- 1Department of Oncology, Queen’s University, Kingston, ON, Canada
- 2Division of Cancer Care and Epidemiology, Queen’s Cancer Research Institute, Kingston, ON, Canada
- 3Princess Margaret Cancer Centre, University Health Network, Toronto, ON, Canada
- 4Division of Medical Oncology & Hematology, Department of Medicine, Princess Margaret Cancer Centre and the University of Toronto, Toronto, ON, Canada
- 5Department of Surgical Oncology, University Health Network, Toronto, ON, Canada
Triple negative breast cancer (TNBC) has poor prognosis when compared to other breast cancer subtypes. Despite pre-clinical data supporting an immune targeted approach for TNBCs, immunotherapy has failed to demonstrate the impressive responses seen in other solid tumor malignancies. Additional strategies to modify the tumor immune microenvironment and potentiate response to immunotherapy are needed. In this review, we summarise phase III data supporting the use of immunotherapy for TNBC. We discuss the role of IL-1β in tumorigenesis and summarize pre-clinical data supporting IL-1β inhibition as a potential therapeutic strategy in TNBC. Finally, we present current trials evaluating IL-1β in breast cancer and other solid tumor malignancies and discuss future studies that may provide a strong scientific rationale for the combination of IL-1β and immunotherapy in the neoadjuvant and metastatic setting for people with TNBC.
Introduction
Breast cancer is the most common malignancy diagnosed in women worldwide (Sung et al., 2021). Triple negative breast cancers (TNBC), defined as tumors lacking expression of the estrogen, progesterone and HER2 receptor, account for approximately 10%–15% of all breast cancers diagnosed in the United States. However, TNBCs are responsible for a relatively large proportion of breast cancer deaths, with 5-year survival being 8%–16% lower than the best prognosis subtype (DeSantis et al., 2019). TNBC is more prevalent in young women, and Black women (19% vs. 9% for White women) (DeSantis et al., 2019), and those with BRCA mutations (Hartman et al., 2012). Emerging research has demonstrated that patients with ER-low disease [1%–9% as per ASCO-CAP criteria (Allison et al., 2020)] have clinical outcomes similar to patients with TNBC (Dieci et al., 2021; Schrodi et al., 2021), raising questions about current classifications systems and treatment paradigms for patients with ER-low disease. Given the poor outcomes associated with this important subtype of breast cancer, there is an unmet need for effective targeted treatments in this population.
TNBCs are heavily infiltrated by immune cells. Multiple studies have demonstrated that high tumor-associated macrophage (TAM) count is inversely related to survival (Schedin et al., 2007; DeNardo et al., 2009; Mahmoud et al., 2012), while high tumor-infiltrating lymphocyte (TIL) count [specifically circulating tumor lymphocytes (CTLs)] is associated with improved survival (Denkert et al., 2010; Loi et al., 2013; Adams et al., 2014; Garcia-Teijido et al., 2016; Savas et al., 2016). In addition to predicting improved survival in TNBC, TIL number predicts increased response to radiotherapy, neoadjuvant and adjuvant chemotherapy, and immunotherapy in a range of tumor types (Stanton et al., 2016; Pruneri et al., 2018). This immune infiltrated microenvironment provides a biological rationale for the treatment of TNBC through modulation of the immune microenvironment with immune checkpoint blockade (ICB) of the programmed death 1 (PD1) or cytotoxic T-lymphocyte-associated protein 4 (CTLA4) pathways (Khalil et al., 2016). In fact, PD-L1 inhibition in combination with chemotherapy has become standard of care for patients with TNBC in the neoadjuvant and metastatic settings. However, even though preclinical data supports an immune-targeted approach for breast cancer, studies to date have failed to demonstrate the impressive results seen in other tumor types such as melanoma and lung cancer, and not all patients with TNBC derive benefit. Ongoing research is needed to identify biomarkers that predict response to immunotherapy in breast cancer. Concurrent strategies to modify the TME to promote response to ICB are needed and one such example is IL-1beta inhibition either alone or in combination with chemotherapy and/or ICB.
Herein, we summarise current clinical evidence supporting the use of ICB in the metastatic and adjuvant/neoadjuvant setting for women with TNBC, discuss the role of IL-1β in tumorigenesis, and summarize pre-clinical and clinical data supporting IL-1β inhibition for TNBC as a novel immune-modulating therapeutic strategy, either alone or in combination with chemotherapy and/or ICB.
Immunotherapy and TNBC–Metastatic and neoadjuvant
Immunotherapy is now a guideline supported treatment for women with TNBC in both the neoadjuvant and metastatic setting. In the metastatic setting, emerging data suggests immunotherapy is active in selected patients with TNBC. Phase III studies investigating the addition of immunotherapy to standard chemotherapy for first-line metastatic TNBC found improved objective response rates [63% versus 55% in Impassion 131 (Miles et al., 2021); 56% vs. 45.9% in Impassion 130 (Schmid et al., 2018); and 41% vs. 35.9% in Keynote-355 (Cortes et al., 2022)]. However, this has not consistently translated into improvements in overall survival in the intention to treat population [mOS 17.2 vs. 15.5 in Keynote 355 (Cortes et al., 2022); 19.2 vs. 22.8 in Impassion 131 (Miles et al., 2021); 21 vs. 18.7 in Impassion 130 (Emens et al., 2021)]. Failure to demonstrate statistically significant improvements in overall survival with atezolizumab in Impassion 130 and the potential detrimental survival effects seen in Impassion 131 led to the withdrawal of approval of atezolizumab for metastatic TNBC by the Food and Drug Administration (FDA). Despite these negative results, subgroup analyses have demonstrated more favourable outcomes in patients with higher levels of PDL1 expression (mOS 25.4 vs. 17.9 months in PDL1 positive Impassion130, 23 vs. 16.1 months in Keynote 355 with CPS>10), but again the findings have been inconsistent (22.1 vs. 28.3 months in Impassion 131).
