- 1Centre for Genetic Disorders, Institute of Science, Banaras Hindu University, Varanasi, India
- 2Centre for Biomedical Education, Cell Biology and Genetics Research Centre, St. George’s University of London, London, United Kingdom
Prostate cancer is the second most common male cancer worldwide showing the highest rates of incidence in Western Europe. Although the measurement of serum prostate-specific antigen levels is the current gold standard in PCa diagnosis, PSA-based screening is not considered a reliable diagnosis and prognosis tool due to its lower sensitivity and poor predictive score which lead to a 22%–43% overdiagnosis, unnecessary biopsies, and over-treatment. These major limitations along with the heterogeneous nature of the disease have made PCa a very unappreciative subject for diagnostics, resulting in poor patient management; thus, it urges to identify and validate new reliable PCa biomarkers that can provide accurate information in regard to disease diagnosis and prognosis. Researchers have explored the analysis of microRNAs (miRNAs), messenger RNAs (mRNAs), small proteins, genomic rearrangements, and gene expression in body fluids and non-solid tissues in search of lesser invasive yet efficient PCa biomarkers. Although the presence of miRNAs in body fluids like blood, urine, and saliva initially sparked great interest among the scientific community; their potential use as liquid biopsy biomarkers in PCa is still at a very nascent stage with respect to other well-established diagnostics and prognosis tools. Up to date, numerous studies have been conducted in search of PCa miRNA-based biomarkers in whole blood or blood serum; however, only a few studies have investigated their presence in urine samples of which less than two tens involve the detection of miRNAs in extracellular vesicles isolated from urine. In addition, there exists some discrepancy around the identification of miRNAs in PCa urine samples due to the diversity of the urine fractions that can be targeted for analysis such as urine circulating cells, cell-free fractions, and exosomes. In this review, we aim to discuss research output from the most recent studies involving the analysis of urinary EVs for the identification of miRNA-based PCa-specific biomarkers.
1 Introduction
Prostate cancer (PCa) is the most common type of male cancer and one of the leading causes of male cancer mortality worldwide (Roehrborn and Black, 2011; Siegel et al., 2021). Advances in clinical screening and early detection of the disease through the detection of prostate-specific antigen (PSA) have resulted in a 90% 5-year survival rate; yet, increase the risk for a man to face a diagnosis of PCa even when the tumor is benign or low-risk (Martin et al., 2018). The United States Preventive Services Task Force has also advised against PSA screening due to its inability to distinguish high-risk PCa and to the high numbers of false positive results that this test provides (Fenton et al., 2018). Therefore, it is necessary to identify PCa-specific biomarkers that allow not only for an early and accurate disease diagnosis but also for tumor stratification in order to provide the best patient clinical management.
1.1 Current status of diagnostics in PCa and the need for new biomarkers
PCa diagnosis currently relies on digital rectal examination (DRE) and blood PSA levels followed by a solid biopsy to confirm and characterize the malignancy. DRE sensitivity and specificity are reported to be 80% and 48.6% respectively which makes it dependent on validation via solid biopsy upon palpation and detection of irregularities in the prostate nodules (Irekpita et al., 2020). Although DRE provides significantly higher success rates in detecting high-grade PCa cases when compared to PSA levels, it still shows a limited overall predictive value and high invasiveness (Jones et al., 2018).
In spite of the recent major developments in biochemical tests and imaging techniques, the primary biochemical tool for PCa diagnosis is serum PSA levels. PSA-based screening is a non-invasive and painless technique that has contributed to reducing the incidence of advanced disease and mortality improving overall PCa diagnosis and prognosis (Martin et al., 2018). However, it is worth mentioning that PSA reliability as a sole biomarker thus remains controversial due to the high rate of false-positive results and low specificity (Fenton et al., 2018).
PSA is a prostate-specific serine protease produced by the prostate epithelium whose function is to liquefy the seminal coagulum allowing sperm to swim freely (Balk et al., 2003). In normal health conditions, epithelial cells release PSA to the seminal fluid and only a small amount escapes into blood circulation through an unknown molecular mechanism (Stenman et al., 1999). Apart from cancer, other pathological conditions such as benign prostatic hyperplasia (BPH) and prostatitis are normally associated with increased PSA levels in the blood (Gao et al., 2019). On this note, the European Randomized Study of Screening for Prostate Cancer has reported that 22%–43% of the PSA-detected malignancies are overdiagnosed (Hugosson et al., 2019) which leads to unnecessary biopsies and over-treatment. In the absence of alternative biochemical PCa biomarkers, the majority of PCa screening guidelines still advise the measurement of total serum PSA (tumor (t)PSA>4 ng/mL) as a benchmark marker for the detection of PCa. However, in the last few years novel PCa detection assays, such as the 4K score and Prostate Health Index (PHI) which integrate PSA with other biomarkers (Fossati et al., 2015; Parekh et al., 2015), have emerged with the aim of reducing the rates of false positives and the number of unnecessary biopsies (Table 1). Nevertheless, these approaches still involve the validation of the results through the extraction of solid biopsies (Voigt et al., 2017).
In the last few years, biopsy-based approaches have been notably improved and highlighting guided biopsy techniques such as Transrectal ultrasound scan (TRUS)-guided biopsy, which have been developed with the goal of reducing procedural mistakes (Hodge et al., 1989). Despite the significant technological advances, investigations so far have demonstrated that guided biopsy still exhibits a significant prevalence of false negatives and tumor under grading due to imprecise positioning of the biopsy needle when compared to radical prostatectomy-informed grading (Wolters et al., 2010).
Thus, there is a clear need for identifying more accurate and reliable, and less invasive PCa biomarkers that overcome the limitations associated with PSA tests and guided biopsy techniques. Additionally, new biomarkers should ideally help improve treatment decision-making through more accurate detection of minimal residual disease and a more efficient clinical staging of the disease taking into account the complexity and heterogeneous nature of PCa at the different stages (i.e., localized tumor, metastatic, and hormone-independent recurrence of PCa). Data so far suggest that novel biomarkers should not be considered as mutually exclusive parameters; instead, their effectiveness should be assessed in conjunction with the current approaches and decision-making algorithms (Fossati et al., 2015; Parekh et al., 2015).
1.2 A new generation of diagnostic biomarkers in PCa
Over the last few decades, a new generation of PCa prognostics and diagnostics tests have emerged. Each test combines several parameters and biomarkers and provides a specific utility and timing of usage in line with the disease stages; for instance, PHI and 4K-score tests are used for diagnostics purposes (Fossati et al., 2015; Parekh et al., 2015), Oncotype DX is applied in risk identification (Hessels et al., 2003; Covas Moschovas et al., 2022), and Polaris and Decipher are used for active monitoring and management of PCa cases (Banerjee and Punnen, 2022). In Table 1 we can observe a summary of the currently available PCa diagnostic tests, apart from PSA and histopathological analysis, used in patients with suspected or proven PCa using molecular markers derived from solid tissue, blood, or urine.
The list of commercialized tests stated in Table 1 shows that mRNAs (PCA3, ExoDx, SelectMDx) and protein expression (PHI and 4K Score) analyzed in liquid biopsies such as blood serum and urine are being extensively used as potential alternatives to DRE, PSA, and solid biopsies. The detection of miRNAs in liquid biopsies for PCa diagnostic, prognosis, and staging in it is still in the early research stage; up to date, there are no commercialized diagnostic kits that involve the detection of miRNAs in PCa or any other type of cancer disease.
1.3 Urine components used for the identification of biomarkers
Urine is considered a rich source of biomarkers for malignancies related to the urinary tract; since it contains cellular debris, proteins from glomerular filtration, and secretions of the urogenital tract, it can reflect a person’s real-time pathophysiology of a disease. For instance, expressed prostatic secretion (EPS) is a fluid released by the prostate to the urine through the urethra, following a digital rectal prostate massage (Drake et al., 2009); it is rich in proteins and metabolites that could provide meaningful information on prostate health status (Drago et al., 2021). However, different urine fractions has been targeted for the identification of new biomarkers like miRNA (e.g., urinary circulating cells, cell-free DNA, and EV) and this, together with the variations in urine collection times, sample sizes, miRNA isolation protocols, the number of miRNAs analyzed, and the internal control employed, is responsible for the discrepancies noted in the PCa-related urine miRNAs reported in the literature to date.
1.4 EV miRNAs as biomarkers
EVs are lipid-bound vesicles that originate from the endosomal system or bud off from the plasma membrane and that contain miRNAs that are transported to target cells for intercellular communication. Prostate cell-derived EVs can be released into the urine through the urethra where they are detected by simple non-invasive techniques. EVs include exosomes (30–100 nm), prostasomes (50–500 nm), estosomes (50–1,000 nm), oncosomes (50–500 nm) and microvesicles (100–1,000 nm) (Drake et al., 2009). It has been demonstrated that EVs mediate cell-cell communication by carrying active genetic material such as miRNAs and mRNAs (Akers et al., 2013; Maia et al., 2018). Since miRNAs are highly stable inside the EVs, easily available body fluids such as urine, blood, semen, and breast milk, have been investigated for the detection and analysis of extravesicular miRNAs that can inform about healthy and pathological conditions (Lässer, 2013; Gámez-Valero et al., 2015; Groot and Lee, 2020). Given that cancer cells are reported to abundantly shed EVs (Brassart et al., 2019), it is possible to detect tumor miRNAs within EVs that inform cancer cell activity (Lässer, 2013; Cheng et al., 2014).
