- 1Key Laboratory of Xinjiang Phytomedicine Resource and Utilization of Ministry of Education, Key Laboratory of Oasis Town and Mountain-basin System Ecology of Xinjiang Production and Construction Corps, College of Life Sciences, Shihezi University, Shihezi, China
- 2College of Life Sciences, Shaanxi Normal University, Xi’an, China
- 3National Key Laboratory of Cotton Biology, Institute of Cotton Research (CAAS), Anyang, China
Introduction: Cotton is an important economic crop to provide natural fibers as raw materials to textile industry, and is significantly affected by biotic and abiotic stress during the whole growth stage, in which Verticillium wilt (VW) caused by Verticillium dahliae is one of the most destructive disease to lead to a significant yield reduction. Heat shock proteins (Hsps) are important molecular chaperones, and play crucial roles in plant growth, development, resistance to biotic and abiotic stress. Hsp40 and Hsp70 are two key Hsps in cell chaperone network, however, the function and regulatory mechanism of Hsp40 and Hsp70 members in VW resistance and abiotic stress in cotton are largely unknown.
Methods and Results: Herein, a systematic and comprehensive analysis of Hsp40s and Hsp70s in four cotton species of Gossypium arboretum, G. raimondii, G. hirsutum, and G. barbadense were performed. A total of 291 Hsp40s and 171 Hsp70s identified in four Gossypium species. Sequence analysis revealed that all Hsp40 proteins contained J domain that provides the binding sites to Hsp70. Protein-protein interaction prediction analysis displayed that GhHsp40-55 might interact with GhHsp70-2 and GhHsp70-13, suggesting their potential function as protein complex. Promoter cis-acting element analysis demonstrated that multiple cis-elements related to disease and stress response consists in GhHsp40 and GhHsp70 promoters. Further expression analysis showed that eight GhHsp40s (Hsp40-2,4,8,11,20,23,53,55) and seven GhHsp70s (Hsp70-2,3,6,8,13,19,22) were up-regulated after V. dahliae infection. In addition, five GhHsp40s (Hsp40-2,8,11,53,55) and four GhHsp70s (Hsp70-3,6,8,13) were up-regulated after salt treatment, six GhHsp40s (Hsp40-4,11,20,23) and three GhHsp70s (Hsp70-2,8,19) were up-regulated after drought treatment, four GhHsp40s (Hsp40-2,11,20,23) and four GhHsp70s (Hsp70-3,6,19,22) were up-regulated after temperature treatment, suggesting these Hsps have possible important function in the process of abiotic stress response.
Discussion: Our results lay a foundation for understanding the function of Hsp40 and Hsp70 in the resistance against V. dahliae and abiotic stress, and elucidating the regulatory mechanism of the protein complex, evolution and molecular mechanism under stress.
Introduction
Since plants do not have the ability to escape adverse environment, their growth, development and production are seriously affected by abiotic and biological stresses (Guo et al., 2021). In recent decades, biological and abiotic threats have reduced crop yield potential by about 70% (Katiyar-Agarwal et al., 2006; Nagaraju et al., 2020), causing serious economic losses to global crop production (Panzade et al., 2021).
Cotton (Gossypium spp.) is an important economic crop in the world, providing the most important natural fiber for the textile industry (Zhao et al., 2021). Studies show that Verticillium wilt (VW) is a typical fungal disease caused by V. dahliae Kleb, which seriously affects the yield and quality of cotton (Zhang et al., 2014; Zhao et al., 2021). VW pathogens usually exist in the soil in the form of microsclerotia, invading xylem and vascular tissue from the root of the host plant, and then spreading to the aboveground part, resulting in the leaves to lose green, necrosis or wilting, leaf and boll shedding until plant death (Zhou et al., 2017). In addition, with global climate change and environmental damage, cotton growth and development are also affected by various environmental pressures, such as drought, salt, alkali, low and high temperature abiotic stress (Parida et al., 2007). These biotic and abiotic stresses affect the growth and development process of cotton by affecting its physiological and metabolic reactions, causing irreversible damage or even death, and ultimately resulting in cotton yield reduction.
In order to ensure successful reproduction, plants have evolved a variety of defense mechanisms against various biotic and abiotic stresses, such as the ability to detect pathogens and induce rapid immune response through innate immune surveillance systems (Nejat & Mantri, 2017; Saijo et al., 2018). In addition, transcription factor (TF) is described as a group of proteins that participate in the regulation of specific gene expression by attaching to the promoter or enhance region of DNA. At the same time, they produce a group of proteins called stress proteins, which need to have a unique three-dimensional structure in order to function. Chaperones, also known as heat shock proteins (Hsps), protect the three-dimensional structure of a large number of proteins under different stress conditions. They are a very large family of proteins that are present in all living organisms. As a template, chaperone protein can fold correctly and form a functional three-dimensional structure immediately during and after synthesis, so that enough chaperone cells can be synthesized to better protect proteins and other structures and survive under stress conditions (Liberek et al., 2008). According to its molecular weight and function, Hsps can be divided into several families, including Hsp100, Hsp90, Hsp70 (also named DnaK in Escherichia coli), Hsp60, Hsp40 (also referred to as DnaJ) and low molecular weight Hsps (Guo et al., 2021). Heat shot protein 70s (Hsp70s) is a key component of the cell chaperone network (Qiu et al., 2006). The expression of Hsp70 family is either induced by various stresses or constitutive. For example, Hsp70-2 and Hsc70t have been shown to play a special role in sperm development (Dix et al., 1997; Eddy, 1999). In Arabidopsis thaliana, the mutant cpHsc70-1 showed a defective phenotype when the germinated seeds were subjected to heat stress (Su and Li, 2008). Heat stress induced the expression of MsHsp70, and transgenic lines of arabidopsis overexpressing MsHsp70 enhanced their tolerance to drought stress (Li et al., 2017). Under stress conditions, Hsp70 also plays an important role in wheat (Duan et al., 2011), cucumber (Li et al., 2014), pepper (Guo et al., 2014), rubber trees and other plants (Zhang et al., 2009). Hsp40, also known as DnaJ protein or J protein (Georgopoulos et al., 1980), is usually composed of a J-domain. As a co-partner of Hsp70, Hsp40 can strictly regulate ATP hydrolysis. Previous studies have shown that Hsp70 and Hsp40 are also involved in disease resistance of various plants, such as MP interacting protein 1 NtMPIP1 (Shimizu et al., 2009), GmHSP40.1 (Liu and Whitham, 2013), and rice DnaJ gene OsDjA6 (Zhong et al., 2018).
Hsps are evolutionarily conservative in many plants. In recent years, several studies have been conducted at the genome level to identify the function of Hsps genes in many plants (Krishna and Gloor, 2001; Zhang et al., 2014), therefore, the identification of Hsps genes are particularly important for further molecular function studies. With the development of sequencing technology, the genome sequencing of many plants has been completed, which greatly promotes the identification and phylogenetic analysis of plant homologous genes (Wang et al., 2016). So far, studies have reported 89 Hsp40s and 18 Hsp70s from Arabidopsis; 104 Hsp40s and 32 Hsp70s were identified in rice; 145 Hsp40s and 34 Hsp70s were identified in poplar; 91 Hsp40s and 27 Hsp70s were identified in eucalyptus (Zhang et al., 2014; Yer et al., 2018). However, the studies on the identification of Hsp40s and Hsp70s in cotton have not been reported yet. Based on the whole genome sequences of Gossypium arboretum, G. raimondii, G. hirsutum, and G. barbadense, we used bioinformatics methods to identify members of the Hsp40s and Hsp70s gene families in four cotton varieties. The number, chromosome location, phylogenetic evolution, expression pattern and interaction of Hsp40s and Hsp70s gene family members in cotton genome were analyzed at the genome level, providing reference for further research on the biological functions of Hsp40s and Hsp70s genes in cotton stress and verticillium wilt resistance.
Materials and methods
Genome databases
The genome sequence of Arabidopsis thaliana used in this study was downloaded from The Arabidopsis Information Resource (TAIR) database (Lamesch et al., 2012). The genome sequences of G. hirsutum genome (NDM8), G. raimondii (JGI_v2.1), G. arboreum (CRI_v3.0), G. barbadense (HAU v1) were downloaded from Cotton FGD (https://cottonfgd.org/) and CottonGEN (https://cottongen.org/).
Identification of Hsp40 and Hsp70 genes in cotton genome
In this research, we used “Heat Shock Protein Database Information Resource” (HSPIR) (https://pdslab.biochem.iisc.ernet.in/hspir/index.php) in order to identify the potential Hsp40s/Hsp70s in the G. hirsutum., G. raimondii, G. arboreum and G. barbadense genomes. Only the genes that were homologous with Hsp40s/Hsp70s (Evalue ≤ 1.0 × 10–5) and contain the Dna J domains were considered as GhHsp40s/Hsp70s, GaHsp40s/Hsp70s, GrHsp40s/Hsp70s and GbHsp40s/Hsp70s. We used the general feature format (GFF) file of the genomes to determine the relative position of Hsp40s/Hsp70s on chromosomes, and visualized the locations with the online software MG2C (Jiangtao et al., 2015). Furthermore, the gene structures of Hsp40s/Hsp70s were also analyzed according to the GFF files, and the “exon-intron” structure was shown by the Gene Structure Display Server (GSDS 2.08).
Sequence analysis of Hsp40s/Hsp70s
Protein motif analysis was performed using MEME9 with a maximum of eight motifs and using other default parameters. The physicochemical properties, including molecular weight (MW), isoelectric point (pI), instability index, and grand average of hydropathicity (GRAVY), were analyzed using the online software ExPASy ProtParam tool (https://web.expasy.org/cgi-bin/protparam/protparam) in GhHsp40s/Hsp70s, GaHsp40s/Hsp70s, GrHsp40s/Hsp70s, and GbHsp40s/Hsp70s, respectively.
Phylogenetic tree construction of Hsp40s/Hsp70s
The Hsp40s/Hsp70s protein sequences of G. hirsutum., G. raimondii, G. arboreum, G. barbadense and A. thaliana were aligned using ClustalW (https://www.genome.jp/tools-bin/clustalw), and the phylogenetic tree was constructed using the neighbor-joining algorithm with MEAG 7.0 (Kumar et al., 2016). Confidence values were obtained with bootstrapping with 1,000 replications.