In phase III studies in the neoadjuvant setting, the addition of immunotherapy to standard chemotherapy for patients with TNBC led to improved pathologic complete response (pCR) (64.8% vs. 51.2% Keynote-522 (Schmid et al., 2020); 58% vs. 41% in Impassion-031; 43.5% vs. 40.8% in NeoTRIP). Some studies have also demonstrated prolonged event free survival [36-month EFS 84.5% vs. 76.8% in Keynote-522 (Schmid et al., 2022)]. As was seen in the metastatic setting, patients with higher PDL1 expression have higher pCR with the addition of immunotherapy, but also have improved response rates to chemotherapy alone, indicating that PDL1 expression may be a prognostic biomarker in the neoadjuvant setting for TNBC.
Although immunotherapy has demonstrated promise as a strategy for women with TNBC, not all patients derive benefit, and additional immune priming strategies to improve ICB response rates in TNBC are needed. Novel therapies capable of modulating immunosuppressive TAMs (Schmid and Varner, 2010; Coussens et al., 2013; Gajewski et al., 2013), which can suppress CTLs through ICB-independent mechanisms (Katakura et al., 2004; Meyer et al., 2014; Noy and Pollard, 2014; Bjoern et al., 2016), are an obvious therapeutic target. IL-1beta is a pleiotropic cytokine capable of recruiting TAMs and generating an immunosuppressive environment, making it an interesting therapeutic target for women with breast cancer.
The role of IL1β in tumorigenesis in TNBC
IL-1 has roles in both physiological and pathological states, including angiogenesis, tumor growth and metastases. Studies have shown that IL-1 can be directly produced by cancer cells, or can educate cells within the tumor microenvironment to produce IL-1 (Malik and Kanneganti, 2018), illustrating the complex signalling and interplay between the tumor and surrounding cells. IL-1α is localised in the cytosol and acts within the intracellular environment whereas IL1β is secreted extracellularly and may act on surrounding tissues. Once IL1β binds to its receptor IL-1R1, downstream signalling leads to the activation of NF-kB dependent genes, which in turn can promote cancer growth. Pre-clinical work has found that tumor cell IL1β drives TAM recruitment, immunosuppression, and tumor progression in TNBC (Shen et al., 2017; Jaiswal et al., 2021) (Figure 1). Experimental evidence across most cancer types, supports a tumor-promoting role for IL1β [reviewed (Maund et al., 2013; Ridker et al., 2017; Baker et al., 2019; Gelfo et al., 2020)] making IL1β a target with clear therapeutic potential.
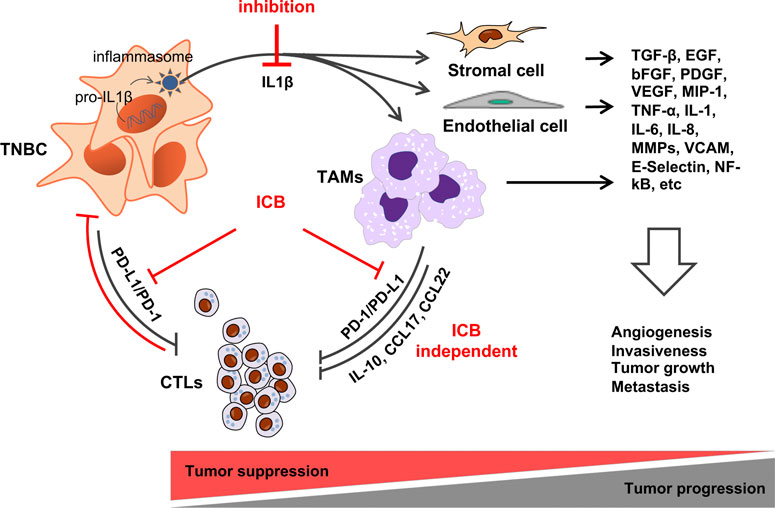
FIGURE 1. Hypothesis: IL1β-induced TAM recruitment to TNBC promotes tumor progression by inducing immune suppression. TAMs can suppress CTLs via immune checkpoint-dependent and - independent mechanisms making ICB ineffective. By preventing TAM recruitment, and the activation of other cell types within the TME, IL1β inhibition will prevent angiogenesis, tumor cell invasion, metastasis and convert tumors to an ICB-sensitive state. See text for details.
The role of IL1β in growth, invasion and metastases (Guo et al., 2016), stemness and EMT (Oh et al., 2016) has been extensively described. IL1β has an established role in angiogenesis (Krelin et al., 2007) across multiple tumor types. IL1β and vascular endothelial growth factors (VEG-F) are drivers in the establishment and maintenance of cancer-related angiogenesis. Studies have demonstrated that angiogenesis and VEG-F production are IL-1 dependent (Dinarello, 2010), and that IL-1 deficient mice do not induce inflammatory or angiogenic responses (Carmi et al., 2009). IL1β also plays an essential role in the maturation of endothelial precursor cells into endothelial cells by synergistically interacting with VEGF (Voronov et al., 2014). Humans treated with the IL1β antagonist Anakinra for rheumatoid arthritis have reduced numbers of blood vessels in the pannus (Cunnane et al., 2001).
IL1β also plays an important role in tumor cell migration and the establishment of metastatic deposits. IL-1 activates downstream pathways including NF-kB which increases the migratory activity of breast cancer cells, and in turn upregulates CXCL8 under oxygen deprivation (Naldini et al., 2010; Filippi et al., 2015; Storr et al., 2017). The binding of CXCL8 to its receptors CXCR1 and CXCR2 leads to the activation and downstream trafficking of inflammatory mediators, tumor proliferation and breast cancer development (Mishra et al., 2021). In mouse models, it has been shown that by blocking IL-1R, tumor progression and metastasis can be slowed (Holen et al., 2016).