However, one of the main limitations of working with extracellular miRNAs is the restricted available published data on the optimal conditions for their isolation and analysis and even more limited in regard to prostate cell-derived extravesicular miRNAs.
Thus, we aim here to review the PCa-specific studies involving the detection of urine-derived EVs-associated miRNAs in order to develop a reliable miRNA panel that can provide information about disease diagnosis, stage, and progression.
2 Materials and methods
2.1 Literature search
Using the electronic database PubMed, we performed a review of studies published before July 2022 that involved the analysis of urine-derived EV-miRNAs in PCa diagnosis. Potentially relevant studies were identified using the PubMed string search and Boolean operators: (“urine”[Subheading] OR “urine”[Text Word] OR “urine”[MeSH Terms]) OR (“urinary tract”[MeSH Terms] OR “urinary tract”[Text Word]) AND (“microRNAs”[MeSH Terms] OR “microRNAs” [Text Word] OR “mirna”[Text Word]) AND (“prostatic neoplasms”[MeSH Terms] OR “prostate cancer”[Text Word]); AND (“exosome”[MeSH Terms] OR “microvesicles”[Text Word] OR “extracellular vesicles”[Text Word]).
2.2 Data extraction
Only the articles that fulfilled all the eligibility criteria, as below, were selected:
(1) Research articles that presented the diagnostic potential of urinary miRNAs in PCa (Siegel et al., 2021); studies that used only uEV (exosomes, microvesicles) as sources to identify miRNAs (Martin et al., 2018), studies that showed data related to the detection of miRNAs focused only in urine or in combination with tissue, serum, plasma, or cell line. Exclusion criteria were as follows (Roehrborn and Black, 2011): case reports, editorials, reviews, duplication of selected articles, and retracted articles (Siegel et al., 2021); studies that report methods and/or protocols.
We excluded non-English studies, studies using miRNAs from cell-free urine fractions, and studies dated before 2007.
The following information was collected from the selected articles and tabulated in Tables 2, 3 (Roehrborn and Black, 2011). Authors’ name, and publication year (Siegel et al., 2021); type of study design—case-control or cohort (Martin et al., 2018); sample size—number of benign, PCa, and healthy controls (Fenton et al., 2018); the volume of urine used for extraction and method of EV isolation (Irekpita et al., 2020); urine collection time point (pre- or post-DRE or DRE not considered) (Jones et al., 2018); method of miRNAs shortlisting for validation, type of RNA extraction (Balk et al., 2003); normalization of miRNA quantification (Stenman et al., 1999); differentially expressed miRNA reported (Gao et al., 2019); identified miRNA after validation, and (Hugosson et al., 2019) it is predictive value as compared to PSA (Tables 2, 3).
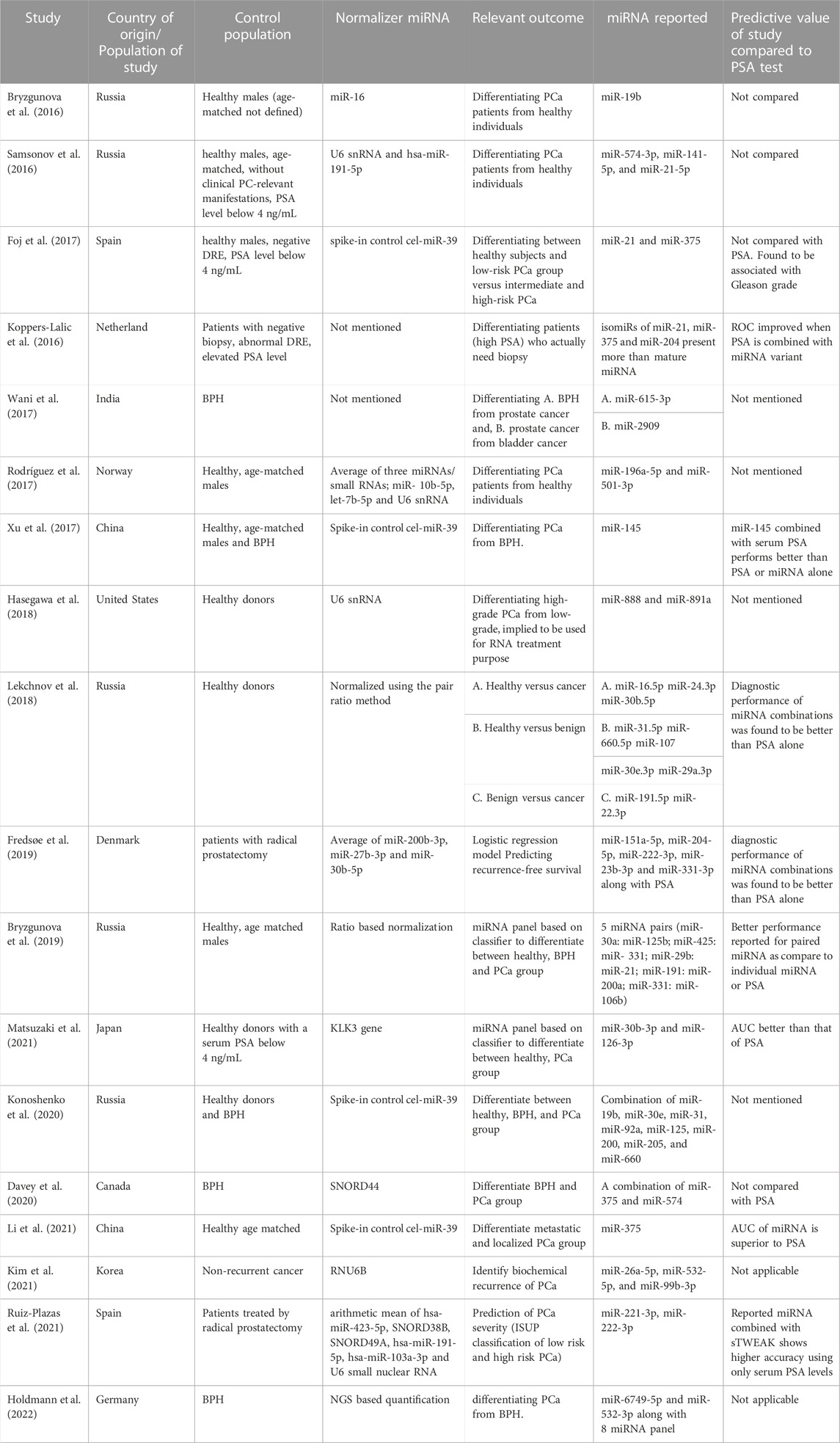
TABLE 2. Summary of relevant studies and urinary-extracellular vesicles miRNAs that they have proposed as potential biomarkers for PCa diagnosis, prognosis and risk classification.
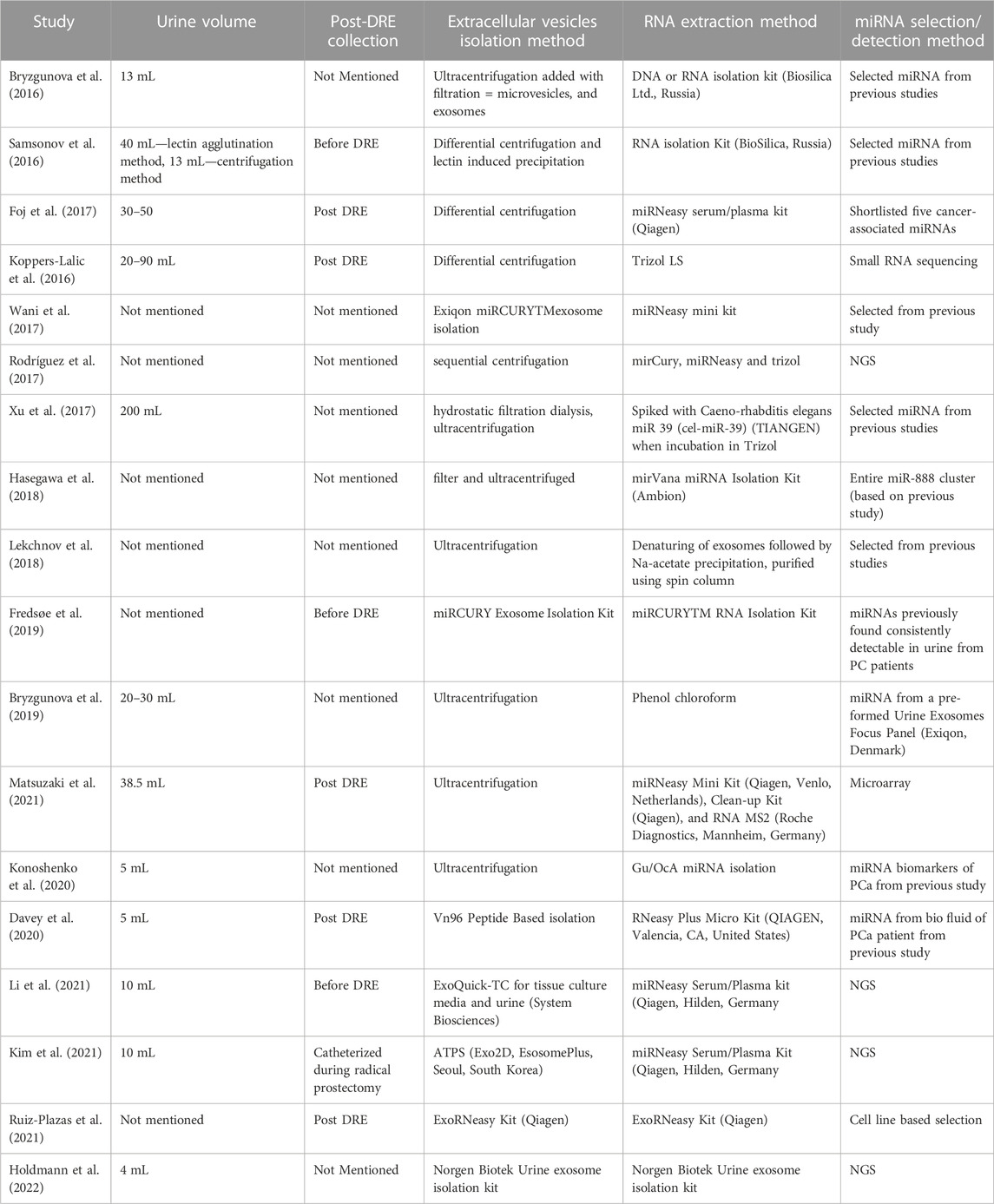
TABLE 3. Summary of studies involving the analysis of urinary-extracellular vesicle (EV) miRNAs that have been analyzed in this review including information on the urine volume used for the isolation of EV, the isolation method, the method used for EV miRNA extraction, and the approach followed to select the miRNAs of study.
