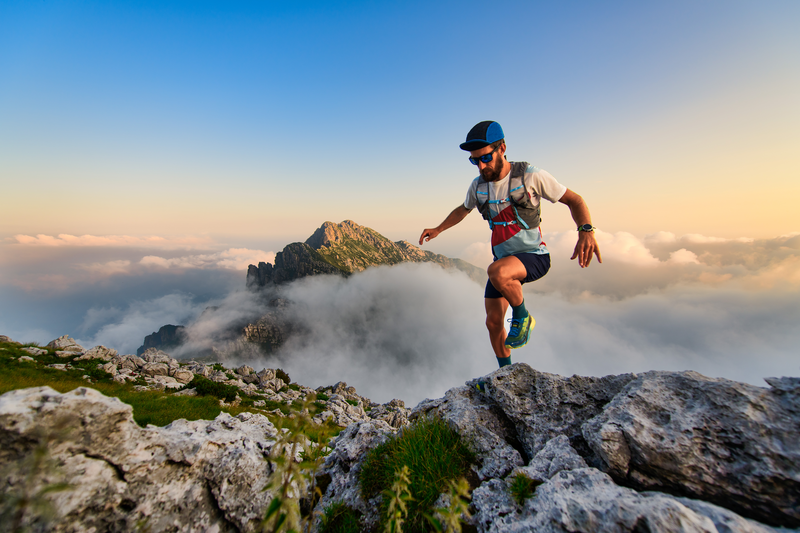
95% of researchers rate our articles as excellent or good
Learn more about the work of our research integrity team to safeguard the quality of each article we publish.
Find out more
ORIGINAL RESEARCH article
Front. Genet. , 27 March 2023
Sec. Cancer Genetics and Oncogenomics
Volume 14 - 2023 | https://doi.org/10.3389/fgene.2023.1107625
Introduction: Bladder cancer (BLCA) is one of the most common malignancies in the urinary system with a poor prognosis and high treatment costs. Identifying potential prognostic biomarkers is significant for exploring new therapeutic and predictive targets of BLCA.
Methods: In this study, we screened differentially expressed genes using the GSE37815 dataset. We then performed a weighted gene co-expression network analysis (WGCNA) to identify the genes correlated with the histologic grade and T stage of BLCA using the GSE32548 dataset. Subsequently, Kaplan Meier survival analysis and Cox regression were used to further identify prognosis-related hub genes using the datasets GSE13507 and TCGA-BLCA. Moreover, we detected the expression of the hub genes in 35 paired samples, including BLCA and paracancerous tissue, from the Shantou Central Hospital by qRT-polymerase chain reaction.
Results: This study showed that Anillin (ANLN) and Abnormal spindle-like microcephaly-associated gene (ASPM) were prognostic biomarkers for BLCA. High expression of ANLN and ASPM was associated with poor overall survival.The qRT-PCR results revealed that ANLN and ASPM genes were upregulated in BLCA, and there was a correlation between the expression of ANLN and ASPM in cancer tissues and paracancerous tissue. Additionally, the increasing multiples in the ANLN gene was obvious in high-grade BLCA.
Discussion: In summary, this preliminary exploration indicated a correlation between ANLN and ASPM expression. These two genes, serving as the risk factors for BLCA progression, might be promising targets to improve the occurrence and progression of BLCA.
Bladder cancer (BLCA) is the tenth most common malignant tumor globally, with approximately 570,000 new cases and 210,000 deaths yearly (Sung et al., 2021). Approximately 75% of patients with BLCA are diagnosed with non-muscle-invasive bladder cancer (NMIBC), whose standard therapy is transurethral resection of bladder tumor (TURBT) combined with intravesical instillation of chemotherapy or immunotherapy. However, 40% of these patients progress to muscle-invasive bladder cancer (MIBC) within 5 years (Sylvester et al., 2006; Babjuk et al., 2019). Specifically, patients with T1G3 NMIBC have a worse prognosis, with disease progression rates of 11.4% and 19.8%, and disease-specific mortality rates of 4.8% and 11.3% in 1 and 5 years, respectively (Cambier et al., 2016). In addition, patients with MIBC are often associated with lymph node metastasis or extravesical invasiveness. A large-sample study showed that the 5-year recurrence-free survival (RFS) of patients with MIBC was about 60%, and cancer-specific survival (CSS) was about 65% after radical cystectomy and pelvic lymphadenectomy (Shariat et al., 2006). However, the CSS of patients with MIBC progressing from NMIBC descended to 35%. The progression to MIBC and bladder cancer related death in high-risk NMIBC were relatively early events occurring within 48 months (van den Bosch and Alfred Witjes, 2011). The RFS of BLCA depends on the lymph node involvement and tumor stage. Studies have shown that with the progression of the tumor stage, the RFS continues to decline: the 5-year RFS of patients with pT1, pT2, pT3, and pT4 BLCA was 76%, 74%, 52%, and 36%, respectively (Stein et al., 2001). Unfortunately, this aggressive biological behavior, coupled with limited treatment options, resulted in a median survival time of only 15 months for patients with metastatic BLCA (von der Maase et al., 2005). This meant that the current treatment strategies based on patient imaging and histopathology could no longer meet the needs of the concept of precise treatment of BLCA. Therefore, exploring diagnosis and prognosis biomarkers for BLCA has great clinical significance.
In this study, we utilized the weighted gene co-expression network analysis (WGCNA) to explore gene modules exhibiting a highly correlated with tumor clinicopathological features (Zhang and Horvath, 2005; Langfelder and Horvath, 2008). This algorithm has been applied to analyze gene expression data in yeast and mice (Carlson et al., 2006; Miller et al., 2010), and has since been extended to explore the biological significance of human tumor-related genes, such as finding the molecular signature of subtypes of non-small-cell lung cancer and the prognostic biomarkers of breast cancer and BLCA (Tang et al., 2018; Niemira et al., 2019; Liu et al., 2021a).
