- 1State Key Laboratory of Food Science and Technology, Nanchang University, Nanchang, China
- 2Sino-German Joint Research Institute, Nanchang University, Nanchang, China
- 3Key Laboratory of Poyang Lake Environment and Resource Utilization of Ministry of Education, School of Chemistry and Chemical Engineering, Nanchang University, Nanchang, China
The limitations of the current genome-walking strategies include strong background and cumbersome experimental processes. Herein, we report a genome-walking method, fusion primer-driven racket PCR (FPR-PCR), for the reliable retrieval of unknown flanking DNA sequences. Four sequence-specific primers (SSP1, SSP2, SSP3, and SSP4) were sequentially selected from known DNA (5'→3′) to perform FPR-PCR. SSP3 is the fragment that mediates intra-strand annealing (FISA). The FISA fragment is attached to the 5′ end of SSP1, generating a fusion primer. FPR-PCR comprises two rounds of amplification reactions. The single-fusion primary FPR-PCR begins with the selective synthesis of the target first strand, then allows the primer to partially anneal to some place(s) on the unknown region of this strand, producing the target second strand. Afterward, a new first strand is synthesized using the second strand as the template. The 3′ end of this new first strand undergoes intra-strand annealing to the FISA site, followed by the formation of a racket-like DNA by a loop-back extension. This racket-like DNA is exponentially amplified in the secondary FPR-PCR performed using SSP2 and SSP4. We validated this FPR-PCR method by identifying the unknown flanks of Lactobacillus brevis CD0817 glutamic acid decarboxylase genes and the rice hygromycin gene.
Introduction
Genome-walking is a method that starts from a known region of the genome or genome library and then sequentially mines the unknown flanking sequences (Leoni et al., 2008; Kotik, 2009). Genome-walking has practical significance in molecular biology and related fields. The successful applications of genome-walking include 1) obtaining the regulatory genes of structural genes; 2) amplifying non-conserved sequences based on the conserved regions of genes; 3) identifying insertion sites for T-DNA or transposons; 4) filling gaps in whole-genome sequencing; 5) discovering new functional genes; and 6) screening microbes (Myrick and Gelbart, 2007; Kotik, 2009; Thirulogachandar et al., 2011; Fraiture et al., 2021; Zhou et al., 2021).
Genome-walking generally includes genome library and PCR-based strategies. The former strategy is cumbersome and labor-intensive due to the construction and then screening of genomic DNA libraries (Li et al., 2015; Zeng et al., 2020). The PCR-based strategy is attractive because of its efficiency and rapidity (Kotik, 2009; Alquezar-Planas et al., 2020). Several PCR-based genome-walking methods have been developed and validated for identifying unknown flanking DNA sequences. These PCR-based methods vary substantially in experimental processes but can be divided into four categories: 1) inverse PCR (Ochman et al., 1988; Uchiyama and Watanabe, 2006); 2) panhandle PCR; 3) ligation-dependent PCR (Yan et al., 2003; Yik et al., 2021); and 4) randomly primed PCR (Sun et al., 2022; Tan et al., 2005; Zhang et al., 2018).
Inverse PCR was the first described PCR-based genome-walking method. In this method, the genomic DNA is digested by an endonuclease. The digested fragments then undergo self-circularization with the help of DNA ligase and the circularized DNA is amplified, like classical end-to-end PCR, by a sequence-specific primer (SSP) pair. The two primers are in the orientation of the 5′ end facing the 5′ end, opposite to the classical one of the 3′ end facing the 3′ end (Ochman et al., 1988; Triglia et al., 1988). Inverse PCR has high specificity owing to the use of an SSP pair. However, the efficiency of this method is hindered by the complicated steps before PCR and the preferential amplification of small DNA (Kotik, 2009; Chang et al., 2018).
Panhandle PCR, as the name implies, requires that the target DNA form a panhandle-like structure before PCR. In routine panhandle PCR, the formation of panhandle-like DNA relies on the digestion of genomic DNA. The ends of the digested product are then ligated to a fragment homologous to a known site (Jones and Winistorfer, 1992; Jones and Winistorfer, 1997). The two improved versions, universal fast walking (Myrick and Gelbart, 2002) and self-formed adapter (Wang et al., 2007) PCR, use a walking primer (with a 5′ part homologous to known DNA) to mediate the formation of panhandle-like DNA through a random priming strategy. Universal fast walking provides long-range walking because only the most distal site partially bound to the primer is further processed as follows: exonuclease I digestion stops at the branch point of this site; thereafter, DNA polymerization starts from the trimmed target DNA 3′ end using the 5′ part of the primer as the template. The extended 3′ end can mediate the formation of panhandle-like DNA (Myrick and Gelbart, 2002). A panhandle PCR is completed using SSP pairs; thus, it shows equivalent amplification specificity to that of inverse PCR.