2.3 Identification of prostate-specific miRNA-target gene
TargetScan and miRDB were used to extract the list of genes that are the most commonly reported as target genes for the particular miRNAs. Only the target genes with a score of >95 were taken into account. Using the term “Prostate cancer” in the gene-cards database, a different list of 12,400 genes related to prostate biology was retrieved. Items in both lists were then compared to find common elements, and the results were plotted in a Venn Diagram using Venny2.1 (Supplementary Material).
3 Results
3.1 Summary of studies included
Following the inclusion criteria, a total of 74 primary articles were found in Pubmed from which 38 were discarded as they were duplicated studies, methodology studies, or reviews. After applying the exclusion criteria, a final number of 18 worldwide studies were selected for this review (Tables 2, 3). Seventeen of the study designs were case-control. The sample size ranged between 14 and 619 per study with a total of 1750 samples analyzed overall. The volume of the urine sample analyzed across studies ranged from 5 to 90 mL.
3.2 Documentation of findings
We notice a considerable degree of inconsistency in the identified biomarkers if we solely concentrate on research that involves uEVs-related miRNAs. Interestingly, not only the differential expression patterns but also the differences in the miRNA distribution pattern, and the existence of miRNA isomeric forms have been crucial parameters for the identification of new PCa biomarkers. Outcomes of the studies that investigated miRNA levels in uEVs included but were not limited to, early diagnosis, differentiating BPH from cancer, predicting biochemical recurrence, and anti-miRNA-based treatment.
The miRNAs that were assessed and identified as relevant biomarkers varied widely (Table 2). Since miRNAs have a variety of functions and strongly-correlated target genes, it would be more logical to evaluate frequently reported PCa biomarker miRNAs based on their prostate-specific target genes and functions; thus we have focused on the target gene evaluation of a total of eight miRNAs that were reported in more than one of the studies included in this review: miR-19b, miR–21-5p, miR-141-5p, miR-375, miR-331-3p, miR-10b-5p, miR-204, and miR-30a/30b (Bryzgunova et al., 2016; Koppers-Lalic et al., 2016; Samsonov et al., 2016; Foj et al., 2017; Bryzgunova et al., 2019; Fredsøe et al., 2019; Matsuzaki et al., 2021). All the eight selected miRNAs, except miR-10b-5p, showed >50% of target genes as PCa-associated genes (Supplementary Data; Table 4).
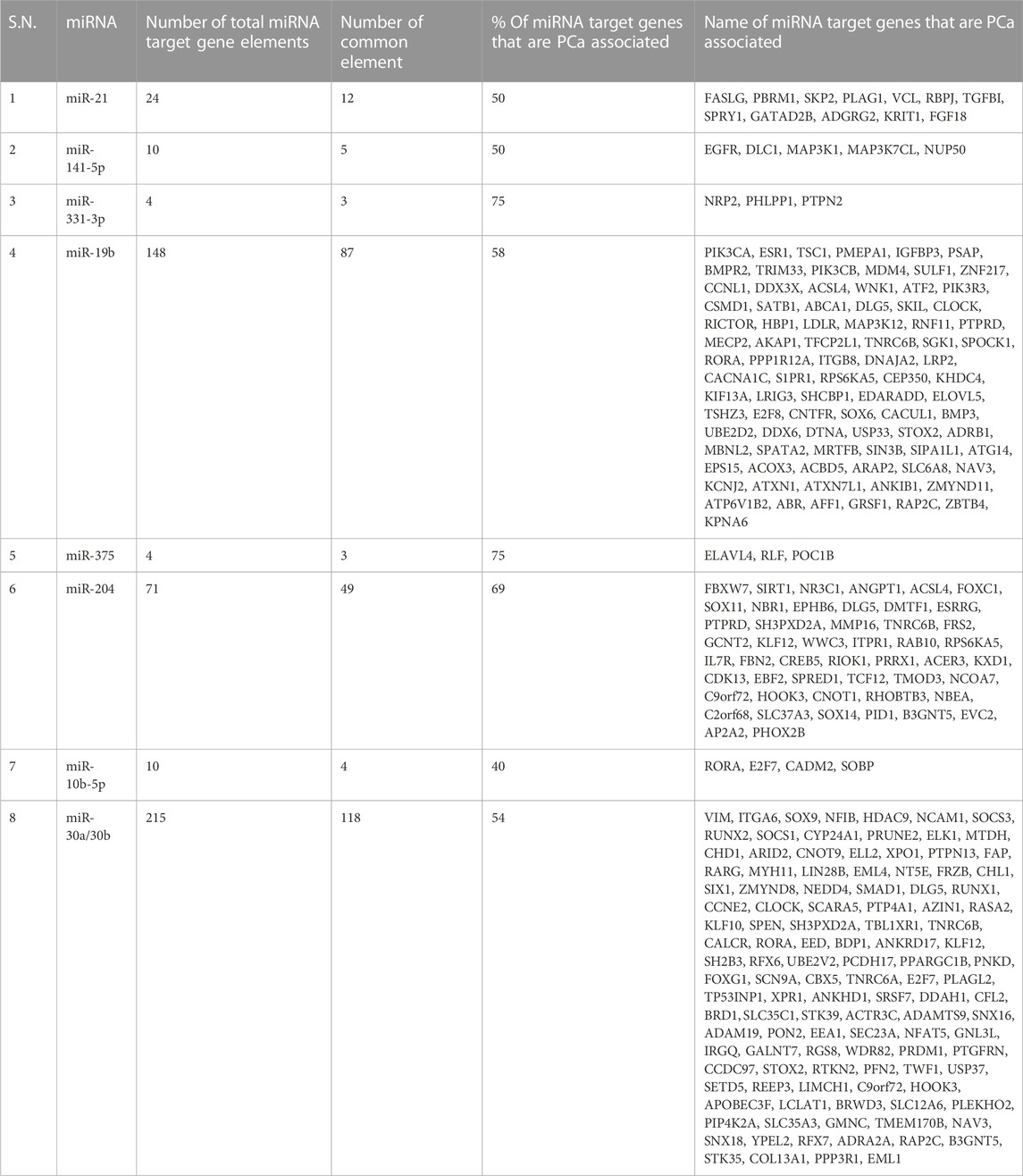
TABLE 4. Most frequently reported dysregulated miRNAs in urinary exosomes of prostate cancer patients. The list of targeted genes for each miRNA was retrieved using miRDB and TargetScan. Only the target genes with a score of >95 were considered in the next step. Another list of prostate-associated genes was retrieved using the keyword “Prostate cancer” in gene cards, providing the output of around 12,400 genes. Elements on both lists were then compared to retrieve common elements using Venny2.1 software and the output result of the common element was collected in the form of a Venn Diagram and list.
4 Discussion
4.1 Discussion on potential PCa miRNAs biomarkers based on their prostate-specific target-genes
4.1.1 miR-21-5p
Elevated expression of miR-21 has been reported in PCa tissue (Damodaran et al., 2021), plasma (Bryant et al., 2012), and urine (Samsonov et al., 2016). miR-21 is known to regulate the proliferation and apoptosis of cancer cells through the PTEN/PI3K/AKT pathway which makes it a good potential novel target for anti-cancer therapies (Liu et al., 2019). In Samsonov et al. (2016) the analysis of PCa–related miRNAs using lectin-induced agglutination method for isolation of in urinary-exosomes revealed significant upregulation of miR-21-5p in PCa patients with PSA ranging from 10 to 20 as compared to healthy control. Although the study reported a notable diagnostic significance of miR-21-5p along with miRNA-574-3p and miR-141-5p, the sensitivity of miR-21-5p (area under the curve (AUC) and receiver operating characteristic curve (ROC) 0.65; 95%, CI = 0.477–0.814, sensitivity = 0.46, p < 0.05) was the lowest among the three. Another RNAseq study performed by Koppers-Lalic et al. (2016) on PCa patients (PSA = 8.5–9.5) observed a significant upregulation of miR-21 expression (p = 0.02) along with miR-375, and miR-204 as compared to healthy controlKoppers-Lalic et al. (2016). When the expression of these miRNAs was validated by RT-qPCR, the results regarding miR-21 were not significant; however, further studies showed later that those differences were associated with the presence of different miR-21-5p isomeric forms. This study proposed that the detection of isomiRs in combination with PSA levels could significantly increase the probability of detecting PCa as compared to mature miRNA or PSA alone (Koppers-Lalic et al., 2016).
