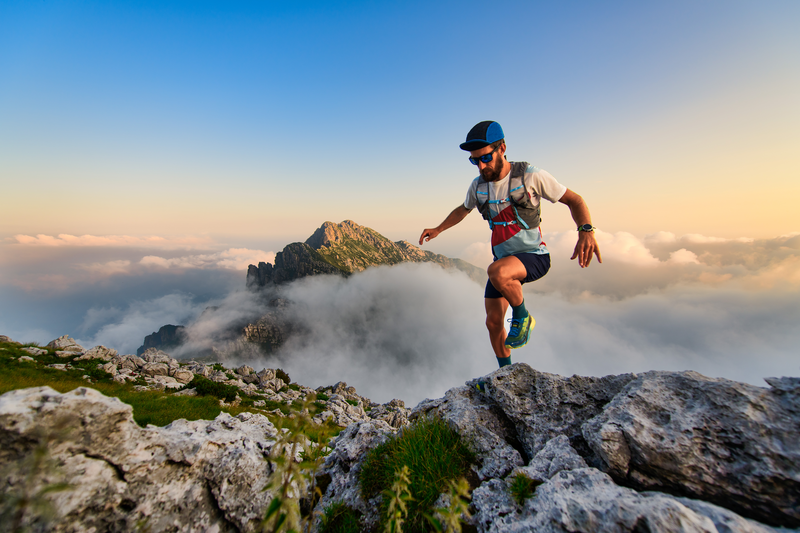
94% of researchers rate our articles as excellent or good
Learn more about the work of our research integrity team to safeguard the quality of each article we publish.
Find out more
ORIGINAL RESEARCH article
Front. Genet. , 17 August 2022
Sec. Livestock Genomics
Volume 13 - 2022 | https://doi.org/10.3389/fgene.2022.969752
This article is part of the Research Topic Technologies in Smallholder Poultry Development: Characterization, Utilization, Conservation, and Improvement View all 11 articles
Feather pecking in chickens is a damaging behavior, seriously impacting animal welfare and leading to economic losses. Feather pecking is a complex trait, which is partly under genetic control. Different hypotheses have been proposed to explain the etiology of feather pecking and notably, several studies have identified similarities between feather pecking and human mental disorders such as obsessive-compulsive disorder and schizophrenia. This study uses transcriptomic and phenotypic data from 167 chickens to map expression quantitative trait loci and to identify regulatory genes with a significant effect on this behavioral disorder using an association weight matrix approach. From 70 of the analyzed differentially expressed genes, 11,790 genome wide significantly associated variants were detected, of which 23 showed multiple associations (≥15). These were located in proximity to a number of genes, which are transcription regulators involved in chromatin binding, nucleic acid metabolism, protein translation and putative regulatory RNAs. The association weight matrix identified 36 genes and the two transcription factors: SP6 (synonym: KLF14) and ENSGALG00000042129 (synonym: CHTOP) as the most significant, with an enrichment of KLF14 binding sites being detectable in 40 differentially expressed genes. This indicates that differential expression between animals showing high and low levels of feather pecking was significantly associated with a genetic variant in proximity to KLF14. This multiallelic variant was located 652 bp downstream of KLF14 and is a deletion of 1-3 bp. We propose that a deletion downstream of the transcription factor KLF14 has a negative impact on the level of T cells in the developing brain of high feather pecking chickens, which leads to developmental and behavioral abnormalities. The lack of CD4 T cells and gamma-Aminobutyric acid (GABA) receptors are important factors for the increased propensity of laying hens to perform feather pecking. As such, KLF14 is a clear candidate regulator for the expression of genes involved in the pathogenic development. By further elucidating the regulatory pathways involved in feather pecking we hope to take significant steps forward in explaining and understanding other mental disorders, not just in chickens.