Thus, our study aimed to identify prognostic biomarkers of bladder urothelial carcinoma using WGCNA and validate their expression characteristics, thereby providing novel targets for precision medicine in BLCA.
The workflow chart is presented in Figure 1. Raw GEO expression data were normalized and log2 transformed using the R package “affy”. The R package “limma” was utilized to screen differentially expressed genes (DEGs) in the dataset GSE37815 which contains 18 BLCA and 6 paracancerous tissue samples. The DEGs screening threshold was set as follows: ① |log2FC| ≥1 ② False discovery rate (FDR) <0.05. Raw TCGA-BLCA dataset were normalized and log2 transformed using the R package “DEseq2”. The dataset GSE32548 was used to construct WGCNA, and the datasets GSE13507 and TCGA-BLCA were used for validation to screen prognostic biomarkers. Samples with missing clinicopathological and survival information were excluded from the aforementioned datasets. The baseline tables of each dataset are presented in Tables 1, 2.
The R package “WGCNA” was used to construct the co-expression network after matching DEGs with dataset GSE32548. First, we checked all samples and deleted the outliers in dataset GSE32548. Then, we selected an appropriate soft-thresholding parameter β to ensure a scale-free network and construct the adjacent matrix. We used the dynamic cut tree method and set a minimum module size (minClusterSize = 30) and medium sensitivity (deepSplit = 2) to identify gene modules with high topological overlap. Furthermore, the obtained modules were combined with the histologic grade and T stage of BLCA to identify biologically meaningful gene modules. The relationship between genes and external clinicopathological features was further quantified by calculating Gene Significance (GS) and Module Membership (MM). We considered the following three criteria as indicators for screening the candidate hub gene: ① MM > 0.8 ② |GS| > 0.5 ③ p < 0.05.
We conducted the univariate and multivariate Cox regression analyses by R package “survival” to further search prognostic genes, using datasets GSE13507 and TCGA-BLCA as validation cohorts. We divided all samples from the validation cohorts into high- and low-expression groups based on the median of gene expression. The R packages “survminer” and “survival” were used to calculate overall survival (OS) and generate the Kaplan Meier (K-M) survival curve. Genes with a significant p-value (p < 0.05) in Cox regression and OS analyses were regarded as the prognosis-related hub genes.
To explore genes highly related to the above-mentioned prognostic hub gene, the GSE13507 dataset was divided into high- and low-expression groups based on the median of the hub gene expression. Subsequently, we screened the DEGs by the R package “limma” according to the above grouping. The screening criteria were set as follows: ①|log2FC| ≥2 ② false discovery rate (FDR) < 0.05. Additionally, pearson correlation analysis was performed using the “corrplot” package to compare the degree of correlation between genes.
From August 2020 to December 2021, 35 paired BLCA and paracancerous tissue samples were collected from newly diagnosed patients admitted to the Urology Department of Shantou Central Hospital. All patients underwent TURBT, and their postoperative pathological diagnosis was bladder urothelial carcinoma. BLCA and paracancerous tissue samples were collected during the surgery and stored in a −80°C refrigerator after frozen by liquid nitrogen. Additionally, we recorded patient clinicopathological information, including age, sex, BLCA invasion depth, histologic grade, tumor size, single or multiple tumors, primary or recurrent, and pre-treatment urine routine. The baseline information of 35 patients with BLCA is presented in Table 3. This research project was approved by the Medical Ethics Committee of Shantou Central Hospital.
TABLE 3. Baseline table of 35 patients with bladder cancer in the Department of Urology, Shantou Central Hospital.
Total RNA was extracted from tissue samples using Trizol reagent (Ambion, United States) following the manufacture’s protocol. The concentration and purity of extracted RNA were measured using a spectrophotometer (Allsheng, China). Then, complementary DNA (cDNA) was synthesized by reverse transcription using HiScript Reverse Transcriptase (RNase H) (VAZYME, China). Subsequently, quantitative real-time polymerase chain reaction (qRT-PCR) was performed using SYBR Green Master Mix (VAZYME, China) on an ABI QuantStudio 6 Real-Time PCR System (Applied Biosystems, United States). The relative expressions levels of related genes were detected using the 2−ΔΔCT method. The primer sequences used for amplification were as follows: Anillin (ANLN) forward 5′-GTGATTCTGTTGCTGTCCCG-3′ and reverse 5′-GCAGCCTTTTCCTCTGATGG-3′ primers; Abnormal spindle-like microcephaly-associated gene (ASPM) forward 5′-CGTCACCTTGGCTTATTGGG-3′ and reverse 5′-GAGGTCCCAGTTCTGTGTGA-3′ primers; β-action forward 5′-AGCGAGCATCCCCCA-AAGTT-3′ and reverse 5′-GGGCACGAAGGCTCATCATT-3′ primers.
The R software 3.6.3 and IBM SPSS software 25.0 were used for all statistical analyses. The data were presented as mean ± standard deviation. The Wilcoxon signed-rank test was used to compare the PCR results of tumor and paracancerous tissues. The Mann Witney Wilcoxon test was used to compare gene expression and clinicopathological characteristics, and Pearson’s coefficient was used to examine the correlation between hub genes. K-M survival curves were constructed to analyze OS between the high-expression and the low-expression groups. Cox regression was performed to screen the related risk factors for BLCA prognosis. A p-value of less than 0.05 was considered statistically significant.