Many studies have proposed the establishment and analysis of batteries of miRNAs, instead of individual miRNA, in order to achieve higher sensitivity and specificity in the diagnosis and stratification of PCa patients (Bryzgunova et al., 2019; Fredsøe et al., 2019; Davey et al., 2020). For instance, a study conducted by Bryzgunova et al. (2019) in uEVs highlighted the relevance of miR-21-5p in the discrimination of PCa and BPH patients when they applied an algorithm-based classifier, which analyzed the expression of five different pairs of diagnostically significant miRNAs: miR-21-5p+miR-29b, miR-30a+miR-125b, miR-425+miR-331, miR-191+miR-200a and miR-331+miR-106b.
To corroborate the association of miR-21-5p with PCa, in this review we have cross-checked the group of miR-21-5p target genes with the PCa-associated genes retrieved from GeneCards, resulting in a list of common genes shown in Table 4. The top 12 miR-21-5p target genes showing a score >95 are PCa-specific genes. This result, together with the aforementioned findings, highlights the relevant role of miR-21-5p in urine-based PCa diagnosis and underlines the necessity of conducting functional studies that help us identify the efficacy and suitability of miR-21-5 and its isomiR, alone or in combination with PSA or other miRNAs, in PCa diagnosis.
4.1.2 miR-141-5p
miR-141 is reported to affect angiogenesis, proliferation, and metastasis in colon cancer and nasopharyngeal carcinoma (Dong et al., 2019; Tong et al., 2021). Although its role in PCa is yet to be defined, studies have reported miR-141 to be commonly present in the blood of PCa patients (Yaman Agaoglu et al., 2011). In regard to studies performed in urine samples, Xu et al. (2017) did not find any significant differences in the expression of uEV miR-141-5p between BPH and PCa but its presence in urinary cells of PCa patients was observed to be significantly higher in the disease group. In contrast, other authors like Samsonov et al. (2016) found upregulated expression of uEV miR-141-5p in PCa patients when compared with healthy donors.
When we cross-checked the miRNA-141-5p target genes with the PCa-specific genes, we observed that the top five common genes are mainly involved in cell survival which does not shed enough light on the role of this miRNA in PCa disease progression (Supplementary Data; Table 4).
To summarize, the role of miR-41-5p as a PCa-specific biomarker remains questionable due to the low statistical significance of the results obtained in the referred studies; research involving larger case-control cohorts is required to obtain concluding results.
4.1.3 miR-375-3p
There exist contradicting findings in regard to this miRNA. Published data shows increased miR-375 levels in the blood of PCa patients with metastatic disease (Bhagirath et al., 2020). Additionally, a Next-Generation Sequencing (NGS)-based investigation revealed that miR-375-3p is significantly downregulated in PCa patients and combination of miR-375 and miR-451a has the potential to serve as a specific and sensitive molecular marker to differentiate PCa patients from BPH patients, and that miR-375 alone can distinguish localized PCa patients from metastatic PCa (Li et al., 2021). However, there is still disagreement about the putative discriminating function of miR-375 in PCa. In a study conducted by Foj et al. (2017), it was demonstrated that both miR-375 and miR-21 are significantly upregulated in intermediate and high risk PCa patients as copare to low risk and healthy population and could discriminate between PCa and non-PCa participants that had shown high PSA levels. Although, another study observed downregulation of miR-375-5p in cancer cases with NGS data from uEVs, the mature versions of miR-375, -141, and -21 cannot effectively distinguish between PCa and healthy individuals unlike their isomeric forms (Koppers-Lalic et al., 2016). In summary, the presence of miR-375 in uEVs has notably been observed in studies, but the significance of mature miR-375 as an overexpressed biomarker is debatable.
4.1.4 miR-10b-5p
NGS of uEVs has revealed miR-10b-5p and other miRNAs such as let-7b-5p, miR-30a-5p, miR-10a-5p, and let-7a-5p, as the most abundant in urinary exosomes (Cheng et al., 2014; Koppers-Lalic et al., 2016; Rodríguez et al., 2017). Their presence does not seem to be associated with the disease state and, although further investigation is required, it has been suggested that these miRNAs could be applied as uEVs-specific normalizers. In fact, one of the studies used an average of three miRNAs/small RNAs for normalization (miR-10b-5p, let-7b-5p, and U6 snRNA) as miR-10b-5p and let-7b-5p were expressed similarly in the control and patient groups according to the NGS study (Rodríguez et al., 2017).
4.1.5 miR-331-3p
miR-331-3p has been reported to affect long-term survival in hepatocellular cancer (Chang et al., 2014). It is also a mediator in the epithelial-to-mesenchymal transition in PCa (Fujii et al., 2016) which has led to its consideration as PCa prognostic marker as it is also supported by its identified target genes in Table 4.
miR-331-3p has already been included in large cohort-based case-control studies as part of a predictor panel for biochemical recurrence and aggressive PCa risk (Fredsøe et al., 2019). Also, one study involving the use of RT-qPCR to quantify miRNAs in urine supernatant observed that miR-331-3p together with miR-92a allowed the differentiation of BPH and PCa cases from healthy controls with a 52% sensitivity (p = 0.025) (Bryzgunova et al., 2019). The same study also analyzed the expression values of miR-331-3p in uEVs which were paired up with miR-425; in that case, the algorithm could differentiate PCa from healthy and BPH cases with a 40% sensitivity (p = 0.002). The combination of miR331-3p and miR-106b in urine supernatant and microvesicles was also evaluated and could differentiate PCa from healthy and BPH cases with a 30% sensitivity (p = 0.036). This study concluded that a five-miRNA pairs panel could be used to develop an expression-based algorithm that allows us to classify PCa, BPH, and healthy with 100% specificity and 97.5% accuracy (Martin et al., 2018).
4.1.6 miR-19b
The presence of miR-19b in uEVs has been proposed as an auxiliary PCa differentiating criterion with a 100% specificity and a 93% sensitivity (Bryzgunova et al., 2016). High levels of this miRNA have been found in both blood serum of PCa patients and mice prostate tumors tissue which supports a potential diagnostic and a prognostic value (Duca et al., 2021). One study conducted by Konoshenko et al. (2020) included miR-19b in a battery of twelve miRNAs that were investigated in 31 combination ratios. Eight of those miRNAs in six ratios, including miR-19b/miR-92a in uEVs could discriminate PCa, BPH, and healthy donors with 100% specificity, and 100% sensitivity. Overall, studies so far suggest a key role of miR-19b, alone or in combination with other miRNAs, in the differentiation of healthy vs. PCa as well as BPH vs. PCa. Moreover, in this review, miR-19b has shown the highest number of prostate-associated target genes with a total of 84 genes (Table 4).
4.1.7 miR-204
miR-204 is known to be an NF-kB pathway inhibitor and therefore hinders various tumor progression-related phenomena including metastasis (Todorova et al., 2016; Wa et al., 2019). The relevance of miR-204 in PCa has been confirmed in studies using RT-qPCR (Fredsøe et al., 2019) and NGS (Koppers-Lalic et al., 2016). Fredsøe et al. (2019) performed a novel logistic regression model comprising PSA and five urine miRNAs, one of them miR-204, which could significantly predict biochemical recurrence and clinical risk stratification of PCa. But further research on this miRNA has demonstrated that not only the copy number variation of miR-204 in uEVs but also the presence of its different isoforms (isomiRs) with 3′-end modifications have the ability to discriminate PCa cases from the healthy subjects. In this sense, a study conducted by Koppers-Lalic et al. (2016) observed that standard mapping protocols involving expression levels of matured miRNAs failed to robustly discriminate disease status whereas isomiRs of miR-21, miR-204, and miR-375 could detect PCa with 72.9% sensitivity and 88% specificity, with an AUC of 0.866.
4.1.8 miR-30a/30b
The miRNA-30 (miR-30) family is a group of tumor suppressor miRNAs consisting of six mature miRNA molecules (miR-30a, miR-30b, miR-30c-1, miR-30c-2, miR-30d, and miR-30e) (Mao et al., 2018). miR-30a has been frequently reported to be downregulated in PCa and its expression inhibits androgen-independent growth in PCa (Zhao et al., 2019). In this sense, deep sequencing analysis conducted by Rodríguez et al. (2017) described miR-30a-5p as one of the most abundant miRNAs in uEVs slightly, but not significantly, downregulated in PCa cases compared to healthy males. In the aforementioned study involving the application of a five-miRNA pair-based algorithm, miR-30a was one of the miRNAs that could discriminate between PCa and BPH patients and healthy samples with 100% specificity and 97.5% accuracy (Bryzgunova et al., 2019). Since miR-30b is also among the most stably expressed miRNAs in uEVs along with miR-200b-3p, and miR-27b-3p, it has been used as an endogenous control in RT-qPCR experiments in some studies (Fredsøe et al., 2019) which questions its validity as PCa biomarker. Nevertheless, in another study, miR-30b-3p along with miR-126-3p was observed to be overexpressed in uEVs of PCa patients as compared to subjects showing cancer-negative biopsy results. This study proposed a PSA and age-adjusted logistic regression analysis for the prediction of PCa with 46.4% sensitivity and 88.0% specificity (Matsuzaki et al., 2021). miR30b along with miR-30e also showed up as members of the most diagnostically significant miRNA pairs in an algorithm created to differentiate PCa patients from a healthy population. This study used median ∆Ct difference, and confidence interval as statistical criteria for determining reliable markers of PCa (Lekchnov et al., 2018).