Like inverse PCR, ligation-dependent PCR also requires endonuclease digestion. The digested products are then ligated to random DNA. Subsequently, the target DNA is enriched by 2–3 rounds of PCR in which the random primers sequentially pair with nested SSPs (Yan et al., 2003; Ji and Braam, 2010). However, background arising from the random primer alone is a drawback (Kotik, 2009; Li et al., 2015).
Randomly primed PCR does not involve any extra operation before PCR (Jia et al., 2017). In the primary PCR, a relaxed-stringency cycle is required to facilitate the walking primer to partially anneal to the unknown flank to create a DNA of interest. This DNA is then gradually enriched by 2–3 rounds of nested PCR based on the superior annealing efficiency of SSPs to the walking primer (Liu and Whittier, 1995; Chang et al., 2018). Like ligation-dependent PCR, the reliability of randomly primed PCR is reduced by the non-target amplification of the walking primer (Kotik, 2009; Yu et al., 2020).
Herein, we propose a novel PCR-based genome-walking method, fusion primer-driven racket PCR (FPR-PCR). This method uses a tri-functional primer fused by two sequence-specific fragments formed in the primary PCR. The tri-functional primer mediates walking, selective enrichment, and intra-strand annealing of the target DNA. We verified the practicability of this method by amplifying the unknown flanks of the Lactobacillus brevis CD0817 glutamic acid decarboxylase genes (gadR/C) (Gao et al., 2019; Jia et al., 2022) and the rice hygromycin gene (hyg) (Li et al., 2015).
Materials and methods
Genomic DNA isolation
The genomic DNA of L. brevis CD0817 (Gao et al., 2019) was isolated using the TIANamp Bacteria DNA Kit (TIANGEN Biotech Co., Ltd., Beijing, China) according to the manufacturer’s instructions.
Primer design
The genome (CP032931.1) of L. brevis CD0817 and the hyg gene (KF206149.1) (Gao et al., 2019; Li et al., 2015, 2022) with the surrounding regions are available in GenBank. A part of gadR (AYM03984.1), gadC (AYM03983.1), or hyg was chosen as the “known sequence” for SSP design, while the adjacent region was assumed to be “unknown sequence”. The “known sequence” plus its “unknown sequence” is here collectively called the “reference sequence” (Supplementary Figure S1). In this study, five sequence-specific fragments with the same orientation, namely, two SSP1s (SSP1α and SSP1β), one SSP2, one SSP3 (fragment mediating intra-strand annealing [FISA]), and one SSP4, were sequentially chosen in the direction of 5′ to 3′ from each known region. Two fusion primers (FPα and FPβ) were made by attaching the FISA to the 5′ ends of SSP1α and SSP1β, respectively, to perform two parallel FPR-PCRs. SSP1α and SSP1β can be entirely irrelevant or partially overlapping; in the latter case, the difference between the two fragments’ 3′ ends must be at least 3 nt. The FISA is 17–18 nt with a Tm of 45–55°C. The SSP1s are 17–21 nt with a Tm of 50–55°C. The fusion primers are 34–39 nt with a Tm of 65–70°C. SSP2 or SSP3 are 25–30 nt with a Tm of 60–66°C. Any primer or primer pair must be free of severe self-hairpin or dimer. The primers used in the present study are listed in Table 1.
PCR procedure
Each FPR-PCR comprised two rounds of PCR amplification. The primary PCR was conducted using a single fusion primer with genomic DNA as the template. The secondary PCR used the primary product as the template and included SSP2 and SSP4. To ensure walking success and efficiency, two parallel FPR-PCRs were simultaneously performed for any selected gene locus. The two primary PCRs were individually driven by the two fusion primers, while the two secondary PCRs were driven by the universal SSP primers SSP2 and SSP4.
The 50-μL primary reaction mixture included 1×LA PCR buffer II (Mg2+ Plus), 0.4 mM of each dNTP, genomic DNA (10–100 ng for the microbe and 100–1,000 ng for rice), 0.2 μM of a fusion primer, and 2.5 U of TaKaRa LA Taq polymerase (TaKaRa, Perking, China). The 50-μL secondary reaction mixtures included 1×LA PCR buffer II (Mg2+ plus), 0.4 mM of each dNTP, 1µL/50 µL primary PCR product, 0.2 μM of SSP2, 0.2 μM of SSP4, and 2.5 U of TaKaRa LA Taq polymerase.