Through differential expression analysis, we identified 694 DEGs (207 upregulated and 487 downregulated) in the GSE37815 dataset. The volcano plot and heatmap of DEGs are shown in Figures 2A, B. Then, 694 DEGs were used to construct WGCNA using the GSE32548 dataset. All 131 samples and their clinical information were included in the analysis without removing any outlier samples (Figure 2C). The network was constructed using a soft threshold β = 9 (scale-free R2 = 0.91) (Supplementary Figure S1 and Supplementary Table S1), and we identified four modules: brown, blue, turquoise, and grey, containing 158, 87, 180, and 267 genes, respectively (Supplementary Figure S2). Among these modules, the brown module showed the most significant correlation with histologic grade (cor = 0.76, p < 0.01) and T stage (cor = 0.63, p < 0.01) and was considered to be the key module in this study (Figure 2D). In addition, we examined the GS and MM related to histologic grade and T stage of BLCA in the brown module, as shown in Figures 2E–H. According to the candidate hub genes screening threshold, we identified 45 genes for further survival analysis (Figure 2I).
FIGURE 2. DEG analysis and WGCNA construction. (A) Volcano plot of GSE37815 DEGs. (B) Heatmap of GSE37815 DEGs. (C) GSE32548 clustering results and corresponding grade and stage information. (D) Correlation between ME of GSE32548 and clinical sample information. Distribution of GS and errors in the modules associated with the histologic grade (E) and T stage (F) of bladder cancer. Scatter plot of GS and MM related to the histologic grade (G) and T stage (H) of bladder cancer in brown module. (I) Venn diagram of bladder cancer grading-related GS, staging-related GS and brown module MM. ME, module eigengenes; GS, gene significance; MM, module membership.
The prognostic value of 45 candidate hub genes was validated in this study using the TCGA-BLCA and GSE13507 validation datasets. Firstly, we conducted univariate Cox regression analysis and found that the ANLN gene (HR = 1.23, 95% CI = 1.07–1.42, p = 0.003) was the only risk factor in the TCGA-BLCA dataset. In the GSE13507 dataset, univariate Cox regression analysis identified 43 genes related to BLCA prognosis, including ANLN (HR = 1.47, 95% CI = 1.18–1.83, p = 0.001). Subsequently, we performed multivariate Cox regression analysis including the 43 genes and identified ANLN (HR = 2.83, 95% CI = 1.29–6.21, p = 0.001) as a potential factor affecting the prognosis of BLCA. K-M survival curve suggested that the high-expression of ANLN had a poor OS in both datasets TCGA-BLCA and GSE13507 datasets (Figures 3A, B).
After determining ANLN as a hub gene, we performed differential expression analysis based on the median of ANLN expression in the GSE13507. We identified 10 DEGs (CEP55, CCNB2, TRIP13, ASPM, TOP2A, UBE2C, CDCA5, CDC20, CRH and CRTAC1) using the following screening criteria: ① |log2FC| ≥ 2 ② false discovery rate (FDR) < 0.05. Pearson correlation analysis revealed that ASPM exhibited the highest correlation with ANLN among all 10 DEGs in both TCGA-BLCA (R = 0.79, p < 0.01) and GSE13507 (R = 0.87, p < 0.01) datasets (Figures 4A, B). Moreover, high expression of ASPM was associated with shorter OS in the TCGA-BLCA and GSE13507 datasets, as detected by K-M survival analysis (Figures 4C, D). Meanwhile, we found that high expression of both ANLN and ASPM was associated with shorter survival time compared to low expression of ANLN and ASPM in the TCGA-BLCA and GSE13507 datasets (Figures 4E, F).
FIGURE 4. Correlation between ANLN related DEGs and ANLN based on TCGA-BLCA (A) and GSE13507 (B). K-M survival curves of ASPM in bladder cancer based on TCGA-BLCA (C) and GSE13507 (D). The K-M survival curves of ANLN and ASPM with different expression levels in bladder cancer based on TCGA-BLCA (E) and GSE13507 (F).
We collected paired samples of BLCA and paracancerous tissues, and measured the mRNA expression levels of ANLN and ASPM using RT-qPCR. As shown in Figures 5A, B and Table 4, the mRNA expression levels of ANLN and ASPM were significantly upregulated in BLCA. These results were consistent when we stratified patients with BLCA into NMIBC and MIBC. Furthermore, we found that there was a correlation between the expression of ANLN and ASPM in BLCA (R = 0.56, p < 0.01) and paracancerous tissues (R = 0.69, p < 0.01), as shown in Figure 5C. To further investigate the relationship between hub genes and BLCA, we defined an increasing multiples as the ratio of mRNA expression levels in BLCA tissues to those in paracancerous tissues. Our results showed that ANLN expression was upregulated in BLCA in 34 out of 35 patients (97.1%) (Figure 5D). Additionally, the increasing multiples of ANLN were significantly higher in high-grade BLCA than in low-grade (low-grade: 2.26 ± 2.12 vs. high-grade: 4.63 ± 3.47, p = 0.046). Similarly, we found that 32 out of 35 patients (91.4%) had higher mRNA expression levels of ASPM in BLCA than in paracancerous tissues (Figure 5E). However, there was no significant difference in the increasing scores of ASPM between high-grade and low-grade BLCA patients (low-grade: 2.10 ± 0.64 vs. high-grade: 4.34 ± 6.44, p = 0.194).
FIGURE 5. Comparison between bladder cancer tissues (N = 35) and paracancerous tissues (N = 35) according to the expression levels of ANLN (A) and ASPM genes (B). Correlation between ANLN and ASPM in bladder cancer tissues (N = 35) and paracancerous tissues (N = 35) (C). Comparison between bladder cancer tissues and normal tissues according to the increasing multiples in the mRNA expression levels of ANLN (D) and ASPM (E).