Within the tumor microenvironment, IL-1 has also been shown to modulate anti-tumor activity. IL1β is involved in tumoral recruitment of TAMs and other pro-tumoral inflammatory cells (Kaplanov et al., 2019a). IL-1 secretion can expand and modulate the activities of myeloid derives suppressor cells (MDSC) and downregulate immune surveillance and antitumor immunity (Tu et al., 2008).
Although the majority of published studies suggesting a tumor promoting role for IL-1β, several studies have also demonstrated a tumor inhibiting effect, and the impact of IL1β signalling may vary depending on the cell type and the microenvironment (Dmitrieva-Posocco et al., 2019). For example, breast cancer tumors can elicit a systemic inflammatory response involving IL-1beta expressing innate immune cells which can then migrate and act at distant metastatic lesions. Once these innate immune cells reach the distant metastatic sites, they prevent conversion of tumor cells to a ZEB-1 negative state, thereby limiting epithelioid differentiation and the metastatic cells ability to establish itself. In some pre-clinical models, inhibiting IL-1beta eliminates the differentiation block and drives metastatic colonization (Castaño et al., 2018). However, once the metastatic deposit has been established, IL1beta inhibition did not alter tumor growth.
IL1β secretion requires two-steps. Step 1 (i.e., “priming”) involves the induction of mRNA and protein production of an inactive IL1β pro-protein (pro-IL-1β). Classically this occurs in cells of the innate immune system in response to molecular motifs called ‘pathogen associated molecular patterns’ (PAMPs) that are carried by invading microbes. However, as demonstrated in pre-clinical models, in TNBC priming is driven by aberrant activation of the Notch developmental signaling pathway (Shen et al., 2017). In step 2 of IL1β production (i.e., “cleavage”), in response to PAMPs or ‘danger associated molecular patterns’ (DAMPs), a multiprotein cytosolic complex called the “inflammasome” is assembled (Lopez-Castejon and Brough, 2011; Winsor et al., 2019). With assembly of this complex, pro-caspase-1 (p45) is recruited and activated (Martinon et al., 2002). Activated caspase-1 promotes proteolytic cleavage, maturation, and secretion of IL-1β (Thornberry et al., 1992; Broz and Dixit, 2016). While inflammasomes have traditionally been studied in immune cells, emerging evidence indicates that inflammasome components including caspase-1 are expressed in TNBC cells and correlate with macrophage recruitment (Shen et al., 2017). Thus, TNBCs are uniquely capable of IL1β production due to Notch-induced IL1β priming, and the presence of inflammasome components required for IL1β cleavage, maturation and secretion, making TNBC an excellent candidate for IL1β blockade.
Pre-clinical models supporting IL1β inhibition for TNBC
Both in vitro (Soria et al., 2011; Ma et al., 2012) and in vivo (Holen et al., 2016) studies have demonstrated an association between IL-1β expression and metastatic potential for breast cancer. High expression of IL-1β in the primary tumor is associated with disease recurrence at any site for breast cancer, and specifically with bony metastases (Nutter et al., 2014). In mouse models, IL-1 blockade using Anakinra reduced the development and progression of bony metastases from breast cancer (Holen et al., 2016). IL1β knock-out mice treated with orthotopically introduced breast cancer cells showed initial tumor growth followed by subsequent regression, due to the recruitment of alternative inflammatory monocytes in the tumor microenvironment (Kaplanov et al., 2019b). In IL1β deficient mice, increased secretion of IL-12 supports anti-tumor immunity and induces the activation of CD8+ T-cell which infiltrate tumors and promote regression (Kaplanov et al., 2019b).
Pre-clinical studies have also shown potential synergistic effects for combining PD1/PD-L1 inhibition with IL-1β inhibition. PD-1 blockade can slow breast cancer tumor growth in some commonly used syngeneic models. However, in one study, combining anti-IL1β antibodies with PD-1 blockade halted tumor progression altogether (Kaplanov et al., 2019b), illustrating that IL1β blockade could facilitate checkpoint inhibition.Clinical data supporting IL1β inhibition in TNBC and other solid tumor malignancies
There are several IL-1β inhibitors already approved by the FDA for a range of rheumatological and autoimmune conditions, including Anakinra, Canakinumab and Rilonacept. IL1β inhibition with either Anakinra or Canakinumab is being explored as a therapeutic strategy in a wide range of solid tumor malignancies, either alone or in combination with chemotherapy, immunotherapy and targeted treatments (Table 1).
