- 1Department of Medical Analysis, College of Pharmacy, Hawler Medical University, Erbil, Iraq
- 2Center of Research and Strategic Studies, Lebanese French University, Erbil, Iraq
- 3Medical Laboratory Science, Lebanese French University, Erbil, Iraq
- 4Department of Biology, College of Education, Salahaddin University-Erbil, Erbil, Iraq
- 5Department of Pharmaceutical Basic Science, Faculty of Pharmacy, Tishk International University, Erbil, Iraq
- 6Department of Medical Laboratory Science, Komar University of Science and Technology, Sulaimany, Iraq
- 7Department of Medical Genetics, School of Medicine, Shahid Beheshti University of Medical Sciences, Tehran, Iran
- 8Institute of Human Genetics, Jena University Hospital, Jena, Germany
- 9Urology and Nephrology Research Center, Shahid Beheshti University of Medical Sciences, Tehran, Iran
- 10Cancer Research Center, Shahid Beheshti University of Medical Sciences, Tehran, Iran
- 11Academy of Medical Sciences, Tehran, Iran
- 12Department of Pathology, Loghman Hakim Hospital, Shahid Beheshti University of Medical Sciences, Tehran, Iran
Breast cancer is the most prevalent type of malignancy among women. Exosomes are extracellular vesicles of cell membrane origin that are released via exocytosis. Their cargo contains lipids, proteins, DNA, and different forms of RNA, including circular RNAs. Circular RNAs are new class of non-coding RNAs with a closed-loop shape involved in several types of cancer, including breast cancer. Exosomes contained a lot of circRNAs which are called exosomal circRNAs. By interfering with several biological pathways, exosomal circRNAs can have either a proliferative or suppressive role in cancer. The involvement of exosomal circRNAs in breast cancer has been studied with consideration to tumor development and progression as well as its effects on therapeutic resistance. However, its exact mechanism is still unclear, and there have not been available clinical implications of exo-circRNAs in breast cancer. Here, we highlight the role of exosomal circRNAs in breast cancer progression and to highlight the most recent development and potential of circRNAas therapeutic targets and diagnostics for breast cancer.
1 Introduction
Breast cancer (BC) is the most common cancer in women and represents a leading cause of death on a worldwide scale in women (Torre et al., 2015; Chen et al., 2016; Siegel et al., 2018). Breast cancer treatment options include surgery, radiotherapy, chemotherapy, endocrine therapy, and targeted therapy that have allowed for more accurate and tailored care for patients with early-stage. However, the 26% 5-year survival rate for metastatic BC patients shows that this treatment is still a real challenge (Malmgren et al., 2018). The exact molecular pathways behind its etiology are still unknown. Recent studies have shown a number of potential mechanisms by which non-coding RNAs (ncRNAs), like circRNAs, regulate the progression of BC.
Exosomes are nanometric vesicles of endosomal origin containing material from the host cell, such as proteins, lipids, DNA, and RNA (Hegmans et al., 2008). These vesicles are secreted by exocytosis and are taken up by other cells, influencing their functions and behavior, and can be used as a drug carrier for cancer therapy (Ghafouri-Fard et al., 2021a; Hussen et al., 2022). Tumorous cells use exosomes to communicate with their surroundings like other body cells. In recent years, research has shown that circRNA-enriched exosomes are involved in the hallmarks of cancer (Su et al., 2019). In addition, they have a vital role in promoting tumor progression by creating a suitable microenvironment for their proliferation and metastasis (Jia et al., 2017; Kalluri and LeBleu, 2020).
Recently, a new class of non-coding RNA called circular RNA (circRNA) was discovered in exosomes. These are single-stranded RNA molecules that have been covalently closed to make a loop (Jeck and Sharpless, 2014). Due to their distinctive structure, circRNAs have a longer half-life and better resistance capabilities to RNase and exonuclease than linear RNAs; and they are abundance in tumorous exosomes (Seimiya et al., 2020).
Initially, Li et al. were the first to report that exosomes contained a lot of circRNAs (Li et al., 2015a). They found that the entry of circRNAs into exosomes was controlled by a number of factors or pathways, such as changing the expression of key miRNAs in the parent cells. Another pathway through which circRNAs get access to exosomes is via binding to RNA-associated proteins (Bao et al., 2016). Exo-circRNAs can have an oncogenic role or suppressive effect by interfering with several biological pathways (Zhang et al., 2019) and sponging miRNAs which can inhibit gene expression at transcriptional level (Xing et al., 2020). More interestingly, new studies revealed that exo-circRNAs can contribute to cancer cell sensitivity to chemotherapy or hormonal and radiation therapy (Liu et al., 2021) (Yang et al., 2020) (Liang et al., 2019a).
Although our understanding of exo-circRNAs’ functions has greatly expanded during the past 2 decades, but their precise mechanism remains unclear. In addition, there is still no clinical implication of exo-circRNAs in BC. Therefore, the main aim of this review is to provide a comprehensive description of the different aspects of the exo-circRNAs that contribute to BCs’ development and to discuss the therapeutic targets based on exo-circRNA as anti-cancer responses.
2 Biogenesis of exosome
Endosomes serve as a starting point for the production of exosomes (Figure 1). The creation of intraluminal vesicles begins with the invagination of the plasma membrane, which leads to the production of early endosomes. Meanwhile, early endosomes develop into multivesicular bodies (MVBs) that store many intraluminal vesicles (van Niel et al., 2011; Raposo and Stoorvogel, 2013). Exosomes originate from these intraluminal vesicles. When MVBs connect with a cell membrane, they release exosomes into the surrounding environment.
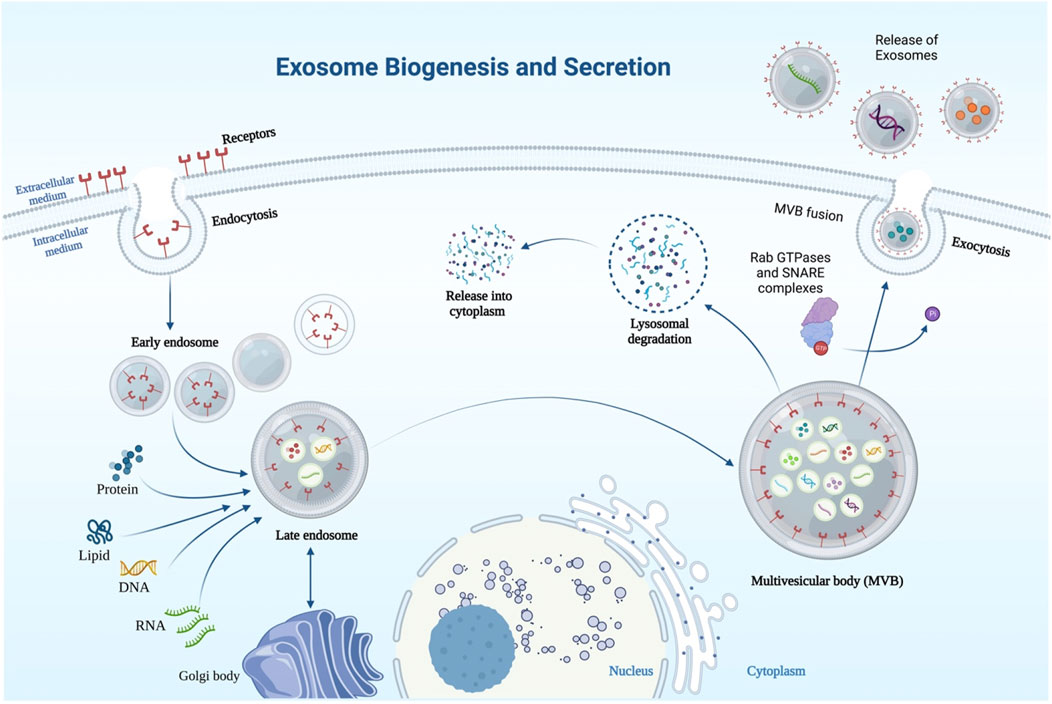
FIGURE 1. Schematic representation of exosome biosynthesis and secretion. Exosomes are formed through endocytic membrane invagination and ILV formation inside the cell. Payloads (RNA, proteins, DNA) are incorporated into ILV in an ESCRT-dependent or/and -independent manner during the maturation of early endosomes, which results in the formation of MVBs. MVBs with the plasma membrane results in the release of exosomes into the extracellular space, and MVBs can be transported along microtubules to the Golgi apparatus for endosome recycling or to lysosomes for degradation. Several key components including Rab GTPases and SNARE complexes are required for MVB fusion with the cellular membrane.
The endosomal sorting complex necessary for transport (ESCRT) is a key player in exosome formation (Mattissek and Teis, 2014). ESCRT is made up of five protein complexes: ESCRT-0, ESCRT-1, ESCRT-2, ESCRT-3, and VPS4A (Henne et al., 2013). The clustering of ubiquitinated payloads is facilitated by ESCRT-0, which is composed of the substrate and signal-transducing adaptor protein for the tyrosine kinase. VPS4A disassembles the ESCRT machinery for recycling; ESCRT-I and ESCRT-II are responsible for bud formation, while ESCRT-III initiates vesicle scission (Hurley and Hanson, 2010). Both early endosomes and MVBs survive after critical subunits of the four ESCRTs are knocked out, despite potential substantial changes in the shape of the components of the endocytic pathway (Stuffers et al., 2009). It is possible that proteins other than ESCRT are involved in regulating endosomal sorting, such as CD63 (van Niel et al., 2011), CD81 tetraspanin ligands (Perez-Hernandez et al., 2013), CD9, CD82 (Chairoungdua et al., 2010), and RAB31 (Wei et al., 2021).
2.1 Secretion of exosomes
Multivesicular endosomes (MVEs) in cells can merge with the lysosome, sending their contents to be degraded, or they can fuse with the plasma membrane to send their contents out into the extracellular environment as exosomes (van Niel et al., 2011; Kowal et al., 2014). Secretion of exosomes is thought to be controlled by certain Rab GTPases (Stenmark, 2009). For instance, Rab27a, Rab27b (Ostrowski et al., 2010), and Rab35 (Yang et al., 2019a) are involved in MVE docking at the plasma membrane. If Rab27a or/and Rab27b silenced, exosome release is suppressed without noticeable changes to the exosomes’ protein composition or morphology. Additionally, two of Rab27’s effectors, Slp4 and Slac2b, are responsible for a decrease in exosome secretion upon their silencing (Ostrowski et al., 2010). Likewise, by preventing Rab27a from being degraded by the proteasome, KIBRA regulates exosome secretion (Song et al., 2019). Furthermore, vesicle-membrane SNAREs (v-SNAREs) and target-membrane SNAREs (t-SNAREs) worked together to control MVE fusion with the plasma membrane (Jahn and Scheller, 2006).
2.2 Biological characteristics of exosomes
Exosomes are made by almost every cell (Zhao et al., 2021). They are detected to be circulating in fluids and secretions of the body, like urine, blood, bile, tears, semen, saliva, cerebrospinal and amniotic fluids (Geng et al., 2020). Moreover, exosomes play crucial regulatory roles in intercellular communications, locally and systematically (Wang et al., 2019a) by acting as signalling vesicles in autocrine, endocrine, juxtracrine, and paracrine (EL Andaloussi et al., 2013). Moreover, they can transport cargo from cell to cell, causing phenotypic alterations in recipient cells (Mathieu et al., 2019).
Exosomes have been implicated in many biological processes and show an essential role in pathological and physiological situations, including cancer, circulatory and metabolic abnormalities, immune responses, and inflammatory illnesses (Wang et al., 2019a; Kalluri and LeBleu, 2020). For example, through releasing cytokines and other bioactive molecules, exosomes create an appropriate microenvironment for tumor growth and regulate cell reproduction, metastasis, and drug resistance (Ye et al., 2022). Exosomes also contribute to early tumorigenesis in BC, can carry signalling molecules to cancer cells inside the TME, assist cancer cells in evading an immune response, promote angiogenesis, and alter the tumor microenvironment among other functions (Jia et al., 2017).
3 Biogenesis of circRNAs
CircRNAs are synthesized by RNA polymerase II transcription from pre-mRNA back-splicing, which links a 5′ splice site (downstream splice donor site) to a 3′ splice site (upstream acceptor splice site) (Ashwal-Fluss et al., 2014). Through alternative back-splicing, multiple circRNAs can be created from identical sequences. Despite decades of research, the precise mechanism underlying circRNA production is not fully understood. CircRNAs are categorized into three classes based on their structure and cycling mechanisms: exonic circRNA (ecircRNA) (Zhang et al., 2014; Chen et al., 2015), circular intronic RNA (ciRNA) (Zhang et al., 2013), and exon-intron circRNA (ElciRNA) (Li et al., 2015b). EcircRNAs are primarily found in the cytoplasm and have one or more exons resulting from alternative splicing (Zhang et al., 2014). The biosynthesis of ecircRNAs can be explained by one of three potential models: lariat-driven, RBP-mediated, and intron-pairing-driven (Jeck et al., 2013). When RNA is folded, exons are skipped and ecircRNAs are created. These rearrangements cause the creation of lariat structures, which are clusters of nearby exons and introns previously located far from each other. After the intron sequence is spliced out using the lariat structure, circular RNAs are produced (Figure 2). It is believed that back-splicing and canonical splicing are linked because the majority of highly expressed circRNAs are constructed from many internal exons of pre-mRNAs and a small number of introns (Zhang et al., 2014). Nevertheless, identifying the co-expression of circRNAs and their putative linear RNAs with exon exclusion has been challenging because of the quick degradation of untranslated linear RNAs (Jeck and Sharpless, 2014).