TABLE 4. Comparison between bladder cancer tissues and paracancerous tissues according to the expression levels of ANLN and ASPM genes in total samples (N = 35), NMIBC (N = 26) and MIBC (N = 9).
An ROC curve was performed to evaluate the diagnostic significance based on the mRNA expression levels of both the ANLN and ASPM genes. The ANLN gene exhibited a sensitivity of 88.6% and a specificity of 60% (p < 0.01), while the ASPM gene had a sensitivity of 82.9% and a specificity of 57.1% (p < 0.01) (Figure 6). Furthermore, we analyzed the relationship between the expression of ANLN and ASPM genes with the clinicopathological characteristics of BLCA. The results suggested no statistically significant difference between the mRNA expression levels of ANLN and ASPM and different clinicopathological features (Table 5).
FIGURE 6. ROC curve for the expression levels of both ANLN and ASPM genes in bladder cancer tissues versus paracancerous tissues. ROC, receiver operating characteristics; AUC, area under the curve.
TABLE 5. Relationship of the expression levels of ANLN and ASPM genes with clinicopathological characteristics in bladder cancer.
More than 1.6 million people live with BLCA worldwide. About 1 in 100 men and 1 in 400 women are diagnosed with BLCA sometime in life globally. BLCA has the highest lifetime treatment costs per patient among all cancer types, with an annual cost of 3.6 billion euro in the United States and almost 5 billion euro in Europe (Richters et al., 2020). Urothelial carcinomas account for 90% of bladder malignant tumors histologically (Lobo et al., 2020). While intravesical Bacillus Calmette-Guerin instillation therapy remains a crucial option for the patients with intermediate and high-risk NMIBC after TURBT, the research into the molecular biology and genetics of BLCA has revolutionized the treatment of MIBC and advanced disease, including immunotherapy and checkpoint inhibition, targeted therapy, and antibody-drug conjugates (Lenis et al., 2020). Therefore, identifying valuable diagnostic, therapeutic, and prognostic targets for BLCA to achieve personalized and precise treatment of BLCA is of clinical significance.
In this study, we utilized bioinformatics analysis to identify ANLN and ASPM genes as prognostic biomarkers for BLCA. The upregulation of ANLN and ASPM genes was observed in BLCA, and their high expression was associated with poor OS in patients with BLCA. The results of RT-qPCR further confirmed the upregulation of ANLN and ASPM in BLCA, which was observed in both MIBC and NMIBC. By comparing the increasing multiples, we found that the increasing multiples of ANLN was higher in high-grade tumors. The proportion of upregulated ANLN and ASPM was 97.1% and 91.4%, respectively, indicating that the upregulation of both genes was a common occurrence in patients with BLCA.
ANLN is located on the short arm of human chromosome 7 and encodes a conserved multi-domain protein that interacts with various biological partners, acting as a scaffold to organize the cytoskeleton (Piekny and Glotzer, 2008). ANLN was originally isolated from Drosophila melanogaster embryonic extract and could bind to F-actin. Therefore, it was also called Anillin actin-binding protein (Field and Alberts, 1995). During cytokinesis, ANLN interacts with regulators of cytoskeletal components such as actin, septins, RhoA, and Ect2, among others, to facilitate cytoplasmic division, morphological changes, and ultimately, cell division into two subunits (Tuan and Lee, 2020). Notably, the injection of antibodies targeting ANLN has been shown to slow furrow ingression and lead to the failure of cytokinesis (Oegema et al., 2000). Similarly, the depletion of ANLN in human or Drosophila cultured cells induces lateral furrow oscillation that fails cytokinesis (Piekny and Maddox, 2010). ANLN has been identified as a upregulated gene in various tumors driving cell proliferation (Tuan and Lee, 2020). In non-small cell lung cancer, inhibition of the ANLN gene expression could suppress tumor cell growth, resulting in larger morphology, multiple nuclei, and ultimately cell death (Suzuki et al., 2005). Knockdown of the ANLN gene in mice hepatocytes blocked cytokinesis and inhibited liver tumor development (Zhang et al., 2018). A study using transcriptome sequencing found that ANLN was significantly upregulated in BLCA tissues (log2FC = 2.926) (Zeng et al., 2017). PCR and immunohistochemical experiments on BLCA and paracancerous normal mucosal tissues showed that the expression of the ANLN gene is upregulated in BLCA, especially in MIBC and high-grade cancers. Meanwhile, patients with BLCA in the high-ANLN expression group showed poor CSS, progression-free survival (PFS) and RFS, compared with the low-ANLN expression group (Zeng et al., 2017). Knockdown of the ANLN gene could inhibit BLCA cell proliferation, migration, and invasion, as well as induce cell cycle arrest and abnormal binucleation (Zeng et al., 2017; Chen et al., 2022). Patients with MIBC exhibiting high expression of the ANLN gene had shorter OS and disease-specific survival after radical cystectomy (Wu et al., 2019). Taken together, these findings suggested that the expression of ANLN gene was upregulated in BLCA and promoted the proliferation and invasion of BLCA cells. High expression of ANLN predicted poor prognosis, which was consistent with our analysis.