Spatial and temporal expression analysis of GhHSP40s/Hsp70s genes
To illustrate the spatial and temporal expression patterns of GhHsp40s/Hsp70s, the RNA-seq data of abiotic stress, and Verticillium wilt expression data were downloaded from NCBI (accession NO. PRJNA248163, PRJNA490626, PRJNA680449) (Zhang et al., 2014; Hu et al., 2019). The expression levels of GhHsp40s/Hsp70s genes were visualized using the R language package Pheatmap (https://cran.r-project.org/web/packages/pheatmap/).
Statistical analysis
Mean values and standard errors were calculated with Microsoft Excel software. Student’s t-test was completed with the SPSS 23.0 program to assess the significance of any differences between the control and treated samples or between time points. The threshold for significance was set at p < .01.
Results
Genome-wide identifification of Hsp40 and Hsp70 gene family members in cotton species
To identify members of the Hsp40 and Hsp70 gene families in cotton, this study used The Arabidopsis Information Resource (TAIR, https://www.arabidopsis.org/) database (Lamesch et al., 2012) to downloaded AtHsp40s and AtHsp70s protein sequences which are used to retrieve the protein Sequences of G. hirsutum (NDM8), G. raimondii (JGI_v2.1), G. arboreum (cri_V0.3), G. hirsutum (NDM8), G. raimondii (JGI_v2.1), G. Arboreum (cri_V0.3). HMMER 3.0 software (on a Windows system) was utilized to search these cotton databases using DnaJ and DnaK domain as query domain. Finally, we identified 291 Hsp40s and 171 Hsp70s, including 93 GhHsp40s, 91 GbHsp40s, 45 GaHsp40s, and 62 GrHsp40s, as well as 77 GhHsp70s, 44 GbHsp70s, 25 GaHsp70s, and 25 GrHsp70s (Supplementary Table S1).
Physio-biochemical properties analysis
Then we analyzed the physical and chemical properties of the members of the Hsp40 and Hsp70 genes families in upland cotton, and found that the amino acid number of the protein encoded by the members of the two families has a large span. The longest Hsp40 was 1,404 amino acids (GhHsp40-42 and GhHsp40-59) and the shortest was 143.
Amino acids (GhHsp40-21). The longest Hsp70 was 886 amino acids (GhHsp70-11) and the shortest was 143 amino acids (GhHsp70-21). The molecular weight of GhHsp40s ranges from 15.61 kDa (GhHsp40-21) to 154.43 kDa (GhHsp40-42 and GhHsp40-59). The molecular weight of GhHsp70s ranges from 6.79 kDa (GhHsp70-21) to 98.69 kDa (GhHsp70-56). Based on isoelectric point (PI) analysis, GhHsp70s were acidic proteins with PI less than 7.0 (average 5.38). In contrast, GhHsp40s were predicted to encode proteins in excess of 7.0 (average 7.71) and to be alkaline. Hydrophilic analysis (GRAVY) found that all GhHsp40s and GhHsp70s were less than 0, indicating that GhHsp40s and GhHsp70s are hydrophilic proteins. According to the analysis of instability index, the mean instability index of GhHsp40s protein is 41.47, among which 53 are less than 40.0 and 41 are greater than 40.0. The instability index values of three GhHsp40s were greater than 60.0 (GhHsp40-4, GhHsp40-38, GhHsp40-61 and GhHsp40-80). The mean instability index of GhHsp70s protein was 36.62, of which 55 GhHsp70s were less than 40.0 and 23 GhHsp70s were greater than 40.0. Detailed physical and chemical properties are shown in Tables 1, 2.
Chromosomal location analysis of Hsp40s and Hsp70s in cotton species
According to the GFF files of G. hirsutum (NDM8), G. raimondii (JGI_v2.1), G. arboreum (CRI_v3.0), G. barbadense (HAU v1), the online software MG2C conducted a visual analysis of its location (Figure 1). The results show that 93 GhHsp40s are evenly distributed in 12 At_subgenomes and 12_Dt subgenomes (Figure 1A), of which 39 GhHsp40s are in the At_subgenome, and 12 are in the Chr06 chromosome (GhHsp40-1, GhHsp40-14, GhHsp40-16, GhHsp40-18, GhHsp40-20, GhHsp40-22, GhHsp40-23, GhHsp40-24, GhHsp40-52, GhHsp40-53, GhHsp40-65, GhHsp40-67). There were 54 GhHsp40s in the Dt subgenome, and more GhHsp40s (8 GhHsp40s) were distributed in the Chr06 chromosome. Ninety-one GbHsp40s were evenly distributed in 10 At_subgenomes and 12 Dt_subgenomes, and 1-5 GbHsp40s were distributed on each chromosome. The distribution of GaHsp40s gene on chromosome is similar to that of GhHsp40s in At_subgenome. At the same time, the distribution of GrHsp40s gene on chromosomes is also similar to that of GhHsp40s in At_subgenome, while on Chr06 chromosome, there is an additional Hsp40s-8 distributed on GrHsp40s, with less Hsp40-52, Hsp40-53, Hsp40-65, and Hsp40-67. This indicates that there is gene deletion or duplication in the genome of upland cotton.
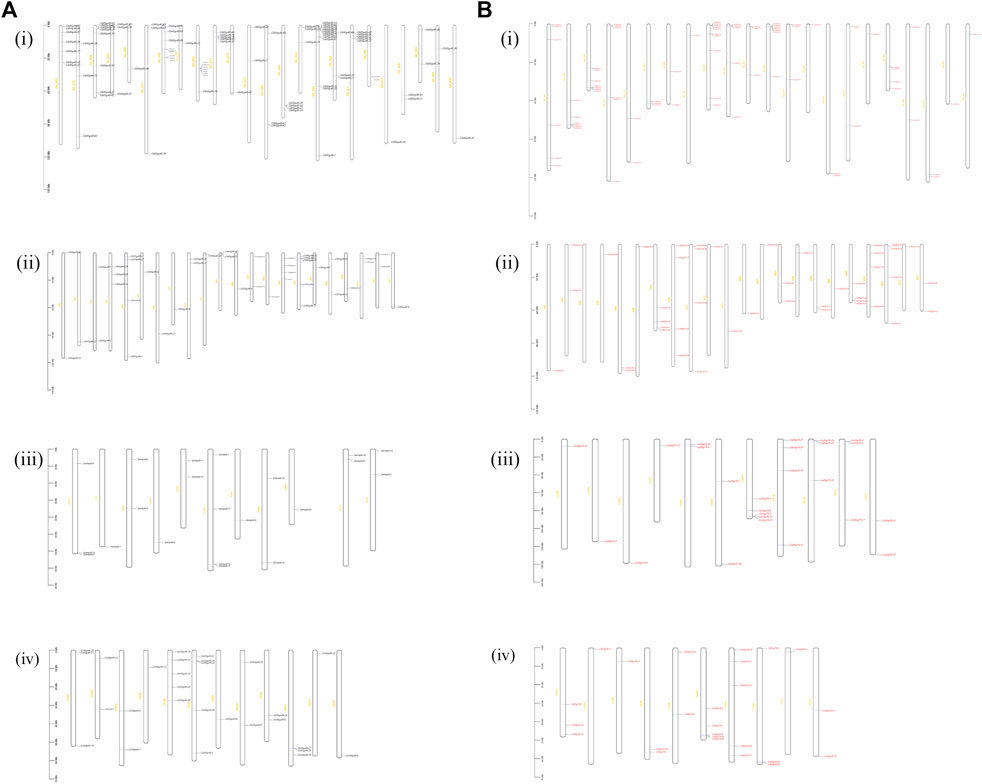
FIGURE 1. Chromosomal localization analysis of Hsp40s and Hsp70s gene family members in four cotton species. (A): (i) Hsp40s gene family members in G. hirsutum (Gh), (ii) Hsp40s gene family members in G. barbadense (Gb), (iii) Hsp40s gene family members in G. arboreum (Ga), (iv) Hsp40s gene family members in G. raimondii (Gr). (B): (i) Hsp70s gene family members in G. hirsutum (Gh), (ii) Hsp70s gene family members in G. barbadense (Gb), (iii) Hsp70s gene family members in G. arboreum (Ga), (iv) Hsp70s gene family members in G. raimondii (Gr).
Seventy-seven GhHsp70s were uniformly distributed in 11 At_subgenomes and 11 Dt_subgenomes (Figure 1B), among which 32 GhHsp70s were found in At_subgenomes, and most of the chromosomes had 2-3 GhHsp70s. The number of chromosomes distributed on Chr09, Chr10 and Chr11 was 6 GhHsp70s. There were 45 GhHsp70s in Dt_subgenome, and there were 10 GhHsp70s in Chr10 chromosome, which were GhHsp70-16, GhHsp70-17, GhHsp70-39, GhHsp70-56, GhHsp70-69, GhHsp70-70, GhHsp70-71, GhHsp70-72, GhHsp70-74, GhHsp70-75. 44 GbHsp70s were evenly distributed in 11 At_subgenomes and 11 Dt_subgenomes, with 21 and 23 GbHsp70s, respectively. The 25 GaHsp70s were similar to those of GhHsp70s in the Dt_subgenome. For example, Hsp70-4 and Hsp70-6 were found in both genomes at similar locations on Chr09. The distribution of 25 GrHsp70s was similar to that of GhHsp70s in the At_subgenome, for example, GrHsp70s-18 in the upper and lower ends of the Chr09 chromosome and GhHsp70s-18 in the upper end of the Chr09 chromosome in the At_subgenome, indicating an inversion in the chromosomes of these cotton species. In addition, it was also found that Hsps were conservatively distributed on Chr05, Chr06, Chr09, Chr10, Chr11 and Chr13 of four cotton species.