The primary PCR comprised three annealing stages: stage 1, five moderate-stringency (55°C) cycles; stage 2, one low-stringency (25°C) cycle; and stage 3, thirty high-stringency (65°C) cycles. The secondary PCR consisted of twenty-five moderate high-stringency (60°C) cycles. The detailed thermal cycling conditions for FPR-PCR are presented in Table 2. The PCR reactions were performed on a Biometra TOne 96G PCR thermal cycler (Analytik Jena, Germany).
DNA manipulation and sequencing
The PCR products were visualized by electrophoresis on 1.5% agarose gels. The major bands of the secondary PCRs were purified with the DiaSpin column DNA Gel Extraction Kit (Sangon Biotech Co. Ltd., Shanghai, China) and then sent to Sangon Biotech Co., Ltd. for sequencing using SSP2 and SSP4. This company uses ABI sequencers (3730xl DNA Analyzer) for DNA sequence analysis.
Results
FPR-PCR outline
The four sequence-specific primers (SSP1, SSP2, SSP3 [FISA], and SSP4) used in FPR-PCR are successively located on the known DNA in the 5′ to 3′ direction. The FPR-PCR comprises two rounds (primary and secondary) of nested amplification reactions. The SSP3 (FISA) is attached to the 5′ end of SSP1 to make a fusion primer mediating the primary FPR-PCR. SSP2 and SSP4 are used together in the secondary FPR-PCR.
The primary FPR-PCR starts with five moderate-stringency (55°C) cycles that permit the fusion primer 3′-part (SSP1) to hybridize only to its complement in the known region to increase the copies of the target first-strand. The subsequent single low-stringency (25°C) cycle helps the fusion primer to partially anneal to place(s) in the unknown region of this strand and extend toward the known region to produce the target second-strand, encompassing the fusion primer and its inverted repeat. Next, the target DNA is exponentially amplified in the following high-stringency (65°C) cycles. Some first-strands undergo intra-strand annealing between the FISA and its inverted repeat at the 3′ end; thereafter, the recessed 3′ end extends along the protruding known region. As a result, a racket-like DNA is formed, in which the unknown single-stranded region is defined by the known double-stranded stem. The secondary PCR is classical end-to-end PCR driven by SSP2 and SSP4 to amplify the target DNA exponentially. Any non-target DNAs cannot be amplified due to the lack of a perfect binding site to SSP2 or SSP4. Finally, the target DNA becomes the major product.
More than one fragment may be chosen near the SSP1 site. A corresponding number of fusion primers can then be created by appending the FISA to the 5′ ends of the chosen fragments. In this situation, parallel FPR-PCRs can be performed to guarantee genome-walking success and efficiency. The present study used two SSP1 fragments (SSP1α and SSP1β) from each given gene, thus resulting in two parallel sets of FPR-PCRs.
FPR-PCR validation
To validate the practicability of FPR-PCR, we applied the method to retrieve the unknown sequences for the segments adjacent to L. brevis CD0817 gadR/C genes and the rice hyg gene. We designed two fusion primers (FPα and FPβ) and one nested SSP primer pair SSP2/SSP4 (Table 1) for each gene to perform FPR-PCR. Thus, each walk in this study comprised two parallel sets of FPR-PCRs. The two primary PCRs were individually performed by the two fusion primers, while the two secondary PCRs were performed by the universal nested SSP primer pair. The detailed experimental processes are described in the Materials and Methods section. After walking, the PCR products were subjected to electrophoretic analysis on 1.5% agarose gels. As shown in Figure 1, each secondary FPR-PCR exhibited 1 – 2 clear DNA band(s). The lengths of the mined unknown DNAs ranged from 0.3 to 1.6 kb in the three walks, with the longest walking distances of 1.2, 1.6, and 0.5 kb for gadR, gadC, and hyg, respectively. These DNA bands were recovered and directly sequenced. The sequencing data showed that all the DNAs were of interest, as each overlapped with the end of the corresponding known region. These results validated the efficiency of the proposed FPR-PCR method.