ASPM is located on the long arm of human chromosome 1, encoding a large protein of 3,477 amino acids (410 kDa). This protein comprises a putative amino-terminal microtubule-binding domain, two calponin homology domains that commonly involve in actin binding, 81 IQ (isoleucine-glutamine) repeat motifs that potentially bind to calmodulin, and a carboxy-terminus (C-terminal domain) of unknown function (Kouprina et al., 2005; Zhong et al., 2005; Paramasivam et al., 2007). The human ASPM gene produces two splice isoforms of 410 and 250 kDa, enriched in centrosomes and intermediates, respectively. This suggests that these isoforms may undertake the significant cellular functions of ASPM and participate in the regulation of mitosis (Paramasivam et al., 2007). The homologous proteins of human ASPM in Drosophila, asp, is involved in spindle microtubule organization and cytokinesis during mitosis and meiosis. Mutations in the human ASPM gene can lead to the defective proliferation of neural progenitor cells, resulting in autosomal recessive primary microcephaly (MCPH) (Bond et al., 2002; Roberts et al., 2002; Bond et al., 2003). Studies have shown that ASPM participates in spindle organization, spindle positioning, and cytokinesis in all dividing cells, not just nerve cells, and widely expresses in various fetal and adult tissue cells, including liver, lung, kidney (Kouprina et al., 2005; Higgins et al., 2010). The ASPM gene is upregulated in various tumors, including ovarian cancer, breast cancer, colon cancer, glioblastoma, and BLCA (Kouprina et al., 2005; Saleh et al., 2020). Patients with BLCA who have high expression levels of ASPM had shorter OS and PFS. The proliferation rate of the T24 cell line transfected with si-ASPM was significantly lower than that in the si-Control group (Xu Y. et al., 2020). Nude mice receiving ASPM knockdown T24 cells developed smaller tumors with lower tumor proliferative activity, indicating a significant role of ASPM in BLCA proliferation and tumorigenesis (Gao et al., 2020). Additionally, the single-cell transcriptome analysis identified a group of ASPM + basal-like cell types, the number of which significantly increased in acute bladder urothelium injury caused by uropathogenic Escherichia coli. This suggested that ASPM + urothelial cells were involved in the bladder urothelial regeneration after injury (Li et al., 2021). Furthermore, bioinformatics analysis revealed that high expression of the ASPM gene is associated with the poor prognosis of patients with BLCA, indicating that BLCA originating from this ASPM + subpopulation might be highly malignant and invasive (Li et al., 2021).
Spearman correlation analysis revealed a correlation between the mRNA expression of ANLN and ASPM in BLCA and paracancerous tissues. The correlation was higher in paracancerous tissues, indicating that the interaction between ANLN and ASPM also exists in normal tissues. Previous studies have confirmed ANLN and ASPM as risk factors for the prognosis of patients with BLCA through silico data analysis (Chen et al., 2019; Wang et al., 2019; Xu et al., 2019; Li et al., 2020; Liu et al., 2021b). Our study aimed to further discuss the impact of these genes on BLCA. ANLN is a highly conserved multi-domain protein that interacts with multiple cytoskeletal components during cell division (Tuan and Lee, 2020). ASPM participates in regulating microtubules, which are the cytoskeletal fibers involved in various physiological functions of cells (Akhmanova and Steinmetz, 2019). Therefore, both ANLN and ASPM are cytoskeleton-associated proteins involved in cytokinesis (Schiewek et al., 2018). The cytoskeleton plays a crucial role in maintaining the shape and internal organization of cells, as well as in cell division and migration. In particular, cell migration is initiated by an actin-dependent protrusion of the cell’s leading edge, which comprises structures known as lamellipodia and filopodia (Imai-Sumida et al., 2017). Regulating the formation of cytoskeleton and pseudopodia promotes the invasion and metastasis of BLCA (Xu N. et al., 2020). Remodeling of the intracellular cytoskeleton is one of the critical mechanisms of tumorigenesis, as the cytoskeleton directly regulates two major characteristics of cancer cells: proliferation and motility (Jiang et al., 2009; Sagona and Stenmark, 2010; Akhshi et al., 2014; Fife et al., 2014). Human cells contain four major cytoskeletal components: actin, microtubules, intermediate filaments, and septin polymers (Spiliotis and Nelson, 2006; Hohmann and Dehghani, 2019). These components have complex interactions, control cellular organization through signaling crosstalk, and play an essential role in tumor development (Jiang et al., 2009; Sagona and Stenmark, 2010; Akhshi et al., 2014). Studies have shown that down-regulating the RAS-driven actin cytoskeleton and phosphatidylinositide 3-kinase/Akt pathway could induce apoptosis and inhibits cell growth, migration and invasion in human BLCA cells (Imai-Sumida et al., 2017).
In summary, our study identified two related prognostic biomarkers for BLCA: ANLN and ASPM. High expression of ANLN and ASPM was associated with poor OS in BLCA. Furthermore, our results showed that ANLN and ASPM were upregulated in BLCA tissues. We also observed a correlation between the mRNA expression of ANLN and ASPM in both BLCA and paracancerous tissues. Notably, the increasing multiples of ANLN was higher in high-grade BLCA. These results suggest that ANLN and ASPM genes may be potentially promising targets for BLCA. We continue to explore the interaction mechanism between both ANLN and ASPM genes in vitro and in vivo experiments.
The original contributions presented in the study are included in the article/Supplementary Material, further inquiries can be directed to the corresponding author.
The studies involving human participants were reviewed and approved by the ethics committee of Shantou Central Hospital. The patients/participants provided their written informed consent to participate in this study.
TY and ZC carried out experiments and data analysis. TY, ZC, GL, XH, and YZ drafted the manuscript. TY, ZC, and YZ participated in study design. SC and KC participated in sample and data collection. All authors read and approved the final manuscript.
The authors declare that the research was conducted in the absence of any commercial or financial relationships that could be construed as a potential conflict of interest.
All claims expressed in this article are solely those of the authors and do not necessarily represent those of their affiliated organizations, or those of the publisher, the editors and the reviewers. Any product that may be evaluated in this article, or claim that may be made by its manufacturer, is not guaranteed or endorsed by the publisher.