Phylogenetic analysis of Hsp40s and Hsp70s
In order to clarify the evolutionary relationship between Hsp40s and Hsp70s, we imported the full-length protein sequences of 291 Hsp40s, 171 Hsp70s, AtHsp40s and AtHsp70s into MEGA7.0 software for comparison. The phylogenetic tree was constructed using the adjacency method, and the Hsp40s/Hsp70 subgroups of G. hirsutum, G. raimondii, G. arboreum, G. barbadense and Arabidopsis thaliana were classified according to the number of branches in the phylogenetic tree (Figure 2). Phylogenetic analysis of Hsp40s family members (Figure 2A) revealed that two species specific Hsp40s branches appeared (At3G11451.1 and At4G39150.3), and all the remaining five species of Hsp40s could be classified into three subgroups. They were named as Hsp40s-I, II and III subgroups. The Hsp40s-I subgroup had the least number of Hsp40s, including 6 GhHsp40s, 3 GbHsp40s, 2 GaHsp40s, and 1 AtHsp40s, 12 Hsp40s in total. Hsp40s-II subgroup includes 33 GhHsp40s, 11 GbHsp40s, 5 GaHsp40s, 6 GrHsp40s, 9 AtHsp40s, and 64 Hsp40s members in total. The Hsp40s-III subgroup has the largest number of members, including 138 GhHsp40s, 29 GbHsp40s, 18 GrHsp40s, 15 GrHsp40s, and 19 AtHsp40s, totaling 213 Hsp40s.
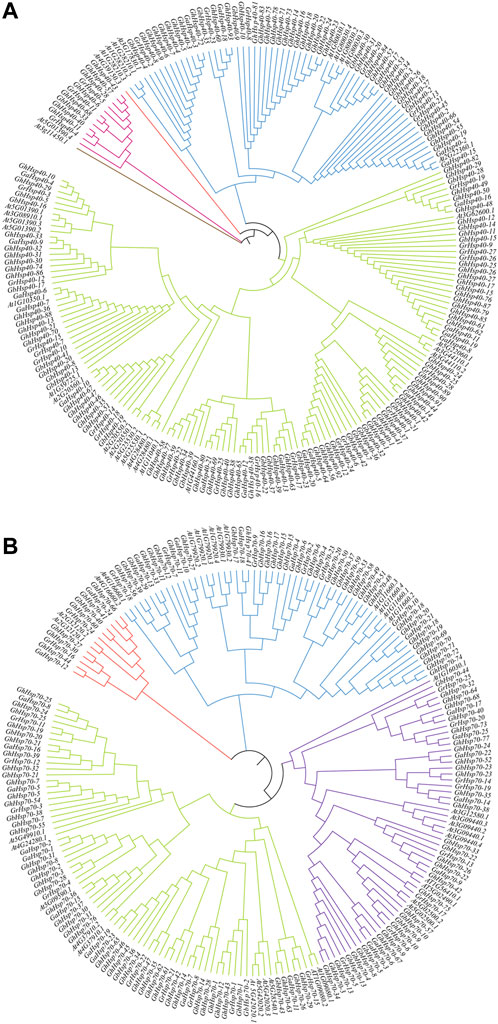
FIGURE 2. Phylogenetic tree of Hsp40s and Hsp70s gene family members from 5 species. (A): Hsp40s gene family members in 5 species. (B): Hsp70s gene family members in 5 species. The gene sequences were selected from G. hirsutum (Gh), G. arboreum (Ga), G. barbadense (Gb), G. raimondii (Gr), A. thaliana (At).
The phylogenetic analysis of members of the Hsp70s gene family shows that (Figure 2B), the Hsp70s gene family was different from the Hsp40s gene family, and there was no species specific Hsp70s branch. All Hsp70s of the five species could be grouped into four subgroups named Hsp70s-I, II, III, and IV. Similar to the Hsp40s gene family, the Hsp70s-I subgroup had the smallest number of Hsp70s members, with only 13 Hsp70s members, including 4 GhHsp70s, 3 GbHsp70s, 2 GaHsp70s, 2 GrHsp70s, and 2 AtHsp70s. There was no significant difference in the number of members of Hsp70s-II and III subgroups, and the distribution of the number of five species in the two subgroups was similar. There were 59 Hsp70s in the Hsp70-II subgroup, including 24 GhHsp40s, 11 GbHsp70s, 6 GaHsp70s, 6 GrHsp70s, and 12 AtHsp70s. There were 57 Hsp70s in the Hsp70-III subgroup, including 21 GhHsp70s, 11 GbHsp70s, 7 GaHsp70s, 8 GrHsp70s, and 10 AtHsp70s. Hsp70s-IV is the subgroup with the largest distribution of Hsp70s gene family members. There are 77 Hsp70s members, including 26 GhHsp70s, 19 GbHsp70s, 10 GaHsp70s, 9 GrHsp70s and 11 AtHsp70s.
The homologous genes of each species mainly contain duplicate genes. The phylogenetic analysis shows that there are specific Hsp40s and Hsp70s gene duplication events in four cotton species. In the Hsp40s gene family, we identified 8 pairs of homologous genes (Hsp40-1, 2, 3, 4, 11, 15, 26, 27). Fourteen pairs of homologous genes were identified in the Hsp70s gene family (Hsp70-1, 2, 5, 6, 10, 13, 14, 15, 16, 18, 21, 23, 24, 25).
Protein features of Hsp40s and Hsp70s
In order to identify the conserved domains of Hsp40s and Hsp70s, we submitted 291 Hsp40s and 171 Hsp70s protein sequences to the CDD tool of NCBI for prediction (Figure 3). Hsp40s protein contains eight conservative domains (Figure 3A): DnaJ, DnaJ_ C, TPR_ I, DnaJ_ C superfamily, DnaJ superfamily, MYB DNA-binding, DcrB superfamily, abhydrolase superfamily. The Hsp40s of the four cotton species all have conserved DnaJ and DnaJ_C domains, which are consistent with the Hsp40s of other species. In particular, GaHsp40s is different from the other three cotton species in that it has only DnaJ and DnaJ_C domains. These results indicate that Hsp40s has increased and lost some conserved domains during evolution. Notably, only GaHsp40s contain two domains (DnaJ and DnaJ_C domains) (Figure 3A iii), suggesting that they are the most conserved. GhHsp40s and GrHsp40s have DnaJ_ C superfamily, DnaJ superfamily, MYB_ DNA-binding, and MYB_ DNA-binding domains. While GrHsp40s has special DcrB superfamily and abhydrolase superfamily domains, which indicates that they are located in G Some special functions may be obtained in G. hirsutum, G. raimondii, G. barbadense. The Hsp70s proteins in four cotton species all contain only two conservative domains (Figure 3B): HSP70, HSP70 superfamily. These results indicate that these two domains of Hsp70s protein are very conservative during evolution. In addition, we used MEME online software to analyze the motif characteristics of Hsp40s and Hsp70s, and identified 20 conserved motifs in Hsp40s and Hsp70s gene families (Figure 4).
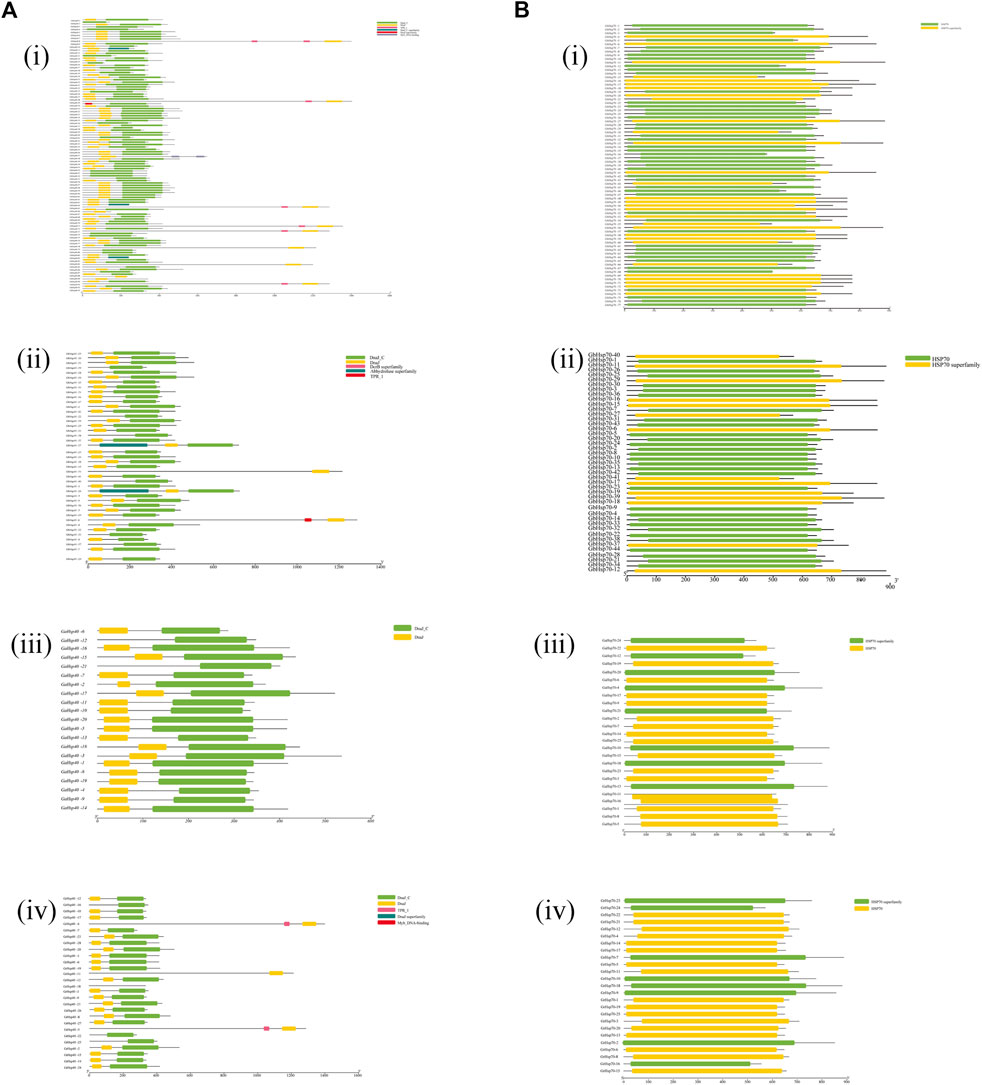
FIGURE 3. Protein features of Hsp40s and Hsp70s in four cotton species. (A): (i) Hsp40s protein in G. hirsutum (Gh), (ii) Hsp40s protein in G. barbadense (Gb), (iii) Hsp40s protein in G. arboreum (Ga), (iv) Hsp40s protein in G. raimondii (Gr). (B): (i) Hsp70s protein in G. hirsutum (Gh), (ii) Hsp70s protein in G. barbadense (Gb), (iii) Hsp70s protein in G. arboreum (Ga), (iv) Hsp70s protein in G. raimondii (Gr).