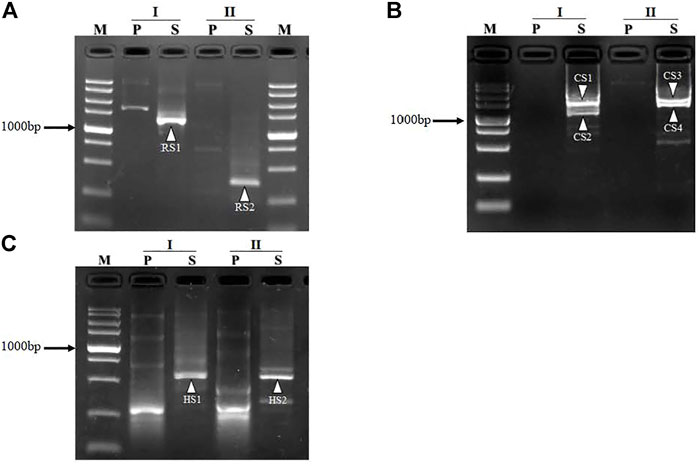
FIGURE 1. Mining unknown regions flanking gadR (A) and gadC (B) in Lactobacillus brevis CD0817 and hyg (C) in rice. I and II denote the two sets of FPR-PCRs in each round of walking. The bands RS1-RS2, CS1-CS4, and HS1-HS2, indicated by white arrowheads, are the secondary PCR products for gadR, gadC, and hyg, respectively. Lane P, primary PCR; lane S, secondary PCR; lane M, TaKaRa DL5000 DNA marker (from top to bottom 5000, 3000, 2000, 1,500, 1,000, 750, 500, 250, and 100 bp).
Analysis of random annealing sites of fusion primers
Based on the sequencing data of the obtained target DNA bands RS1-RS2, CS1-CS4, and HS1-HS2 (Figure 1), sequence alignments between the fusion primers and their partial annealing loci were performed, as summarized in Figure 2. The results demonstrated that any functional partial annealing showed a correct match of at least two base pairs at the 3′ terminus of a fusion primer. We also observed that the total number of matched base pairs ranged from 14 to 19. In general, the base paring was skewed towards primer 3′ end.
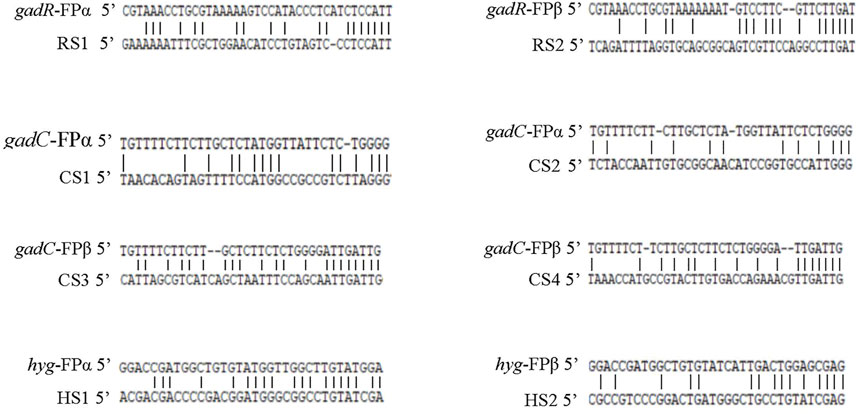
FIGURE 2. Partial annealing loci of the fusion primers. The upper and lower sequences are the fusion primer and annealing site, respectively. RS1-RS2, CS1-CS4, and HS1-HS2 correspond to the DNA bands described in Figure 1.
Discussion
The currently available PCR-based genome-walking methods are unsatisfactory due to limitations including low success rates, high background, and complicated procedures (Myrick and Gelbart, 2002; Wang et al., 2022). Therefore, practical PCR-based genome-walking methods have been rigorously pursued. In this study, we report an efficient and reliable genome-walking method, FPR-PCR. The key to this method is the use of a tri-functional primer in primary PCR, which is created by splicing FISA to the 5′ end of SSP1. The three functions of this primer are: (I) mediate genomic walking by acting as the walking primer; (II) mediate sequence-specific annealing through the 3′ end of SSP1 to enhance the specificity; and (III) mediate intra-strand annealing through the FISA sequence to integrate known sequence into another site in the unknown region.
The primary amplification in existing randomly primed PCR methods requires a walking primer with a random sequence, in addition to the outermost SSP. Therefore, three types of undesired species are generally formed, including (I) SSP alone; (II) the combination of SSP and walking primer; and (III) the walking primer alone (Liu and Whittier, 1995; Kotik, 2009; Wang et al., 2022). In the FPR-PCR primary reaction, however, the fusion primer acts as not only SSP but also the walking primer. Thus, only one type of non-target species, primed by this fusion primer alone, is produced. This feature of FPR-PCR contributes to the specificity of this method.
The secondary FPR-PCR is completely sequence-specific. In this regard, FPR-PCR has identical specificity as those of the inverse (Ochman et al., 1988; Triglia et al., 1988) or panhandle (Jones, 1995; Myrick and Gelbart, 2002; Wang et al., 2007) PCR methods. However, inverse PCR requires DNA digestion and ligation before amplification, while panhandle PCR requires the complicated step to form the panhandle-like DNA. Compared to these strategies, the proposed FPR-PCR is a highly intensive and streamlined method involving two seamlessly connected, single-tube PCRs and a regular thermal cycling program. From the aspect of convenience, FPR-PCR is superior to both inverse and panhandle PCR methods.