Finally, there are other miRNAs that have been reported to show significantly low expression levels in PCa compared to a healthy population and these are miR-196a-5p, miR-34a-5p, miR-143-3p, miR-501-3p, and miR-92a-1-5p in uEVs (Rodríguez et al., 2017). According to one study, miR-2909 can be used to monitor PCa aggression (Wani et al., 2017). Another study observed elevated levels of miR-888 cluster in patients with high-grade prostate cancer as compared to lower grades of the disease (Hasegawa et al., 2018). Three miRNAs from uEVS - miR-26a-5p, miR-532-5p, and miR-99b-3p, are suggested to be used in the prediction of biochemical recurrence of PCa (Kim et al., 2021). Using a machine learning method, the two miRNAs hsa-miR-532-3p and hsa-miR-6749-5p could distinguish BPH patients from those with PCa with 80% specificity and 66.7% sensitivity (Holdmann et al., 2022). miR-423-5p and miR-193-3p were downregulated in uEVS of high-risk patients’ post-digital rectal examination but their diagnostic significance is not known (Ruiz-Plazas et al., 2021).
4.2 Limitations and potential cofounders
The observations reported for the majority of the aforementioned uEV miRNAs show inconsistent or contradictory results despite the fact that few of them are common to the 18 studies included in this review: miR-21-5p, miR-375, miR-125, miR-19b, miR-191, miR-200, miR-30b, miR-574-3p (Table 5). The origin of this inconsistency could reside in the lack of consensus in the volume of urine used for the study, in the heterogeneity of methodology applied for the isolation of both uEVs and miRNAs, and in the purification of these nucleic acids (Table 2); nonetheless, we have also observed high variability in other aspects related to the selection of subjects and miRNAs for study and analysis of results.
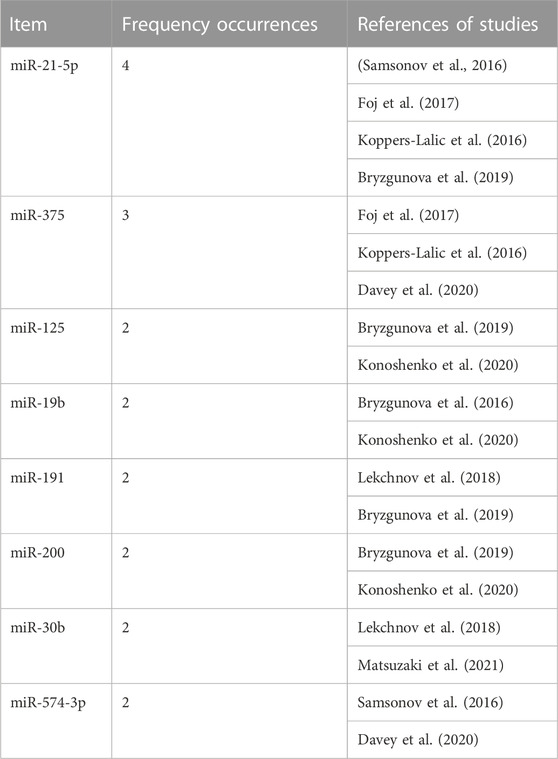
TABLE 5. Proposed miRNAs panel with potential use for the discrimination between control or bening prostatic hyperplasia populations and prostate cancer patients. Using each miRNA as an array element, the table shows the reporting frequency for each miRNA and the studies where they were reported.
Next, we will expand on these study limitations and potential cofounders that may be influencing the associations observed between the miRNAs and PCa diagnosis, prognosis, and stratification.
4.2.1 Sample-selection cofounders
We believe that the disparity in the results could be influenced by the variations in the selected control groups; whilst ten of the studies included in this review involved healthy donors as controls (Bryant et al., 2012; Cheng et al., 2014; Bryzgunova et al., 2016; Fujii et al., 2016; Samsonov et al., 2016; Wani et al., 2017; Bryzgunova et al., 2019; Dong et al., 2019; Fredsøe et al., 2019; Liu et al., 2019), three studies compared PCa with BPH samples (Foj et al., 2017; Damodaran et al., 2021; Kim et al., 2021), two studies looked at both BPH and healthy controls (Koppers-Lalic et al., 2016; Davey et al., 2020), and one study used non-recurrent PCa as control (Hasegawa et al., 2018) (Figure 1; Table 3).
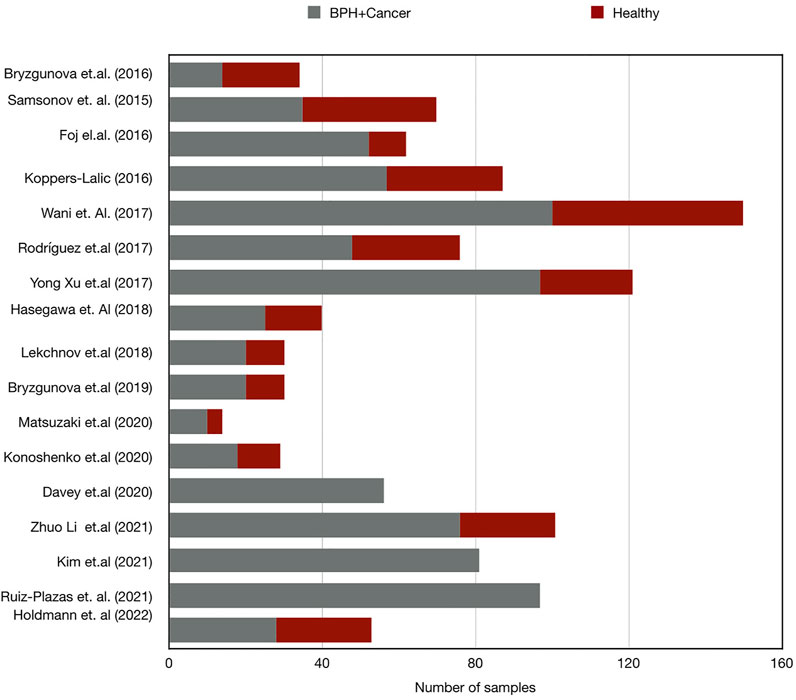
FIGURE 1. Bar chart demonstrating the number of samples in case and control group sets in miRNA research in PCa. Control, healthy subject; BPH, Benign prostate hyperplasia; Cancer, prostate cancer.
Studies involving healthy subjects as control groups were subject to diversity of the population characteristics or previous history of infections which, together, may bias the miRNA-level differences between groups. With the exception of a few studies where controls were mentioned to be age-matched (Bryant et al., 2012; Cheng et al., 2014; Bryzgunova et al., 2016; Fujii et al., 2016; Koppers-Lalic et al., 2016; Liu et al., 2019), most of the studies did not mention age or risk factors in the matched controls. Furthermore, the inclusion of BPH patients as a control population adds an extra layer of diversity to the global picture.
In regard to the case group, all studies rightly disclosed detailed information on the patient population analyzed including PSA values, Gleason scores, and clinical presentation (Table 2).
4.2.2 Diversity in sample collection and processing
The collection of urine samples for prostate-related analysis can take place before/pre-DRE or after/post-DRE. Prostate stimulation during a DRE generates the release of cells and vesicles to the urethra, thus urine collection post-DRE is advised for the identification of PCa biomarkers (Pellegrini et al., 2017). Five publications in our review (Koppers-Lalic et al., 2016; Foj et al., 2017; Davey et al., 2020; Matsuzaki et al., 2021; Ruiz-Plazas et al., 2021) highlighted post-DRE urine sample collection whereas three have done so pre-DRE (Samsonov et al., 2016; Fredsøe et al., 2019; Li et al., 2021) and the others have not specified this criterion. Barreiro et al. (2021) have demonstrated that storage time, temperature, and format impact the quantity and quality of the uEV miRNAs yielded. Most of the studies reviewed did not detail the sample storage conditions and those that did, indicated a storage temperature of −80° (Koppers-Lalic et al., 2016; Samsonov et al., 2016; Xu et al., 2017; Bryzgunova et al., 2019; Fredsøe et al., 2019; Davey et al., 2020; Kim et al., 2021; Li et al., 2021; Matsuzaki et al., 2021); other authors preferred to use fresh samples in order to avoid miRNAs degradation or loss. We believe that these aspects should be taken into account in future experiments and their standardization would help decrease inconsistencies in the results obtained across studies.
4.2.3 Selection of miRNAs, methods of expression quantification and analysis
Another limitation identified in this review is the variability in the criteria used for the initial selection of the miRNAs of study; only few studies employed initial microarrays (Bryzgunova et al., 2019; Matsuzaki et al., 2021) or deep sequencing techniques for their further validation in case-control populations (Rodríguez et al., 2017; Kim et al., 2021; Li et al., 2021; Holdmann et al., 2022) whereas the rest of the projects based their miRNA selection on previously published data reporting a correlation between their high levels of expression in serum/blood/tissue and PCa.