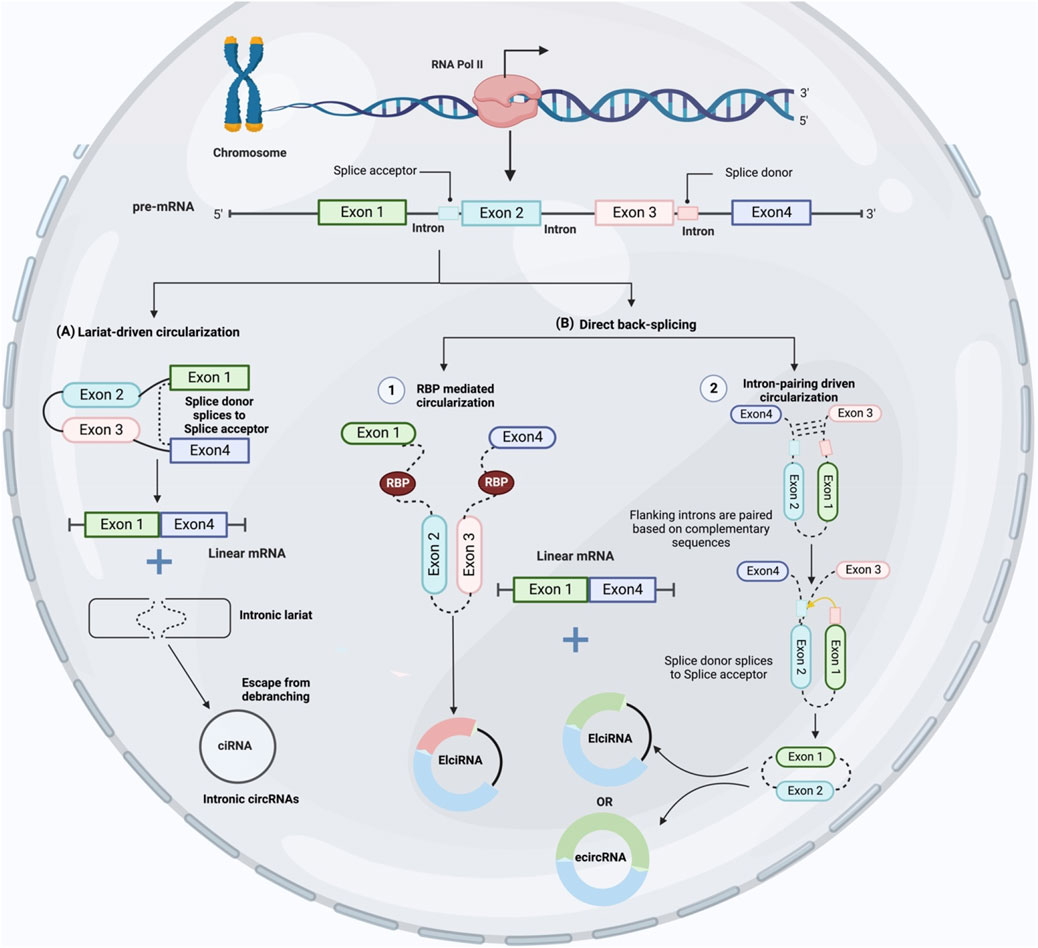
FIGURE 2. CircRNA biogenesis. (A) Exon skipping creates an mRNA with exons one and four and a lariat structure or intronic lariat escape from debranching and produced intronic circRNAs (ciRNAs). (B) Back-splicing occurs initially, followed by further processing to create linear mRNA from circRNA with exons and introns. The first type, called “intron-pairing driven circularization,” occurs when complementary segments on either side of an intron pair up to carry the splice sites closer together and facilitate circularization. The second type, called “RBP mediated circularization,” occurs when RBPs bind with the intron binding sites to bring the splice sites closer together and initiate circularization.
CircRNA biogenesis could be divided into two subprocesses based on the sequence of their basic steps. Lariat-driven circularization or back-splicing of a linear RNA including skipped exons and a long lariat of introns and exons generates a circRNA using the canonical splicing pathway which is called exon skipping model (Figure 1A). However, If the RNA precursor undergoes back-splicing early, a circRNA and an intermediate containing introns and exons will be generated. After this, a linear RNA is synthesized from the RNA precursor which is called direct back splicing (Figure 1B). Recent studies have shown that both developed models are successful in vivo (Lasda and Parker, 2014; Chen and Yang, 2015). Direct back splicing is further categorized into two other groups: “RBP mediated circularization” and “intron-pairing driven circularization” based on the differences in circularization mechanisms (Huang and Zhu, 2021). Furthermore, it is exciting to discover how these factors control circRNA expression and how they modify during carcinogenesis.
3.1 Biological characteristics of circular RNAs
Expression and regulation of circRNAs take place specifically by cells and tissues at different developmental stages (Conn et al., 2015). The majority primarily exists in the cytoplasm. Scientists have studied their function as post-transcriptional regulators (Han et al., 2018). Although there is no comprehensive description of circRNAs properties and biological implications (Tran et al., 2020), thus far, it has been shown to act like miRNA sponges, interact with proteins, regulate the splicing or transcription of genes, translate proteins, and exert epigenetic regulations (Han et al., 2018). Having diverse functions in the body, circRNAs involvement in various body diseases, such as cardiovascular diseases (Altesha et al., 2019), renal diseases (Jin et al., 2020a), chronic liver diseases (Zeng et al., 2021), skin diseases (Wu et al., 2020) and cancers (Ghafouri-Fard et al., 2022a; Ghafouri-Fard et al., 2022b).
Studies continuously demonstrate that abnormally expressed circRNAs are associated with several features of cancer (Li J. et al., 2020), in particular carcinogenesis, programmed cell death, proliferation, invasiveness, metastatic abilities, resistance to radiotherapy and chemotherapy, and cancer prognosis (Geng et al., 2020).
Dysregulation in circRNA gene expression is considered one of the important factors causing the onset and progression of gynecological cancers (Tran et al., 2020). In addition, numerous studies over the last years have shown circRNAs to be tightly linked to BC, and these studies can be categorized into two major groups: those that investigate the regulatory effect in BC development and those focused on detecting different patterns of expression to elucidate possible biomarkers for diagnosis of BC or molecular subtypes (Ghafouri-Fard et al., 2021b).
4 Biological implications of exosomal circRNAs in breast cancer
4.1 Tumor regulation, cell proliferation, and angiogenesis
Exo-circRNAs’ contribution to tumor regulation, proliferation, and angiogenesis has been shown in many studies. When Li et al. studied tumor and paracancerous sample tissues of BC patients, they detected significant overexpression of circ_002178 in cancer tissue compared to surrounding cells. They also found that overexpression of circ_002178 in vitro caused a considerable increase in the rate of survival and the number of SUM149PT cell clones, which facilitates tumorigenesis through targeting miR-1258 and modulating the expression of KDM7A (Li W. et al., 2020). Further, Liu et al. used the gain and loss of function approach to study the functional role of hsa_circRNA_002178 in BC angiogenesis. They revealed that overexpression of has_circRNA_002178 was associated with poor prognosis in their microarray-based gene expression analysis. Later they proved that hsa_circRNA_002178 knockdown inhibited angiogenesis by decreasing cell survival, energy usage, and the ability to form tubes (Liu et al., 2020a). Likewise, Cai et al. used the same gain and loss of function to study hsa_circ_0000515. First, they detected overexpressed hsa_circ_0000515 in BC tissues. Afterward, they silenced hsa_circ_0000515 in the MCF-7 cell line. Finally, they found that MCF-7 cells were neither able to progress through their cell cycle nor able to proliferate or invade, and their angiogenetic potential was diminished through sponging miR-296-5p and modulating CXCL10 expression (Cai et al., 2021).
Furthermore, Liang and his team performed a circRNA microarray to screen for dysregulated circRNA in BC tissues. Among 2,587 abnormally expressed circRNA, there was significant overexpression of circ-ABCB10 with five to ten times more in BC cells than normal ones. Then, their bioinformatics study found that miR-1271 could represent a target for circ-ABCB10. They also found that knocking down circ-ABCB10 led to cell cycle arrest in the G0/G1 phase, suppression of colony formation, and inhibition of cell proliferation in vitro (Liang et al., 2017). Circ-Dnmt1 (hsa_circRNA_102,439) was overly expressed in BC cells, which caused inducing of proliferation and survival of cells. It also led to stimulation of cellular autophagy, inhibition of cellular biological aging, and increment of tumor growth (Du et al., 2018). These outcomes resulted from circ-Dnmt1 binding to p53 and Auf1, which triggered nuclear translocation of these tumor suppressor proteins and lowered p53 transcription (Tesfaye et al., 2021). Table 1 shows the expression levels of several different carcinogenic exo-circRNAs in BC along with their targets and hallmarks.
Cancer cells utilize a significant amount of their energy using anaerobic glycolysis (Warburg effect), a phenomenon of changing glucose to lactate for energy generation, succeeded by lactate fermentation in the absence of oxygen (Fadaka et al., 2017). In BC cells, circRNF20 promoted anaerobic glycolysis by increasing glucose uptake, lactate production, and ATP levels, in addition to reducing apoptosis and increasing proliferation (Cao et al., 2020).
Despite this, several circRNAs are extensively downregulated in vitro and in vivo studies of BC; these circRNAs inhibit tumor growth by sponging oncogenic miRNA or targeting other oncogenic products (Table 2). For example, Zhang and Ling Mao found circ_0000442 to be downregulated in cell lines and patient specimens. Using a variety of methods, they were able to identify the tumor suppressor circ_0000442 by its ability to sponge miR-148b-3p and decrease its proliferative effect. Furthermore, the G1 arrest effect was enhanced by circ_0000442 overexpression in cell lines, and colony formation was inhibited (Zhang and Mao, 2021). In the same way, Peng and Wen found that circDDX17 expression was low and there was a direct interaction between circDDX17 and miR-605, which controlled the expression of cell cycle genes (increased p21 expression and suppressed CDK1 expression) (Peng and Wen, 2020).
Furthermore, Li et al. compared the expression of circ-VRK1 in 350 BC tumor tissues and 163 adjacent tissues, and the results showed lower circ-VRK1 expression in tumor tissues. They also found that patients with a low level of circVRK1 expression had unfavorable clinicopathological features (Li and Li, 2020). Likewise, Liu and his colleagues used plasmid transfection to overexpress circRNA_103809 in cell lines, results showed that overexpression of circRNA_103809 could significantly suppress the growth of these cell lines. The team also studied proteins that are involved in the cell cycle (CyB1 and Cyd1) and found that overexpression of circRNA_103809 could stop the cell cycle at the G2/M phase (Liu et al., 2020b). Additionally, Xiao et al. showed a low level of circAHNAK1 in TNBC patients and cell lines. Then, they overexpressed circAHNAK1 in BC cells and found that it inhibited cancer proliferation and suppressed colony formation in two different cell lines by affecting miR-421 and RASA1 (Xiao et al., 2019).
These findings shed light on previously unknown functions of circRNAs in the BC tumor microenvironment, particularly in tumor growth regulation.
4.2 Regulation of EMT and tumor metastasis
Breast cancer is a systemic disease that commonly leads to metastatic spread (Hüsemann et al., 2008). Metastasis is a complex and multi-step process (Lambert et al., 2017) that is responsible for the majority of cancer patients’ deaths (Wittekind and Neid, 2005). Therefore, knowing the mechanisms behind metastasis can be crucial to improve alternative therapies and creating new approaches for better managing metastatic diseases (Parker et al., 2022).
Tumor exo-circRNAs are more highly expressed in BC (De Palma et al., 2022). Their significance in cancer metastasis and EMT was demonstrated through sponging miRNAs and modifying tumor suppressor genes or specific signalling pathways (Figure 3). For instance, Zeng and his colleagues showed that patients with overexpressed circANKS1B had a higher risk of metastasis to lymph nodes and higher clinical stage, as in vitro and in vivo research outcomes demonstrate that circANKS1B enhanced metastasis and invasion by inducing epithelial-to-mesenchymal transition (EMT) through TGF-β1 signalling pathway (Zeng et al., 2018). Meanwhile, Chen et al. detected overexpression of FECR1 circRNA in BC cell lines and tissue samples. Circular RNA FECR1 made tumors more aggressive and spread to other parts of the body by controlling how genes methylated and demethylated their DNA. It could do this by interacting with the FLI1 promoter in cis to start TET1 demethylase and by turning down DNMT1 in trans (Chen N. et al., 2018). Zhou et al. selected overexpressed hsa_circ_0011946 among 152 differentially expressed circRNA in BC. Based on miR26a/b sponging, they hypothesized that hsa_circ_0011946 targeted replication factor RFC3. When hsa_circ_0011946 was knocked down, RFC3 mRNA and protein expression were silenced. Thus, migration and invasion abilities were inhibited (Zhou et al., 2018). Moreover, it was discovered by Song et al. that hsa_circ_0007534, when elevated, promoted BC cell proliferation and invasion via MUC19 to modify miR-593 production, whereas hsa_circ_0007534, when downregulated, lost its oncogene properties and increased apoptosis (Song and Xiao, 2018).