The annealing temperature of the low-stringency cycle in primary FPR-PCR is low as 25°C. At such low temperatures, the fusion primer may identify some site(s) on the unknown flank suitable for partial annealing. Once this partial annealing occurs, walking should succeed. This low-stringency cycle is crucial to the success of FPR-PCR. Additionally, the ability to perform parallel simultaneous experiments provides a further guarantee for the success of FPR-PCR, as at least one FPR-PCR will give a positive outcome. FPR-PCR provides a high success rate similar to those reported for randomly primed PCRs, such as partially overlapping primer-based PCR (Li et al., 2015), stepwise partially overlapping primer-based PCR (Chang et al., 2018), and wristwatch PCR (Wang et al., 2022).
A straightforward genome-walking method has been highly pursued to allow rapid data acquisition and technology advances. FPR-PCR is one such straightforward genome-walking method, as it abolishes not only DNA pretreatments before PCR but also subsequent molecular cloning that are standard in the currently prevailing methods. Another criterion for straightforwardness met by FPR-PCR is that the purified secondary amplicon can be directly sequenced due to the sufficient amplification specificity.
Most PCR-based methods have walking abilities of 0.3–3.0 kb (Liu and Whittier, 1995; Tan et al., 2005; Ashrafmansouri et al., 2020). However, universal fast walking (Myrick and Gelbart, 2002) and self-formed adapter (Wang et al., 2007) PCR show stronger walking abilities. The universal fast walking benefits from the fact that only the most distal site bound by walking primer can mediate intra-strand annealing (Myrick and Gelbart, 2002). Regarding self-formed adapter PCR (Wang et al., 2007), the improvement is presumably due to the limited number of templates, as weak partial annealing of the walking primer has few opportunities to form the adapter. Therefore, the probability of obtaining a long target DNA increases due to the major partial annealing. In the present study, the longest walking distances were 1.2, 1.6, and 0.5 kb, respectively, for the loci of gadR, gadC, and hyg (Figure 3). However, the length of the target amplicon is unpredictable in any PCR-based walking method, as it depends on the distance from the major partial annealing site of the walking primer to the known region. FPR-PCR can ensure walking efficiency by employing parallel experiments.
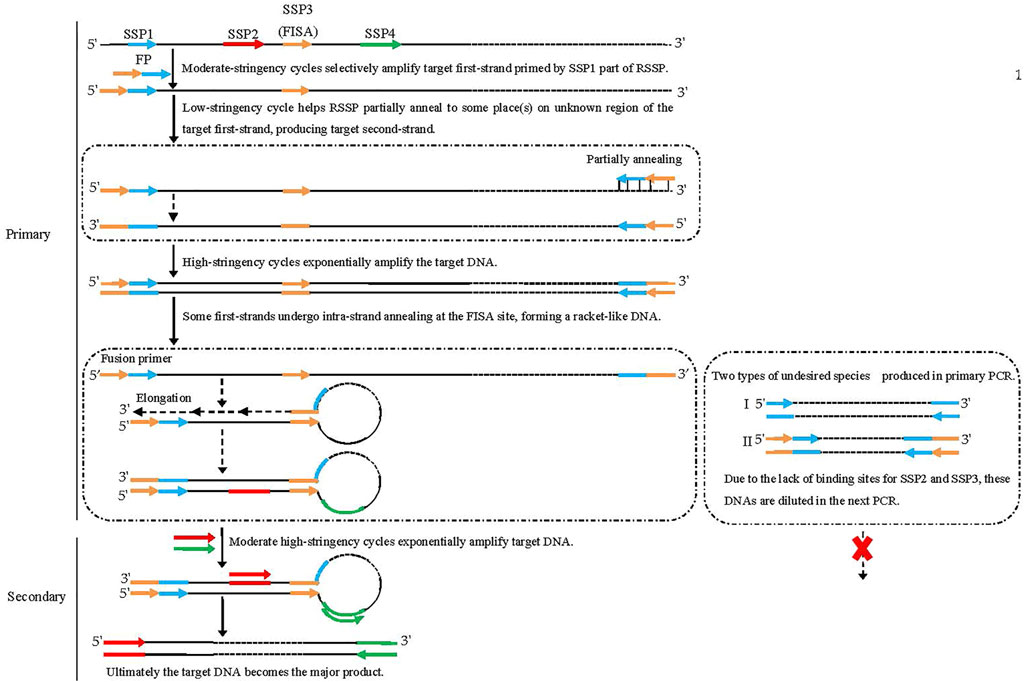
FIGURE 3. Schematic diagram of fusion primer-driven racket PCR. The fusion primer 3′-part is SSP1, while the 5′-part is the FISA fragment. Black solid lines: known sequences; black dashed lines: unknown sequences; colored solid lines with arrows: primers; colored solid lines: primers complements.