Feather pecking (FP) behavior in chickens is a serious issue, which has an impact on animal welfare, where damage to the feathers, bodily injuries and even death can occur (Hughes and Duncan, 1972; Allen and Perry, 1975). As well as this negative impact on animal welfare, there is also a financial impact for the chicken producer, through the increased animal care costs, and ultimately with the loss of injured animals (Huber-eicher and Wechsler, 1997). FP is a trait observed in chickens that are kept in all types of housing (Appleby and Hughes, 1991). Previous attempts to manage this disorder have seen producers utilizing beak trimming, a process that is now being banned in more and more countries due to its impact on the welfare of individual animals (Duncan et al., 1989; Gentle et al., 1990).
Some studies have hypothesized that pecking at the plumage of other birds is related to foraging behavior and occurs due to the low incentive value of floors without litter, (Hoffmeyer, 1969; Blokhuis, 1986; Huber-eicher and Wechsler, 1997), while others have indicated links with behavioral traits such as general locomotor activity (GLA), and fearfulness (Vestergaard et al., 1993; Kjaer, 2017). This suggests that the reasons underpinning FP are associated with both environmental and genetic factors, with a number of studies indicating the possibility of reducing FP by means of breeding, to select for low FP (Kjaer and Sørensen, 1997; Rodenburg et al., 2003; Bennewitz et al., 2014). Quantitative trait loci (QTL) mapping studies and genome wide association studies (GWAS) confirmed the assumption that this trait is polygenically determined with some trait-associated chromosomal regions (Buitenhuis et al., 2003; Lutz et al., 2017; Falker-Gieske et al., 2020a).
There have been a number of studies undertaken to attempt to describe the underlying processes of FP, using gene expression analysis and mapping studies to link FP to immune response and multiple signaling pathways (Wysocki et al., 2010; Haas and van der Eijk, 2018), with some studies linking FP to schizophrenia and obsessive-compulsive disorders, indicating its use as a potential model for these diseases (Falker-Gieske et al., 2021). However, these studies have so far been unable to elucidate the exact mechanism for this feather pecking.
Here the transcriptomic and phenotypic data from 167 chickens was analyzed through a comprehensive strategy combining expression quantitative trait loci (eQTL) mapping and the network analysis of gene-gene interactions to predict SNPs and regulatory pathways that had significant impacts on these abnormal behavior patterns. It is also hoped that the data presented here can be used as a possible model system for both obsessive-compulsive disorder and schizophrenia, due to the similarities of the disease (Falker-Gieske et al., 2021). As such, further elucidating the pathways involved in FP could prove significant in explaining and understanding other mental disorders, not just in chickens.
The samples used in the following study were collected as previously described in Falker-Gieske et al. (2020a); Falker-Gieske et al., 2020b). Briefly, White Leghorn strains were selected for more than ten generations based on estimated breeding values for feather pecking (Grams et al., 2015). These lines were created and are maintained at the Hohenheim University and neither commercially obtained nor from a private source, with rearing and husbandry conditions being previously described (Bennewitz et al., 2014; Falker-Gieske et al., 2020b). A total of 492 birds from two experimental runs were separated into 7 groups of 39–42 birds (ratio of 1:1 LFP-HFP), and 6 groups of 39–42 birds (ratio of 1:2 LFP-HFP) (Iffland et al., 2020b). At 31–33 weeks, the hens, were phenotyped according to established ethograms. In short, feather pecking [feather pecks delivered (FPD), and feather pecks received (FPR)], and aggressive behavior [aggressive pecks delivered (APD) and aggressive pecks received (APR)], were recorded and hens were marked with numbered plastic batches on their backs to aid in identification (Lutz et al., 2017; Falker-Gieske et al., 2020a; Iffland et al., 2020a). Observation of FP behavior was done in 20-min sessions on four consecutive days by a minimum of six different trained observers. To prevent FP, birds were kept under low light conditions. One bird from each full-sib pair kept under dark conditions was sacrificed, and due to time constraints, the whole brains were immediately collected for RNA isolation in order to preserve expression levels. Chickens were CO2-stunned and sacrificed by ventral neck cutting. For light stimulation, the remaining birds were kept under increased light intensity (≥100 lux) for several hours. Upon initiation of FP behavior these birds were then sacrificed and brains were collected for RNA isolation. 48 birds were utilized for the transcriptomic analysis described previously (Falker-Gieske et al., 2020b), with 167 animals in total (the previously mentioned 48 animals plus a further 119 animals) being collected and utilized in this study.