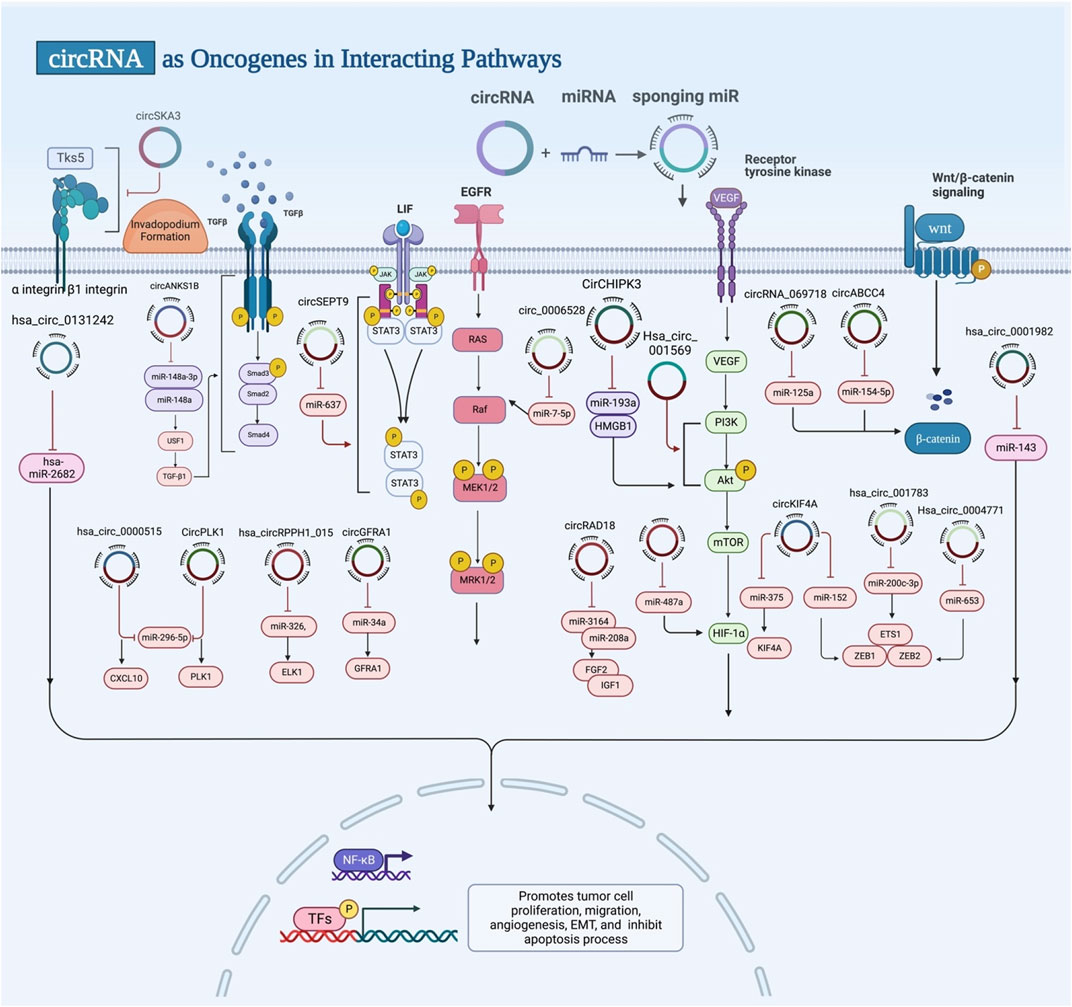
FIGURE 3. Exosomal circRNAs act as oncogenes. Exosomal circRNAs can promote tumor cell proliferation, migration, angiogenesis, EMT and inhibit apoptosis by targeting miRNA, genes, and signalling pathways.
Fu and his colleagues showed that circBCBM1 encourages cancer proliferation and migration. In vivo studies with mice showed that circBCBM1 also helps growth of cells and spread to the brain. Their finding suggested that circBCBM1 sponges miR-125a and modulates BRD4 regulation, leading to changes in the MMP9 expression via the Sonic hedgehog (SHH) signalling pathway (Fu et al., 2021). Also, Zhou et al. looked at BC and lung tissues that had spread to other parts of the body, and they found that metastatic lung samples had the most circFBXL5 upregulation. They showed that the knockdown of circFBXL5 in mouse models diminished the growth and lung metastasis of tumors. Furthermore, they revealed that circFBXL5 modulated the expression of SRSF6 via sponging miR-660 (Zhou et al., 2020a). Similarly, Song et al. detected circHMCU upregulation in BC cell lines, which was linked to the fast proliferative process and metastasize to the lungs. Furthermore, they found that circHMCU could impact the G1 phase cell cycle checkpoint and epithelial-mesenchymal transition (EMT) pathway of BC cells, enhancing proliferation, migration, and invasion (Song et al., 2020).
On the other hand, the expression levels of certain exo-circRNAs are reduced or inhibited in BC (Figure 4). For example, Xu and his team performed high-throughput circular RNA microarray assays of primary BC tissues and lung metastatic tissues samples and found circNFIC to be most downregulated in metastatic lung tissues among 20 dysregulated circRNAs. Then, they overexpressed circNFIC in mouse models and detected suppression of tumor growth and lung metastasis (Xu et al., 2020a). Hou and his team detected the most downregulation of circASS1 in 1,137 dysregulated circRNAs in BC cells. Overexpression of circASS1 was discovered to have anti-invasion and anti-migration effects. The group hypothesized that circASS1 promotes expression of its parent gene ASS1 via sponging miR-443 (Hou et al., 2019). Furthermore, significant downregulation of circNR3C2 in TNBC was discovered by Fan et al., and this was inversely linked with distant metastasis and tumor invasiveness. Overexpression of circNR3C2 inhibits tumor development and metastasis by degrading vimentin, as shown by gain-of-function studies. It also promotes expression of the tumor suppressor gene HRD1 (Fan et al., 2021). Moa et al. determined a low level of circRNA_000554 and ZFP36 while a high level of miR-182 in BC tissues. In addition, an immunofluorescence assay was performed to evaluate expressions of EMT markers Vimentin, N-cad, and E-cad in MCF-7 cells and the results showed reduced expression of Vimentin and N-cad, and increased expression of E-cad (Mao et al., 2020).
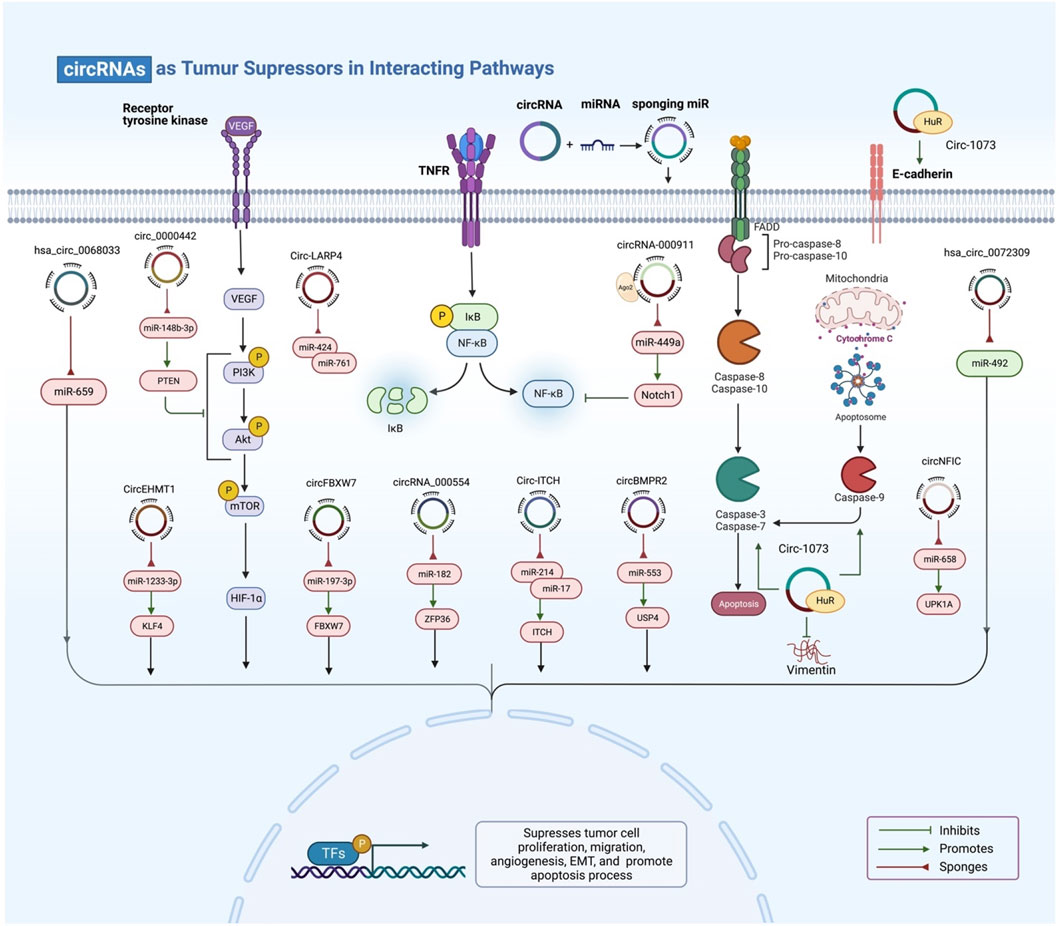
FIGURE 4. Exosomal circRNAs act as tumor suppressors. By targeting miRNA, genes, and a variety of signalling pathways, exosomal circRNAs can inhibit tumor cell proliferation, migration, angiogenesis, and EMT and stimulate apoptosis.
Additionally, circular mitochondrial RNAs (circ-mtRNAs) have been found to regulate mitochondrial function and cellular metabolism in BC. For example, a specific circ-mtRNA, called circRNA_103809, was significantly down-regulated in BC tissues compared to adjacent normal tissues (Liu et al., 2020b). In breast cancer cells, the overexpression of circRNA_103809 could interfere with the EMT signalling pathway, causing miR-532-3p to function improperly, resulting in G2/M phase arrest and a reduction of cell proliferation and metastasis (Liu et al., 2020b). However, further studies are required to better understand circ-mtRNAs’ functions and their potential as diagnostic or therapeutic targets in breast cancer.
These studies show that circRNAs can influence the spread of tumor cells to distant parts of the body through different mechanisms and pathways by suppressing or promoting invasion and metastasis.
4.3 Exosomal circRNAs and apoptosis
Apoptosis is programmed cell death that normally occurs through the developmental and aging stages of cells as a natural body mechanism to control the population of cells in tissues (Kaczanowski, 2016). Apoptosis occurs just in a single cell and does not trigger an immune response. The relative abundance of pro-apoptotic and anti-apoptotic signals within and around a cell determines whether the cell will survive or die by apoptosis (Henne et al., 2013).
Through interactions with downstream signalling pathways, circRNA can control the apoptotic process and contribute to BC pathogenesis (Figure 5). For example, Jiang and Cheng detected overexpression of circABCC4 in BC tissues, and they found circABCC4 to be involved in cell viability, migration and apoptosis via sponging miR-154-5p. Then, with the downregulation of circABCC4, they discovered enhancement of apoptosis, as well as significant downregulation of Bc1-2 and upregulation of Cleaved/Caspase-3 (Jiang and Cheng, 2020). Zheng et al. investigated the role of circSEPT9, and they found upregulation of circSEPT9 was associated with advanced clinical stage and bad prognosis in TNBC. They also revealed circSEPT9’s role in tumor growth, migration, invasion, and apoptosis via miR-637/LIF axis (Zheng et al., 2020). Likewise, Xie et al. detected high expression of hsa_circ_0004771 and ZEB2 in BC tumor tissues, with a reduction in the expression of miR-653. Differential knockdown of circ_0004771 and ZEB2, as well as overexpression of miR-653 in BC cells, decreased cell growth and triggered apoptosis (Xie et al., 2019).
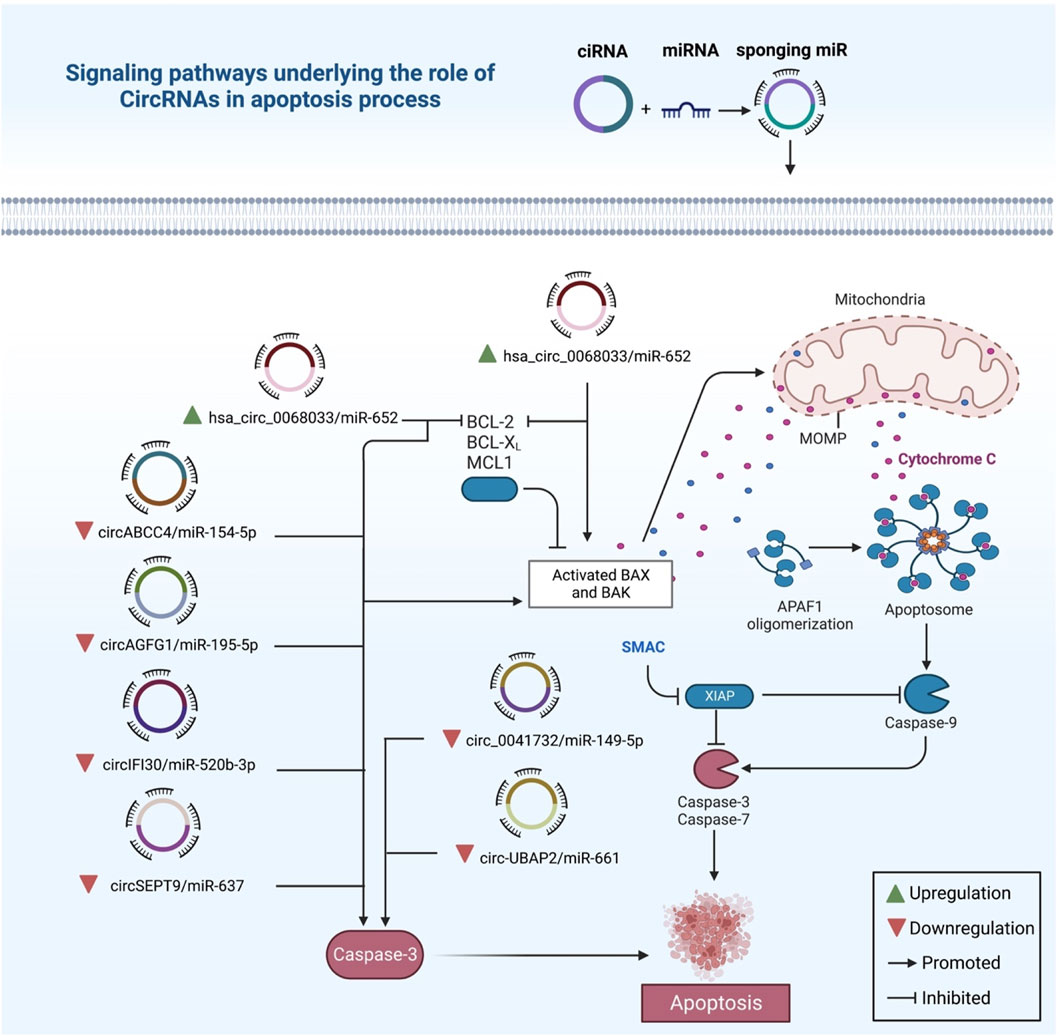
FIGURE 5. The intrinsic apoptotic pathway in breast cancer and the regulatory roles of exo-circRNAs. The production of caspase-3 is reduced when tumor suppressor circRNAs are downregulated, which in turn reduces apoptosis. Contrarily, oncogenic circRNAs like hsa_circ_0068033 promote oncogenic activity by inhibiting apoptosis via activating BAX and BAK genes.