As discussed above, FPR-PCR is an effective genome-walking scheme based on its specificity, probability of success, walk length, and experimental process. Table 3 summarizes FPR-PCR and available PCR-based genome-walking methods from these important aspects.
In some situations, more than one clear target DNA band appeared (Figure 1). This multiple-band phenomenon is common in randomly primed PCRs and occurs due to the annealing of the walking primers to multiple sites on the unknown flank in the low-stringency cycle (Chang et al., 2018; Wang et al., 2022). At most two major bands appeared in each secondary FPR-PCR in the present study, much fewer than the approximately five bands reported in the other methods (Chang et al., 2018; Wang et al., 2022). As mentioned, the amplicons resulting from the primary FPR-PCR encompassed the fusion primer and its inverted repeat. These amplicons tend to form hairpins via intra-strand annealing mediated by the ends. In the secondary PCR, any minority product or short fragment derived from the primary PCR is preferentially formed into a hairpin instead of being amplified. The annoying multi-band phenomenon common in routine randomly primed PCR is, thus, significantly improved in FPR-PCR. This helps the PCR system to centralize limited resources to intensify the target product(s), improving the conciseness of the amplification result.
Amplification can occur even for oligonucleotide sequence mismatch rates of up to 60% between the walking primer and the annealing site (Parker et al., 1991). The match of the primer 3′ end is especially important for priming DNA synthesis (Parks et al., 1991). The pairing of two bases at the 3′ end of the walking primer appears to be mandatory for initiating primer extension (Parker et al., 1991; Parks et al., 1991). In the present study, the number of paired bases between fusion primers and the correspondingly partial annealing loci ranged from 14 to 19 bases, among which at least two were located at the 3′ end of the primer (Figure 1). Our results are similar to those reported previously (Parker et al., 1991; Parks et al., 1991).
Conclusion
FPR-PCR, a genome-walking approach with high efficiency, was developed to retrieve unknown flanking DNA sequences. The feasibility of the proposed method was validated by probing the unknown flanks of the gadR/C genes in L. brevis CD0817 and the hyg gene in rice. Thus, FPR-PCR is a promising alternative to the available genome-walking protocols.
Data availability statement
This study analyzed publicly available datasets. These data can be found on GenBank under accession nos. CP032931.1 and KF206149.1.
Author contributions
Investigation, methodology, and writing—original draft preparation, JP; visualization, TS; data curation, LW; project administration, ZP; formal analysis, XG; resource; supervision, conceptualization, funding acquisition, and writing—review and editing, HL All authors have read and agreed to the published version of the manuscript.
Funding
This research was funded by the National Natural Science Foundation of China (grant Nos 32160014 and 31570070) and the State Key Laboratory of Food Science and Technology at Nanchang University (grant No SKLF-ZZB-202118), and the Graduate Innovative Funding Project of Jiangxi Province (grant No YC2021-C119).
Acknowledgments
The authors thank Xiaojue Peng of Nanchang University (Nanchang, China) for providing rice genomic DNA.
Conflict of interest
The authors declare that the research was conducted in the absence of any commercial or financial relationships that could be construed as a potential conflict of interest.
Publisher’s note
All claims expressed in this article are solely those of the authors and do not necessarily represent those of their affiliated organizations, or those of the publisher, the editors, and the reviewers. Any product that may be evaluated in this article, or claim that may be made by its manufacturer, is not guaranteed or endorsed by the publisher.