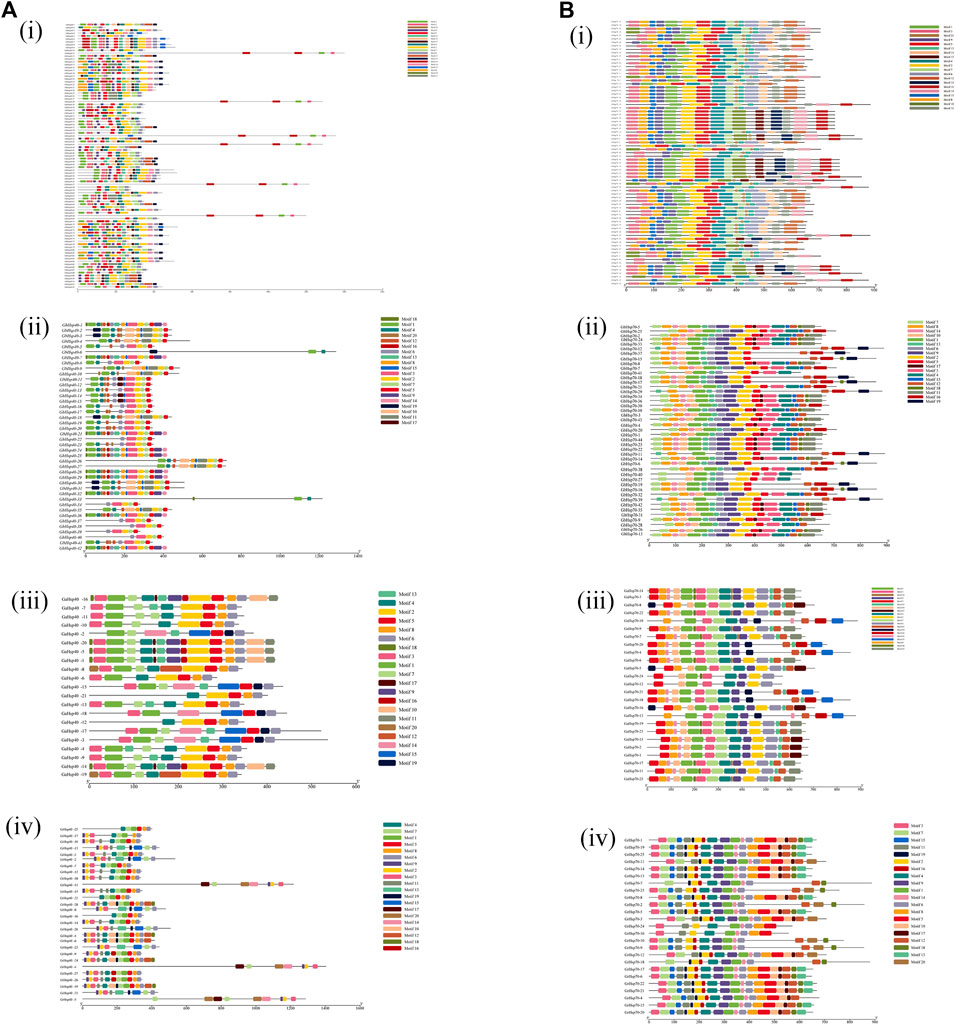
FIGURE 4. The motifs of Hsp40s and Hsp70s sequences from our cotton species. (A): (i) Hsp40s protein in G. hirsutum (Gh), (ii) Hsp40s protein in G. barbadense (Gb), (iii) Hsp40s protein in G. arboreum (Ga), (iv) Hsp40s protein in G. raimondii (Gr). (B): (i) Hsp70s protein in G. hirsutum (Gh), (ii) Hsp70s protein in G. barbadense (Gb), (iii) Hsp70s protein in G. arboreum (Ga), (iv) Hsp70s protein in G. raimondii (Gr).
Collinearity analysis Hsp40s and Hsp70s
Tandem and fragment DNA replication are the main driving forces driving the expansion of gene families and the evolution of the entire genome. In order to explore the evolutionary relationship between Hsp40s and Hsp70s of diploid and tetraploid cotton, we analyzed the collinearity between diploid and tetraploid cotton. The results showed that six GaHsp40s were collinear with six GhHsp40s genes. There was a collinear relationship between GhHsp40s of 16 G. hirsutum and GbHsp40s of 12 G. raimondii (Figure 5A). Six GaHsp70s genes of G. arboreum were collinear with six GhHsp70s of G. hirsutum. Fourteen GhHsp70s genes of G. hirsutum were collinear with eight GbHsp70s of G. raimondii (Figure 5B). Collinearity analysis showed that each Hsp40 or Hsp70 from diploid cotton (G. arboreum or G. raimondii) corresponded to at least one homologous gene in the At_and Dt_subgenomes of tetraploid cotton species (G. hirsutum or G. marimondii).
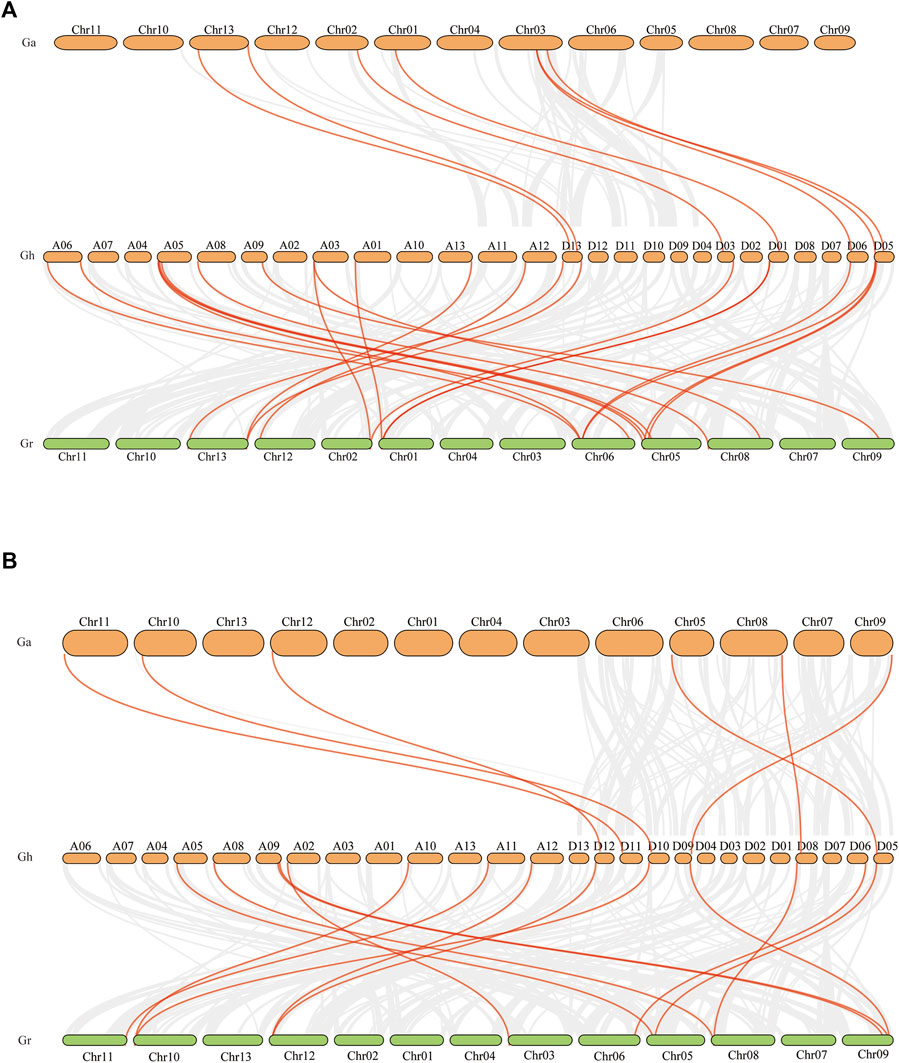
FIGURE 5. Collinearity analysis of Hsp40s and Hsp70s genes. (A): Collinearity analysis of Hsp40s genes in G. hirsutum, G. arboreum, and G. raimondii; (B): Collinearity analysis of Hsp70s genes in G. barbadense, G. arboreum, and G. raimondii.
Cis-promoter element analysis of Hsp40s and Hsp70s
We further analyzed the cis-regulatory elements in the upstream region of Hsp40s and Hsp70s. The analysis results showed that there were 13 different cis-acting elements in Hsp40s of the four cotton seeds (Figure 6A), which were divided into two types according to their different functions, namely, the assumed abiotic stress response elements and the hormone response elements. In GhHsp40s promoter, hormone-related elements occupy the largest proportion. Examples include gibberellin-responsive element, salicylic acid responsive element and abscisic acid responsive element. The second is low temperature responsive element, anaerobic induction responsive element and wound-responsive element. Except that the promoter of GhHsp40s contains one hormone response element, the promoters of other GhHsp40s all have at least two hormone response elements. Among the promoters of GbHsp40s, defense and stress responsive elements account for the largest proportion, followed by gibberellin responsive element and MeJA responsive element. Among GaHsp40s promoters, low temperature responsive element, anaerobic induction responsive element and wow responsive element account for the most. Among the promoters of GrHsp40s, the ratio of defense and stress responsive element, wound responsive element and gibberellin responsive element is the highest.