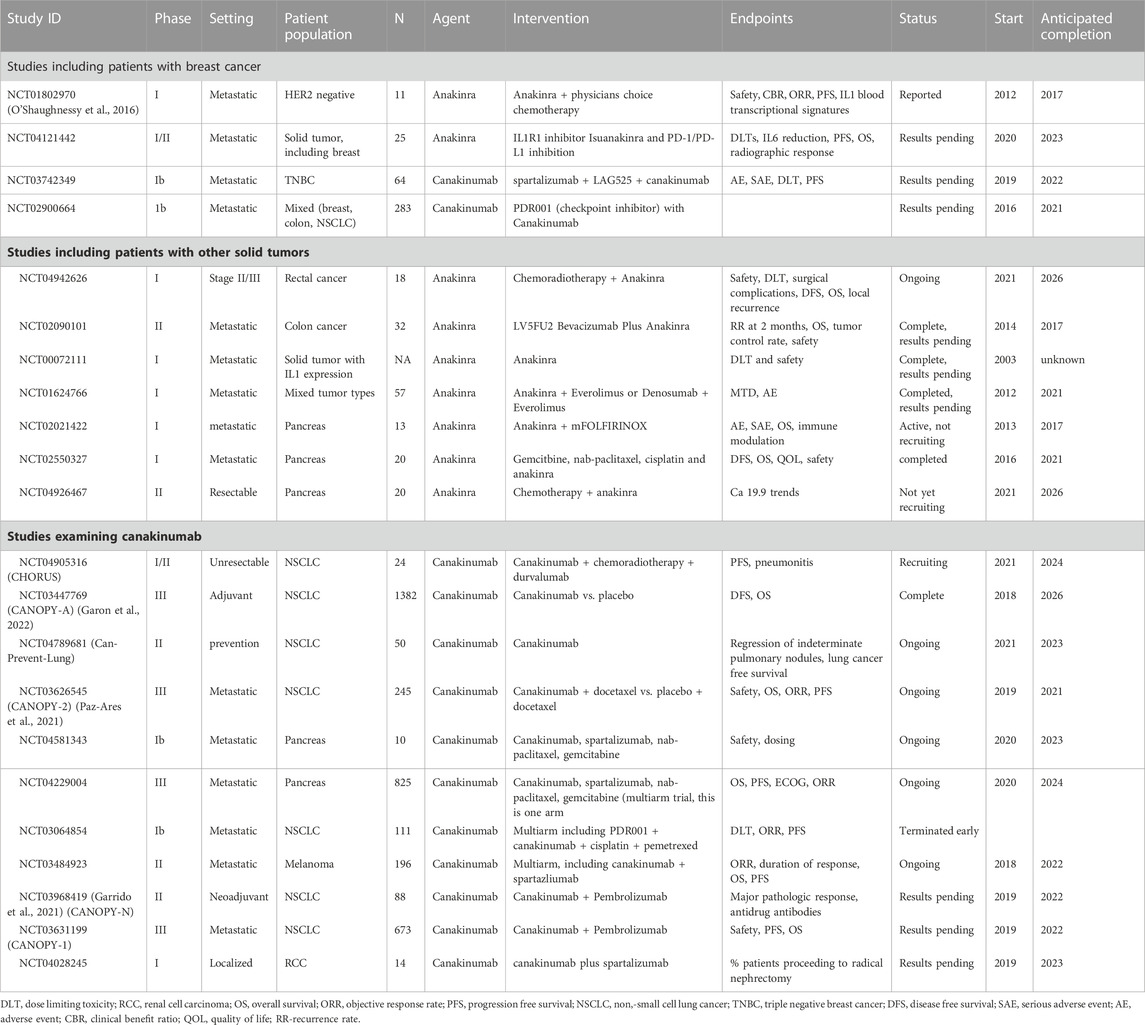
TABLE 1. Ongoing and complete trials examining IL1β inhibition for solid tumor malignancies (datasource: clinicaltrials.gov).
To our knowledge, only one reported early phase trial has investigated IL1β inhibition for women with breast cancer. NCT01802970 (O'Shaughnessy et al., 2016) enrolled 11 patients with HER2 negative metastatic breast cancer and treated them with daily administration of Anakinra in addition to physician’s choice chemotherapy. The combination was well tolerated, with the predominant toxicity of Anakinra being injection site reactions (O'Shaughnessy et al., 2016). Four patients had reduction in tumor volume, 4 had stable disease, and 3 had progressive disease, for an overall response rate of 36%. Two patients stopped Anakinra for injection site reactions. Several other studies in breast cancer examining IL-1β inhibition either alone or in combination with chemotherapy or immunotherapy in the metastatic setting are ongoing (NCT04121442, NCT03742349, NCT02900664).
In addition to the early phase data supporting the role of IL1β inhibition in solid tumor malignancies, the phase III CANTOS trial randomised 10,061 patients with heart failure to the IL1β inhibitor canakinumab (Ilaris®) or placebo (Ridker et al., 2017), and found a greater than 50% reduction in the risk of death from all cancers. Although the primary endpoint of this study was non-fatal myocardial infarction, non-fatal stroke or cardiovascular death, and the study was not powered to investigate differences in cancer outcomes, these findings support pre-clinical data on the potential role of IL1β inhibition for cancer control. However, subsequent phase III studies in lung cancer including CANOPY-A and CANOPY-2 have failed to demonstrated improvements in progression-free or overall survival (Paz-Ares et al., 2021; Garon et al., 2022), raising questions about this therapeutic strategy in unselected patients. More recent data has identified a potential mechanism for the recognised link between air pollution and lung cancer. Swanton et al. (2022) found that exposure to 2.5 µm particulate matter led to an influx of macrophages and inflammatory mediators including IL-1β which induces a progenitor-like state in the lung epithelium harbouring mutant EGFR, promoting tumor formation. Together, these findings provide compelling evidence of a role for IL-1β in tumorigenesis, and highlight the ongoing work needed to identify valid biomarkers in this patient population in order harness the therapeutic potential of IL-1β blockade for patients with breast cancer and other solid tumor malignancies.
Future directions and ongoing research
Ongoing work in vivo is required to better understand the immunomodulatory effects of IL-1β inhibition in patients with TNBC, and the potential synergism with ICB and other immunomodulatory drugs. In an upcoming study, we will evaluate IL-1β blockade in the neoadjuvant setting for women with early TNBC, examining changes to key immune biomarkers of the tumor microenvironment (including TILs, TAMs, NK cells, IL-1β and inflammasome expression) using paired samples pre and post anti-IL-1β therapy. This in-depth analysis using multiplex immunohistochemistry, high-dimensional mass cytometry, and T and B-cell repertoire analysis will provide much needed granular data on the immunomodulatory effects of IL-1β blockade for women with TNBC and provide a strong scientific rationale for future studies examining the therapeutic potential of the combination of IL-1β blockade and immunotherapy in the neoadjuvant and metastatic setting.
Author contributions
BW-concept development, manuscript writing. DC-concept development, manuscript writing. QS-concept development, manuscript writing. MR-concept development, manuscript writing.