cDNA needed for the HT-qPCR analysis was generated from selected high feather pecking (HFP) and low feather pecking (LFP) animals. RNA was extracted using an RNeasy kit (Qiagen, Hilden, Germany) as per the provided protocol. cDNA preparation was then performed using the Fluidigm Reverse Transcription assay kit (Fluidigm Corporation, San Francisco, CA, United States).
In order to quantitatively analyze the gene expression in feather pecking, HT-qPCR analysis was performed for 86 differentially expressed genes (DEGs) highlighted by our previous transcriptomic study (Falker-Gieske et al., 2020b). A Fluidigm BioMark system, using a 96.96 Dynamic Array integrated fluidic circuit for gene expression (IFC; Fluidigm Corporation, San Francisco, CA, United States), and Delta Gene Assays (Fluidigm Corporation, San Francisco, CA, United States) were used, and delta Ct values were obtained by normalization against GAPDH. The primers utilised in this study can be found in the Supplementary Material S1
Whole genome sequencing genotypes mapped to chicken reference assembly GRCg6a (GCF_000002315.5 RefSeq assembly) from our previous study (Falker-Gieske et al., 2020a) were used for expression genome wide associations studies (eGWAS). Imputation from 60k chip genotypes to whole genome sequence level was performed with Beagle 5.2 (Browning et al., 2018) to make use of the latest imputation algorithm and with the setting ne = 1000 to factor in the small effective population size. Since the default setting is ne = 1000000 we adjusted the setting according to conclusions of Pook et al. who showed that this improves imputation accuracy in small populations (Pook et al., 2020). eGWAS were performed with gcta v. 1.92.3 beta3 (Yang et al., 2011) with the setting maf 0.01 and hatch as covariate. Population structure was accounted for in the linear mixed model based on the GRM, which is included in the analysis.
An association weight matrix (AWM) utilizes the results of multiple GWAS as the basis for calculation of gene-gene or gene-variant associations. Followed by the application of network inference algorithms it generates gene networks with regulatory and functional significance. Input variants for the AWM were selected to contain only highly associated signals, either with the main phenotype (feather pecks delivered box-cox-transformed, FPD_BC) or multiple of the gene expression phenotypes. Since the AWM analysis was designed for SNP chip data, stringent pre-filtering of whole genome sequencing data is necessary to produce a compatible input dataset. Therefore, variants with a p-value < 1 × 10–5 for the main phenotype or variants that were associated (p < 1 × 10–5) with at least ten of the gene expression phenotypes were retained. This resulted in the selection of 2,753 variants, which represent 0.042% of all genome wide variants that were studied. Distances up to 100 kb to the closest genes for each variant were predicted with the Ensembl Variant Effect Predictor (Assembly: GRCg6a, accessed Jan. 13th 2022). The AWM was constructed with an established method by Reverter and Fortes (Reverter and Fortes, 2013). The analysis was performed with the following settings: the p-value thresholds for primary SNP selection and selection of non-key phenotype SNPs were set to 5 × 10–6, the p-value threshold for secondary SNP selection was set to 1 × 10–9. SNP based variants with a distance ≤ 2,500 bp to the closest gene were considered close and variants with a distance ≥ 20,000 bp were considered far. Associations between SNP based variants and phenotypes, the gene expression values for DEGs between high feather peckers (HFP) and low feather peckers (LFP), were analyzed with the partial correlation and information (PCIT) algorithm (Reverter and Chan, 2008) to retrieve significant associations between the closest genes to SNP based variants and DEGs. The analysis with the PCIT algorithm results in pairs of genes, which are predicted to interact. The gene-gene interaction network was visualized with Cytoscape (Shannon et al., 2003) and gene ontology classifications were assigned with PANTHER v. 16.0 (Mi et al., 2017). Genes, which were not annotated by PANTHER were manually annotated using information provided on the UniProt website (accessed March 2022) (UniProt: the universal protein knowledgebase in 2021., 2021).