PI3K/AKT signalling pathway is one of the main signal transition pathways inside cells, and they are remarkably important in apoptosis and cell survival regulation (Meng et al., 2017). According to recent evidence, the expression of cancer-related genes modulated by the circRNA/PI3K/AKT axis and suppressed apoptosis. For instance, Xu et al. detected significant upregulation of hsa_circ_001569 both in cell lines and BC tissues. When they glanced at the effects of silencing has_circ_001569 in cell lines, they found that has_circ_001569 knockdown not only stopped migration and invasion but also changed EMT markers and caused apoptosis by preventing the activation of the PI3K/AKT signalling pathway (Xu et al., 2019a).
On the other hand, the upregulation of circRNAs may induce the apoptosis process through sponging miRNAs. For example, Hu et al. found that the upregulation of circRNA_0001283 significantly inhibited cell growth and invasion while promoting apoptosis by sponging miR-187 and decreasing its expression (Hu Y. et al., 2020). Additionally, Yuan et al. detected downregulated hsa_circ_0068033 in BC tissues, which was significantly associated with the TNM stage and tumor size of patients. Furthermore, Yuan and his colleagues performed in vitro experiments and also found overexpressed hsa_circ_0068033 to sponge miR-659 and inhibit tumor growth in addition to inducing apoptosis through activation of intrinsic apoptotic pathways (Yuan et al., 2020). Furthermore, Wang et al. detected circRNA_000911 to be downregulated in BC cells. When they overexpressed circRNA_000911 in cell lines, they observed suppression of proliferation, migration, and invasion, with increased apoptotic abilities of the cells through sponging miR-449a, thus enhancing Notch1 and NF-κB signalling pathway (Wang et al., 2018a). Focusing on mechanisms in which circRNAs affect apoptosis is essential in BC since studies show that treating BC through promoting apoptosis is a promising approach.
4.4 Modulation of chemotherapy resistance
Therapy for breast cancer consists of multifaceted strategies, including surgery, radiotherapy, neoadjuvant and adjuvant therapy. It is crucial that the chosen therapy has an optimal therapeutic effect for the proper treatment of BC (Fisusi and Akala, 2019).
Tamoxifen is known as an endocrine therapy that is most frequently used for hormone receptor-positive (HR positive) patients suffering from BC. Sang and his team used RNA-seq to detect the downregulation of circRNA_0025202 in tamoxifen-resistant cells, they performed further analysis and found that circRNA_0025202 acted as a tumor suppressor. Then they treated BC cells with circRNA_0025202 alongside tamoxifen, which led to tumor growth suppression and enhanced tamoxifen sensitivity in vivo (Sang et al., 2019). Similarly, Liang et al. detected downregulation of circBMPR2 in BC tissues. They knocked down circBMPR2 in tamoxifen-treated cell lines, and assay results showed enhanced tamoxifen resistance by suppressing tamoxifen influenced apoptosis (Liang et al., 2019a).
On the other hand, Hu et al. found that circ_UBE2D2 was upregulated in BC tamoxifen-resistant cells and promoted resistance through combination with miR-200a-3p to modulate viability of cells, metastasis, and Erα level in vivo and in vitro studies (Hu K. et al., 2020).
Monastrol is a chemotherapeutic agent that suppresses tumors by hindering mitotic kinesin Eg5 needed for forming bipolar spindle. Liu et al. performed a human circRNA microarray on cancer cells resistant to monastrol and found circRNA-MTO1 to be downregulated. The team then upgraded circRNA-MTO1 expression in BC cells resistant to monastrol and showed that circRNA-MTO1 not only reversed resistance but also had a synergetic effect when used with monastrol through binding to TRAF4 and inhibiting Eg5 protein (Liu et al., 2018). Even though BC cells showed over-expression of circFBXL5, even more upregulation was detected in antitumor agent 5-fluorouracil (5-FU) resistant BC cells. According to study results performed by Zhu and his colleagues, circFBXL5 appeared to regulate cell migration, invasion, and apoptosis by modulating the miR-216b/HMGA2 axis (Zhu et al., 2021).
In contrast, Liang et al. detected circKDM4C downregulation in metastasized tissues of BC, which was involved in both BC advancement and chemotherapy resistance. Resistant cells to doxorubicin showed a low level of circKDM4C, while transfection of MDA-MB-231/DOX cells with circKDM4C expression vector led to reduced cell proliferation and lowered resistance (Liang et al., 2019b). Likewise, Zhang et al. found downregulation of circKDM4C in BC tissues and intended overexpression of circKDM4C increased sensitivity of doxorubicin-resistant cells (Zhang et al., 2020). Another circular RNA that impacted therapy with doxorubicin was circUBE2D2, but negatively, as it induced doxorubicin resistance. When Dou and his colleagues knocked down circUBE2D2 in TNBC cells, it reversed the chemoresistance via downregulation of miR-512-3p or upregulation of CDCA3 (Dou et al., 2020). Similarly, overexpression of hsa_circ_0092276 was detected in BC doxorubicin-resistant cells by Wang et al., and this overexpression contributed to the resistance through altering autophagy-related gene 7 (ATG7) via sponging miR-384 (Wang et al., 2021).
Resistance to paclitaxel is one of the concerns in TNBC patients undergoing chemotherapy. Ma and his colleagues overexpressed circAMOTL1 in BC cells through plasmid construct, which improved cell viability, reduced apoptosis, and promoted invasion ability in MDA-MB-231 cells treated with paclitaxel. CircAMOTL contributed to resistance via AKT pathway regulation, promotion of anti-apoptotic protein, and suppression of pro-apoptotic protein (Ma et al., 2019). Meanwhile, Zang et al. observed circ-RNF111 upregulation in cells resistant to paclitaxel compared to cells responding to paclitaxel. When the team knocked down Circ-RNF111, the resistance of BC cells to paclitaxel was suppressed both in vivo and in vitro by upregulating E2F3 via sponging miR-140-5p (Zang et al., 2020). Li et al. detected 3.34 times increase of hsa_circ_0000199 in TNBC tissues compared to non-TNBC tissues. Breast cancer cells with silenced hsa_circ_0000199 became more sensitive to paclitaxel, cisplatin, gemcitabine, and adriamycin therapies in TNBC. Improved chemotherapeutic outcomes were achieved through enhanced expression of miR-206/miR-613 and inactivated PI3K/Akt/mTOR signalling (Li et al., 2021). In addition, Goa and his colleagues spotted a higher level of circ_0006528 in BC cells resistant to adriamycin compared to sensitive cells, and even though the mechanism was not known, there was a noticeable increase in sensitivity to adriamycin with downregulation of circ0006528 (Gao et al., 2017) (Table 3).
It can be observed through these studies that circRNAs have essential roles in the efficacy of a wide variety of treatment options for BC through their contribution to resistance or sensitivity of therapies.
5 Role of exosomal circRNAs in diagnosis and prognosis
Exosomes contain many biologically active agents with the potential to be used as biomarkers in BC (Jia et al., 2017), including circRNAs (Jahani et al., 2020). Two characteristics of circRNAs that make them a suitable candidate for biomarkers of BC are: they are remarkedly stable in comparison to linear RNAs, and they have ten times more expression than linear isomers (Guo et al., 2014). Furthermore, there is evidence suggesting that circRNAs have a crucial part in BC progression and can be used for BC screening as tumor markers due to being stable and tissue-specific (Lü et al., 2017).
Determining biomarkers has a crucial role in diagnosing, anticipating prognosis, and identifying the clinical importance of BC patients. Liang et al. detected 1.5-fold overexpression of linear CDYL and 3.2-fold elevation of circular CDYL expression in BC tissues compared to paired noncancerous tissues. However, expression of circCDYL was associated significantly with clinicopathological characteristics of BC patients, while linear CDYL was not. Therefore, Liang and his colleagues suggest that circCDYL has the capacity to help in diagnosis and prognosis in BC (Liang et al., 2020). Furthermore, Yin et al. performed ROC curve to analyze the diagnostic value of hsa_circ_0001785, and their results revealed that hsa_circ_0001785 had 0.784 AUC value, supporting its great potential to be a biomarker for BC early detection. Similarly, Yuan et al. suggested that hsa_circ_0068033 is a valuable biomarker for early detection of BC for the reason that ROC analysis showed that hsa_circ_0068033 has a high diagnostic value (AUC = 0.8480) (Yuan et al., 2020).
Additionally, He et al. used. Kaplan-Meier survival analysis to examine prognostic determinant abilities of circGFRA1. According to results used to draw OS and DFS graphs, over-expressed circGFRA1 was linked to bad prognosis in TNBC patients (He et al., 2017). Furthermore, in two different studies, circEPSTI1 and circKIF4A were investigated in 240 triple-breast cancer patients; results showed overexpression of both circular RNAs. The high expression level of both was direct relation to size of the tumor, lymph node invasion, stage of TNM, as well as bad prognosis (Chen B. et al., 2018; Tang et al., 2019). Similarly, Qu et al. discovered that overexpressed circ_0103,552 in BC patients appeared to be correlation with bad pathological features and fewer survival chances. The independent prognostic factor of circ_0103,552 was also examined by multivariate analysis, and results showed circ_0103,552 to be an independent prognostic predictor for BC (p = 0.042) (Yang et al., 2019b). Additionally, Chen et al. detected overexpression of cirCHIPK3 in tumor tissues, which assisted BC cells in developing and progressing through modulating HMGB1/PI3K/AKT, and its high level was associated closely with lower prognosis (Chen et al., 2020).
On the contrary, Yin and his colleagues measured hsa_circ_0001785 plasma levels in BC patients before and after surgery, and it showed a significant decrease in hsa_circ_0001785 in postoperative patients (Yin et al., 2018). Likewise, Zhang et al. performed RT-qPCR on sample tissues of 283 female BC patients, and the level of circ-LARP4 appeared to be low. Downregulated circ-LARP4 at the tumor site of BC was related to increased tumor size, advanced clinical stage, and bad prognosis in BC (Zhang et al., 2020). Furthermore, Liang et al. detected downregulation of circKDM4C in BC cells through microarray results, and the low level was linked to bad prognosis, metastasis, and low survival chances (Liang et al., 2019b).
Being an effective indicator of both diagnosis and prognosis, circRNAs have the potential to be used to detect early tumor formation and to predict the survival chance of BC patients.
6 Exosomal circRNAs as potential therapeutic targets in breast cancer
Breast cancer is very heterogenous, and despite advancements in therapies, the prognosis of the disease is still not very promising. Thus, novel and specific mechanism-based drugs should be designed and synthesized for treating BC. Recent studies related to circRNA in cancer showed that circRNA could be used as a therapeutic strategy (Hussen et al., 2022; Wu et al., 2022).
Ye and his colleagues detected downregulation of CircFBXW7 in BC cell lines, and the expression level was associated with tumor size and metastasis of patients. When circFBXW7 was overexpressed in cell lines using a vector, it sponged miR-197-3p, increasing tumor suppressor gene FBXW7 expression, which encoded for FBXW7-185aa protein. Consecutively, the migration and proliferation capabilities of cells were diminished, suggesting circFBXW7 to be a good target for new therapies and a prognostic biomarker of BC (Ye et al., 2019). In another most recent study supporting the use of circRNA as a therapeutic target for TNBC, Li et al. detected upregulation of circ_0041732 in cancer cells, which contributed to tumor formation via sponging miR-149-5p. Fibroblast growth factor 5 (FGF5) is considered a target for miR-149-5p. Silencing of circ_0041732 lead to tumor formation inhibition by reducing FGF5 expression via miR-149-5p in vivo (Li et al., 2022a). Liang et al. spotted a low expression level of circBMPR2 in BC cells, that was negatively linked to cell proliferation, invasion, and migration in BC. According to the recent data, circBMPR2 to profusely sponge miR-553, and miR-553 promoted cancer cells to proliferate and migrate through tumor suppressor USP4. Thus, circBMPR2/miR-553/USP4 could be an excellent therapeutic candidate (Liang et al., 2019a).
Similarly, Liu et al. detected downregulation of circRNA_103,809 in BC tissues, which was linked to tumor size, stage of TNM, and HER-2 levels. While intended overexpression of circRNA_103809 was able to suppress EMT pathway substantially via sponging miR-532-3p (Liu et al., 2020b). On the contrary, Huang and his colleagues detected upregulation of circ_0103,552, which acted like an oncogene by overexpressing CYR61 through targeting miR-515-5p and consequently promoting division, migration, and invasion characteristics. circ_0103552/miR-515-5p/CYR61 axis has a good aptitude to be developed for the treatment of BC (Huang et al., 2021).
Another therapeutic approach that can be developed from circRNA is pathway interactions. Wang et al. detected considerable downregulation of circRNA_000911 in BC cells, and through a biotin-labelled circRNA_000911 probe, they found that circRNA_000911 sponges miR-449a, consecutively modulating signalling flow of NF-κB and Notch1 (Wang et al., 2018a). Further, Chen et al. detected upregulation of cirCHIPK3 in BC tissues, which increased the expression of HMGB1 when sponging miR-193a (Chen et al., 2020). PI3K/AKT axis has an essential role in tumor progression (Chen et al., 2020), and HMGB1 has been shown to encourage angiogenesis and migration of tumors through PI3K/AKT signalling. Likewise, Western blot analysis revealed that HMGB1-mediated phosphorylation of PI3k and AKT was diminished in cells with silenced cirCHIPK3. Thus, suggesting that cirCHIPK3 is a very valuable candidate for finding novel therapies for BC (Chen et al., 2020).