Funding
This review was supported by funds to MR from the Canadian Cancer Society (Award #707511). This work was funded in part by the Ontario Ministry of Health and Long Term Care.
Conflict of interest
DC reports consulting/advisory fees from AstraZeneca, Eisai, Exact Sciences, GlaxoSmithKline, INFLEX Ltd, Lilly, Merck, Novartis, Pfizer, Roche and SAGA Diagnostics; research support (to institution) from AstraZeneca, Gilead, GlaxoSmithKline, Inivata, Knight Therapeutics, Merck, Pfizer and Roche outside the submitted work; and intellectual property including methods of treating cancers characterized by a high expression level of spindle and kinetochore associated complex subunit 3 (ska3) gene (US62/675,228), all outside of the submitted work.
The remaining authors declare that the research was conducted in the absence of any commercial or financial relationships that could be construed as a potential conflict of interest.
Publisher’s note
All claims expressed in this article are solely those of the authors and do not necessarily represent those of their affiliated organizations, or those of the publisher, the editors and the reviewers. Any product that may be evaluated in this article, or claim that may be made by its manufacturer, is not guaranteed or endorsed by the publisher.
References
Adams, S., Gray, R. J., Demaria, S., Goldstein, L., Perez, E. A., Martino, S., et al. (2014). Prognostic value of tumor-infiltrating lymphocytes in triple-negative breast cancers from two phase III randomized adjuvant breast cancer trials: ECOG 2197 and ECOG 1199. J. Clin. Oncol. 32, 2959–2966. doi:10.1200/JCO.2013.55.0491
Allison, K. H., Hammond, M. E. H., Dowsett, M., McKernin, S. E., Carey, L. A., Fitzgibbons, P. L., et al. (2020). Estrogen and progesterone receptor testing in breast cancer: ASCO/CAP guideline update. J. Clin. Oncol. 38 (12), 1346–1366. doi:10.1200/JCO.19.02309
Baker, K. J., Houston, A., and Brint, E. (2019). IL-1 family members in cancer; two sides to every story. Front. Immunol. 10, 1197. doi:10.3389/fimmu.2019.01197
Bjoern, J., Juul Nitschke, N., Zeeberg Iversen, T., Schmidt, H., Fode, K., and Svane, I. M. (2016). Immunological correlates of treatment and response in stage IV malignant melanoma patients treated with Ipilimumab. Oncoimmunology 5 (4), e1100788. doi:10.1080/2162402X.2015.1100788
Broz, P., and Dixit, V. M. (2016). Inflammasomes: Mechanism of assembly, regulation and signalling. Nat. Rev. Immunol. 16 (7), 407–420. doi:10.1038/nri.2016.58
Carmi, Y., Voronov, E., Dotan, S., Lahat, N., Rahat, M. A., Fogel, M., et al. (2009). The role of macrophage-derived IL-1 in induction and maintenance of angiogenesis. J. Immunol. 183 (7), 4705–4714. doi:10.4049/jimmunol.0901511
Castaño, Z., San Juan, B. P., Spiegel, A., Pant, A., DeCristo, M. J., Laszewski, T., et al. (2018). IL1β inflammatory response driven by primary breast cancer prevents metastasis-initiating cell colonization. Nat. Cell Biol. 20 (9), 1084–1097. doi:10.1038/s41556-018-0173-5
Cortes, J., Rugo, H. S., Cescon, D. W., Im, S. A., Yusof, M. M., Gallardo, C., et al. (2022). Pembrolizumab plus chemotherapy in advanced triple-negative breast cancer. N. Engl. J. Med. 387 (3), 217–226. doi:10.1056/NEJMoa2202809
Coussens, L. M., Zitvogel, L., and Palucka, A. K. (2013). Neutralizing tumor-promoting chronic inflammation: A magic bullet? Science 339 (6117), 286–291. doi:10.1126/science.1232227
Cunnane, G., Madigan, A., Murphy, E., FitzGerald, O., and Bresnihan, B. (2001). The effects of treatment with interleukin-1 receptor antagonist on the inflamed synovial membrane in rheumatoid arthritis. Rheumatology 40 (1), 62–69. doi:10.1093/rheumatology/40.1.62
DeNardo, D. G., Barreto, J. B., Andreu, P., Vasquez, L., Tawfik, D., Kolhatkar, N., et al. (2009). CD4+ T cells regulate pulmonary metastasis of mammary carcinomas by enhancing protumor properties of macrophages. Cancer Cell 16 (2), 91–102. doi:10.1016/j.ccr.2009.06.018
Denkert, C., Loibl, S., Noske, A., Roller, M., Muller, B. M., Komor, M., et al. (2010). Tumor-associated lymphocytes as an independent predictor of response to neoadjuvant chemotherapy in breast cancer. J. Clin. Oncol. 28 (1), 105–113. doi:10.1200/JCO.2009.23.7370
DeSantis, C. E., Ma, J., Gaudet, M. M., Newman, L. A., Miller, K. D., Goding Sauer, A., et al. (2019). Breast cancer statistics, 2019. CA a cancer J. Clin. 69 (6), 438–451. doi:10.3322/caac.21583
Dieci, M. V., Griguolo, G., Bottosso, M., Tsvetkova, V., Giorgi, C. A., Vernaci, G., et al. (2021). Impact of estrogen receptor levels on outcome in non-metastatic triple negative breast cancer patients treated with neoadjuvant/adjuvant chemotherapy. NPJ Breast Cancer 7 (1), 101–107. doi:10.1038/s41523-021-00308-7