Transcription factor enrichment analysis was performed with CiiiDER (build 15 May 2020) (Gearing et al., 2019). Genes that reached genome wide significance (p-value < 7.6 × 10–9) for at least one variant associated with the transcription factor were used as input. Background genes with an absolute log2 fold-change (abs. LFC) < 0.5 were selected from the differential expression analysis results from our previous study (Falker-Gieske et al., 2020b), which resulted in a set of 19,088 genes. The p-value threshold for gene coverage enrichment was set to 0.05, base position upstream scan limit to 1,500 bp, and base position downstream scan limit to 500 bp. To predict transcription factor binding to a target DNA sequence, a position frequency matrix (PFM) is required, which assigns nucleotide frequencies to each position in the binding motif. For the analysis of SP6 (synonym: KLF14) the PFM MA0740.1 (https://testjaspar.uio.no/matrix/MA0740.1/) was used. Additionally the PFMs of all KLF transcriptions factors available on the Jaspar homepage have been included in the analysis, namely: KLF1 (MA0493.2), KLF10 (MA1511.2), KLF11 (MA1512.1), KLF12 (MA0742.2), KLF13 (MA0657.1), KLF15 (MA1513.1), Klf15 (MA 1963.1), KLF16 (MA0741.1), KLF2 (MA1515.1), KLF3 (MA1516.1), Klf3/8/12 (MA 1964.1), KLF4 (MA0039.4), KLF5 (MA0599.1), Klf5-like (MA 1965.1), KLF6 (MA1517.1), Klf6-7-like (MA 1966.1), KLF7 (MA 1870.1), and KLF9 (MA1107.2). Binding sites of ENSGALG00000042129 (synonym: CHTOP) were screened with PFM MA1153.1 (https://jaspar.genereg.net/matrix/MA1153.1/). All PFMs were acquired in JASPAR format (Castro-Mondragon et al., 2022). The transcription factor enrichment plot was created with CiiiDER.
Conducting an eGWAS on 86 genes that were differentially expressed between 167 chickens (84 HFP and 83 LFP, normalized gene expression results in Supplementary Material S2) yielded Manhattan plots with prominent peaks for the genes AvBD4, BTN3A3L2, CHDSD, KIFC1, LOC769512, LOC770352, LOC112530399, LOC112531493, and MUC4 (Figure 1, Manhattan plots for all differentially expressed genes (DEGs) in Supplementary Material S3). QTL for these eGWAS are shown in Supplementary Material S4. We discovered 41 genome wide significant variants in proximity to (<1 kb distance to gene start or gene end) the DEGs AvBD4, LOC769512, and MUC4 (Supplementary Material S5), which are candidates for being cis-regulatory elements. Although QTL regions were detected through the eGWAS, the use of an associated weight matrix allowed for the further recovery of associated variants from this data set. In total 11,790 genome wide significant associations (Bonferroni threshold p-value = 7.6 × 10–9) for 70 of the analyzed DEGs were detected, of which 9,677 were unique. Stringent filtering (p-value < 1 × 10–8 in more than 15 eGWAS) was applied, which yielded 23 highly associated variants (Table 1). Due to the nature of an eQTL study one would expect to also discover trans-regulatory elements and regulators of transcription to be associated with DEGs (Gilad et al., 2008). Unsurprisingly, the PANTHER Classification System revealed KLF14 and CHTOP belong to the protein class “gene-specific transcriptional regulator (PC00264)”, ASH1L to the class “chromatin/chromatin-binding, or -regulatory protein (PC00077)”, ENSGALG00000042129 to the class “nucleic acid metabolism protein (PC00171)”, and DAP3 to the class “translational protein (PC00263)”. Furthermore, as in previous studies we found putative regulatory RNAs, namely long non-coding RNAs (lncRNAs) among the top associated genes: ENSGALG00000050482, ENSGALG00000048366, ENSGALG00000046959, and ENSGALG00000054815.