Over the last few decades, a great deal of attention has been given to the development and evaluation of nanoparticles based on their potential to be utilized as good agents for cancer diagnosis and therapy (Baetke et al., 2015), with BC included (Mu et al., 2017). For example, When Yi et al. injected a mouse tumor site with nanoparticles containing circ-1073 plasmid, they concluded that the circ-1073 could bind to HuR to increase its expression, which in turn suppressed malignancy of BC cells by elevating E-cadherin and cleaved-Caspase 3/9 levels (Yi et al., 2020).
In particular, using exo-circRNAs as a way to treat cancer has both challenges and potential opportunities, especially when it comes to regulating circRNA expression in vivo, off-target effects, circRNA delivery, and drug resistance. Many approaches and strategies have been proposed to overcome these challenges.
In term of circRNAs regulating circRNA expression in vivo, Researchers proposed to use transposon to deliver a circRNA expression cassette (Mecozzi et al., 2022). In addition, it is possible to produce circRNAs artificially and enhance their capabilities (Rossbach, 2019).
On the other hand, the off-target limitation affects the capacity of circRNAs in BC therapy. To overcome this challenge siRNA approach can be used to reduce the expression of none-desired circRNA which may decrease the off-target effects of circRNA. For example, Zhang and his colleagues used siRNA to reduce the expression of none-desired circRNA which consequently decreased the off-target effects of circRNA (Zhang et al., 2018a).
Besides that, delivery of circRNA using lipid nanoparticles and delivery of circRNA via exosomes can be used to overcome delivery challenges. For example, according to genome-wide RNA-seq research, Li et al. found that exosomes are a rich source of circRNAs, and they were proven to be abundant in exosomes as compared to parental cells (Lener et al., 2015). Similarly, in a mouse model, Li and his team showed that circRNA can be successfully encapsulated and distributed into aggressive tumors by using lipid nanoparticles (Li et al., 2022b).
Additionally, the development of resistance to anti-cancer drugs is another issue that complicates BC therapy. Researchers found that tumor cells might use exosomes to handle their drug resistance via ciRS-122 to susceptible cells (Xu et al., 2020b).
7 Conclusion and future direction
Exosomes are nanometric particles that mediate the transfer of local or systemic cell-to-cell oncogenic signals and play a crucial role in driving cancer progression by providing a suitable environment for cancer cell proliferation and invasion. Exo-circular RNAs can act as oncogenes or tumor suppressors in cancer. They are involved in hallmarks of BC such as, proliferation, angiogenesis, migration, invasion, metastasis, and apoptosis through modulating different pathways. Breast cancer therapy can also be affected by exo-circRNAs, as they can contribute to the promotion of sensitivity and resistance to therapeutic drugs. Therefore, exo-circRNAs have the potential to be used as biomarkers or prognostic markers. In the future direction, practical treatment approaches can be developed from circRNA as they contribute to the formation and progression of BC through many different mechanisms and pathways. Especially due to its potential clinical value, studying circRNAs as cancer therapies is an exciting but difficult and challenging field of study. Advances in the research have shown their therapeutic value, and their prospective significance in individualized diagnostics and therapy. Nevertheless, their potential value as clinical studies for BC have not been evaluated in large clinical cohorts like that of other ncRNAs. The lack of methodological standards and the troubling methodological heterogeneity are two issues that, in our opinion, ought to be addressed in future studies. Large and more characterized cohorts, adequate validated procedures, proper control groups, and understanding BC tissue heterogeneity are required for applying into clinical translation. Accordingly, more research is needed to fully realize the value of exo-circRNAs and integrate them into the design and synthesis of novel therapies for BC.
Author contributions
MT and BH designed and supervised the study. SG-F, EJ, HH and SM wrote the draft and revised it. SA, MS, MK, and GH collected the data and designed the figures and tables. All the authors read the submitted version and approved it.
Conflict of interest
The authors declare that the research was conducted in the absence of any commercial or financial relationships that could be construed as a potential conflict of interest.
Publisher’s note
All claims expressed in this article are solely those of the authors and do not necessarily represent those of their affiliated organizations, or those of the publisher, the editors and the reviewers. Any product that may be evaluated in this article, or claim that may be made by its manufacturer, is not guaranteed or endorsed by the publisher.
References
Altesha, M. A., Ni, T., Khan, A., Liu, K., and Zheng, X. (2019). Circular RNA in cardiovascular disease. J. Cell. physiology 234 (5), 5588–5600. doi:10.1002/jcp.27384
Ashwal-Fluss, R., Meyer, M., Pamudurti, N. R., Ivanov, A., Bartok, O., Hanan, M., et al. (2014). circRNA biogenesis competes with pre-mRNA splicing. Mol. Cell. 56 (1), 55–66. doi:10.1016/j.molcel.2014.08.019
Baetke, S. C., Lammers, T., and Kiessling, F. (2015). Applications of nanoparticles for diagnosis and therapy of cancer. Br. J. radiology 88 (1054), 20150207. doi:10.1259/bjr.20150207
Bao, C., Lyu, D., and Huang, S. (2016). Circular RNA expands its territory. Mol. Cell. Oncol. 3 (2), e1084443. doi:10.1080/23723556.2015.1084443
Cai, F., Fu, W., Tang, L., Tang, J., Sun, J., Fu, G., et al. (2021). Hsa_circ_0000515 is a novel circular RNA implicated in the development of breast cancer through its regulation of the microRNA-296-5p/CXCL10 axis. FEBS J. 288 (3), 861–883. doi:10.1111/febs.15373
Cao, L., Wang, M., Dong, Y., Xu, B., Chen, J., Ding, Y., et al. (2020). Circular RNA circRNF20 promotes breast cancer tumorigenesis and Warburg effect through miR-487a/HIF-1α/HK2. Cell. Death Dis. 11 (2), 145. doi:10.1038/s41419-020-2336-0
Chairoungdua, A., Smith, D. L., Pochard, P., Hull, M., and Caplan, M. J. (2010). Exosome release of β-catenin: A novel mechanism that antagonizes wnt signaling. J. Cell. Biol. 190 (6), 1079–1091. doi:10.1083/jcb.201002049
Chen, B., Wei, W., Huang, X., Xie, X., Kong, Y., Dai, D., et al. (2018). circEPSTI1 as a prognostic marker and mediator of triple-negative breast cancer progression. Theranostics 8 (14), 4003–4015. doi:10.7150/thno.24106
Chen, I., Chen, C. Y., and Chuang, T. J. (2015). Biogenesis, identification, and function of exonic circular RNAs. Wiley Interdiscip. Rev. RNA 6 (5), 563–579. doi:10.1002/wrna.1294
Chen, L. L., and Yang, L. (2015). Regulation of circRNA biogenesis. RNA Biol. 12 (4), 381–388. doi:10.1080/15476286.2015.1020271
Chen, N., Zhao, G., Yan, X., Lv, Z., Yin, H., Zhang, S., et al. (2018). A novel FLI1 exonic circular RNA promotes metastasis in breast cancer by coordinately regulating TET1 and DNMT1. Genome Biol. 19 (1), 218. doi:10.1186/s13059-018-1594-y
Chen, W., Zheng, R., Baade, P. D., Zhang, S., Zeng, H., Bray, F., et al. (2016). Cancer statistics in China, 2015. CA Cancer J. Clin. 66 (2), 115–132. doi:10.3322/caac.21338
Chen, Z. G., Zhao, H. J., Lin, L., Liu, J. B., Bai, J. Z., and Wang, G. S. (2020). Circular RNA CirCHIPK3 promotes cell proliferation and invasion of breast cancer by sponging miR-193a/HMGB1/PI3K/AKT axis. Thorac. Cancer 11 (9), 2660–2671. doi:10.1111/1759-7714.13603
Conn, S. J., Pillman, K. A., Toubia, J., Conn, V. M., Salmanidis, M., Phillips, C. A., et al. (2015). The RNA binding protein quaking regulates formation of circRNAs. Cell. 160 (6), 1125–1134. doi:10.1016/j.cell.2015.02.014
Cui, Y., Fan, J., Shi, W., and Zhou, Z. (2022). Circ_0001667 knockdown blocks cancer progression and attenuates adriamycin resistance by depleting NCOA3 via releasing miR-4458 in breast cancer. Drug Dev. Res. 83 (1), 75–87. doi:10.1002/ddr.21845
De Palma, F. D. E., Salvatore, F., Pol, J. G., Kroemer, G., and Maiuri, M. C. (2022). Circular RNAs as potential biomarkers in breast cancer. Biomedicines 10 (3), 725. doi:10.3390/biomedicines10030725
Dou, D., Ren, X., Han, M., Xu, X., Ge, X., Gu, Y., et al. (2020). CircUBE2D2 (hsa_circ_0005728) promotes cell proliferation, metastasis and chemoresistance in triple-negative breast cancer by regulating miR-512-3p/CDCA3 axis. Cancer Cell. Int. 20, 454. doi:10.1186/s12935-020-01547-7
Du, W. W., Yang, W., Li, X., Awan, F. M., Yang, Z., Fang, L., et al. (2018). A circular RNA circ-DNMT1 enhances breast cancer progression by activating autophagy. Oncogene 37 (44), 5829–5842. doi:10.1038/s41388-018-0369-y
Du, W. W., Yang, W., Li, X., Fang, L., Wu, N., Li, F., et al. (2020). The circular RNA circSKA3 binds integrin β1 to induce invadopodium formation enhancing breast cancer invasion. Mol. Ther. J. Am. Soc. Gene Ther. 28 (5), 1287–1298. doi:10.1016/j.ymthe.2020.03.002
El Andaloussi, S., Mäger, I., Breakefield, X. O., and Wood, M. J. (2013). Extracellular vesicles: Biology and emerging therapeutic opportunities. Nat. Rev. Drug Discov. 12 (5), 347–357. doi:10.1038/nrd3978
Fadaka, A., Ajiboye, B., Ojo, O., Adewale, O., Olayide, I., and Emuowhochere, R. (2017). Biology of glucose metabolization in cancer cells. J. Oncol. Sci. 3 (2), 45–51. doi:10.1016/j.jons.2017.06.002
Fan, Y., Wang, J., Jin, W., Sun, Y., Xu, Y., Wang, Y., et al. (2021). CircNR3C2 promotes HRD1-mediated tumor-suppressive effect via sponging miR-513a-3p in triple-negative breast cancer. Mol. cancer 20 (1), 25. doi:10.1186/s12943-021-01321-x
Fisusi, F. A., and Akala, E. O. (2019). Drug combinations in breast cancer therapy. Pharm. Nanotechnol. 7 (1), 3–23. doi:10.2174/2211738507666190122111224
Fu, B., Liu, W., Zhu, C., Li, P., Wang, L., Pan, L., et al. (2021). Circular RNA circBCBM1 promotes breast cancer brain metastasis by modulating miR-125a/BRD4 axis. Int. J. Biol. Sci. 17 (12), 3104–3117. doi:10.7150/ijbs.58916