Dinarello, C. A. (2010). Why not treat human cancer with interleukin-1 blockade? Cancer Metastasis Rev. 29, 317–329. doi:10.1007/s10555-010-9229-0
Dmitrieva-Posocco, O., Dzutsev, A., Posocco, D. F., Hou, V., Yuan, W., Thovarai, V., et al. (2019). Cell-type-specific responses to interleukin-1 control microbial invasion and tumor-elicited inflammation in colorectal cancer. Immunity 50 (1), 166–180. e7. doi:10.1016/j.immuni.2018.11.015
Emens, L., Adams, S., Barrios, C., Dieras, V., Iwata, H., Loi, S., et al. (2021). First-line atezolizumab plus nab-paclitaxel for unresectable, locally advanced, or metastatic triple-negative breast cancer: IMpassion130 final overall survival analysis. Ann. Oncol. 32 (8), 983–993. doi:10.1016/j.annonc.2021.05.355
Filippi, I., Carraro, F., and Naldini, A. (2015). Interleukin-1β affects MDAMB231 breast cancer cell migration under hypoxia: Role of HIF-1α and NFκB transcription factors. Mediat. Inflamm. 2015, 789414. doi:10.1155/2015/789414
Gajewski, T. F., Schreiber, H., and Fu, Y. X. (2013). Innate and adaptive immune cells in the tumor microenvironment. Nat. Immunol. 14 (10), 1014–1022. doi:10.1038/ni.2703
Garcia-Teijido, P., Cabal, M. L., Fernandez, I. P., and Perez, Y. F. (2016). Tumor-infiltrating lymphocytes in triple negative breast cancer: The future of immune targeting. Clin. Med. Insights Oncol. 10, 31–39. doi:10.4137/CMO.S34540
Garon, E., Lu, S., Goto, Y., De Marchi, P., Paz-Ares, L., Spigel, D., et al. (2022). LBA49 canopy-a: Phase III study of canakinumab (CAN) as adjuvant therapy in patients (pts) with completely resected non-small cell lung cancer (NSCLC). Ann. Oncol. 33, S1414–S1415. doi:10.1016/j.annonc.2022.08.049
Garrido, P., Pujol, J-L., Kim, E. S., Lee, J. M., Tsuboi, M., Gomez-Rueda, A., et al. (2021). Canakinumab with and without pembrolizumab in patients with resectable non-small-cell lung cancer: CANOPY-N study design. Future Oncol. 17 (12), 1459–1472. doi:10.2217/fon-2020-1098
Gelfo, V., Romaniello, D., Mazzeschi, M., Sgarzi, M., Grilli, G., Morselli, A., et al. (2020). Roles of IL-1 in cancer: From tumor progression to resistance to targeted therapies. Int. J. Mol. Sci. 21 (17), 6009. doi:10.3390/ijms21176009
Guo, B., Fu, S., Zhang, J., Liu, B., and Li, Z. (2016). Targeting inflammasome/IL-1 pathways for cancer immunotherapy. Sci. Rep. 6, 36107. doi:10.1038/srep36107
Hartman, A. R., Kaldate, R. R., Sailer, L. M., Painter, L., Grier, C. E., Endsley, R. R., et al. (2012). Prevalence of BRCA mutations in an unselected population of triple-negative breast cancer. Cancer 118 (11), 2787–2795. doi:10.1002/cncr.26576
Holen, I., Lefley, D. V., Francis, S. E., Rennicks, S., Bradbury, S., Coleman, R. E., et al. (2016). IL-1 drives breast cancer growth and bone metastasis in vivo. Oncotarget 7 (46), 75571–75584. doi:10.18632/oncotarget.12289
Jaiswal, A., Murakami, K., Elia, A., Shibahara, Y., Done, S. J., Wood, S. A., et al. (2021). Therapeutic inhibition of USP9x-mediated Notch signaling in triple-negative breast cancer. Proc. Natl. Acad. Sci. U. S. A. 118 (38), e2101592118. doi:10.1073/pnas.2101592118
Kaplanov, I., Carmi, Y., Kornetsky, R., Shemesh, A., Shurin, G. V., Shurin, M. R., et al. (2019). Blocking IL-1β reverses the immunosuppression in mouse breast cancer and synergizes with anti-PD-1 for tumor abrogation. Proc. Natl. Acad. Sci. U. S. A. 116 (4), 1361–1369. doi:10.1073/pnas.1812266115
Kaplanov, I., Carmi, Y., Kornetsky, R., Shemesh, A., Shurin, G. V., Shurin, M. R., et al. (2019). Blocking IL1β reverses the immunosuppression in mouse breast cancer and synergizes with anti–PD-1 for tumor abrogation. Proc. Natl. Acad. Sci. 116 (4), 1361–1369. doi:10.1073/pnas.1812266115
Katakura, T., Miyazaki, M., Kobayashi, M., Herndon, D. N., and Suzuki, F. (2004). CCL17 and IL-10 as effectors that enable alternatively activated macrophages to inhibit the generation of classically activated macrophages. J. Immunol. 172 (3), 1407–1413. doi:10.4049/jimmunol.172.3.1407
Khalil, D. N., Smith, E. L., Brentjens, R. J., and Wolchok, J. D. (2016). The future of cancer treatment: Immunomodulation, CARs and combination immunotherapy. Nat. Rev. Clin. Oncol. 13 (5), 273–290. doi:10.1038/nrclinonc.2016.65
Krelin, Y., Voronov, E., Dotan, S., Elkabets, M., Reich, E., Fogel, M., et al. (2007). Interleukin-1beta-driven inflammation promotes the development and invasiveness of chemical carcinogen-induced tumors. Cancer Res. 67 (3), 1062–1071. doi:10.1158/0008-5472.CAN-06-2956
Loi, S., Sirtaine, N., Piette, F., Salgado, R., Viale, G., Van Eenoo, F., et al. (2013). Prognostic and predictive value of tumor-infiltrating lymphocytes in a phase III randomized adjuvant breast cancer trial in node-positive breast cancer comparing the addition of docetaxel to doxorubicin with doxorubicin-based chemotherapy: Big 02-98. J. Clin. Oncol. 31 (7), 860–867. doi:10.1200/JCO.2011.41.0902