Supplementary material
The Supplementary Material for this article can be found online at: https://www.frontiersin.org/articles/10.3389/fgene.2022.969840/full#supplementary-material
References
Alquezar‐Planas, D. E., Löber, U., Cui, P., Quedenau, C., Chen, W., Greenwood, A. D., et al. (2020). DNA sonication inverse PCR for genome scale analysis of uncharacterized flanking sequences. Methods Ecol. Evol. 12, 182–195. doi:10.1111/2041-210x.13497
Ashrafmansouri, S. S., Kamaladini, H., Haddadi, F., and Seidi, M. (2020). Simple innovative adaptor to improve genome walking with convenient PCR. J. Genet. Eng. Biotechnol. 18, 64. doi:10.1186/s43141-020-00082-2
Chang, K., Wang, Q., Shi, X., Wang, S., Wu, H., Nie, L., et al. (2018). Stepwise partially overlapping primer-based PCR for genome walking. Amb. Express 8, 77. doi:10.1186/s13568-018-0610-7
Fraiture, M. A., Papazova, N., and Roosens, N. H. C. (2021). DNA walking strategy to identify unauthorized genetically modified bacteria in microbial fermentation products. Int. J. Food Microbiol. 337, 108913. doi:10.1016/j.ijfoodmicro.2020.108913
Gao, D., Chang, K., Ding, G., Wu, H., Chen, Y., Jia, M., et al. (2019). Genomic insights into a robust gamma-aminobutyric acid-producer Lactobacillus brevis CD0817. Amb. Express 9, 72. doi:10.1186/s13568-019-0799-0
Ji, J., and Braam, J. (2010). Restriction site extension PCR: A novel method for high-throughput characterization of tagged DNA fragments and genome walking. PloS one 5, e10577. doi:10.1371/journal.pone.0010577
Jia, M., Zhu, Y., Wang, L., Sun, T., Pan, H., and Li, H. (2022). pH auto-sustain-based fermentation supports efficient gamma-aminobutyric acid production by Lactobacillus brevis CD0817. Fermentation 8, 208. doi:10.3390/fermentation8050208
Jia, X., Lin, X., and Chen, J. (2017). Linear and exponential TAIL-PCR: A method for efficient and quick amplification of flanking sequences adjacent to Tn5 transposon insertion sites. Amb. Express 7, 195. doi:10.1186/s13568-017-0495-x
Jones, D. H., and Winistorfer, S. C. (1997). Amplification of 4-9-kb human genomic DNA flanking a known site using a panhandle PCR variant. Biotechniques 23, 132–138. doi:10.2144/97231rr01
Jones, D. H., and Winistorfer, S. C. (1993). Genome walking with 2- to 4-kb steps using panhandle PCR. PCR Methods Appl. 2, 197–203. doi:10.1101/gr.2.3.197
Jones, D. H., and Winistorfer, S. C. (1992). Sequence specific generation of a DNA panhandle permits PCR amplification of unknown flanking DNA. Nucleic Acids Res. 20, 595–600. doi:10.1093/nar/20.3.595
Kotik, M. (2009). Novel genes retrieved from environmental DNA by polymerase chain reaction: Current genome-walking techniques for future metagenome applications. J. Biotechnol. 144, 75–82. doi:10.1016/j.jbiotec.2009.08.013
Leoni, C., Gallerani, R., and Ceci, L. R. (2008). A genome walking strategy for the identification of eukaryotic nucleotide sequences adjacent to known regions. Biotechniques 44, 232–235. doi:10.2144/000112680
Li, H., Ding, D., Cao, Y., Yu, B., Guo, L., and Liu, X. (2015). Partially overlapping primer-based PCR for genome walking. PloS one 10, e0120139. doi:10.1371/journal.pone.0120139
Li, H., Sun, T., Jia, M., Wang, L., Wei, C., Pei, J., et al. (2022). Production of gamma-aminobutyric acid by Levilactobacillus brevis CD0817 by coupling fermentation with self-buffered whole-cell catalysis. Fermentation 8, 321. doi:10.3390/fermentation8070321
Liu, Y., and Whittier, R. (1995). Thermal asymmetric interlaced PCR: Automatable amplification and sequencing of insert end fragments from P1 and YAC clones for chromosome walking. Genomics 25, 674–681. doi:10.1016/0888-7543(95)80010-j
Myrick, K. V., and Gelbart, W. M. (2002). Universal Fast Walking for direct and versatile determination of flanking sequence. Gene 284, 125–131. doi:10.1016/s0378-1119(02)00384-0
Myrick, K. V., and Gelbart, W. M. (2007). 新方法 A modified universal fast walking method for single-tube transposon mapping. Nat. Protoc. 2, 1556–1563. doi:10.1038/nprot.2007.223
Ochman, H., Gerber, A., and Hartl, D. (1988). Genetic applications of an inverse polymerase chain-reaction. Genetics 120, 621–623. doi:10.1093/genetics/120.3.621