Gao, D., Qi, X., Zhang, X., Fang, K., Guo, Z., and Li, L. (2019). hsa_circRNA_0006528 as a competing endogenous RNA promotes human breast cancer progression by sponging miR-7-5p and activating the MAPK/ERK signaling pathway. Mol. Carcinog. 58 (4), 554–564. doi:10.1002/mc.22950
Gao, D., Zhang, X., Liu, B., Meng, D., Fang, K., Guo, Z., et al. (2017). Screening circular RNA related to chemotherapeutic resistance in breast cancer. Epigenomics 9 (9), 1175–1188. doi:10.2217/epi-2017-0055
Geng, X., Lin, X., Zhang, Y., Li, Q., Guo, Y., Fang, C., et al. (2020). Exosomal circular RNA sorting mechanisms and their function in promoting or inhibiting cancer. Oncol. Lett. 19 (5), 3369–3380. doi:10.3892/ol.2020.11449
Ghafouri-Fard, S., Hussen, B. M., Taheri, M., and Ayatollahi, S. A. (2021). Emerging role of circular RNAs in breast cancer. Pathology, Res. Pract. 223, 153496. doi:10.1016/j.prp.2021.153496
Ghafouri-Fard, S., Khoshbakht, T., Hussen, B. M., Sarfaraz, S., Taheri, M., and Ayatollahi, S. A. (2022). Circ_CDR1as: A circular RNA with roles in the carcinogenesis. Pathology - Res. Pract. 236, 153968. doi:10.1016/j.prp.2022.153968
Ghafouri-Fard, S., Najafi, S., Hussen, B. M., Basiri, A., Hidayat, H. J., Taheri, M., et al. (2022). The role of circular RNAs in the carcinogenesis of bladder cancer. Front. Oncol. 12. doi:10.3389/fonc.2022.801842
Ghafouri-Fard, S., Niazi, V., Hussen, B. M., Omrani, M. D., Taheri, M., and Basiri, A. (2021). The emerging role of exosomes in the treatment of human disorders with a special focus on mesenchymal stem cells-derived exosomes. Front. Cell. Dev. Biol. 9, 653296. doi:10.3389/fcell.2021.653296
Guo, J. U., Agarwal, V., Guo, H., and Bartel, D. P. (2014). Expanded identification and characterization of mammalian circular RNAs. Genome Biol. 15 (7), 409. doi:10.1186/s13059-014-0409-z
Han, B., Chao, J., and Yao, H. (2018). Circular RNA and its mechanisms in disease: From the bench to the clinic. Pharmacol. Ther. 187, 31–44. doi:10.1016/j.pharmthera.2018.01.010
He, R., Liu, P., Xie, X., Zhou, Y., Liao, Q., Xiong, W., et al. (2017). circGFRA1 and GFRA1 act as ceRNAs in triple negative breast cancer by regulating miR-34a. J. Exp. Clin. cancer Res. CR 36 (1), 145. doi:10.1186/s13046-017-0614-1
Hegmans, J. P., Gerber, P. J., and Lambrecht, B. N. (2008). Exosomes. Methods Mol. Biol. 484, 97–109. doi:10.1007/978-1-59745-398-1_7
Henne, W. M., Stenmark, H., and Emr, S. D. (2013). Molecular mechanisms of the membrane sculpting ESCRT pathway. Cold Spring Harb. Perspect. Biol. 5 (9), a016766. doi:10.1101/cshperspect.a016766
Hou, J. C., Xu, Z., Zhong, S. L., Zhang, H. D., Jiang, L. H., Chen, X., et al. (2019). Circular RNA circASS1 is downregulated in breast cancer cells MDA-MB-231 and suppressed invasion and migration. Epigenomics 11 (2), 199–213. doi:10.2217/epi-2017-0167
Hu, K., Liu, X., Li, Y., Li, Q., Xu, Y., Zeng, W., et al. (2020). Exosomes mediated transfer of Circ_UBE2D2 enhances the resistance of breast cancer to tamoxifen by binding to MiR-200a-3p. Med. Sci. Monit. Int. Med. J. Exp. Clin. Res. 26, e922253. doi:10.12659/MSM.922253
Hu, Y., Guo, F., Zhu, H., Tan, X., Zhu, X., Liu, X., et al. (2020). Circular RNA-0001283 suppresses breast cancer proliferation and invasion via MiR-187/HIPK3 Axis. Med. Sci. Monit. Int. Med. J. Exp. Clin. Res. 26, e921502. doi:10.12659/MSM.921502
Huang, F. J., Dang, J. Q., Zhang, S., and Cheng, Z. Y. (2020). Circular RNA hsa_circ_0008039 promotes proliferation, migration and invasion of breast cancer cells through upregulating CBX4 via sponging miR-515-5p. Eur. Rev. Med. Pharmacol. Sci. 24 (4), 1887–1898. doi:10.26355/eurrev_202002_20367
Huang, Q., He, Y., Zhang, X., and Guo, L. (2021). Circular RNA hsa_circ_0103552 promotes proliferation, migration, and invasion of breast cancer cells through upregulating cysteine-rich angiogenic inducer 61 (CYR61) expression via sponging MicroRNA-515-5p. Tohoku J. Exp. Med. 255 (2), 171–181. doi:10.1620/tjem.255.171
Huang, Y., and Zhu, Q. (2021). Mechanisms regulating abnormal circular RNA biogenesis in cancer. Cancers (Basel) 13 (16), 4185. doi:10.3390/cancers13164185
Hurley, J. H., and Hanson, P. I. (2010). Membrane budding and scission by the ESCRT machinery: it's all in the neck. Nat. Rev. Mol. Cell. Biol. 11 (8), 556–566. doi:10.1038/nrm2937
Hüsemann, Y., Geigl, J. B., Schubert, F., Musiani, P., Meyer, M., Burghart, E., et al. (2008). Systemic spread is an early step in breast cancer. Cancer Cell. 13 (1), 58–68. doi:10.1016/j.ccr.2007.12.003
Hussen, B. M., Faraj, G. S. H., Rasul, M. F., Hidayat, H. J., Salihi, A., Baniahmad, A., et al. (2022). Strategies to overcome the main challenges of the use of exosomes as drug carrier for cancer therapy. Cancer Cell. Int. 22 (1), 323. doi:10.1186/s12935-022-02743-3
Jahani, S., Nazeri, E., Majidzadeh, A. K., Jahani, M., and Esmaeili, R. (2020). Circular RNA; a new biomarker for breast cancer: A systematic review. J. Cell. physiology 235 (7-8), 5501–5510. doi:10.1002/jcp.29558
Jahn, R., and Scheller, R. H. (2006). SNAREs-engines for membrane fusion. Nat. Rev. Mol. Cell. Biol. 7 (9), 631–643. doi:10.1038/nrm2002
Jeck, W. R., and Sharpless, N. E. (2014). Detecting and characterizing circular RNAs. Nat. Biotechnol. 32 (5), 453–461. doi:10.1038/nbt.2890
Jeck, W. R., Sorrentino, J. A., Wang, K., Slevin, M. K., Burd, C. E., Liu, J., et al. (2013). Circular RNAs are abundant, conserved, and associated with ALU repeats. Rna 19 (2), 141–157. doi:10.1261/rna.035667.112
Jia, Q., Ye, L., Xu, S., Xiao, H., Xu, S., Shi, Z., et al. (2020). Circular RNA 0007255 regulates the progression of breast cancer through miR-335-5p/SIX2 axis. Thorac. cancer 11 (3), 619–630. doi:10.1111/1759-7714.13306
Jia, Y., Chen, Y., Wang, Q., Jayasinghe, U., Luo, X., Wei, Q., et al. (2017). Exosome: Emerging biomarker in breast cancer. Oncotarget 8 (25), 41717–41733. doi:10.18632/oncotarget.16684
Jiang, J., and Cheng, X. (2020). Circular RNA circABCC4 acts as a ceRNA of miR-154-5p to improve cell viability, migration and invasion of breast cancer cells in vitro. Cell. cycleGeorget. Tex) 19 (20), 2653–2661. doi:10.1080/15384101.2020.1815147
Jin, J., Sun, H., Shi, C., Yang, H., Wu, Y., Li, W., et al. (2020a). Circular RNA in renal diseases. J. Cell. Mol. Med. 24 (12), 6523–6533. doi:10.1111/jcmm.15295
Jin, Y., Yang, L., Li, X., and Liu, F. (2020b). Circular RNA KIF4A promotes cell migration, invasion and inhibits apoptosis through miR-152/ZEB1 axis in breast cancer. Diagn. Pathol. 15 (1), 55. doi:10.1186/s13000-020-00963-7
Kaczanowski, S. (2016). Apoptosis: Its origin, history, maintenance and the medical implications for cancer and aging. Phys. Biol. 13 (3), 031001. doi:10.1088/1478-3975/13/3/031001
Kalluri, R., and LeBleu, V. S. (2020). The biology, function, and biomedical applications of exosomes. Science 367 (6478), eaau6977. doi:10.1126/science.aau6977
Kong, Y., Yang, L., Wei, W., Lyu, N., Zou, Y., Gao, G., et al. (2019). CircPLK1 sponges miR-296-5p to facilitate triple-negative breast cancer progression. Epigenomics 11 (10), 1163–1176. doi:10.2217/epi-2019-0093
Kowal, J., Tkach, M., and Théry, C. (2014). Biogenesis and secretion of exosomes. Curr. Opin. Cell. Biol. 29, 116–125. doi:10.1016/j.ceb.2014.05.004
Lambert, A. W., Pattabiraman, D. R., and Weinberg, R. A. (2017). Emerging biological principles of metastasis. Cell. 168 (4), 670–691. doi:10.1016/j.cell.2016.11.037
Lasda, E., and Parker, R. (2014). Circular RNAs: Diversity of form and function. Rna 20 (12), 1829–1842. doi:10.1261/rna.047126.114
Lener, T., Gimona, M., Aigner, L., Börger, V., Buzas, E., Camussi, G., et al. (2015). Applying extracellular vesicles based therapeutics in clinical trials–an ISEV position paper. J. Extracell. vesicles 4 (1), 30087. doi:10.3402/jev.v4.30087
Li, H., Yin, H., and Yan, Y. (2022a). Circ_0041732 regulates tumor properties of triple-negative breast cancer cells by the miR-149-5p/FGF5 pathway. Int. J. Biol. markers 37 (2), 178–190. doi:10.1177/03936155221086599
Li, H., Peng, K., Yang, K., Ma, W., Qi, S., Yu, X., et al. (2022b). Circular RNA cancer vaccines drive immunity in hard-to-treat malignancies. Theranostics 12 (14), 6422–6436. doi:10.7150/thno.77350
Li, H., Xu, W., Xia, Z., Liu, W., Pan, G., Ding, J., et al. (2021). Hsa_circ_0000199 facilitates chemo-tolerance of triple-negative breast cancer by interfering with miR-206/613-led PI3K/Akt/mTOR signaling. Aging 13 (3), 4522–4551. doi:10.18632/aging.202415
Li, J., Sun, D., Pu, W., Wang, J., and Peng, Y. (2020). Circular RNAs in cancer: Biogenesis, function, and clinical significance. Trends cancer 6 (4), 319–336. doi:10.1016/j.trecan.2020.01.012
Li, W., Yang, X., Shi, C., and Zhou, Z. (2020). Hsa_circ_002178 promotes the growth and migration of breast cancer cells and maintains cancer stem-like cell properties through regulating miR-1258/kdm7a Axis. Cell. Transplant. 29, 963689720960174. doi:10.1177/0963689720960174
Li, Y., and Li, H. (2020). Circular RNA VRK1 correlates with favourable prognosis, inhibits cell proliferation but promotes apoptosis in breast cancer. J. Clin. laboratory analysis 34 (1), e22980. doi:10.1002/jcla.22980
Li, Y., Shi, P., Zheng, T., Ying, Z., and Jiang, D. (2020). Circular RNA hsa_circ_0131242 promotes triple-negative breast cancer progression by sponging hsa-miR-2682. OncoTargets Ther. 13, 4791–4798. doi:10.2147/OTT.S246957
Li, Y., Zheng, Q., Bao, C., Li, S., Guo, W., Zhao, J., et al. (2015). Circular RNA is enriched and stable in exosomes: A promising biomarker for cancer diagnosis. Cell. Res. 25 (8), 981–984. doi:10.1038/cr.2015.82
Li, Z., Huang, C., Bao, C., Chen, L., Lin, M., Wang, X., et al. (2015). Exon-intron circular RNAs regulate transcription in the nucleus. Nat. Struct. Mol. Biol. 22 (3), 256–264. doi:10.1038/nsmb.2959
Liang, G., Ling, Y., Mehrpour, M., Saw, P. E., Liu, Z., Tan, W., et al. (2020). Autophagy-associated circRNA circCDYL augments autophagy and promotes breast cancer progression. Mol. cancer 19 (1), 65. doi:10.1186/s12943-020-01152-2
Liang, H. F., Zhang, X. Z., Liu, B. G., Jia, G. T., and Li, W. L. (2017). Circular RNA circ-ABCB10 promotes breast cancer proliferation and progression through sponging miR-1271. Am. J. Cancer Res. 7 (7), 1566–1576.