Lopez-Castejon, G., and Brough, D. (2011). Understanding the mechanism of IL-1β secretion. Cytokine Growth Factor Rev. 22 (4), 189–195. doi:10.1016/j.cytogfr.2011.10.001
Ma, L., Lan, F., Zheng, Z., Xie, F., Wang, L., Liu, W., et al. (2012). Epidermal growth factor (EGF) and interleukin (IL)-1β synergistically promote ERK1/2-mediated invasive breast ductal cancer cell migration and invasion. Mol. cancer 11 (1), 79–11. doi:10.1186/1476-4598-11-79
Mahmoud, S., Lee, A., Paish, E., Macmillan, R., Ellis, I., and Green, A. (2012). Tumour-infiltrating macrophages and clinical outcome in breast cancer. J. Clin. pathology 65 (2), 159–163. doi:10.1136/jclinpath-2011-200355
Malik, A., and Kanneganti, T. D. (2018). Function and regulation of IL-1α in inflammatory diseases and cancer. Immunol. Rev. 281 (1), 124–137. doi:10.1111/imr.12615
Martinon, F., Burns, K., and Tschopp, J. (2002). The inflammasome: A molecular platform triggering activation of inflammatory caspases and processing of proIL-beta. Mol. Cell 10 (2), 417–426. doi:10.1016/s1097-2765(02)00599-3
Maund, S. L., Shi, L., and Cramer, S. D. (2013). A role for interleukin-1 alpha in the 1,25 dihydroxyvitamin D3 response in mammary epithelial cells. PLoS One 8 (11), e81367. doi:10.1371/journal.pone.0081367
Meyer, C., Cagnon, L., Costa-Nunes, C. M., Baumgaertner, P., Montandon, N., Leyvraz, L., et al. (2014). Frequencies of circulating MDSC correlate with clinical outcome of melanoma patients treated with ipilimumab. Cancer Immunol. Immunother. CII 63 (3), 247–257. doi:10.1007/s00262-013-1508-5
Miles, D., Gligorov, J., André, F., Cameron, D., Schneeweiss, A., Barrios, C., et al. (2021). Primary results from IMpassion131, a double-blind, placebo-controlled, randomised phase III trial of first-line paclitaxel with or without atezolizumab for unresectable locally advanced/metastatic triple-negative breast cancer. Ann. Oncol. 32 (8), 994–1004. doi:10.1016/j.annonc.2021.05.801
Mishra, A., Suman, K. H., Nair, N., Majeed, J., and Tripathi, V. (2021). An updated review on the role of the CXCL8-CXCR1/2 axis in the progression and metastasis of breast cancer. Mol. Biol. Rep. 48, 6551–6561. doi:10.1007/s11033-021-06648-8
Naldini, A., Filippi, I., Miglietta, D., Moschetta, M., Giavazzi, R., and Carraro, F. (2010). Interleukin-1β regulates the migratory potential of MDAMB231 breast cancer cells through the hypoxia-inducible factor-1α. Eur. J. Cancer 46 (18), 3400–3408. doi:10.1016/j.ejca.2010.07.044
Noy, R., and Pollard, J. W. (2014). Tumor-associated macrophages: From mechanisms to therapy. Immunity 41 (1), 49–61. doi:10.1016/j.immuni.2014.06.010
Nutter, F., Holen, I., Brown, H. K., Cross, S. S., Evans, C. A., Walker, M., et al. (2014). Different molecular profiles are associated with breast cancer cell homing compared with colonisation of bone: Evidence using a novel bone-seeking cell line. Endocrine-related cancer 21 (2), 327–341. doi:10.1530/ERC-13-0158
O'Shaughnessy, J., Young, R. R., Levin, M. K., Baisch, J., Timis, R., Muniz, L. S., et al. (2016). Safety and immunologic activity of anakinra in HER2-negative metastatic breast cancer (MBC). United States: American Society of Clinical Oncology.
Oh, K., Lee, O. Y., Park, Y., Seo, M. W., and Lee, D. S. (2016). IL-1β induces IL-6 production and increases invasiveness and estrogen-independent growth in a TG2-dependent manner in human breast cancer cells. BMC Cancer 16 (1), 724. doi:10.1186/s12885-016-2746-7
Paz-Ares, L., Goto, Y., Lim, W., Halmos, B., Cho, B., Dols, M. C., et al. (2021). 1194MO canakinumab (CAN) + docetaxel (DTX) for the second- or third-line (2/3L) treatment of advanced non-small cell lung cancer (NSCLC): CANOPY-2 phase III results. Ann. Oncol. 32, S953–S954. doi:10.1016/j.annonc.2021.08.1799
Pruneri, G., Vingiani, A., and Denkert, C. (2018). Tumor infiltrating lymphocytes in early breast cancer. Breast 37, 207–214. doi:10.1016/j.breast.2017.03.010
Ridker, P. M., MacFadyen, J. G., Thuren, T., Everett, B. M., Libby, P., Glynn, R. J., et al. (2017). Effect of interleukin-1β inhibition with canakinumab on incident lung cancer in patients with atherosclerosis: Exploratory results from a randomised, double-blind, placebo-controlled trial. Lancet 390 (10105), 1833–1842. doi:10.1016/S0140-6736(17)32247-X
Savas, P., Salgado, R., Denkert, C., Sotiriou, C., Darcy, P. K., Smyth, M. J., et al. (2016). Clinical relevance of host immunity in breast cancer: From TILs to the clinic. Nat. Rev. Clin. Oncol. 13 (4), 228–241. doi:10.1038/nrclinonc.2015.215
Schedin, P., O'Brien, J., Rudolph, M., Stein, T., and Borges, V. (2007). Microenvironment of the involuting mammary gland mediates mammary cancer progression. J. Mammary Gland. Biol. Neoplasia 12 (1), 71–82. doi:10.1007/s10911-007-9039-3