FIGURE 1. Manhattan plots of expression genome wide association studies (eGWAS) that yielded highly significant QTL, conducted on genes differentially expressed between high and low feather pecking hens (Bonferroni threshold
TABLE 1. Genomic variants with a p-value < 1 × 10–8 in more than 15 expression genome wide association studies (eGWAS). Only one variant per gene is shown since they represent the respective associated haplotype block. Closest genes up to a distance of 100 kb were considered.
To further analyze the dataset at hand we created an AWM using highly associated eGWAS signals (p-value < 1 × 10–5 for the main phenotype (FPD_BC) or p-value < 1 × 10–5 in at least 10 eGWAS) from the 86 DEGs that were analyzed in this study. Significant associations between genes that are close to variants that indicated significant association to DEGs between HFP and LFP were identified with the PCIT algorithm (Reverter and Chan, 2008), which detects meaningful gene-gene associations in co-expression networks. This led to the discovery of 34 genes based on 2,753 input variants, which we visualized in a gene association interaction map, where nodes located at the center of the network indicates their centrality in the interaction cluster (Figure 2). Among these were four genes, which were associated with at least 30 DEGs (Table 1): ASH1L, KLF14, NUP214, and CHTOP, all of which are involved in gene transcription or translation.
FIGURE 2. Significant gene-gene interactions, which were discovered with an association weight matrix followed by analysis with the PCIT algorithm. Input genes were derived from expression genome wide association studies with differentially expressed genes between high and low feather pecking chickens as phenotypes.
Since KLF14 and CHTOP are transcription factors, we decided to conduct transcription factor binding site enrichment analysis to clarify if DEGs, which were significantly associated with KLF14 or CHTOP, are potential targets of these transcription factors. NCBI Conserved Domain Search (Marchler-Bauer et al., 2015) revealed that CHTOP contains an N-terminal Mad Homology 1 (MH1) domain as in the transcription factor SMAD4, which recognizes the palindromic DNA sequence GTCTAGAC. Transcription factor binding site enrichment analysis of putative target genes that were associated with genome wide significance (p-value < 7.6 × 10–9) yielded no significant result for CHTOP with the SMAD4 recognition site. eGWAS revealed that 40 of the DEGs (Supplementary Material S6) between HFP and LFP were significantly associated with a multiallelic variant located 652 bp downstream of KLF14 (Chromosome: 27 Position: 6521246 bp) and which is a deletion of 1–3 bp. An enrichment of KLF14 binding sites (Figure 3A) was detectable in those 40 genes (Figure 3B). To ensure, that this enrichment is significant in relation to other transcription factor binding sites, all available PFMs for KLF transcription factors were included in the analyses with the complete results summarized in Supplementary Material S7. Although significant enrichment of binding sites for other KLFs were detected, KLF14 resulted in the highest log2 enrichment value, while being statistically significant.
FIGURE 3. (A) Graphical representation of the position frequency matrix of the KLF14 transcription factor. (B) Result of transcription factor binding site enrichment analysis for KLF14 with genes from expression genome wide associations studies with genome wide significant association. All available KLF transcription factors were included as controls for the specificity of KLF14 binding site enrichment.
Out of those 40 putative KLF14 regulated target genes 10 are involved in the immune system with the majority being leukocyte-immunoglobulin receptors (Table 2).
TABLE 2. Differentially expressed genes between high and low feather peckers significantly associated with KLF14, which are involved in the immune system. Obsolete gene Symbols, due to the recent release of new genome assemblies, are shown in parentheses.