The Supplementary Material for this article can be found online at: https://www.frontiersin.org/articles/10.3389/fgene.2023.1107625/full#supplementary-material
Akhmanova, A., and Steinmetz, M. O. (2019). Microtubule minus-end regulation at a glance. J. Cell Sci. 132 (11), jcs227850. doi:10.1242/jcs.227850
Akhshi, T. K., Wernike, D., and Piekny, A. (2014). Microtubules and actin crosstalk in cell migration and division. Cytoskelet. Hob. 71 (1), 1–23. doi:10.1002/cm.21150
Babjuk, M., Burger, M., Comperat, E. M., Gontero, P., Mostafid, A. H., Palou, J., et al. (2019). European association of Urology guidelines on non-muscle-invasive bladder cancer (TaT1 and carcinoma in situ) - 2019 update. Eur. Urol. 76 (5), 639–657. doi:10.1016/j.eururo.2019.08.016
Bond, J., Roberts, E., Mochida, G. H., Hampshire, D. J., Scott, S., Askham, J. M., et al. (2002). ASPM is a major determinant of cerebral cortical size. Nat. Genet. 32 (2), 316–320. doi:10.1038/ng995
Bond, J., Scott, S., Hampshire, D. J., Springell, K., Corry, P., Abramowicz, M. J., et al. (2003). Protein-truncating mutations in ASPM cause variable reduction in brain size. Am. J. Hum. Genet. 73 (5), 1170–1177. doi:10.1086/379085
Cambier, S., Sylvester, R. J., Collette, L., Gontero, P., Brausi, M. A., van Andel, G., et al. (2016). EORTC nomograms and risk groups for predicting recurrence, progression, and disease-specific and overall survival in non-muscle-invasive stage Ta-T1 urothelial bladder cancer patients treated with 1-3 Years of maintenance Bacillus calmette-guerin. Eur. Urol. 69 (1), 60–69. doi:10.1016/j.eururo.2015.06.045
Carlson, M. R., Zhang, B., Fang, Z., Mischel, P. S., Horvath, S., and Nelson, S. F. (2006). Gene connectivity, function, and sequence conservation: Predictions from modular yeast co-expression networks. BMC Genomics 7, 40. doi:10.1186/1471-2164-7-40
Chen, Q., Hu, J., Deng, J., Fu, B., and Guo, J. (2019). Bioinformatics analysis identified key molecular changes in bladder cancer development and recurrence. Biomed. Res. Int. 2019, 3917982. doi:10.1155/2019/3917982
Chen, S., Gao, Y., Chen, F., and Wang, T. B. (2022). ANLN serves as an oncogene in bladder urothelial carcinoma via activating JNK signaling pathway. Urol. Int., 1–11. doi:10.1159/000524204
Field, C. M., and Alberts, B. M. (1995). Anillin, a contractile ring protein that cycles from the nucleus to the cell cortex. J. Cell Biol. 131 (1), 165–178. doi:10.1083/jcb.131.1.165
Fife, C. M., McCarroll, J. A., and Kavallaris, M. (2014). Movers and shakers: Cell cytoskeleton in cancer metastasis. Br. J. Pharmacol. 171 (24), 5507–5523. doi:10.1111/bph.12704
Gao, Z. Y., Yu, F., Jia, H. X., Ye, Z., and Yao, S. J. (2020). ASPM predicts poor prognosis and regulates cell proliferation in bladder cancer. Kaohsiung J. Med. Sci. 36 (12), 1021–1029. doi:10.1002/kjm2.12284
Higgins, J., Midgley, C., Bergh, A. M., Bell, S. M., Askham, J. M., Roberts, E., et al. (2010). Human ASPM participates in spindle organisation, spindle orientation and cytokinesis. BMC Cell Biol. 11, 85. doi:10.1186/1471-2121-11-85
Hohmann, T., and Dehghani, F. (2019). The cytoskeleton-A complex interacting meshwork. Cells 8 (4), 362. doi:10.3390/cells8040362
Imai-Sumida, M., Chiyomaru, T., Majid, S., Saini, S., Nip, H., Dahiya, R., et al. (2017). Silibinin suppresses bladder cancer through down-regulation of actin cytoskeleton and PI3K/Akt signaling pathways. Oncotarget 8 (54), 92032–92042. doi:10.18632/oncotarget.20734
Jiang, P., Enomoto, A., and Takahashi, M. (2009). Cell biology of the movement of breast cancer cells: Intracellular signalling and the actin cytoskeleton. Cancer Lett. 284 (2), 122–130. doi:10.1016/j.canlet.2009.02.034
Kouprina, N., Pavlicek, A., Collins, N. K., Nakano, M., Noskov, V. N., Ohzeki, J., et al. (2005). The microcephaly ASPM gene is expressed in proliferating tissues and encodes for a mitotic spindle protein. Hum. Mol. Genet. 14 (15), 2155–2165. doi:10.1093/hmg/ddi220
Langfelder, P., and Horvath, S. (2008). Wgcna: an R package for weighted correlation network analysis. BMC Bioinforma. 9, 559. doi:10.1186/1471-2105-9-559
Lenis, A. T., Lec, P. M., Chamie, K., and Mshs, M. D. (2020). Bladder cancer: A review. JAMA 324 (19), 1980–1991. doi:10.1001/jama.2020.17598
Li, J., Lou, Y., Li, S., Sheng, F., Liu, S., Du, E., et al. (2020). Identification and immunocorrelation of prognosis-related genes associated with development of muscle-invasive bladder cancer. Front. Mol. Biosci. 7, 598599. doi:10.3389/fmolb.2020.598599
Li, Y., Liu, Y., Gao, Z., Zhang, L., Chen, L., Wu, Z., et al. (2021). Single-cell transcriptomes of mouse bladder urothelium uncover novel cell type markers and urothelial differentiation characteristics. Cell Prolif. 54 (4), e13007. doi:10.1111/cpr.13007