Schmid, M. C., and Varner, J. A. (2010). Myeloid cells in the tumor microenvironment: Modulation of tumor angiogenesis and tumor inflammation. J. Oncol. 2010, 201026. doi:10.1155/2010/201026
Schmid, P., Adams, S., Rugo, H. S., Schneeweiss, A., Barrios, C. H., Iwata, H., et al. (2018). Atezolizumab and nab-paclitaxel in advanced triple-negative breast cancer. N. Engl. J. Med. 379 (22), 2108–2121. doi:10.1056/NEJMoa1809615
Schmid, P., Cortes, J., Dent, R., Pusztai, L., McArthur, H., Kummel, S., et al. (2022). Event-free survival with pembrolizumab in early triple-negative breast cancer. N. Engl. J. Med. 386 (6), 556–567. doi:10.1056/NEJMoa2112651
Schmid, P., Cortes, J., Pusztai, L., McArthur, H., Kummel, S., Bergh, J., et al. (2020). Pembrolizumab for early triple-negative breast cancer. N. Engl. J. Med. 382 (9), 810–821. doi:10.1056/NEJMoa1910549
Schrodi, S., Braun, M., Andrulat, A., Harbeck, N., Mahner, S., Kiechle, M., et al. (2021). Outcome of breast cancer patients with low hormone receptor positivity: Analysis of a 15-year population-based cohort. Ann. Oncol. 32 (11), 1410–1424. doi:10.1016/j.annonc.2021.08.1988
Shen, Q., Cohen, B., Zheng, W., Rahbar, R., Martin, B., Murakami, K., et al. (2017). Notch shapes the innate immunophenotype in breast cancer. Cancer Discov. 7 (11), 1320–1335. doi:10.1158/2159-8290.CD-17-0037
Soria, G., Ofri-Shahak, M., Haas, I., Yaal-Hahoshen, N., Leider-Trejo, L., Leibovich-Rivkin, T., et al. (2011). Inflammatory mediators in breast cancer: Coordinated expression of TNFα & IL1β with CCL2 & CCL5 and effects on epithelial-to-mesenchymal transition. BMC cancer 11 (1), 130. doi:10.1186/1471-2407-11-130
Stanton, S. E., Adams, S., and Disis, M. L. (2016). Variation in the incidence and magnitude of tumor-infiltrating lymphocytes in breast cancer subtypes: A systematic review. JAMA Oncol. 2 (10), 1354–1360. doi:10.1001/jamaoncol.2016.1061
Storr, S. J., Safuan, S., Ahmad, N., El-Refaee, M., Jackson, A. M., and Martin, S. G. (2017). Macrophage-derived interleukin-1beta promotes human breast cancer cell migration and lymphatic adhesion in vitro. Cancer Immunol. Immunother. 66, 1287–1294. doi:10.1007/s00262-017-2020-0
Sung, H., Ferlay, J., Siegel, R. L., Laversanne, M., Soerjomataram, I., Jemal, A., et al. (2021). Global cancer statistics 2020: GLOBOCAN estimates of incidence and mortality worldwide for 36 cancers in 185 countries. CA a cancer J. Clin. 71 (3), 209–249. doi:10.3322/caac.21660
Swanton, C., Hill, W., Lim, E., Lee, C., Weeden, C., Augustine, M., et al. (2022). LBA1 Mechanism of action and an actionable inflammatory axis for air pollution induced non-small cell lung cancer: Towards molecular cancer prevention. Ann. Oncol. 33, S1413. doi:10.1016/j.annonc.2022.08.046
Thornberry, N. A., Bull, H. G., Calaycay, J. R., Chapman, K. T., Howard, A. D., Kostura, M. J., et al. (1992). A novel heterodimeric cysteine protease is required for interleukin-1 beta processing in monocytes. Nature 356 (6372), 768–774. doi:10.1038/356768a0
Tu, S., Bhagat, G., Cui, G., Takaishi, S., Kurt-Jones, E. A., Rickman, B., et al. (2008). Overexpression of interleukin-1beta induces gastric inflammation and cancer and mobilizes myeloid-derived suppressor cells in mice. Cancer Cell 14 (5), 408–419. doi:10.1016/j.ccr.2008.10.011
Voronov, E., Carmi, Y., and Apte, R. N. (2014). The role IL-1 in tumor-mediated angiogenesis. Front. physiology 5, 114. doi:10.3389/fphys.2014.00114
Keywords: triple negative breast cancer, IL1beta, immunotherapy, tumor microenvironment, inflammasome
Citation: Wilson BE, Shen Q, Cescon DW and Reedijk M (2023) Exploring immune interactions in triple negative breast cancer: IL-1β inhibition and its therapeutic potential. Front. Genet. 14:1086163. doi: 10.3389/fgene.2023.1086163
Received: 01 November 2022; Accepted: 13 March 2023;
Published: 29 March 2023.
Edited by:
Changgang Sun, Affiliated Hospital of Weifang Medical University, ChinaReviewed by:
Pranabananda Dutta, Charles R. Drew University of Medicine and Science, United StatesZhen Wang, Zhejiang University, China
Copyright © 2023 Wilson, Shen, Cescon and Reedijk. This is an open-access article distributed under the terms of the Creative Commons Attribution License (CC BY). The use, distribution or reproduction in other forums is permitted, provided the original author(s) and the copyright owner(s) are credited and that the original publication in this journal is cited, in accordance with accepted academic practice. No use, distribution or reproduction is permitted which does not comply with these terms.
*Correspondence: Michael Reedijk, bWljaGFlbC5yZWVkaWprQHVobi5jYQ==