To untangle the regulatory network behind feather pecking behavior we conducted an eQTL study on 86 genes that were differentially expressed between divergently selected HFP and LFP (Falker-Gieske et al., 2020b) in 167 chickens. The main result of this study is a gene-gene interaction map based on an AWM, which revealed two clusters of interacting genes (Figure 2). A number of those genes have previously been described to be involved with brain-related functions and have generally not been detected by simple filtering based on eGWAS p-values (Table 1). The DMD gene encodes the protein dystrophin, which colocalizes with GABAA receptors in postsynaptic densities of neurons in the cerebral cortex of mice. Dystrophin deficient mice show an altered clustering of GABAA receptors (Knuesel et al., 1999). We previously reported that mutations within or close to GABA receptors as well as differential expression of GABRA2, GABRB2, GABRE, and GABRG3 are associated with feather pecking (Falker-Gieske et al., 2021). The central position of DMD in the gene-gene interaction network provides further evidence for our theory that feather pecking is a GABAergic dysfunction disorder [already discussed here (Falker-Gieske et al., 2021)]. A lack of GABA receptor expression has been linked to schizophrenia in humans [reviewed here (Chiapponi et al., 2016)]. In previous studies we found a considerable amount of genes involved in schizophrenia to be associated with feather pecking (Falker-Gieske et al., 2020a; Falker-Gieske et al., 2020b). The results of the AWM extend this list with PPP1R9A, which encodes the protein Neurabin-1 and has been linked to dendritic spine loss in schizophrenia (Konopaske et al., 2015). TAMALIN, a trafficking molecule of Metabotropic glutamate receptor 5 (mGluR5), showed increased expression in the hippocampal region of individuals with schizophrenia (Matosin et al., 2015). Furthermore, we discovered INPP4A, a gene linked to schizophrenia (Föcking et al., 2015), epilepsy (Wang et al., 2012), and intellectual disability (Banihashemi et al., 2020) as well as RIMS4, which is involved in synaptic plasticity and the development of autism (Leblond et al., 2019). Genes that have been classified as protein modifiers in the gene-gene interaction map have also been implicated in neurological disorders. A LIPT1 deficiency has been reported in a case of early infantile epileptic encephalopathy (Stowe et al., 2018). Low expression of MGAT4A in the dorsolateral prefrontal cortex was reported in cases of schizophrenia (Kippe et al., 2015). We previously proposed that chickens selected for FP behavior could serve as a model for human psychiatric disorders (Falker-Gieske et al., 2021). In this respect Johnsson et al. conducted a study on an intercross between domestic chickens and Red Junglefowl focusing on the suitability of chickens as a model for anxiety behavior. Here, two genes also discovered in our study LOC770352 and GABRB2, were identified as top candidate genes affecting stress and anxiety behavior (Johnsson et al., 2016). As this study did not use selection lines, their findings indicate that results derived from selection lines are clearly relevant in the general production populations. Furthermore, these common findings of homologous genes such as SP6/KLF14, GABRB2 and LOC770352 underlines the suitability of chickens as model organisms for behavioral disorders of the brain.
With AvBD4, LOC769512, and MUC4 we discovered three potential cis-regulated DEGs in eGWAS. However, from a regulatory point of view those genes identified by the PCIT algorithm that are involved in transcription and translation are the most relevant. Variants in the NUP214 gene cause acute febrile encephalopathy (Fichtman et al., 2019) and rare mutations in ASH1L are connected to numerous neurodevelopmental disorders [reviewed in (Zhang et al., 2021)]. Furthermore, among those genes were two transcription factors - KLF14 and CHTOP. Whilst we found the presence of a missense mutation in CHTOP, leading to an alanine being replaced with a valine, no significance in the enrichment analysis was observed. Dysregulation at the cellular level of CHTOP has been shown however, to play an important role in the tumorigenicity of glioblastoma cells (van Dijk et al., 2010b), as well as being a critical regulator of γ-globin gene expression (van Dijk et al., 2010a). Although very little is still known about the molecular mechanism of transcriptional control that it mediates, it has also been associated with the methylosome complex containing PRMT1, PRMT5, MEP50, and ERH, which are critical for mammalian development through transcription regulation, DNA repair, RNA splicing, and signal transduction (Fanis et al., 2012; Liang et al., 2021) (Fanis et al., 2012). In fact, Hannon et al. identified robust psychosis associated differences derived from DNA methylation, with increased proportions of monocytes and granulocytes and decreased proportions of natural killer cells, CD4+ T-cells and CD8+ T-cells (Hannon et al., 2021).