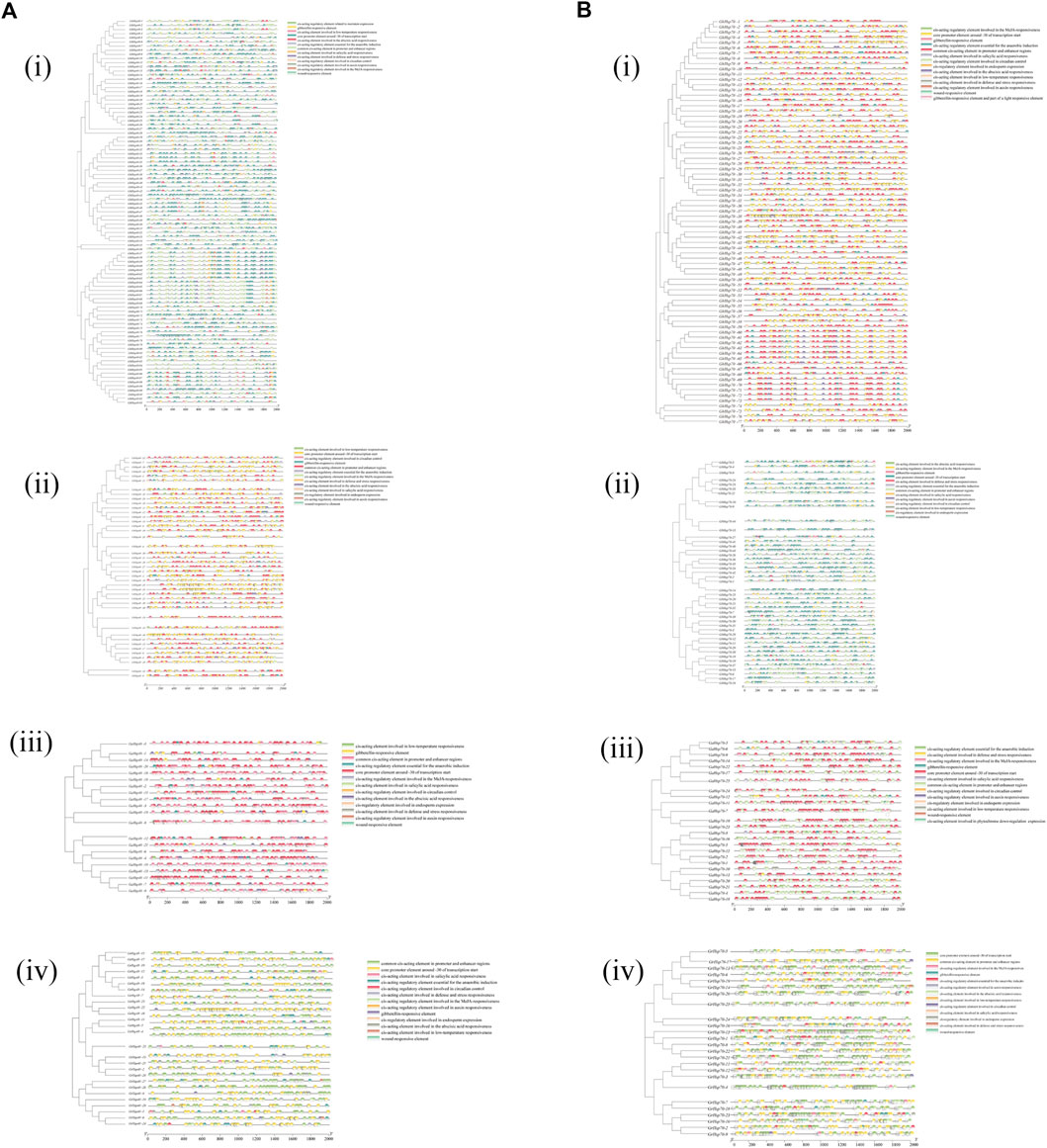
FIGURE 6. The distribution of cis-acting elements in upstream sequence of Hsp40s and Hsp70s in four cotton species. (A): (i) Hsp40s gene family members in G. hirsutum (Gh), (ii) Hsp40s gene family members in G. barbadense (Gb), (iii) Hsp40s gene family members in G. arboreum (Ga), (iv) Hsp40s gene family members in G. raimondii (Gr). (B): (i) Hsp70s gene family members in G. hirsutum (Gh), (ii) Hsp70s gene family members in G. barbadense (Gb), (iii) Hsp70s gene family members in G. arboreum (Ga), (iv) Hsp70s gene family members in G. raimondii (Gr).
We identified 15 distinct cis-acting elements in GhHsp70s promoters of four cotton species (Figure 6B), which were classified into three categories based on their roles, including photoresponsive, hormonal, and abiotic stress response elements. In the promoter of GhHsp70s, salicylic acid responsive element, anaerobic induction responsive element and wound-responsive element. Among the promoters of GbHsp70s, abscisic acid responsive element and anaerobic induction responsive element make up the largest proportion. Second is the MeJA responsive element. Among GaHsp70s promoters, we found that the phytochrome downregulation expression element, which is not found in the Hsp70s promoter, and low temperature responsive element, defense and stress responsive element and anaerobic induction responsive element, which are non-biological stress related elements, account for the largest proportion, followed by the hormone related element gibberellin responsive element. For GrHsp70s promoter, anaerobic induction responsive element occupies the largest proportion, followed by hormone-related element gibberellin-responsive element.
Expression analysis of GhHsp40s and GhHsp70s genes under biological and abiotic stress conditions
Upland cotton is one of the important economic crops in the world. At present, it dominates the world cotton trade and natural cotton linter (Li et al., 2015; Hu et al., 2019). In order to further understand the potential function of GhHsp40s and GhHsp70s involved in the response to V. dahliae and abiotic stress (high temperature, low temperature, salt, and drought), we analyzed the profile of all members of GhHsp40s and GhHsp70s gene families. We found that, compared to the control group, GhHsp40-2, GhHsp40-4, GhHsp40-8, GhHsp40-11, GhHsp40-20, GhHsp40-23, GhHsp40-25, GhHsp40-53, GhHsp40-55 were all expressed in V. dahliae infection 6 h after dahliae infection, it was significantly higher (Figure 7; Supplementary Figure S5). The expressions of GhHsp70-2, GhHsp70-3, GhHsp70-6, GhHsp70-8, GhHsp70-13, GhHsp70-19 and GhHsp70-22 were significantly increased 24 h after inoculation with V. dahliae infection (Figure 8; Supplementary Figure S5).
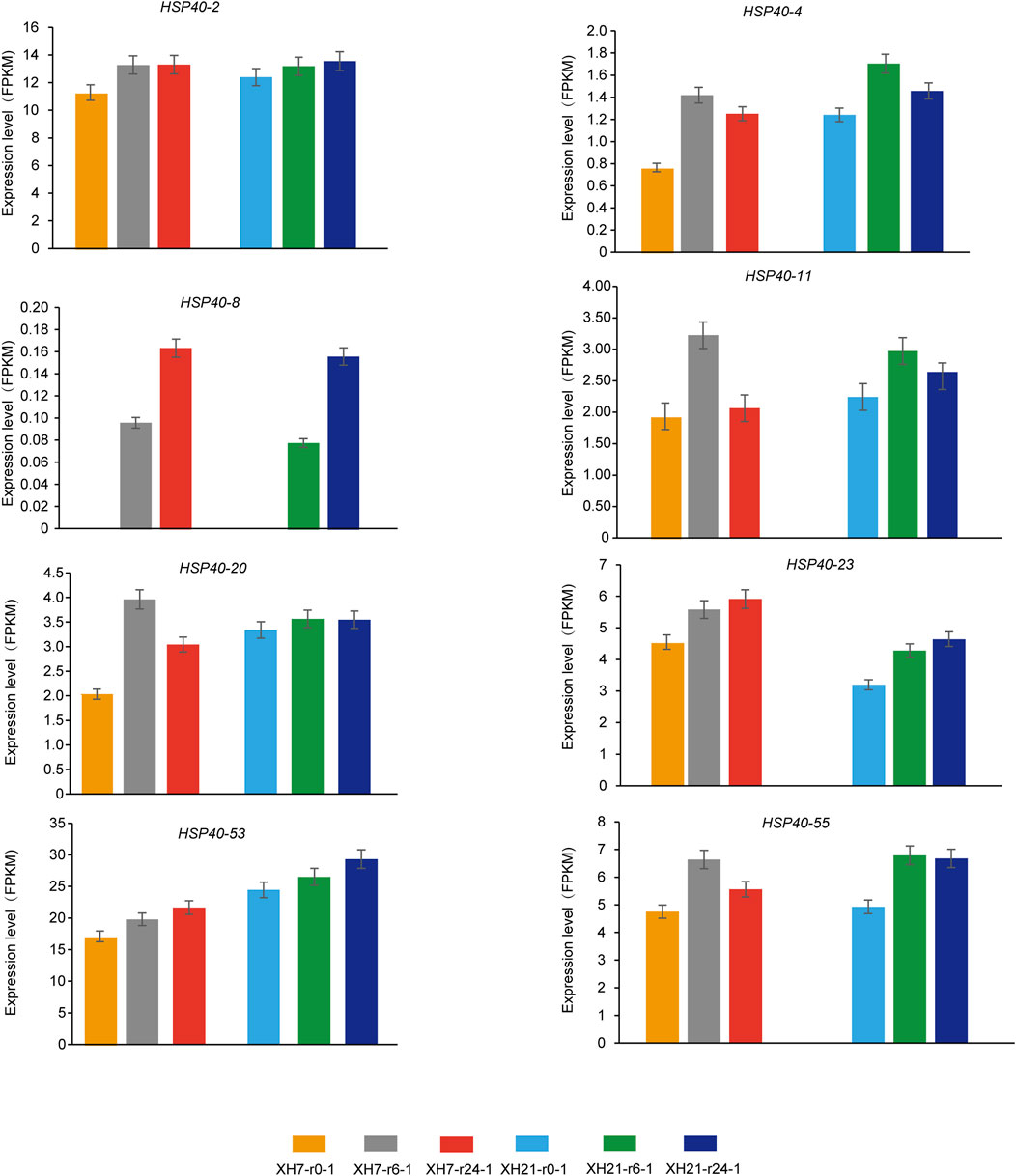
FIGURE 7. Temporal and spatial expression profiles of Hsp40s gene family members after inoculation with V. dahliae infection.