Liang, Y., Song, X., Li, Y., Ma, T., Su, P., Guo, R., et al. (2019a). Targeting the circBMPR2/miR-553/USP4 Axis as a potent therapeutic approach for breast cancer. Mol. Ther. Nucleic acids 17, 347–361. doi:10.1016/j.omtn.2019.05.005
Liang, Y., Song, X., Li, Y., Su, P., Han, D., Ma, T., et al. (2019b). circKDM4C suppresses tumor progression and attenuates doxorubicin resistance by regulating miR-548p/PBLD axis in breast cancer. Oncogene 38 (42), 6850–6866. doi:10.1038/s41388-019-0926-z
Liu, M., Luo, C., Dong, J., Guo, J., Luo, Q., Ye, C., et al. (2020b). CircRNA_103809 suppresses the proliferation and metastasis of breast cancer cells by sponging MicroRNA-532-3p (miR-532-3p). Front. Genet. 11, 485. doi:10.3389/fgene.2020.00485
Liu, P., Zou, Y., Li, X., Yang, A., Ye, F., Zhang, J., et al. (2020c). circGNB1 facilitates triple-negative breast cancer progression by regulating miR-141-5p-igf1r Axis. Front. Genet. 11, 193. doi:10.3389/fgene.2020.00193
Liu, T., Ye, P., Ye, Y., Lu, S., and Han, B. (2020a). Circular RNA hsa_circRNA_002178 silencing retards breast cancer progression via microRNA-328-3p-mediated inhibition of COL1A1. J. Cell. Mol. Med. 24 (3), 2189–2201. doi:10.1111/jcmm.14875
Liu, X. Y., Zhang, Q., Guo, J., Zhang, P., Liu, H., Tian, Z. B., et al. (2021). The role of circular RNAs in the drug resistance of cancers. Front. Oncol. 11, 790589. doi:10.3389/fonc.2021.790589
Liu, Y., Dong, Y., Zhao, L., Su, L., and Luo, J. (2018). Circular RNA-MTO1 suppresses breast cancer cell viability and reverses monastrol resistance through regulating the TRAF4/Eg5 axis. Int. J. Oncol. 53 (4), 1752–1762. doi:10.3892/ijo.2018.4485
Liu, Z., Zhou, Y., Liang, G., Ling, Y., Tan, W., Tan, L., et al. (2019). Circular RNA hsa_circ_001783 regulates breast cancer progression via sponging miR-200c-3p. Cell. Death Dis. 10 (2), 55. doi:10.1038/s41419-018-1287-1
Lü, L., Sun, J., Shi, P., Kong, W., Xu, K., He, B., et al. (2017). Identification of circular RNAs as a promising new class of diagnostic biomarkers for human breast cancer. Oncotarget 8 (27), 44096–44107. doi:10.18632/oncotarget.17307
Lu, M., Wu, Y., Zeng, B., Sun, J., Li, Y., Luo, J., et al. (2020). CircEHMT1 inhibits metastatic potential of breast cancer cells by modulating miR-1233-3p/KLF4/MMP2 axis. Biochem. biophysical Res. Commun. 526 (2), 306–313. doi:10.1016/j.bbrc.2020.03.084
Ma, J., Fang, L., Yang, Q., Hibberd, S., Du, W. W., Wu, N., et al. (2019). Posttranscriptional regulation of AKT by circular RNA angiomotin-like 1 mediates chemoresistance against paclitaxel in breast cancer cells. Aging 11 (23), 11369–11381. doi:10.18632/aging.102535
Malmgren, J. A., Mayer, M., Atwood, M. K., and Kaplan, H. G. (2018). Differential presentation and survival of de novo and recurrent metastatic breast cancer over time: 1990-2010. Breast Cancer Res. Treat. 167 (2), 579–590. doi:10.1007/s10549-017-4529-5
Mao, Y., Lv, M., Cao, W., Liu, X., Cui, J., Wang, Y., et al. (2020). Circular RNA 000554 represses epithelial-mesenchymal transition in breast cancer by regulating microRNA-182/ZFP36 axis. FASEB J. 34 (9), 11405–11420. doi:10.1096/fj.201903047R
Mathieu, M., Martin-Jaular, L., Lavieu, G., and Théry, C. (2019). Specificities of secretion and uptake of exosomes and other extracellular vesicles for cell-to-cell communication. Nat. Cell. Biol. 21 (1), 9–17. doi:10.1038/s41556-018-0250-9
Mattissek, C., and Teis, D. (2014). The role of the endosomal sorting complexes required for transport (ESCRT) in tumorigenesis. Mol. Membr. Biol. 31 (4), 111–119. doi:10.3109/09687688.2014.894210
Mecozzi, N., Nenci, A., Vera, O., Bok, I., Falzone, A., DeNicola, G. M., et al. (2022). Genetic tools for the stable overexpression of circular RNAs. RNA Biol. 19 (1), 353–363. doi:10.1080/15476286.2022.2043041
Meng, L., Chang, S., Sang, Y., Ding, P., Wang, L., Nan, X., et al. (2022). Circular RNA circCCDC85A inhibits breast cancer progression via acting as a miR-550a-5p sponge to enhance MOB1A expression. Breast cancer Res. BCR 24 (1), 1. doi:10.1186/s13058-021-01497-6
Meng, Y., Wang, W., Kang, J., Wang, X., and Sun, L. (2017). Role of the PI3K/AKT signalling pathway in apoptotic cell death in the cerebral cortex of streptozotocin-induced diabetic rats. Exp. Ther. Med. 13 (5), 2417–2422. doi:10.3892/etm.2017.4259
Mu, Q., Wang, H., and Zhang, M. (2017). Nanoparticles for imaging and treatment of metastatic breast cancer. Expert Opin. drug Deliv. 14 (1), 123–136. doi:10.1080/17425247.2016.1208650
Ostrowski, M., Carmo, N. B., Krumeich, S., Fanget, I., Raposo, G., Savina, A., et al. (2010). Rab27a and Rab27b control different steps of the exosome secretion pathway. Nat. Cell. Biol. 12 (1), 19–30. sup pp 1-13. doi:10.1038/ncb2000
Pan, G., Mao, A., Liu, J., Lu, J., Ding, J., and Liu, W. (2020). Circular RNA hsa_circ_0061825 (circ-TFF1) contributes to breast cancer progression through targeting miR-326/TFF1 signalling. Cell. Prolif. 53 (2), e12720. doi:10.1111/cpr.12720
Parker, A. L., Benguigui, M., Fornetti, J., Goddard, E., Lucotti, S., Insua-Rodríguez, J., et al. (2022). Current challenges in metastasis research and future innovation for clinical translation. Clin. Exp. Metastasis 39 (2), 263–277. doi:10.1007/s10585-021-10144-5
Peng, H. H., and Wen, Y. G. (2020). CircDDX17 acts as a competing endogenous RNA for miR-605 in breast cancer progression. Eur. Rev. Med. Pharmacol. Sci. 24 (12), 6794–6801. doi:10.26355/eurrev_202006_21668
Perez-Hernandez, D., Gutiérrez-Vázquez, C., Jorge, I., López-Martín, S., Ursa, A., Sánchez-Madrid, F., et al. (2013). The intracellular interactome of tetraspanin-enriched microdomains reveals their function as sorting machineries toward exosomes. J. Biol. Chem. 288 (17), 11649–11661. doi:10.1074/jbc.M112.445304
Raposo, G., and Stoorvogel, W. (2013). Extracellular vesicles: Exosomes, microvesicles, and friends. J. Cell. Biol. 200 (4), 373–383. doi:10.1083/jcb.201211138
Ren, S., Liu, J., Feng, Y., Li, Z., He, L., Li, L., et al. (2019). Knockdown of circDENND4C inhibits glycolysis, migration and invasion by up-regulating miR-200b/c in breast cancer under hypoxia. J. Exp. Clin. Cancer Res. 38 (1), 388. doi:10.1186/s13046-019-1398-2
Rossbach, O. (2019). Artificial circular RNA sponges targeting MicroRNAs as a novel tool in molecular biology. Mol. Ther. Nucleic Acids 17, 452–454. doi:10.1016/j.omtn.2019.06.021
Sang, Y., Chen, B., Song, X., Li, Y., Liang, Y., Han, D., et al. (2019). circRNA_0025202 regulates tamoxifen sensitivity and tumor progression via regulating the miR-182-5p/FOXO3a Axis in breast cancer. Mol. Ther. J. Am. Soc. Gene Ther. 27 (9), 1638–1652. doi:10.1016/j.ymthe.2019.05.011
Seimiya, T., Otsuka, M., Iwata, T., Shibata, C., Tanaka, E., Suzuki, T., et al. (2020). Emerging roles of exosomal circular RNAs in cancer. Front. Cell. Dev. Biol. 8, 568366. doi:10.3389/fcell.2020.568366
Siegel, R. L., Miller, K. D., and Jemal, A. (2018). Cancer statistics, 2018. CA Cancer J. Clin. 68 (1), 7–30. doi:10.3322/caac.21442
Song, L., Tang, S., Han, X., Jiang, Z., Dong, L., Liu, C., et al. (2019). KIBRA controls exosome secretion via inhibiting the proteasomal degradation of Rab27a. Nat. Commun. 10 (1), 1639. doi:10.1038/s41467-019-09720-x
Song, L., and Xiao, Y. (2018). Downregulation of hsa_circ_0007534 suppresses breast cancer cell proliferation and invasion by targeting miR-593/MUC19 signal pathway. Biochem. biophysical Res. Commun. 503 (4), 2603–2610. doi:10.1016/j.bbrc.2018.08.007
Song, X., Liang, Y., Sang, Y., Li, Y., Zhang, H., Chen, B., et al. (2020). circHMCU promotes proliferation and metastasis of breast cancer by sponging the let-7 family. Mol. Ther. Nucleic acids 20, 518–533. doi:10.1016/j.omtn.2020.03.014
Stenmark, H. (2009). Rab GTPases as coordinators of vesicle traffic. Nat. Rev. Mol. Cell. Biol. 10 (8), 513–525. doi:10.1038/nrm2728
Stuffers, S., Sem Wegner, C., Stenmark, H., and Brech, A. (2009). Multivesicular endosome biogenesis in the absence of ESCRTs. Traffic 10 (7), 925–937. doi:10.1111/j.1600-0854.2009.00920.x
Su, M., Xiao, Y., Ma, J., Tang, Y., Tian, B., Zhang, Y., et al. (2019). Circular RNAs in cancer: Emerging functions in hallmarks, stemness, resistance and roles as potential biomarkers. Mol. Cancer 18 (1), 90. doi:10.1186/s12943-019-1002-6
Tang, H., Huang, X., Wang, J., Yang, L., Kong, Y., Gao, G., et al. (2019). circKIF4A acts as a prognostic factor and mediator to regulate the progression of triple-negative breast cancer. Mol. cancer 18 (1), 23. doi:10.1186/s12943-019-0946-x
Tang, Y. Y., Zhao, P., Zou, T. N., Duan, J. J., Zhi, R., Yang, S. Y., et al. (2017). Circular RNA hsa_circ_0001982 promotes breast cancer cell carcinogenesis through decreasing miR-143. DNA Cell. Biol. 36 (11), 901–908. doi:10.1089/dna.2017.3862
Tesfaye, E., Martinez-Terroba, E., Bendor, J., Winkler, L., Olivero, C., Chen, K., et al. (2021). The p53 transcriptional response across tumor types reveals core and senescence-specific signatures modulated by long noncoding RNAs. Proc. Natl. Acad. Sci. 118 (31), e2025539118. doi:10.1073/pnas.2025539118
Torre, L. A., Bray, F., Siegel, R. L., Ferlay, J., Lortet-Tieulent, J., and Jemal, A. (2015). Global cancer statistics, 2012. CA Cancer J. Clin. 65 (2), 87–108. doi:10.3322/caac.21262
Tran, A. M., Chalbatani, G. M., Berland, L., Cruz De Los Santos, M., Raj, P., Jalali, S. A., et al. (2020). A new world of biomarkers and therapeutics for female reproductive system and breast cancers: Circular RNAs. Front. Cell. Dev. Biol. 8, 50. doi:10.3389/fcell.2020.00050
van Niel, G., Charrin, S., Simoes, S., Romao, M., Rochin, L., Saftig, P., et al. (2011). The tetraspanin CD63 regulates ESCRT-independent and -dependent endosomal sorting during melanogenesis. Dev. Cell. 21 (4), 708–721. doi:10.1016/j.devcel.2011.08.019
Wang, H., Xiao, Y., Wu, L., and Ma, D. (2018a). Comprehensive circular RNA profiling reveals the regulatory role of the circRNA-000911/miR-449a pathway in breast carcinogenesis. Int. J. Oncol. 52 (3), 743–754. doi:10.3892/ijo.2018.4265
Wang, N., Gu, Y., Li, L., Wang, F., Lv, P., Xiong, Y., et al. (2018b). Circular RNA circMYO9B facilitates breast cancer cell proliferation and invasiveness via upregulating FOXP4 expression by sponging miR-4316. Archives Biochem. biophysics 653, 63–70. doi:10.1016/j.abb.2018.04.017
Wang, S., Li, Q., Wang, Y., Li, X., Wang, R., Kang, Y., et al. (2018c). Upregulation of circ-UBAP2 predicts poor prognosis and promotes triple-negative breast cancer progression through the miR-661/MTA1 pathway. Biochem. biophysical Res. Commun. 505 (4), 996–1002. doi:10.1016/j.bbrc.2018.10.026
Wang, Q., Liang, D., Shen, P., Yu, Y., Yan, Y., and You, W. (2021). Hsa_circ_0092276 promotes doxorubicin resistance in breast cancer cells by regulating autophagy via miR-348/ATG7 axis. Transl. Oncol. 14 (8), 101045. doi:10.1016/j.tranon.2021.101045
Wang, Y., Liu, J., Ma, J., Sun, T., Zhou, Q., Wang, W., et al. (2019a). Exosomal circRNAs: Biogenesis, effect and application in human diseases. Mol. Cancer 18 (1), 116. doi:10.1186/s12943-019-1041-z
Wang, Q., Li, Z., Hu, Y., Zheng, W., Tang, W., Zhai, C., et al. (2019b). Circ-TFCP2L1 promotes the proliferation and migration of triple negative breast cancer through sponging miR-7 by inhibiting PAK1. J. mammary Gl. Biol. neoplasia 24 (4), 323–331. doi:10.1007/s10911-019-09440-4
Wang, S. T., Liu, L. B., Li, X. M., Wang, Y. F., Xie, P. J., Li, Q., et al. (2019c). Circ-ITCH regulates triple-negative breast cancer progression through the Wnt/β-catenin pathway. Neoplasma 66 (2), 232–239. doi:10.4149/neo_2018_180710N460
Wei, D., Zhan, W., Gao, Y., Huang, L., Gong, R., Wang, W., et al. (2021). RAB31 marks and controls an ESCRT-independent exosome pathway. Cell. Res. 31 (2), 157–177. doi:10.1038/s41422-020-00409-1
Wittekind, C., and Neid, M. (2005). Cancer invasion and metastasis. Oncology 69 (1), 14–16. doi:10.1159/000086626
Wu, J., Jiang, Z., Chen, C., Hu, Q., Fu, Z., Chen, J., et al. (2018). CircIRAK3 sponges miR-3607 to facilitate breast cancer metastasis. Cancer Lett. 430, 179–192. doi:10.1016/j.canlet.2018.05.033
Wu, N., Qadir, J., and Yang, B. B. (2022). CircRNA perspective: New strategies for RNA therapy. Trends Mol. Med. 28 (4), 343–344. doi:10.1016/j.molmed.2022.02.002
Wu, X., Ren, Y., Yao, R., Zhou, L., and Fan, R. (2021). Circular RNA circ-MMP11 contributes to lapatinib resistance of breast cancer cells by regulating the miR-153-3p/ANLN Axis. Front. Oncol. 11, 639961. doi:10.3389/fonc.2021.639961
