna: Springer, 415–431.
Xu, L., and Hampton, R. O. (1996). Molecular detection of Bean common mosaic and Bean common mosaic necrosis potyviruses and pathogroups. Arch. Virol. 141 (10), 1961–1977. doi:10.1007/bf01718207
Yadav, N., and Khurana, S. M. P. (2016). Plant virus detection and diagnosis: Progress and challenges in frontier discoveries and innovations in interdisciplinary. New Delhi: Springer.
Yeken, M. Z., Özer, G., Çelik, A., and Çíftçi, V. (2018). Identification of genes related to resistance for bean common mosaic virus and bean common mosaic necrosis virus in commercial common bean cultivars in Turkey. Turk. J. Agri. Nat. Sci. 5, 613–619. doi:10.30910/turkjans.471371
Keywords: BCMV, real-time PCR, SYBR green, common bean, viral load
Citation: Çelik A, Emiralioğlu O, Yeken MZ, Çiftçi V, Özer G, Kim Y, Baloch FS and Chung YS (2023) A novel study on bean common mosaic virus accumulation shows disease resistance at the initial stage of infection in Phaseolus vulgaris. Front. Genet. 14:1136794. doi: 10.3389/fgene.2023.1136794
Received: 03 January 2023; Accepted: 06 March 2023;
Published: 20 March 2023.
Edited by:
Muhammad Amjad Ali, University of Agriculture, Faisalabad, PakistanReviewed by:
Muhammad Nadir Naqqash, Muhammad Nawaz Shareef University of Agriculture, PakistanFarrukh Azeem, Government College University, Faisalabad, Pakistan
Copyright © 2023 Çelik, Emiralioğlu, Yeken, Çiftçi, Özer, Kim, Baloch and Chung. This is an open-access article distributed under the terms of the Creative Commons Attribution License (CC BY). The use, distribution or reproduction in other forums is permitted, provided the original author(s) and the copyright owner(s) are credited and that the original publication in this journal is cited, in accordance with accepted academic practice. No use, distribution or reproduction is permitted which does not comply with these terms.
*Correspondence: Ali Çelik, YWxpY2VsaWswMzJAZ21haWwuY29t; Göksel Özer, Z29rb3plckBnbWFpbC5jb20=; Faheem Shehzad Baloch, YmFsb2NoZmFoZWVtMTNAZ21haWwuY29t; Yong Suk Chung, eXNjaHVuZ0BqZWp1bnUuYWMua3I=