Liu, Z., Sun, T., Zhang, Z., Bi, J., and Kong, C. (2021a). An 18-gene signature based on glucose metabolism and DNA methylation improves prognostic prediction for urinary bladder cancer. Genomics 113, 896–907. doi:10.1016/j.ygeno.2020.10.022
Liu, Z., Xu, L., Lin, Y., Hong, H., Wei, Y., Ye, L., et al. (2021b). Identification of biomarkers related to prognosis of bladder transitional cell carcinoma. Front. Genet. 12, 682237. doi:10.3389/fgene.2021.682237
Lobo, N., Shariat, S. F., Guo, C. C., Fernandez, M. I., Kassouf, W., Choudhury, A., et al. (2020). What is the significance of variant histology in urothelial carcinoma? Eur. Urol. Focus 6 (4), 653–663. doi:10.1016/j.euf.2019.09.003
Miller, J. A., Horvath, S., and Geschwind, D. H. (2010). Divergence of human and mouse brain transcriptome highlights Alzheimer disease pathways. Proc. Natl. Acad. Sci. U. S. A. 107 (28), 12698–12703. doi:10.1073/pnas.0914257107
Niemira, M., Collin, F., Szalkowska, A., Bielska, A., Chwialkowska, K., Reszec, J., et al. (2019). Molecular signature of subtypes of non-small-cell lung cancer by large-scale transcriptional profiling: Identification of key modules and genes by weighted gene Co-expression network analysis (WGCNA). Cancers (Basel) 12 (1), 37. doi:10.3390/cancers12010037
Oegema, K., Savoian, M. S., Mitchison, T. J., and Field, C. M. (2000). Functional analysis of a human homologue of the Drosophila actin binding protein anillin suggests a role in cytokinesis. J. Cell Biol. 150 (3), 539–552. doi:10.1083/jcb.150.3.539
Paramasivam, M., Chang, Y. J., and LoTurco, J. J. (2007). ASPM and citron kinase co-localize to the midbody ring during cytokinesis. Cell Cycle 6 (13), 1605–1612. doi:10.4161/cc.6.13.4356
Piekny, A. J., and Glotzer, M. (2008). Anillin is a scaffold protein that links RhoA, actin, and myosin during cytokinesis. Curr. Biol. 18 (1), 30–36. doi:10.1016/j.cub.2007.11.068
Piekny, A. J., and Maddox, A. S. (2010). The myriad roles of Anillin during cytokinesis. Semin. Cell Dev. Biol. 21 (9), 881–891. doi:10.1016/j.semcdb.2010.08.002
Richters, A., Aben, K. K. H., and Kiemeney, L. (2020). The global burden of urinary bladder cancer: An update. World J. Urol. 38 (8), 1895–1904. doi:10.1007/s00345-019-02984-4
Roberts, E., Hampshire, D. J., Pattison, L., Springell, K., Jafri, H., Corry, P., et al. (2002). Autosomal recessive primary microcephaly: An analysis of locus heterogeneity and phenotypic variation. J. Med. Genet. 39 (10), 718–721. doi:10.1136/jmg.39.10.718
Sagona, A. P., and Stenmark, H. (2010). Cytokinesis and cancer. FEBS Lett. 584 (12), 2652–2661. doi:10.1016/j.febslet.2010.03.044
Saleh, A. A., Gohar, S. F., Hemida, A. S., Elgharbawy, M., and Soliman, S. E. (2020). Evaluation of ASPM and TEF gene expressions as potential biomarkers for bladder cancer. Biochem. Genet. 58 (3), 490–507. doi:10.1007/s10528-020-09962-1
Schiewek, J., Schumacher, U., Lange, T., Joosse, S. A., Wikman, H., Pantel, K., et al. (2018). Clinical relevance of cytoskeleton associated proteins for ovarian cancer. J. Cancer Res. Clin. Oncol. 144 (11), 2195–2205. doi:10.1007/s00432-018-2710-9
Shariat, S. F., Karakiewicz, P. I., Palapattu, G. S., Lotan, Y., Rogers, C. G., Amiel, G. E., et al. (2006). Outcomes of radical cystectomy for transitional cell carcinoma of the bladder: A contemporary series from the bladder cancer research consortium. J. Urol. 176, 2414–2422; discussion 2422. ; discussion 2422. doi:10.1016/j.juro.2006.08.004
Spiliotis, E. T., and Nelson, W. J. (2006). Here come the septins: Novel polymers that coordinate intracellular functions and organization. J. Cell Sci. 119, 4–10. doi:10.1242/jcs.02746
Stein, J. P., Lieskovsky, G., Cote, R., Groshen, S., Feng, A. C., Boyd, S., et al. (2001). Radical cystectomy in the treatment of invasive bladder cancer: Long-term results in 1,054 patients. J. Clin. Oncol. 19 (3), 666–675. doi:10.1200/JCO.2001.19.3.666
Sung, H., Ferlay, J., Siegel, R. L., Laversanne, M., Soerjomataram, I., Jemal, A., et al. (2021). Global cancer statistics 2020: GLOBOCAN estimates of incidence and mortality worldwide for 36 cancers in 185 countries. CA Cancer J. Clin. 71 (3), 209–249. doi:10.3322/caac.21660
Suzuki, C., Daigo, Y., Ishikawa, N., Kato, T., Hayama, S., Ito, T., et al. (2005). ANLN plays a critical role in human lung carcinogenesis through the activation of RHOA and by involvement in the phosphoinositide 3-kinase/AKT pathway. Cancer Res. 65 (24), 11314–11325. doi:10.1158/0008-5472.CAN-05-1507
Sylvester, R. J., van der Meijden, A. P., Oosterlinck, W., Witjes, J. A., Bouffioux, C., Denis, L., et al. (2006). Predicting recurrence and progression in individual patients with stage Ta T1 bladder cancer using EORTC risk tables: A combined analysis of 2596 patients from seven EORTC trials. Eur. Urol. 49 (3), 466–465; discussion 475-477. discussion 475-467. doi:10.1016/j.eururo.2005.12.031