Wu, X., Xiao, Y., Ma, J., and Wang, A. (2020). Circular RNA: A novel potential biomarker for skin diseases. Pharmacol. Res. 158, 104841. doi:10.1016/j.phrs.2020.104841
Xiao, W., Zheng, S., Zou, Y., Yang, A., Xie, X., Tang, H., et al. (2019). CircAHNAK1 inhibits proliferation and metastasis of triple-negative breast cancer by modulating miR-421 and RASA1. Aging 11 (24), 12043–12056. doi:10.18632/aging.102539
Xie, H., and Zheng, R. (2022). Circ_0085495 knockdown reduces adriamycin resistance in breast cancer through miR-873-5p/integrin β1 axis. Anti-cancer drugs 33 (1), e166–e177. doi:10.1097/CAD.0000000000001174
Xie, R., Tang, J., Zhu, X., and Jiang, H. (2019). Silencing of hsa_circ_0004771 inhibits proliferation and induces apoptosis in breast cancer through activation of miR-653 by targeting ZEB2 signaling pathway. Biosci. Rep. 39 (5), BSR20181919. doi:10.1042/BSR20181919
Xing, L., Yang, R., Wang, X., Zheng, X., Yang, X., Zhang, L., et al. (2020). The circRNA circIFI30 promotes progression of triple-negative breast cancer and correlates with prognosis. Aging 12 (11), 10983–11003. doi:10.18632/aging.103311
Xu, J. H., Wang, Y., and Xu, D. (2019). Hsa_circ_001569 is an unfavorable prognostic factor and promotes cell proliferation and metastasis by modulating PI3K-AKT pathway in breast cancer. Cancer biomarkers Sect. A Dis. markers 25 (2), 193–201. doi:10.3233/CBM-182293
Xu, J. Z., Shao, C. C., Wang, X. J., Zhao, X., Chen, J. Q., Ouyang, Y. X., et al. (2019). circTADA2As suppress breast cancer progression and metastasis via targeting miR-203a-3p/SOCS3 axis. Cell. Death Dis. 10 (3), 175. doi:10.1038/s41419-019-1382-y
Xu, G., Ye, D., Zhao, Q., He, R., Ma, W., Li, Y., et al. (2020a). circNFIC suppresses breast cancer progression by sponging miR-658. J. Cancer 11 (14), 4222–4229. doi:10.7150/jca.38830
Xu, Y., Kong, S., Qin, S., Shen, X., and Ju, S. (2020). Exosomal circRNAs: Sorting mechanisms, roles and clinical applications in tumors. Front. Cell. Dev. Biol. 8, 581558. doi:10.3389/fcell.2020.581558
Xu, Y., Yao, Y., Leng, K., Ji, D., Qu, L., Liu, Y., et al. (2018). Increased expression of circular RNA circ_0005230 indicates dismal prognosis in breast cancer and regulates cell proliferation and invasion via miR-618/CBX8 signal pathway. Cell. physiology Biochem. 51 (4), 1710–1722. doi:10.1159/000495675
Yan, L., Zheng, M., and Wang, H. (2019). Circular RNA hsa_circ_0072309 inhibits proliferation and invasion of breast cancer cells via targeting miR-492. Cancer Manag. Res. 11, 1033–1041. doi:10.2147/CMAR.S186857
Yang, L., Peng, X., Li, Y., Zhang, X., Ma, Y., Wu, C., et al. (2019a). Long non-coding RNA HOTAIR promotes exosome secretion by regulating RAB35 and SNAP23 in hepatocellular carcinoma. Mol. Cancer 18 (1), 78. doi:10.1186/s12943-019-0990-6
Yang, L., Song, C., Chen, Y., Jing, G., and Sun, J. (2019b). Circular RNA circ_0103552 forecasts dismal prognosis and promotes breast cancer cell proliferation and invasion by sponging miR-1236. J. Cell. Biochem. 120 (9), 15553–15560. doi:10.1002/jcb.28822
Yang, R., Xing, L., Zheng, X., Sun, Y., Wang, X., and Chen, J. (2019c). The circRNA circAGFG1 acts as a sponge of miR-195-5p to promote triple-negative breast cancer progression through regulating CCNE1 expression. Mol. cancer 18 (1), 4. doi:10.1186/s12943-018-0933-7
Yang, Q., Du, W. W., Wu, N., Yang, W., Awan, F. M., Fang, L., et al. (2017). A circular RNA promotes tumorigenesis by inducing c-myc nuclear translocation. Cell. death Differ. 24 (9), 1609–1620. doi:10.1038/cdd.2017.86
Yang, W., Gong, P., Yang, Y., Yang, C., Yang, B., and Ren, L. (2020). Circ-ABCB10 contributes to paclitaxel resistance in breast cancer through let-7a-5p/DUSP7 Axis. Cancer Manag. Res. 12, 2327–2337. doi:10.2147/CMAR.S238513
Ye, D., Gong, M., Deng, Y., Fang, S., Cao, Y., Xiang, Y., et al. (2022). Roles and clinical application of exosomal circRNAs in the diagnosis and treatment of malignant tumors. J. Transl. Med. 20 (1), 161. doi:10.1186/s12967-022-03367-x
Ye, F., Gao, G., Zou, Y., Zheng, S., Zhang, L., Ou, X., et al. (2019). circFBXW7 inhibits malignant progression by sponging miR-197-3p and encoding a 185-aa protein in triple-negative breast cancer. Mol. Ther. Nucleic acids 18, 88–98. doi:10.1016/j.omtn.2019.07.023
Yi, Z., Li, Y., Wu, Y., Zeng, B., Li, H., Ren, G., et al. (2020). Circular RNA 0001073 attenuates malignant biological behaviours in breast cancer cell and is delivered by nanoparticles to inhibit mice tumour growth. OncoTargets Ther. 13, 6157–6169. doi:10.2147/OTT.S248822
Yin, W. B., Yan, M. G., Fang, X., Guo, J. J., Xiong, W., and Zhang, R. P. (2018). Circulating circular RNA hsa_circ_0001785 acts as a diagnostic biomarker for breast cancer detection. Clin. chimica acta; Int. J. Clin. Chem. 487, 363–368. doi:10.1016/j.cca.2017.10.011
Yuan, P., Lei, L., Dong, S., and Liu, D. (2020). Circular RNA hsa_circ_0068033 acts as a diagnostic biomarker and suppresses the progression of breast cancer through sponging miR-659. OncoTargets Ther. 13, 1921–1929. doi:10.2147/OTT.S223542
Zang, H., Li, Y., Zhang, X., and Huang, G. (2020). Circ-RNF111 contributes to paclitaxel resistance in breast cancer by elevating E2F3 expression via miR-140-5p. Thorac. cancer 11 (7), 1891–1903. doi:10.1111/1759-7714.13475
Zeng, K., He, B., Yang, B. B., Xu, T., Chen, X., Xu, M., et al. (2018). The pro-metastasis effect of circANKS1B in breast cancer. Mol. cancer 17 (1), 160. doi:10.1186/s12943-018-0914-x
Zeng, X., Yuan, X., Cai, Q., Tang, C., and Gao, J. (2021). Circular RNA as an epigenetic regulator in chronic liver diseases. Cells 10 (8), 1945. doi:10.3390/cells10081945
Zhang, J., Xu, H. D., Xing, X. J., Liang, Z. T., Xia, Z. H., and Zhao, Y. (2019). CircRNA_069718 promotes cell proliferation and invasion in triple-negative breast cancer by activating Wnt/β-catenin pathway. Eur. Rev. Med. Pharmacol. Sci. 23 (12), 5315–5322. doi:10.26355/eurrev_201906_18198
Zhang, L., Liang, D., Chen, C., Wang, Y., Amu, G., Yang, J., et al. (2018a). Circular siRNAs for reducing off-target effects and enhancing long-term gene silencing in cells and mice. Mol. Therapy-Nucleic Acids. 10, 237–244. doi:10.1016/j.omtn.2017.12.007
Zhang, H. D., Jiang, L. H., Hou, J. C., Zhong, S. L., Zhou, S. Y., Zhu, L. P., et al. (2018b). Circular RNA hsa_circ_0052112 promotes cell migration and invasion by acting as sponge for miR-125a-5p in breast cancer. Biomed. Pharmacother. = Biomedecine Pharmacother. 107, 1342–1353. doi:10.1016/j.biopha.2018.08.030
Zhang, H. D., Jiang, L. H., Hou, J. C., Zhou, S. Y., Zhong, S. L., Zhu, L. P., et al. (2018c). Circular RNA hsa_circ_0072995 promotes breast cancer cell migration and invasion through sponge for miR-30c-2-3p. Epigenomics 10 (9), 1229–1242. doi:10.2217/epi-2018-0002
Zhang, X., Su, X., Guo, Z., Jiang, X., and Li, X. (2020). Circular RNA La-related RNA-binding protein 4 correlates with reduced tumor stage, as well as better prognosis, and promotes chemosensitivity to doxorubicin in breast cancer. J. Clin. laboratory analysis 34 (7), e23272. doi:10.1002/jcla.23272
Zhang, X. O., Wang, H. B., Zhang, Y., Lu, X., Chen, L. L., and Yang, L. (2014). Complementary sequence-mediated exon circularization. Cell. 159 (1), 134–147. doi:10.1016/j.cell.2014.09.001
Zhang, X. Y., and Mao, L. (2021). Circular RNA Circ_0000442 acts as a sponge of MiR-148b-3p to suppress breast cancer via PTEN/PI3K/Akt signaling pathway. Gene 766, 145113. doi:10.1016/j.gene.2020.145113
Zhang, Y., Zhang, X. O., Chen, T., Xiang, J. F., Yin, Q. F., Xing, Y. H., et al. (2013). Circular intronic long noncoding RNAs. Mol. Cell. 51 (6), 792–806. doi:10.1016/j.molcel.2013.08.017
Zhao, B., Song, X., and Guan, H. (2020a). CircACAP2 promotes breast cancer proliferation and metastasis by targeting miR-29a/b-3p-COL5A1 axis. Life Sci. 244, 117179. doi:10.1016/j.lfs.2019.117179
Zhao, C., Li, L., Li, Z., Xu, J., Yang, Q., Shi, P., et al. (2020b). A novel circular RNA hsa_circRPPH1_015 exerts an oncogenic role in breast cancer by impairing miRNA-326-mediated ELK1 inhibition. Front. Oncol. 10, 906. doi:10.3389/fonc.2020.00906
Zhao, Z., Zhao, G., Yang, S., Zhu, S., Zhang, S., and Li, P. (2021). The significance of exosomal RNAs in the development, diagnosis, and treatment of pancreatic cancer. Cancer Cell. Int. 21 (1), 364. doi:10.1186/s12935-021-02059-8
Zheng, S. R., Huang, Q. D., Zheng, Z. H., Zhang, Z. T., and Guo, G. L. (2021). circGFRA1 affects the sensitivity of triple-negative breast cancer cells to paclitaxel via the miR-361-5p/TLR4 pathway. J. Biochem. 169 (5), 601–611. doi:10.1093/jb/mvaa148
Zheng, X., Huang, M., Xing, L., Yang, R., Wang, X., Jiang, R., et al. (2020). The circRNA circSEPT9 mediated by E2F1 and EIF4A3 facilitates the carcinogenesis and development of triple-negative breast cancer. Mol. cancer 19 (1), 73. doi:10.1186/s12943-020-01183-9
Zhou, H., Tang, G., Zhao, M., Xie, L., Xie, Y., Zhang, Z., et al. (2020a). circFBXL5 promotes breast cancer progression by sponging miR-660. J. Cell. Mol. Med. 24 (1), 356–361. doi:10.1111/jcmm.14737
Zhou, J., Zhang, W. W., Peng, F., Sun, J. Y., He, Z. Y., and Wu, S. G. (2018). Downregulation of hsa_circ_0011946 suppresses the migration and invasion of the breast cancer cell line MCF-7 by targeting RFC3. Cancer Manag. Res. 10, 535–544. doi:10.2147/CMAR.S155923
Zhou, S. Y., Chen, W., Yang, S. J., Li, J., Zhang, J. Y., Zhang, H. D., et al. (2020b). Circular RNA circVAPA regulates breast cancer cell migration and invasion via sponging miR-130a-5p. Epigenomics 12 (4), 303–317. doi:10.2217/epi-2019-0124
Zhu, M., Wang, Y., Wang, F., Li, L., and Qiu, X. (2021). CircFBXL5 promotes the 5-FU resistance of breast cancer via modulating miR-216b/HMGA2 axis. Cancer Cell. Int. 21 (1), 384. doi:10.1186/s12935-021-02088-3
Zou, Y., Zheng, S., Xiao, W., Xie, X., Yang, A., Gao, G., et al. (2019). circRAD18 sponges miR-208a/3164 to promote triple-negative breast cancer progression through regulating IGF1 and FGF2 expression. Carcinogenesis 40 (12), 1469–1479. doi:10.1093/carcin/bgz071
Glossary
ANLN Anillin, Actin Binding Protein
ASS1 Argininosuccinate Synthase 1
ATG7 Autophagy Related 7
CDCA3 cell division cycle associated 3
CDK Cyclin-Dependent Kinase
CircRNA circular RNA
CYR61 Cysteine-rich angiogenic inducer 61
E2F3 E2F Transcription Factor 3
Exo-circRNA exosomal circularRNA
FFPE formalin-fixed, paraffin embedded
FGF2 Fibroblast Growth Factor 2
FOXO3 Forkhead Box O3
HIF-1α hypoxia-inducible factor-1α
HIPK3 Homeodomain Interacting Protein Kinase 3
HMGA2 high-mobility group AT-hook 2
HRD1 E3 ubiquitin-protein ligase HRD1
IGF1 Insulin Like Growth Factor 1
MMP9 matrix metallopeptidase 9
MVEs multivesicular endosomes
MTA1 Metastasis Associated 1
NCOA3 Nuclear Receptor Coactivator 3
NF-κB Nuclear factor-κB
Notch1 Neurogenic locus notch homolog protein 1
p53 Tumor Protein P53
PAK1 p21 protein-activated kinase 1
PTEN Phosphatase and Tensin Homolog
RASA1 Ras p21 protein activator 1
RFC3 Replication factor C subunit 3
SOCS3 Suppressor of Cytokine Signalling 3
SRSF6 Serine and Arginine Rich Splicing Factor 6
TLR4 Toll Like Receptor 4
TNBC Triple negative breast cancer
TNM Tumor (T), nodes (N), and metastases (M)
TRAF4 TNF Receptor Associated Factor 4
Keywords: breast cancer (BC), exosome, circular RNA (circRNA), exosomal circRNA (exo-circRNA), therapeutic target
Citation: Hussen BM, Mohamadtahr S, Abdullah SR, Hidayat HJ, Rasul MF, Hama Faraj GS, Ghafouri-Fard S, Taheri M, Khayamzadeh M and Jamali E (2023) Exosomal circular RNAs: New player in breast cancer progression and therapeutic targets. Front. Genet. 14:1126944. doi: 10.3389/fgene.2023.1126944
Received: 18 December 2022; Accepted: 20 February 2023;
Published: 28 February 2023.
Edited by:
Manoj Kumar Kashyap, Amity University Gurgaon, IndiaReviewed by:
Manju Kashyap, San Sebastián University, ChileXichen Bao, Guangzhou Institutes of Biomedicine and Health (CAS), China
Copyright © 2023 Hussen, Mohamadtahr, Abdullah, Hidayat, Rasul, Hama Faraj, Ghafouri-Fard, Taheri, Khayamzadeh and Jamali. This is an open-access article distributed under the terms of the Creative Commons Attribution License (CC BY). The use, distribution or reproduction in other forums is permitted, provided the original author(s) and the copyright owner(s) are credited and that the original publication in this journal is cited, in accordance with accepted academic practice. No use, distribution or reproduction is permitted which does not comply with these terms.
*Correspondence: Mohammad Taheri, mohammad_823@yahoo.com; Maryam Khayamzadeh, khayamzadeh@yahoo.com; Elena Jamali, Elena.jamali@yahoo.com