In regard to the endogenous controls used in the miRNAs quantification, some studies utilized spike-in miRNA levels like cel-miR-39 (Foj et al., 2017; Xu et al., 2017; Konoshenko et al., 2020; Li et al., 2021), and others used more than one endogenous miRNA or their arithmetic mean (Rodríguez et al., 2017; Fredsøe et al., 2019; Ruiz-Plazas et al., 2021) and others utilized the pair ratio method (Lekchnov et al., 2018; Bryzgunova et al., 2019). In this sense, this field lacks validated internal controls and, given that the exact mechanisms of action of many miRNAs are yet to be elucidated, we may be using controls whose function is associated with PCa disease which could provide unreliable results.
The statistical approaches used in the analysis of the expression of uEV miRNAs evaluated by RT-qPCR also differed across studies. For instance, early studies in the field used the Mann–Whitney test and T-test (Bryzgunova et al., 2016; Samsonov et al., 2016; Foj et al., 2017; Wani et al., 2017; Xu et al., 2017; Hasegawa et al., 2018; Lekchnov et al., 2018) to determine the significance of the identified miRNAs in PCa diagnosis whereas most recent studies applied uni- or multivariate regression-based methods (Fredsøe et al., 2019; Davey et al., 2020; Li et al., 2021).
4.3 Future studies
While urine-derived EVs-associated miRNA biomarkers have shown promising results in the early detection and stratification of PCa, there is no current evidence for their application in the screening or diagnosis of the disease in clinical practice until their clinical significance is further validated. The field of liquid biopsies is expected to evolve in the next few years toward the standardization of the isolation and analysis methods; moreover, technological advances involving the development of more sensitive and specific uEV binding resins will also help mitigate the methodological limitations associated with urine EVs purification. miRNAs, single or in combination with other miRNAs or with other PCa diagnostic tools such as PSA and imaging techniques, are promising strategies yet to refine. On this note, future studies investigating the potential diagnostics value of uEV miRNAs should involve a minimum of a 1-year patient follow-up in order to be able to adequately assess the functionality of the reported biomarker. The approaches for miRNA screening and selection should be unbiased and broader, involving deep sequencing of urine miRNAs that provides a complete miRNA profile in liquid biopsy; as discussed in this review, only a few studies have been close to achieving these goals. The selection of only previously-described PCa miRNA markers for further analysis limits the results and does not open any option to the identification of new biomarkers; this is substantially relevance given that this is a relatively new field lacking further information and knowledge on the biological mechanisms of miRNA. In addition, to avoid measurement bias, all the studies should consider stringent quality checks during sample processing and miRNA measurements such as staged non-human spiked in miRNA and the inclusion of biological and experimental replicates. They should also take into account potential confounders including patients’ risk factors and medications. This review has also detected a lack of consensus in the scientific community in regard to the urine sampling timing, pre- or post-DRE, and the sample volume. Another important point needing improvement in order to ensure data reproducibility is the use of both a discovery and a validation cohort; this approach will help in identifying significantly dysregulated microRNAs in a discovery cohort followed by validation of these findings in an independent larger validation cohort.
4.4 Review limitations
This review did not apply any quality assessment tool like QUADAS for diagnostic accuracy studies, as it was not feasible due to the heterogeneity of the data. For this review, we only selected papers that have been published in the English language.
5 Conclusion
Taken together, the results of the studies covered in this review suggest that the application of uEVs miRNAs in PCa prognostics, diagnostic, and stratification could be effective in combination with traditional tools such as PSA and imaging. There are several limiting factors in the studies published so far involving uEV miRNAs and PCa in regard to the analysis of small case-control populations, the inconsistencies in sample collection times and processing, the limited patient follow-up over time, and the criteria used for miRNA selection.
In this review, we have considered studies on uEV miRNAs that differentiated the control population from BPH subjects and compared them with PCa patients. Based on the collected information, we propose here a panel of eight miRNAs, including miR-21-5p, miR-375, miR-125, miR-19b, miR-191, miR-200, miR-30b, and miR-574-3p, to further investigate and apply in PCa diagnosis, prognosis and stratification.
In the near future, the application of standardized protocols along with the development of multicentre studies involving large cohorts of patients and controls will allow us to define and validate the role of the uEV miRNAs as PCa biomarkers that can be applied in clinical practice.
Author contributions
Research concept and design: GJ and PD; Collection and/or assembly of data: GJ; Data analysis and interpretation: GJ, CC-B, PR, and N; Writing the article: GJ and CC-B; Critical revision of the article: CC-B and FV. All the authors have approved the submitted version and agree to be personally accountable for the author’s own contributions and for ensuring that questions related to the accuracy or integrity of any part of the work.
Funding
This work was supported by a fellowship from the Institute of Eminence, BHU (MPDF to GJ), Indian Council of Medical Research (SRF to PR), Banaras Hindu University (RET Fellowship to N).
Conflict of interest
The authors declare that the research was conducted in the absence of any commercial or financial relationships that could be construed as a potential conflict of interest.
Publisher’s note
All claims expressed in this article are solely those of the authors and do not necessarily represent those of their affiliated organizations, or those of the publisher, the editors and the reviewers. Any product that may be evaluated in this article, or claim that may be made by its manufacturer, is not guaranteed or endorsed by the publisher.
Supplementary material
The Supplementary Material for this article can be found online at: https://www.frontiersin.org/articles/10.3389/fgene.2023.1065757/full#supplementary-material
References
Akers, J. C., Gonda, D., Kim, R., Carter, B. S., and Chen, C. C. (2013). Biogenesis of extracellular vesicles (EV): Exosomes, microvesicles, retrovirus-like vesicles, and apoptotic bodies. J. Neurooncol 113 (1), 1–11. PMID 23456661. doi:10.1007/s11060-013-1084-8
Balk, S. P., Ko, Y. J., and Bubley, G. J. (2003). Biology of prostate-specific antigen. J. Clin. Oncol. 21 (2), 383–391. PMID 12525533. doi:10.1200/JCO.2003.02.083
Banerjee, P. S., and Punnen, S. (2022). A review on the role of tissue-based molecular biomarkers for active surveillance. World J. Urol. 40 (1), 27–34. PMID 33590277. doi:10.1007/s00345-021-03610-y
Barreiro, K., Dwivedi, O. P., Valkonen, S., Groop, P. H., Tuomi, T., Holthofer, H., et al. (2021). Urinary extracellular vesicles: Assessment of pre-analytical variables and development of a quality control with focus on transcriptomic biomarker research. J. Extracell. Vesicles 10 (12), e12158. PMID 34651466, PMCID PMC8517090. doi:10.1002/jev2.12158
Bhagirath, D., Liston, M., Patel, N., Akoto, T., Lui, B., Yang, T. L., et al. (2020). MicroRNA determinants of neuroendocrine differentiation in metastatic castration-resistant prostate cancer. Oncogene 39 (49), 7209–7223. PMID 33037409. doi:10.1038/s41388-020-01493-8
Brassart, B., Da Silva, J., Donet, M., Seurat, E., Hague, F., Terryn, C., et al. (2019). Tumour cell blebbing and extracellular vesicle shedding: Key role of matrikines and ribosomal protein SA. Br. J. Cancer 120 (4), 453–465. PMID 30739912. doi:10.1038/s41416-019-0382-0
Bryant, R. J., Pawlowski, T., Catto, J. W., Marsden, G., Vessella, R. L., Rhees, B., et al. (2012). Changes in circulating microRNA levels associated with prostate cancer. Br. J. Cancer 106 (4), 768–774. PMID 22240788. doi:10.1038/bjc.2011.595
Bryzgunova, O. E., Zaporozhchenko, I. A., Lekchnov, E. A., Amelina, E. V., Konoshenko, M. Y., Yarmoschuk, S. V., et al. (2019). Data analysis algorithm for the development of extracellular miRNA-based diagnostic systems for prostate cancer. PLOS ONE 14 (4), e0215003. PMID 30970027. doi:10.1371/journal.pone.0215003
Bryzgunova, O. E., Zaripov, M. M., Skvortsova, T. E., Lekchnov, E. A., Grigor’eva, A. E., Zaporozhchenko, I. A., et al. (2016). Comparative study of extracellular vesicles from the urine of healthy individuals and prostate cancer patients. PLOS ONE 11 (6), e0157566. PMID 27305142. doi:10.1371/journal.pone.0157566
Chang, R. M., Yang, H., Fang, F., Xu, J. F., and Yang, L. Y. (2014). MicroRNA-331-3p promotes proliferation and metastasis of hepatocellular carcinoma by targeting PH domain and leucine-rich repeat protein phosphatase. Hepatology 60 (4), 1251–1263. PMID 24825302. doi:10.1002/hep.27221
Cheng, L., Sun, X., Scicluna, B. J., Coleman, B. M., and Hill, A. F. (2014). Characterization and deep sequencing analysis of exosomal and non-exosomal miRNA in human urine. Kidney Int. 86 (2), 433–444. PMID 24352158. doi:10.1038/ki.2013.502
Covas Moschovas, M., Chew, C., Bhat, S., Sandri, M., Rogers, T., Dell’Oglio, P., et al. (2022). Association between oncotype DX genomic prostate score and adverse tumor pathology after radical prostatectomy. Eur. Urol. Focus 8 (2), 418–424. PMID 33757735. doi:10.1016/j.euf.2021.03.015
Damodaran, M., Chinambedu Dandapani, M., SimonDuraiRaj, S., VenkatRamanan, S., Ramachandran, I., Venkatesan, V., et al. (2021). Differentially expressed miR-20, miR-21, miR-100, miR-125a and miR-146a as a potential biomarker for prostate cancer. Mol. Biol. Rep. 48 (4), 3349–3356. PMID 33948855. doi:10.1007/s11033-021-06384-z
Davey, M., Benzina, S., Savoie, M., Breault, G., Ghosh, A., and Ouellette, R. J. (2020). Affinity captured urinary extracellular vesicles provide mRNA and miRNA biomarkers for improved accuracy of prostate cancer detection: A pilot study. Int. J. Mol. Sci. 21 (21), 8330. PMID 33172003. doi:10.3390/ijms21218330
Dong, H., Weng, C., Bai, R., Sheng, J., Gao, X., Li, L., et al. (2019). The regulatory network of miR-141 in the inhibition of angiogenesis. Angiogenesis 22 (2), 251–262. PMID 30465119. doi:10.1007/s10456-018-9654-1
Drago, D., Andolfo, A., Mosca, E., Orro, A., Nocera, L., Cucchiara, V., et al. (2021). A novel expressed prostatic secretion (EPS)-urine metabolomic signature for the diagnosis of clinically significant prostate cancer. Cancer Biol. Med. 18 (2), 604–615. PMID 34037347. doi:10.20892/j.issn.2095-3941.2020.0617
Drake, R. R., White, K. Y., Fuller, T. W., Igwe, E., Clements, M. A., Nyalwidhe, J. O., et al. (2009). Clinical collection and protein properties of expressed prostatic secretions as a source for biomarkers of prostatic disease. J. Proteomics 72 (6), 907–917. PMID 19457353. doi:10.1016/j.jprot.2009.01.007
Duca, R. B., Massillo, C., Dalton, G. N., Farré, P. L., Graña, K. D., Gardner, K., et al. (2021). MiR-19b-3p and miR-101-3p as potential biomarkers for prostate cancer diagnosis and prognosis. Am. J. Cancer Res. 11 (6), 2802–2820. PMID 34249429.