Tang, J., Kong, D., Cui, Q., Wang, K., Zhang, D., Gong, Y., et al. (2018). Prognostic genes of breast cancer identified by gene Co-expression network analysis. Front. Oncol. 8, 374. doi:10.3389/fonc.2018.00374
Tuan, N. M., and Lee, C. H. (2020). Role of anillin in tumour: From a prognostic biomarker to a novel target. Cancers (Basel) 12 (6), 1600. doi:10.3390/cancers12061600
van den Bosch, S., and Alfred Witjes, J. (2011). Long-term cancer-specific survival in patients with high-risk, non-muscle-invasive bladder cancer and tumour progression: A systematic review. Eur. Urol. 60 (3), 493–500. doi:10.1016/j.eururo.2011.05.045
von der Maase, H., Sengelov, L., Roberts, J. T., Ricci, S., Dogliotti, L., Oliver, T., et al. (2005). Long-term survival results of a randomized trial comparing gemcitabine plus cisplatin, with methotrexate, vinblastine, doxorubicin, plus cisplatin in patients with bladder cancer. J. Clin. Oncol. 23 (21), 4602–4608. doi:10.1200/JCO.2005.07.757
Wang, Y., Chen, L., Ju, L., Qian, K., Liu, X., Wang, X., et al. (2019). Novel biomarkers associated with progression and prognosis of bladder cancer identified by Co-expression analysis. Front. Oncol. 9, 1030. doi:10.3389/fonc.2019.01030
Wu, S., Nitschke, K., Heinkele, J., Weis, C. A., Worst, T. S., Eckstein, M., et al. (2019). ANLN and TLE2 in muscle invasive bladder cancer: A functional and clinical evaluation based on in silico and in vitro data. Cancers (Basel) 11 (12), 1840. doi:10.3390/cancers11121840
Xu, N., Qu, G. Y., Wu, Y. P., Lin, Y. Z., Chen, D. N., Li, X. D., et al. (2020a). ARPC4 promotes bladder cancer cell invasion and is associated with lymph node metastasis. J. Cell Biochem. 121 (1), 231–243. doi:10.1002/jcb.29136
Xu, Y., Wu, G., Li, J., Li, J., Ruan, N., Ma, L., et al. (2020b). Screening and identification of key biomarkers for bladder cancer: A study based on tcga and GEO data. Biomed. Res. Int. 2020, 8283401. doi:10.1155/2020/8283401
Xu, Z., Zhang, Q., Luh, F., Jin, B., and Liu, X. (2019). Overexpression of the ASPM gene is associated with aggressiveness and poor outcome in bladder cancer. Oncol. Lett. 17 (2), 1865–1876. doi:10.3892/ol.2018.9762
Zeng, S., Yu, X., Ma, C., Song, R., Zhang, Z., Zi, X., et al. (2017). Transcriptome sequencing identifies ANLN as a promising prognostic biomarker in bladder urothelial carcinoma. Sci. Rep. 7 (1), 3151. doi:10.1038/s41598-017-02990-9
Zhang, B., and Horvath, S. (2005). A general framework for weighted gene co-expression network analysis. Stat. Appl. Genet. Mol. Biol. 4, Article17. Article17. doi:10.2202/1544-6115.1128
Zhang, S., Nguyen, L. H., Zhou, K., Tu, H. C., Sehgal, A., Nassour, I., et al. (2018). Knockdown of anillin actin binding protein blocks cytokinesis in hepatocytes and reduces liver tumor development in mice without affecting regeneration. Gastroenterology 154 (5), 1421–1434. doi:10.1053/j.gastro.2017.12.013
Keywords: ANLN, ASPM, bladder urothelial carcinoma, weighted gene co-expression network analysis, WGCNA
Citation: Yang T, Chi Z, Liu G, Hong X, Cao S, Cheng K and Zhang Y (2023) Screening ANLN and ASPM as bladder urothelial carcinoma-related biomarkers based on weighted gene co-expression network analysis. Front. Genet. 14:1107625. doi: 10.3389/fgene.2023.1107625
Received: 25 November 2022; Accepted: 14 March 2023;
Published: 27 March 2023.
Edited by:
Eswar Shankar, The Ohio State University, United StatesReviewed by:
Prem P. Kushwaha, Case Western Reserve University, United StatesCopyright © 2023 Yang, Chi, Liu, Hong, Cao, Cheng and Zhang. This is an open-access article distributed under the terms of the Creative Commons Attribution License (CC BY). The use, distribution or reproduction in other forums is permitted, provided the original author(s) and the copyright owner(s) are credited and that the original publication in this journal is cited, in accordance with accepted academic practice. No use, distribution or reproduction is permitted which does not comply with these terms.
*Correspondence: Yonghai Zhang, emhhbmdfeW9uZ2hhaUAxMjYuY29t
†These authors share first authorship
Disclaimer: All claims expressed in this article are solely those of the authors and do not necessarily represent those of their affiliated organizations, or those of the publisher, the editors and the reviewers. Any product that may be evaluated in this article or claim that may be made by its manufacturer is not guaranteed or endorsed by the publisher.
Research integrity at Frontiers
Learn more about the work of our research integrity team to safeguard the quality of each article we publish.