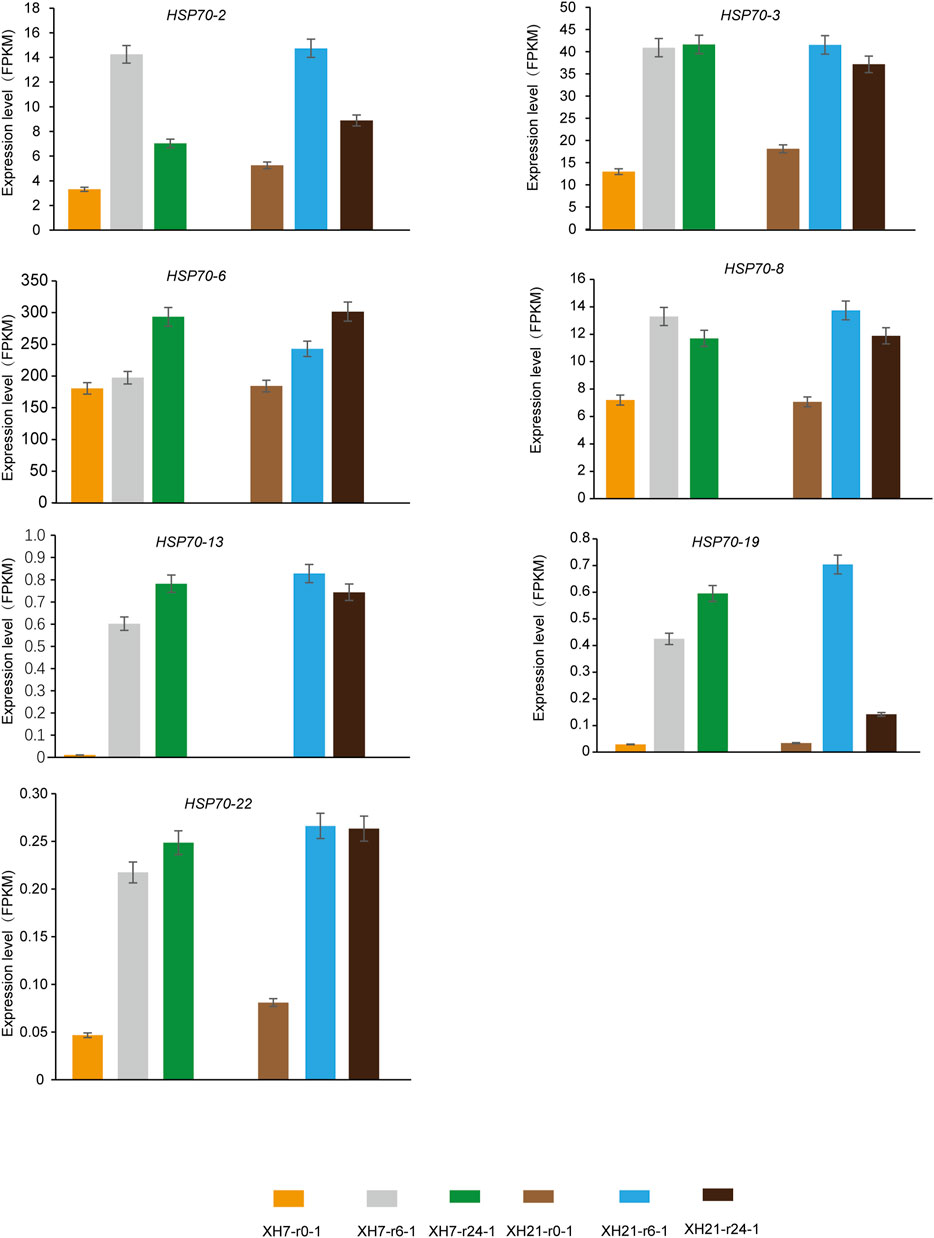
FIGURE 8. Temporal and spatial expression profiles of Hsp70s gene family members after inoculation with V. dahliae infection.
Compared with the control group, GhHsp40-4, GhHsp40-20, GhHsp40-23, GhHsp40-53, GhHsp70-8, GhHsp70-13 and GhHsp70-19 were upregulated under high temperature stress, while GhHsp40-4 was downregulated under high temperature stress (Supplementary Figure S1). Under the condition of low temperature stress, the expression of GhHsp40-8 and GhHsp70-19 increased significantly after 1 h and 2 h of treatment (Supplementary Figure S2). Under salt stress, the expressions of GhHsp40-11 and GhHsp40-2 were significantly increased after 3 h of treatment, while the expressions of GhHsp70-19 were significantly increased after 1 h of treatment (Supplementary Figure S3). Under PEG stress, the expressions of GhHsp40-2 and GhHsp70-13 increased significantly after 3 h treatment. The expressions of GhHsp40-11, GhHsp70-2 and GhHsp70-19 were significantly increased after treatment for 1 h. The expression of GhHsp40-20 was significantly increased after 6 h of treatment (Supplementary Figure S4).
In addition, we also found that the expression of GhHsp40-2, GhHsp40-4, GhHsp40-8, GhHsp40-11, GhHsp40-20, GhHsp70-13, GhHsp70-19 increased significantly with treatment time under verticillium wilt resistance and various stress treatments. Phylogenetic tree analysis showed (Figure 2) that these Hsp40s were mainly located in Hsp40-I and Hsp40-II subgroups, while GhHsp70-13 and GhHsp70-19 were located in Hsp70-III and Hsp70-IV subgroups, respectively. These subgroups may regulate verticillium wilt resistance and abiotic stress response of upland cotton.
Discussion
Heat shock proteins (e.g., Hsp40 (DnaJ), Hsp60, Hsp70, Hsp90, and Hsp101) are one of the most common chaperones and participate in a variety of biological processes (Guo et al., 2021). In the research on the function of HSPs in animals, researchers found that they can also directly stimulate the cells of the innate immune system, which indicates that they are the activators of the innate immune system of animals (Cui et al., 2011). For example, non-lethal heat shock induces the synthesis of Hsp70, and promotes Penaeus vannamei’s tolerance to heat, ammonia and metal induced pressure (Sung et al., 2018). So far, researchers have identified Hsp40 and Hsp70 of several plants, such as barley (Landi et al., 2019) and Brachypodium distachyon (L.) Beauv. (Wen et al., 2017), A. thaliana (Li et al., 2017) and California poplar (Yer et al., 2018). Although the functional studies on Hsp40 and Hsp70 in plants are limited, these proteins are believed to have multiple roles in plants (Guo et al., 2021) and play similar functions as other eukaryotes.
The Hsp40 protein plays a key role in assisting protein folding by activating the ATPase domain of Hsp70. Despite their importance, the Hsp40s and Hsp70s of cotton have not been reported. In this study, we identified 291 Hsp40s and 171 Hsp70s in the genomes of four cotton species. The Hsp40s identified all contain the DNA-J domain to stimulate the ATPase activity of Hsp70, which is essential for the maintenance of Hsp40 function, suggesting that all of these Hsp40s are conserved and can act as copartners of Hsp70s. Sequence analysis showed that there were differences between Hsp40s and Hsp70s in PI and domain combinations. The average PI value of Hsp40s protein is 7.70, while the average PI value of Hsp70 protein is 5.38. One is acidic protein and the other is basic protein, which indicates that acidic protein and basic protein have different positions and functions in cells. The instability index indicates the in silico stability of protein (Gonzalez-Diaz et al., 2007), it was found that 41 out of 94 GhHsp40s were stable proteins, and the instability coefficient of GhHsp40-4, GhHsp40-38, GhHsp40-61 and GhHsp40-80 were more than 60, indicating that they were easy to degrade. The instability coefficient of most GhHsp70s proteins was less than 40, indicating that GhHsp70s was stable compared with GhHsp40s.
Gene duplication events of entire genomes, chromosomal segments, and individual genes play a role in the expansion of gene family members (Cannon et al., 2004; Maere et al., 2005). These events also contribute to the evolution of novel gene functions and potentially play an important role enhancing plant environmental adaptability (Huang et al., 2015). In order to understand the phylogenetic relationships between Hsp40s and Hsp70s, and to identify homology relationships within and among four cotton species, we constructed a phylogenetic tree using amino acid sequences of Hsp40s and Hsp70s. According to the phylogenetic tree, the sequence relationship of Hsp40s and Hsp70s can be divided into three groups and four groups respectively. At the same time, we have identified eight pairs of homologous genes in the Hsp40s gene family of four cotton varieties, and 14 pairs of homologous genes in the Hsp70s gene family. This suggests that the Hsp40s and Hsp70s gene families are species-specific in all four cotton species (Liu et al., 2018).
Cis-regulatory elements of promoter region play an important role in the functional differentiation of Hsp40s and Hsp70s genes. Analysis of cis-regulatory elements shows three types of cis-regulatory elements, including hormone responsive elements, stress responsive elements and light responsive elements. Among them, hormone response elements accounted for a large number, such as gibberellin-responsive element, auxin responsive element and abscisic acid responsive element. Plant hormones are important regulators of plant growth and development. Abscisic acid (ABA) regulates the formation of secondary cell wall and lignin deposition in Arabidopsis thaliana by phosphorylating NAC Secondary-wall Thicking protein factor 1 (NST1) (Liu et al., 2021). Auxin and gibberellin are promoters of cotton fiber cell elongation (Singh et al., 2009; Xiao et al., 2010). Salicylic acid responsive element and MeJA responsive element, which promote the synthesis and integrity of cell wall through salicylic acid and jasmonic acid metabolism, participate in plant pathogen defense response (Takahashi et al., 2004). Low temperature responsive element, anaerobic induction responsive element and wound-responsive element indicate that Hsp40s and Hsp70s genes can control environmental conditions. Hsp40s and Hsp70s may play important roles in different abiotic stress responses due to the existence of multiple cis-responsive elements. Therefore, this study provides a theoretical basis for further study of its actual mechanism of action.