Genome-wide significant associations with a novel deletion downstream of KLF14 were detected in 40 of the 86 DEGs that we screened in the eGWAS. A number of which were associated with natural killer (NK) cells, where major dysfunctions have been linked with functioning impairment correlated with psychotic, manic, and depressive symptoms in subsequently diagnosed patients with schizophrenia and bi-polar disorder (Tarantino et al., 2021). Transcription factor binding sites for KLF14 were significantly enriched in those 40 genes (Figure 3B). Hence, we propose that KLF14 is a candidate regulator for the expression of genes that are involved in the pathogenic development that leads to feather pecking behavior. 10 of the 40 potential KLF14 targets play a role in the immune system, more specifically in leukocytes. CD4 T cells belong to the generic term leukocytes and have recently been shown to be essential for healthy development from the fetal to the adult brain in mice and humans (Pasciuto et al., 2020). Single-cell sequencing revealed that in the absence of murine CD4 T cells, resident microglia remained suspended between the fetal and adult states. This maturation defect resulted in excess immature neuronal synapses and behavioral abnormalities. The authors proposed that CD4 T cells play a so far neglected role in the development and evolution of the neurological system. An involvement of the immune system in feather pecking behavior has been proposed in numerous studies (Parmentier et al., 2009; Mashimo et al., 2017; Gandal et al., 2018; Sneeboer et al., 2019; van der Eijk et al., 2019; Falker-Gieske et al., 2020b; Falker-Gieske et al., 2021). Interestingly van der Eijk et al. showed that a HFP line expressed significantly lower amounts of CD4, CD4+ and CD8α+ T cells in comparison to an LFP line (van der Eijk et al., 2019). This would mirror results in human studies that also showed decreased proportions of natural killer cells, CD4+ T-cells and CD8+ T-cells in psychosis-associated patients (Hannon et al., 2021).
Although these analyses have been conducted in adult chickens it is fair to assume that lower T cell counts are also present during the embryonic development of HFP. The tissue expression of KLF14 is by far highest in human placenta, 4-fold higher compared to skin (Fagerberg et al., 2014). As such, we submit a possible model in which a deletion downstream of the transcription factor KLF14 has a negative impact on the level of T cells in the developing brain of HFP chickens, leading to developmental and behavioral abnormalities. As the evidence of the GABAergic systems involvement mounts, we currently believe that a lack of CD4 T cells and GABA receptors are major contributors of the propensity to perform feather pecking behavior in laying hens. Using transcriptional and computational analysis brings its own limits to the outcome of this study. Here we have put forward a solid hypothesis for the role of KLF14. It is however important, to understand that without further practical analysis this cannot be proven. Through further quantification of CD4 T cells and GABA receptors in HFP and LFP animals, throughout multiple stages of development, it could be possible to elucidate the role that KLF14 plays in FP. Further analysis of other neurological disorder looking at the role KLF14 plays there, could also open the door for the use of HFP animals as a model to study other neurological disorders.
We propose that a deletion downstream of the transcription factor KLF14 has a negative impact on the level of T cells in the developing brain of FP chickens, which leads to developmental and behavioral abnormalities. This reduction of CD4 T cells and GABA receptors are a major cause for the propensity of laying hens to perform feather pecking behavior. As such, KLF14 is a clear candidate key regulator for the expression of genes involved in the pathogenic development. By further elucidating the regulatory pathways involved in FP we hope to take significant steps forward in explaining and understanding other mental disorders, not just in chickens.
The datasets presented in this study can be found in online repositories. The names of the repository/repositories and accession number(s) can be found below: https://www.ebi.ac.uk/ena, PRJNA664592 https://www.ebi.ac.uk/ena, PRJNA656654.