Fenton, J. J., Weyrich, M. S., Durbin, S., Liu, Y., Bang, H., and Melnikow, J. (2018). Prostate-specific antigen–based screening for prostate cancer: A systematic evidence review for the us preventive Services Task Force. JAMA 319 (18), 1914–1913. doi:10.1001/jama.2018.3712
Foj, L., Ferrer, F., Serra, M., Arévalo, A., Gavagnach, M., Giménez, N., et al. (2017). Exosomal and non-exosomal urinary miRNAs in prostate cancer detection and prognosis. Prostate 77 (6), 573–583. PMID 27990656. doi:10.1002/pros.23295
Fossati, N., Lazzeri, M., Haese, A., McNicholas, T., de la Taille, A., Buffi, N. M., et al. (2015). Clinical performance of serum isoform [−2]proPSA (p2PSA), and its derivatives %p2PSA and the prostate health Index, in men aged <60 years: Results from a multicentric European study. BJU Int. 115 (6), 913–920. PMID 24589357. doi:10.1111/bju.12718
Fredsøe, J., Rasmussen, A. K. I., Mouritzen, P., Borre, M., Ørntoft, T., and Sørensen, K. D. (2019). A five-microRNA model (pCaP) for predicting prostate cancer aggressiveness using cell-free urine. Int. J. Cancer 145 (9), 2558–2567. PMID 30903800. doi:10.1002/ijc.32296
Fujii, T., Shimada, K., Tatsumi, Y., Tanaka, N., Fujimoto, K., and Konishi, N. (2016). Syndecan-1 up-regulates microRNA-331-3p and mediates epithelial-to-mesenchymal transition in prostate cancer. Mol. Carcinog. 55 (9), 1378–1386. PMID 26259043. doi:10.1002/mc.22381
Gámez-Valero, A., Lozano-Ramos, S. I., Bancu, I., Lauzurica-Valdemoros, R., and Borràs, F. E. (2015). Urinary extracellular vesicles as source of biomarkers in kidney diseases. Front. Immunol. 6, 6. PMID 25688242. doi:10.3389/fimmu.2015.00006
Gao, Y., Zhang, M., Li, X., Zeng, P., Wang, P., and Zhang, L. (2019). Serum PSA levels in patients with prostate cancer and other 33 different types of diseases. Prog. Mol. Biol. Transl. Sci. 162, 377–390. PMID 30905464. doi:10.1016/bs.pmbts.2018.12.013
Groot, M., and Lee, H. (2020). Sorting mechanisms for microRNAs into extracellular vesicles and their associated diseases. Cells 9 (4), 1044. PMID 32331346. doi:10.3390/cells9041044
Hasegawa, T., Glavich, G. J., Pahuski, M., Short, A., Semmes, O. J., Yang, L., et al. (2018). Characterization and evidence of the miR-888 cluster as a novel cancer network in prostate. Mol. Cancer Res. 16 (4), 669–681. PMID 29330297. doi:10.1158/1541-7786.MCR-17-0321
Hessels, D., Klein Gunnewiek, J. M., van Oort, I., Karthaus, H. F., van Leenders, G. J., van Balken, B., et al. (2003). DD3(PCA3)-based molecular urine analysis for the diagnosis of prostate cancer. Eur. Urol. 44 (1), 8–15. PMID 12814669. doi:10.1016/s0302-2838(03)00201-x
Hodge, K. K., McNeal, J. E., and Stamey, T. A. (1989). Ultrasound guided transrectal core biopsies of the palpably abnormal prostate. J. Urol. 142 (1), 66–70. PMID 2659826. doi:10.1016/S0022-5347(17)38663-9
Holdmann, J., Markert, L., Klinger, C., Kaufmann, M., Schork, K., Turewicz, M., et al. (2022). MicroRNAs from urinary exosomes as alternative biomarkers in the differentiation of benign and malignant prostate diseases. J. Circ. Biomark. 11, 5–13. PMID 35173821. doi:10.33393/jcb.2022.2317
Hugosson, J., Roobol, M. J., Månsson, M., Tammela, T. L. J., Zappa, M., Nelen, V., et al. (2019). A 16-yr follow-up of the European randomized study of screening for prostate cancer. Eur. Urol. 76 (1), 43–51. PMID 30824296. doi:10.1016/j.eururo.2019.02.009
Irekpita, E., Achor, G. O., and Alili, U. (2020). Assessment of the value of the different variants of abnormal digital rectal examination finding in predicting carcinoma of the prostate: A preliminary report of a two-center study. Afr. J. Urol. 26 (1), 3. doi:10.1186/s12301-019-0013-2
Jones, D., Friend, C., Dreher, A., Allgar, V., and Macleod, U. (2018). The diagnostic test accuracy of rectal examination for prostate cancer diagnosis in symptomatic patients: A systematic review. BMC Fam. Pract. 19 (1), 79. PMID 29859531. doi:10.1186/s12875-018-0765-y
Kim, M. Y., Shin, H., Moon, H. W., Park, Y. H., Park, J., and Lee, J. Y. (2021). Urinary exosomal microRNA profiling in intermediate-risk prostate cancer. Sci. Rep. 11 (1), 7355. PMID 33795765. doi:10.1038/s41598-021-86785-z
Konoshenko, M. Y., Lekchnov, E. A., Bryzgunova, O. E., Zaporozhchenko, I. A., Yarmoschuk, S. V., Pashkovskaya, O. A., et al. (2020). The panel of 12 cell-free MicroRNAs as potential biomarkers in prostate neoplasms. Diagn. (Basel) 10 (1), 38. PMID 31936850. doi:10.3390/diagnostics10010038
Koppers-Lalic, D., Hackenberg, M., de Menezes, R., Misovic, B., Wachalska, M., Geldof, A., et al. (2016). Noninvasive prostate cancer detection by measuring miRNA variants (isomiRs) in urine extracellular vesicles. Oncotarget 7 (16), 22566–22578. PMID 26992225. doi:10.18632/oncotarget.8124
Lässer, C. (2013). Identification and analysis of circulating exosomal microRNA in human body fluids. Methods Mol. Biol. 1024, 109–128. PMID 23719946. doi:10.1007/978-1-62703-453-1_9
Lekchnov, E. A., Amelina, E. V., Bryzgunova, O. E., Zaporozhchenko, I. A., Konoshenko, M. Y., Yarmoschuk, S. V., et al. (2018). Searching for the novel specific predictors of prostate cancer in urine: The analysis of 84 miRNA expression. Int. J. Mol. Sci. 19 (12), 4088. PMID 30562989. doi:10.3390/ijms19124088
Li, Z., Li, L. X., Diao, Y. J., Wang, J., Ye, Y., and Hao, X. K. (2021). Identification of urinary exosomal miRNAs for the non-invasive diagnosis of prostate cancer. Cancer Manag. Res. 13, 25–35. PMID 33442291. doi:10.2147/CMAR.S272140
Liu, H. Y., Zhang, Y. Y., Zhu, B. L., Feng, F. Z., Yan, H., Zhang, H. Y., et al. (2019). miR-21 regulates the proliferation and apoptosis of ovarian cancer cells through PTEN/PI3K/AKT. Eur. Rev. Med. Pharmacol. Sci. 23 (10), 4149–4155. PMID 31173285. doi:10.26355/eurrev_201905_17917
Maia, J., Caja, S., Strano Moraes, M. C., Couto, N., and Costa-Silva, B. (2018). Exosome-based cell-cell communication in the tumor microenvironment. Front. Cell Dev. Biol. 6, 18. PMID 29515996. doi:10.3389/fcell.2018.00018
Mao, L., Liu, S., Hu, L., Jia, L., Wang, H., Guo, M., et al. (2018). miR-30 family: A promising regulator in development and disease. Biomed. Res. Int. 2018, 9623412–9623418. doi:10.1155/2018/9623412
Margolis, E., Brown, G., Partin, A., Carter, B., McKiernan, J., Tutrone, R., et al. (2022). Predicting high-grade prostate cancer at initial biopsy: Clinical performance of the ExoDx (EPI) prostate intelliscore test in three independent prospective studies. Prostate Cancer Prostatic Dis. 25 (2), 296–301. PMID 34593984. doi:10.1038/s41391-021-00456-8