There are 7 different domain combinations in the identified Hsp40s. The combination of domains leads to the diversity of cotton Hsp40s functions. The DnaJ domain is a specific feature that defines a protein as Hsp40 or Hsp40-like protein. In addition to the conserved domain, Hsp40 is bound to other domains, which may determine the functional diversity of Hsp40s. For example, tetratricopeptide repeat (TPR) domains, which mediate protein-protein interactions (Dermouche et al., 2021), Studies have shown that the C-terminal of Hsp90 dimer forms a functional receptor site for a co-partner carrying a tetratricopeptide repeat (TPR) domain, and they bind in a mutually exclusive or competitive manner. The biology of the glucocorticoid receptor (Hsp90•GR) complex is more potent, and it is a major player in the GR transport process (Mazaira et al., 2021). Most MYB proteins act as transcription factors and have been shown to be involved in regulating various cellular processes, including cell cycle and morphogenesis, biological and abiotic stress responses (Roy, 2016). In addition to TPR domains, we identified Myb DNA-binding domain in Hsp40 protein, suggesting that Hsp40 protein may bind to MYB transcription factors and be involved in mediating cell development, biological and abiotic stress responses. Compared with Hsp40s, there were only two types of proteins in Hsp70s, indicating that Hsp70 had more conservative functions.
The diversity of domain combinations resulted in the functional diversity of Hsp40s and the conserved function of Hsp70s. By analyzing the transcriptome of Hsp40s and Hsp70s under low temperature, high temperature, salt and PEG stress, it was found that Hsp40-I, Hsp40-II, Hsp70-III and Hsp70-IV subfamily members may respond to verticillium wilt resistance and abiotic stress. Such as, GhHsp40-2, GhHsp40-4, GhHsp40-8, GhHsp40-11, GhHsp40-20, GhHsp70-13 and GhHsp70-19 showed significantly increased expression levels with the increase of treatment time under verticillium wilt resistance and each stress treatment condition. They play a positive regulatory role in cotton response to verticillium wilt resistance and abiotic stress. This provided a theoretical basis for further study of gene family in resistance to abiotic stress and verticillium wilt resistance.
Conclusion
In this study, 291 Hsp40s and 171 Hsp70s gene family members were identified in cotton by bioinformatics. The analysis of their gene distribution and protein structure shows that these two Hsp families have complex evolutionary history. Promoter analysis showed that Hsp40s and Hsp70s were involved in abiotic stress tolerance and verticillium wilt resistance, which was verified by the expression profile analysis of high temperature, low temperature, PEG, salt stress and verticillium wilt resistance. Therefore, this study shows that GhHsp40-2, GhHsp40-4, GhHsp40-8, GhHsp40-11, GhHsp40-20, GhHsp70-13, GhHsp70-19 are potential candidate genes for further functional analysis in cotton variety improvement research. This study provided a good theoretical basis for further study of the biological functions of Hsp40s and Hsp70s gene families in cotton.
Data availability statement
The datasets presented in this study can be found in online repositories. The names of the repository/repositories and accession number(s) can be found in the article/Supplementary Material.
Author contributions
XnZ, LS, RT and YD contributed to conception and design of the study. XnZ, LS, RT, and YD performed and data analyses, XnZ and LS performed the statistical analysis. XnZ and LS wrote the first draft of the manuscript, FW, RL, and QX wrote sections of the manuscript. All authors contributed to manuscript revision, read and approved the submitted version.
Funding
This work is supported by the National Natural Science Foundation of China (32070549, 32270578, and 32200444), China Postdoctoral Acience Foundation (2022M712005), Natural Science Basic Research Plan in the Shaanxi Province Basic Research Plan in the Anhui Province of China (2022JQ-197), Fundamental Research Funds for the Central University (GK202002005) and State Key Laboratory of Cotton Biology Open Fund (CB2022A01, CB2021A05, and CB2021A21). Project for Postdoctoral and High-level Flexible Talents of Xinjiang Uygur Autonomous Region (Grant No. RSSQ00066509), Major Science and Technology Program of Changji Hui Autonomous Prefecture (Grant No. 2021Z01-01), Central Leading Local Science and Technology Development Fund Project of Xinjiang Uygur Autonomous Region (ZYYD2023C06) and Project for Developing Elite Varieties of Agriculture in Henan Province (2022010301).
Conflict of interest
The authors declare that the research was conducted in the absence of any commercial or financial relationships that could be construed as a potential conflict of interest.
Publisher’s note
All claims expressed in this article are solely those of the authors and do not necessarily represent those of their affiliated organizations, or those of the publisher, the editors and the reviewers. Any product that may be evaluated in this article, or claim that may be made by its manufacturer, is not guaranteed or endorsed by the publisher.
Supplementary material
The Supplementary Material for this article can be found online at: https://www.frontiersin.org/articles/10.3389/fgene.2023.1120861/full#supplementary-material
Abbreviation
Hsp, heat shock proteins.
References
Cannon, S. B., Mitra, A., Baumgarten, A., Young, N. D., and May, G. (2004). The roles of segmental and tandem gene duplication in the evolution of large gene families in Arabidopsis thaliana. BMC plant biology 4 (1), 10–21. doi:10.1186/1471-2229-4-10
Cui, Y., Zhang, X., Gong, Y., Niu, S., Yin, N., Yao, R., et al. (2011). Immunization with DnaJ (hsp40) could elicit protection against nasopharyngeal colonization and invasive infection caused by different strains of Streptococcus pneumoniae. Vaccine 29 (9), 1736–1744. doi:10.1016/j.vaccine.2010.12.126
Dermouche, S., Chagot, M. E., Manival, X., and Quinternet, M. (2021). Optimizing the first TPR domain of the human SPAG1 protein provides insight into the HSP70 and HSP90 binding properties. Biochemistry 60 (30), 2349–2363. doi:10.1021/acs.biochem.1c00052
Dix, D. J., Allen, J. W., Collins, B. W., Poorman-Allen, P., Mori, C., Blizard, D. R., et al. (1997). HSP70-2 is required for desynapsis of synaptonemal complexes during meiotic prophase in juvenile and adult mouse spermatocytes. Development 124 (22), 4595–4603. doi:10.1242/dev.124.22.4595
Duan, Y. H., Guo, J., Ding, K., Wang, S. J., Zhang, H., Dai, X. W., et al. (2011). Characterization of a wheat HSP70 gene and its expression in response to stripe rust infection and abiotic stresses. Mol.ecular Biology Reports 38 (1), 301–307. doi:10.1007/s11033-010-0108-0
Eddy, E. M. (1999). Role of heat shock protein HSP70-2 in spermatogenesis. Reviews of Reproduction 4, 23–30. doi:10.1530/ror.0.0040023
Georgopoulos, C. P., Lundquist-Heil, A., Yochem, J., and Feiss, M. (1980). Identification of the E. coli dnaJ gene product. Molecular and eneral Genetics MGG 178 (3), 583–588. doi:10.1007/BF00337864
González-Díaz, H., Pérez-castillo, Y., Podda, G., and Uriarte, E. (2007). Computational chemistry comparison of stable/nonstable protein mutants classification models based on 3D and topological indices. Journal of Computational Chemistry 28 (12), 1990–1995. doi:10.1002/jcc.20700
Guo, H., Zhang, H., Wang, G., Wang, C., Wang, Y., Liu, X., et al. (2021). Identification and expression analysis of heat-shock proteins in wheat infected with powdery mildew and stripe rust. Plant Genome 14 (2), e20092. doi:10.1002/tpg2.20092
Guo, M., Zhai, Y. F., Lu, J. P., Chai, L., Chai, W. G., Gong, Z. H., et al. (2014). Characterization of CaHsp70-1, a pepper heat-shock protein gene in response to heat stress and some regulation exogenous substances in Capsicum annuum L. International Journal of Molecular Sciences 15 (11), 19741–19759. doi:10.3390/ijms151119741
Hu, Y., Chen, J., Fang, L., Zhang, Z., Ma, W., Niu, Y., et al. (2019). Gossypium barbadense and Gossypium hirsutum genomes provide insights into the origin and evolution of allotetraploid cotton. Nature Genetics 51 (4), 739–748. doi:10.1038/s41588-019-0371-5
Huang, Y., Li, M. Y., Wang, F., Xu, Z. S., Huang, W., Wang, G. L., et al. (2015). Heat shock factors in carrot: Genome-wide identification, classification, and expression profiles response to abiotic stress. Molecular Biology Reports 42 (5), 893–905. doi:10.1007/s11033-014-3826-x
Jiangtao, C., Yingzhen, K., Qian, W., Yuhe, S., Daping, G., Jing, L. V., et al. (2015). MapGene2Chrom, a tool to draw gene physical map based on Perl and SVG languages. Yi chuan= Hereditas 37 (1), 91–97. doi:10.16288/j.yczz.2015.01.013
Katiyar-Agarwal, S., Morgan, R., Dahlbeck, D., Borsani, O., Villegas, A., Zhu, J. K., et al. (2006). A pathogen-inducible endogenous siRNA in plant immunity. Proceedings of the National Academy of Sciences 103 (47), 18002–18007. doi:10.1073/pnas.0608258103
Krishna, P., and Gloor, G. (2001). The Hsp90 family of proteins in Arabidopsis thaliana. Cell Stress and Chaperones 6 (3), 238–246. doi:10.1379/1466-1268(2001)006<0238:thfopi>2.0.co;2