Parker, J. D., Rabinovitch, P. S., and Burmer, G. C. (1991). 非常重要Targeted gene walking polymerase chain reaction. Nucleic Acids Res. 19, 3055–3060. doi:10.1093/nar/19.11.3055
Parks, C. L., Chang, L. S., and Shenk, T. (1991). 非常重要A polymerase chain reaction mediated by a single primer: Cloning of genomic sequences adjacent to a serotonin receptor protein coding region. Nucleic Acids Res. 19, 7155–7160. doi:10.1093/nar/19.25.7155
Sun, T., Jia, M., Wang, L., Li,, Z., Lin, Z., Wei, C., et al. (2005). DAR-PCR: A new tool for efficient retrieval of unknown flanking genomic DNA. AMB Express. doi:10.1186/s13568-022-01471-1
Tan, G., Gao, Y., Shi, M., Zhang, X., He, S., Chen, Z., et al. (2005). SiteFinding-PCR: A simple and efficient PCR method for chromosome walking. Nucleic Acids Res. 33, e122. doi:10.1093/nar/gni124
Thirulogachandar, V., Pandey, P., Vaishnavi, C. S., and Reddy, M. K. (2011). An affinity-based genome walking method to find transgene integration loci in transgenic genome. Anal. Biochem. 416, 196–201. doi:10.1016/j.ab.2011.05.021
Triglia, T., Peterson, M. G., and Kemp, D. J. (1988). A procedure for in vitro amplification of DNA segments that lie outside the boundaries of known sequences. Nucleic Acids Res. 16, 8186. doi:10.1093/nar/16.16.8186
Uchiyama, T., and Watanabe, K. (2006). Improved inverse PCR scheme for metagenome walking. Biotechniques 41, 183–188. doi:10.2144/000112210
Wang, L., Jia, M., Li, Z., Liu, X., Sun, T., Pei, J., et al. (2022). Wristwatch PCR: A versatile and efficient genome walking strategy. Front. Bioeng. Biotechnol. 10, 792848. doi:10.3389/fbioe.2022.792848
Wang, S., He, J., Cui, Z., and Li, S. (2007). Self-formed adaptor PCR: A simple and efficient method for chromosome walking. Appl. Environ. Microbiol. 73, 5048–5051. doi:10.1128/AEM.02973-06
Yan, Y., An, C., Li, L., Gu, J., Tan, G., and Chen, Z. (2003). T-linker-specific ligation PCR (T-linker PCR): An advanced PCR technique for chromosome walking or for isolation of tagged DNA ends. Nucleic Acids Res. 31, e68. doi:10.1093/nar/gng068
Yik, M. H., Lo, Y. T., Lin, X., Sun, W., Chan, T. F., and Shaw, P. C. (2021). Authentication of Hedyotis products by adaptor ligation-mediated PCR and metabarcoding. J. Pharm. Biomed. Anal. 196, 113920. doi:10.1016/j.jpba.2021.113920
Yu, D., Zhou, T., Sun, X., Sun, Z., Sheng, X., Tan, Y., et al. (2020). Cyclic digestion and ligation-mediated PCR used for flanking sequence walking. Sci. Rep. 10, 3434. doi:10.1038/s41598-020-60411-w
Zeng, T., Zhang, D., Li, Y., Li, C., Liu, X., Shi, Y., et al. (2020). Identification of genomic insertion and flanking sequences of the transgenic drought-tolerant maize line "SbSNAC1-382" using the single-molecule real-time (SMRT) sequencing method. PLoS One 15, e0226455. doi:10.1371/journal.pone.0226455
Zhang, H., Xu, W., Feng, Z., and Hong, Z. (2018). A low degenerate primer pool improved the efficiency of high-efficiency thermal asymmetric interlaced PCR to amplify T-DNA flanking sequences in Arabidopsis thaliana. 3 Biotech. 8, 14. doi:10.1007/s13205-017-1032-y
Keywords: fusion primer, walking primer, partial annealing, racket-like DNA, genome-walking
Citation: Pei J, Sun T, Wang L, Pan Z, Guo X and Li H (2022) Fusion primer driven racket PCR: A novel tool for genome walking. Front. Genet. 13:969840. doi: 10.3389/fgene.2022.969840
Received: 16 June 2022; Accepted: 26 September 2022;
Published: 18 October 2022.
Edited by:
Youri I. Pavlov, University of Nebraska Medical Center, United StatesReviewed by:
Birgit Henrich, Heinrich Heine University of Düsseldorf, GermanyKazutoshi Okuno, University of Tsukuba, Japan
Copyright © 2022 Pei, Sun, Wang, Pan, Guo and Li. This is an open-access article distributed under the terms of the Creative Commons Attribution License (CC BY). The use, distribution or reproduction in other forums is permitted, provided the original author(s) and the copyright owner(s) are credited and that the original publication in this journal is cited, in accordance with accepted academic practice. No use, distribution or reproduction is permitted which does not comply with these terms.
*Correspondence: Haixing Li, aHhsaUBuY3UuZWR1LmNu