Martin, R. M., Donovan, J. L., Turner, E. L., Metcalfe, C., Young, G. J., Walsh, E. I., et al. (2018). Effect of a low-intensity PSA-based screening intervention on prostate cancer mortality: The CAP randomized clinical trial. JAMA 319 (9), 883–895. PMID 29509864. doi:10.1001/jama.2018.0154
Matsuzaki, K., Fujita, K., Tomiyama, E., Hatano, K., Hayashi, Y., Wang, C., et al. (2021). MiR-30b-3p and miR-126-3p of urinary extracellular vesicles could be new biomarkers for prostate cancer. Transl. Androl. Urol. 10 (4), 1918–1927. PMID 33968679. doi:10.21037/tau-20-421
Parekh, D. J., Punnen, S., Sjoberg, D. D., Asroff, S. W., Bailen, J. L., Cochran, J. S., et al. (2015). A multi-institutional prospective trial in the USA confirms that the 4Kscore accurately identifies men with high-grade prostate cancer. Eur. Urol. 68 (3), 464–470. PMID 25454615. doi:10.1016/j.eururo.2014.10.021
Pellegrini, K. L., Patil, D., Douglas, K. J. S., Lee, G., Wehrmeyer, K., Torlak, M., et al. (2017). Detection of prostate cancer-specific transcripts in extracellular vesicles isolated from post-DRE urine. Prostate 77 (9), 990–999. PMID 28419548PMCID PMC5907935. doi:10.1002/pros.23355
Rodríguez, M., Bajo-Santos, C., Hessvik, N. P., Lorenz, S., Fromm, B., Berge, V., et al. (2017). Identification of non-invasive miRNAs biomarkers for prostate cancer by deep sequencing analysis of urinary exosomes. Mol. Cancer 16 (1), 156. PMID 28982366. doi:10.1186/s12943-017-0726-4
Roehrborn, C. G., and Black, L. K. (2011). The economic burden of prostate cancer. BJU Int. 108 (6), 806–813. PMID 21884356. doi:10.1111/j.1464-410X.2011.10365.x
Ruiz-Plazas, X., Altuna-Coy, A., Alves-Santiago, M., Vila-Barja, J., García-Fontgivell, J. F., Martínez-González, S., et al. (2021). Liquid biopsy-based Exo-oncomiRNAs can predict prostate cancer aggressiveness. Cancers (Basel) 13 (2), 250. PMID 33440913. doi:10.3390/cancers13020250
Salagierski, M., and Schalken, J. A. (2012). Molecular diagnosis of prostate cancer: PCA3 and TMPRSS2:ERG gene fusion. J. Urol. 187 (3), 795–801. PMID 22245323. doi:10.1016/j.juro.2011.10.133
Samsonov, R., Shtam, T., Burdakov, V., Glotov, A., Tsyrlina, E., Berstein, L., et al. (2016). Lectin-induced agglutination method of urinary exosomes isolation followed by miRNA analysis: Application for prostate cancer diagnostic. Prostate 76 (1), 68–79. PMID 26417675. doi:10.1002/pros.23101
Siegel, R. L., Miller, K. D., Fuchs, H. E., and Jemal, A. (2021). Cancer statistics, 2016. CA Cancer J. Clin. 71 (1), 7–30. PMID 33433946. doi:10.3322/caac.21332
Stenman, U. H., Leinonen, J., Zhang, W. M., and Finne, P. (1999). Prostate-specific antigen. Semin. Cancer Biol. 9 (2), 83–93. PMID 10202130. doi:10.1006/scbi.1998.0086
Stewart, G. D., Van Neste, L., Delvenne, P., Delrée, P., Delga, A., McNeill, S. A., et al. (2013). Clinical utility of an epigenetic assay to detect occult prostate cancer in histopathologically negative biopsies: Results of the MATLOC study. J. Urol. 189 (3), 1110–1116. doi:10.1016/j.juro.2012.08.219
Todorova, K., Metodiev, M. V., Metodieva, G., Zasheva, D., Mincheff, M., and Hayrabedyan, S. (2016). miR-204 is dysregulated in metastatic prostate cancer in vitro. Mol. Carcinog. 55 (2), 131–147. PMID 25630658. doi:10.1002/mc.22263
Tong, S. J., Zhang, X. Y., Guo, H. F., Yang, J., Qi, Y. P., and Lu, S. (2021). Study on effects of miR-141-3p in proliferation, migration, invasion and apoptosis of colon cancer cells by inhibiting Bcl2. Clin. Transl. Oncol. 23 (12), 2526–2535. PMID 34086253. doi:10.1007/s12094-021-02653-2
Van Neste, L., Hendriks, R. J., Dijkstra, S., Trooskens, G., Cornel, E. B., Jannink, S. A., et al. (2016). Detection of high-grade prostate cancer using a urinary molecular biomarker-based risk score. Eur. Urol. 70, 740–748. doi:10.1016/j.eururo.2016.04.012
Voigt, J. D., Dong, Y., Linder, V., and Zappala, S. (2017). Use of the 4Kscore test to predict the risk of aggressive prostate cancer prior to prostate biopsy: Overall cost savings and improved quality of care to the us healthcare system. Rev. Urol. 19 (1), 1–10. PMID 28522924. doi:10.3909/riu0753
Wa, Q., Huang, S., Pan, J., Tang, Y., He, S., Fu, X., et al. (2019). miR-204-5p represses bone metastasis via inactivating NF-κB signaling in prostate cancer. Mol. Ther. Nucleic Acids 18, 567–579. PMID 31678733. doi:10.1016/j.omtn.2019.09.008
Wani, S., Kaul, D., Mavuduru, R. S., Kakkar, N., and Bhatia, A. (2017). Urinary-exosomal miR-2909: A novel pathognomonic trait of prostate cancer severity. J. Biotechnol. 259, 135–139. PMID 28764970. doi:10.1016/j.jbiotec.2017.07.029
Wolters, T., van der Kwast, T. H., Vissers, C. J., Bangma, C. H., Roobol, M., Schröder, F. H., et al. (2010). False-negative prostate needle biopsies: Frequency, histopathologic features, and follow-up. Am. J. Surg. Pathol. 34 (1), 35–43. PMID 19935058. doi:10.1097/PAS.0b013e3181c3ece9
Xu, Y., Qin, S., An, T., Tang, Y., Huang, Y., and Zheng, L. (2017). MiR-145 detection in urinary extracellular vesicles increase diagnostic efficiency of prostate cancer based on hydrostatic filtration dialysis method. Prostate 77 (10), 1167–1175. PMID 28617988. doi:10.1002/pros.23376
Yaman Agaoglu, F., Kovancilar, M., Dizdar, Y., Darendeliler, E., Holdenrieder, S., Dalay, N., et al. (2011). Investigation of miR-21, miR-141, and miR-221 in blood circulation of patients with prostate cancer. Tumour Biol. 32 (3), 583–588. PMID 21274675. doi:10.1007/s13277-011-0154-9
Keywords: prostate cancer, biomarker, micro-RNA, liquid biopsy, extracellular vesicles (EV)
Citation: Jain G, Das P, Ranjan P, Neha , Valderrama F and Cieza-Borrella C (2023) Urinary extracellular vesicles miRNA—A new era of prostate cancer biomarkers. Front. Genet. 14:1065757. doi: 10.3389/fgene.2023.1065757
Received: 10 October 2022; Accepted: 09 January 2023;
Published: 20 January 2023.
Edited by:
Marco Morselli, University of Parma, ItalyReviewed by:
Hojat Dehghanbanadaki, Tehran University of Medical Sciences, IranÁlvaro González Hernández, University Clinic of Navarra, Spain
Copyright © 2023 Jain, Das, Ranjan, Neha, Valderrama and Cieza-Borrella. This is an open-access article distributed under the terms of the Creative Commons Attribution License (CC BY). The use, distribution or reproduction in other forums is permitted, provided the original author(s) and the copyright owner(s) are credited and that the original publication in this journal is cited, in accordance with accepted academic practice. No use, distribution or reproduction is permitted which does not comply with these terms.
*Correspondence: Garima Jain, Z2FyaW1hLmphaW5AYmh1LmFjLmlu; Clara Cieza-Borrella, Y2NpZXphLWJAc2d1bC5hYy51aw==