Kumar, S., Stecher, G., and Tamura, K. (2016). MEGA7: Molecular evolutionary genetics analysis version 7.0 for bigger datasets. Molecular Biology and Evolution 33 (7), 1870–1874. doi:10.1093/molbev/msw054
Lamesch, P., Berardini, T. Z., Li, D., Swarbreck, D., Wilks, C., Sasidharan, R., et al. (2012). The arabidopsis information Resource (TAIR): Improved gene annotation and new tools. Nucleic Acids Research 40 (D1), D1202–D1210. doi:10.1093/nar/gkr1090
Landi, S., Capasso, G., Ben Azaiez, F. E., Jallouli, S., Ayadi, S., Trifa, Y., et al. (2019). Different roles of heat shock proteins (70 kDa) during abiotic stresses in barley (Hordeum vulgare) genotypes. Plants 8 (8), 248. doi:10.3390/plants8080248
Li, F., Fan, G., Lu, C., Xiao, G., Zou, C., Kohel, R. J., et al. (2015). Genome sequence of cultivated Upland cotton (Gossypium hirsutum TM-1) provides insights into genome evolution. Nature biotechnology 33 (5), 524–530. doi:10.1038/nbt.3208
Li, H. A. O., Liu, S. S., Yi, C. Y., Wang, F., Zhou, J. I. E., Xia, X. J., et al. (2014). Hydrogen peroxide mediates abscisic acid-induced HSP 70 accumulation and heat tolerance in grafted cucumber plants. Plant, Cell & Environment 37 (12), 2768–2780. doi:10.1111/pce.12360
Li, Z., Long, R., Zhang, T., Wang, Z., Zhang, F., Yang, Q., et al. (2017). Molecular cloning and functional analysis of the drought tolerance gene MsHSP70 from alfalfa (Medicago sativa L.). Journal of Plant Research 130 (2), 387–396. doi:10.1007/s10265-017-0905-9
Liberek, K., Lewandowska, A., and Ziętkiewicz, S. (2008). Chaperones in control of protein disaggregation. The European Molecular Biology Organization Journal 27 (2), 328–335. doi:10.1038/sj.emboj.7601970
Liu, C., Yu, H., Rao, X., Li, L., and Dixon, R. A. (2021). Abscisic acid regulates secondary cell-wall formation and lignin deposition in Arabidopsis thaliana through phosphorylation of NST1. Proceedings of the National Academy of Sciences 118 (5), e2010911118. doi:10.1073/pnas.2010911118
Liu, J., Pang, X., Cheng, Y., Yin, Y., Zhang, Q., Su, W., et al. (2018). The Hsp70 gene family in Solanum tuberosum: Genome-wide identification, phylogeny, and expression patterns. Scientific Reports 8 (1), 16628–16711. doi:10.1038/s41598-018-34878-7
Liu, J. Z., and Whitham, S. A. (2013). Overexpression of a soybean nuclear localized type–III DnaJ domain-containing HSP40 reveals its roles in cell death and disease resistance. The Plant Journal 74 (1), 110–121. doi:10.1111/tpj.12108
Maere, S., De Bodt, S., Raes, J., Casneuf, T., Van Montagu, M., Kuiper, M., et al. (2005). Modeling gene and genome duplications in eukaryotes. Proceedings of the National Academy of Sciences 102 (15), 5454–5459. doi:10.1073/pnas.0501102102
Mazaira, G. I., Echeverría, P. C., Ciucci, S. M., Monte, M., Gallo, L. I., Erlejman, A. G., et al. (2021). Differential regulation of the glucocorticoid receptor nucleocytoplasmic shuttling by TPR-domain proteins. Biochimica et Biophysica Acta (BBA)-Molecular Cell Research 1868 (6), 119000. doi:10.1016/j.bbamcr.2021.119000
Nagaraju, M., Reddy, P. S., Kumar, S. A., Kumar, A., Rajasheker, G., Rao, D. M., et al. (2020). Genome-wide identification and transcriptional profiling of small heat shock protein gene family under diverse abiotic stress conditions in Sorghum bicolor (L.). International Journal of Biological Macromolecules 142, 822–834. doi:10.1016/j.ijbiomac.2019.10.023
Nejat, N., and Mantri, N. (2017). Plant immune system: Crosstalk between responses to biotic and abiotic stresses the missing link in understanding plant defence. Current Issues in Molecular Biology 23 (1), 1–16. doi:10.21775/cimb.023.001
Panzade, K. P., Kale, S. S., Chavan, N. R., and Hatzade, B. (2021). Genome-wide analysis of Hsp70 and Hsp100 gene families in Ziziphus jujuba. Cell Stress and Chaperones 26 (2), 341–353. doi:10.1007/s12192-020-01179-w
Parida, A. K., Dagaonkar, V. S., Phalak, M. S., Umalkar, G. V., and Aurangabadkar, L. P. (2007). Alterations in photosynthetic pigments, protein and osmotic components in cotton genotypes subjected to short-term drought stress followed by recovery. Plant Biotechnology Reports 1 (1), 37–48. doi:10.1007/s11816-006-0004-1
Qiu, X. B., Shao, Y. M., Miao, S., and Wang, L. (2006). The diversity of the DnaJ/Hsp40 family, the crucial partners for Hsp70 chaperones. Cellular and Molecular Life Sciences CMLS 63 (22), 2560–2570. doi:10.1007/s00018-006-6192-6
Roy, S. (2016). Function of MYB domain transcription factors in abiotic stress and epigenetic control of stress response in plant genome. Plant Signaling and Behavior 11 (1), e1117723. doi:10.1080/15592324.2015.1117723
Saijo, Y., Loo, E. P. I., and Yasuda, S. (2018). Pattern recognition receptors and signaling in plant–microbe interactions. The Plant Journal 93 (4), 592–613. doi:10.1111/tpj.13808
Shimizu, T., Yoshii, A., Sakurai, K., Hamada, K., Yamaji, Y., Suzuki, M., et al. (2009). Identification of a novel tobacco DnaJ-like protein that interacts with the movement protein of tobacco mosaic virus. Archives of Virology 154 (6), 959–967. doi:10.1007/s00705-009-0397-6
Singh, B., Cheek, H. D., and Haigler, C. H. (2009). A synthetic auxin (NAA) suppresses secondary wall cellulose synthesis and enhances elongation in cultured cotton fiber. Plant Cell Reports 28 (7), 1023–1032. doi:10.1007/s00299-009-0714-2
Su, P. H., and Li, H. M. (2008). Arabidopsis stromal 70-kD heat shock proteins are essential for plant development and important for thermotolerance of germinating seeds. Plant Physiology 146 (3), 1231–1241. doi:10.1104/pp.107.114496
Sung, Y. Y., Rahman, N. A., Shazili, N. A. M., Chen, S., Lv, A., Sun, J., et al. (2018). Non-lethal heat shock induces Hsp70 synthesis and promotes tolerance against heat, ammonia and metals in post-larvae of the white leg shrimp Penaeus vannamei (Boone, 1931). Aquaculture 483, 21–26. doi:10.1016/j.aquaculture.2017.09.034
Takahashi, H., Kanayama, Y., Zheng, M. S., Kusano, T., Hase, S., Ikegami, M., et al. (2004). Antagonistic interactions between the SA and JA signaling pathways in Arabidopsis modulate expression of defense genes and gene-for-gene resistance to cucumber mosaic virus. Plant and Cell Physiology 45 (6), 803–809. doi:10.1093/pcp/pch085
Wang, S., Chang, Y., and Ellis, B. (2016). Overview of OVATE Family Proteins, a novel class of plant-specific growth regulators. Frontiers in Plant science 7, 417. doi:10.3389/fpls.2016.00417
Wen, F., Wu, X., Li, T., Jia, M., Liu, X., Li, P., et al. (2017). Genome-wide survey of heat shock factors and heat shock protein 70s and their regulatory network under abiotic stresses in Brachypodium distachyon. PloS One 12 (7), e0180352. doi:10.1371/journal.pone.0180352
Xiao, Y. H., Li, D. M., Yin, M. H., Li, X. B., Zhang, M., Wang, Y. J., et al. (2010). Gibberellin 20-oxidase promotes initiation and elongation of cotton fibers by regulating gibberellin synthesis. Journal of Plant Physiology 167 (10), 829–837. doi:10.1016/j.jplph.2010.01.003
Yer, E. N., Baloglu, M. C., and Ayan, S. (2018). Identification and expression profiling of all Hsp family member genes under salinity stress in different poplar clones. Gene 678, 324–336. doi:10.1016/j.gene.2018.08.049
Zhang, J., Fang, H., Zhou, H., Sanogo, S., and Ma, Z. (2014). Genetics, breeding, and marker-assisted selection for Verticillium wilt resistance in cotton. Crop Science 54 (4), 1289–1303. doi:10.2135/cropsci2013.08.0550
Zhang, Z. L., Zhu, J. H., Zhang, Q. Q., and Cai, Y. B. (2009). Molecular characterization of an ethephon-induced Hsp70 involved in high and low-temperature responses in Hevea brasiliensis. Plant Physiology and Biochemistry 47 (10), 954–959. doi:10.1016/j.plaphy.2009.06.003
Zhao, Y., Jing, H., Zhao, P., Chen, W., Li, X., Sang, X., et al. (2021). GhTBL34 is associated with verticillium wilt resistance in cotton. International Journal of Molecular Sciences 22 (17), 9115. doi:10.3390/ijms22179115
Zhong, X., Yang, J., Shi, Y., Wang, X., and Wang, G. L. (2018). The DnaJ protein OsDjA6 negatively regulates rice innate immunity to the blast fungus Magnaporthe oryzae. Molecular Plant Pathology 19 (3), 607–614. doi:10.1111/mpp.12546
Keywords: cotton, HSP40 gene family, HSP70 gene family, Verticillium wilt resistance, abiotic stress response
Citation: Zhou X, Su L, Tang R, Dong Y, Wang F, Li R, Xie Q, Zhang X, Xiao G and Li H (2023) Genome-wide analysis of Hsp40 and Hsp70 gene family in four cotton species provides insights into their involvement in response to Verticillium dahliae and abiotic stress. Front. Genet. 14:1120861. doi: 10.3389/fgene.2023.1120861
Received: 10 December 2022; Accepted: 09 January 2023;
Published: 26 January 2023.
Edited by:
Hang Zhao, Qufu Normal University, ChinaCopyright © 2023 Zhou, Su, Tang, Dong, Wang, Li, Xie, Zhang, Xiao and Li. This is an open-access article distributed under the terms of the Creative Commons Attribution License (CC BY). The use, distribution or reproduction in other forums is permitted, provided the original author(s) and the copyright owner(s) are credited and that the original publication in this journal is cited, in accordance with accepted academic practice. No use, distribution or reproduction is permitted which does not comply with these terms.
*Correspondence: Xianliang Zhang, zhangxianliang@caas.cn; Guanghui Xiao, guanghuix@snnu.edu.cn; Hongbin Li, lihb@shzu.edu.cn
†These authors contributed equally to this work