The animal study was reviewed and approved by The animal treatment was approved by the German Ethical Commission of Animal Welfare of the Provincial Government of Baden-Wuerttemberg, Germany (code: HOH 35/15 PG, date of approval: 25 April 2017).
AM designed primers, analysed expression data, and wrote the manuscript. AM optimized primers. SP performed the Fluidigm experiments. JT and JB developed the project outline. JT developed data analysis strategies, and contributed to the manuscript. CF-G performed all bioinformatics and statistical analyses and wrote the manuscript. All authors have read and approved the manuscript.
The study was funded by the German Research Foundation (DFG) under file numbers TE622/4–2 and BE3703/8–2. The funders had no role in study design, data collection and analysis, and interpretation of data and in writing the manuscript. Publication fee was covered by the Open Access Publication Funds of the Göttingen University. We acknowledge support by the Open Access Publication Funds of the Göttingen University.
The authors declare that the research was conducted in the absence of any commercial or financial relationships that could be construed as a potential conflict of interest.
All claims expressed in this article are solely those of the authors and do not necessarily represent those of their affiliated organizations, or those of the publisher, the editors and the reviewers. Any product that may be evaluated in this article, or claim that may be made by its manufacturer, is not guaranteed or endorsed by the publisher.
The Supplementary Material for this article can be found online at: https://www.frontiersin.org/articles/10.3389/fgene.2022.969752/full#supplementary-material
Allen, J., and Perry, G. C. (1975). Feather pecking and cannibalism in a caged layer flock. Br. Poult. Sci. 16, 441–451. doi:10.1080/00071667508416212
Appleby, M. C., and Hughes, B. O. (1991). Welfare of laying hens in cages and alternative systems: Environmental, physical and behavioural aspects. World's. Poult. Sci. J. 47, 109–128. doi:10.1079/WPS19910013
Banihashemi, S., Tahmasebi-Birgani, M., Mohammadiasl, J., and Hajjari, M. (2020). Whole exome sequencing identified a novel nonsense INPP4A mutation in a family with intellectual disability. Eur. J. Med. Genet. 63, 103846. doi:10.1016/j.ejmg.2020.103846
Bennewitz, J., Bögelein, S., Stratz, P., Rodehutscord, M., Piepho, H. P., Kjaer, J. B., et al. (2014). Genetic parameters for feather pecking and aggressive behavior in a large F2-cross of laying hens using generalized linear mixed models. Poult. Sci. 93, 810–817. doi:10.3382/ps.2013-03638
Blokhuis, H. J. (1986). Feather-pecking in poultry: Its relation with ground-pecking. Appl. Anim. Behav. Sci. 16, 63–67. doi:10.1016/0168-1591(86)90040-7
Browning, B. L., Zhou, Y., and Browning, S. R. (2018). A one-penny imputed genome from next-generation reference panels. Am. J. Hum. Genet. 103, 338–348. doi:10.1016/j.ajhg.2018.07.015
Buitenhuis, A. J., Rodenburg, T. B., van Hierden, Y. M., Siwek, M., Cornelissen, S. J. B., Nieuwland, M. G. B., et al. (2003). Mapping quantitative trait loci affecting feather pecking behavior and stress response in laying hens. Poult. Sci. 82, 1215–1222. doi:10.1093/ps/82.8.1215
Castro-Mondragon, J. A., Riudavets-Puig, R., Rauluseviciute, I., Berhanu Lemma, R., Turchi, L., Blanc-Mathieu, R., et al. (2022). Jaspar 2022: the 9th release of the open-access database of transcription factor binding profiles. Nucleic Acids Res. 50, D165–D173. doi:10.1093/nar/gkab1113
Chiapponi, C., Piras, F., Piras, F., Caltagirone, C., and Spalletta, G. (2016). GABA system in schizophrenia and mood disorders: A mini review on third-generation imaging studies. Front. Psychiatry 7, 61. doi:10.3389/fpsyt.2016.00061
Duncan, I. J., Slee, G. S., Seawright, E., and Breward, J. (1989). Behavioural consequences of partial beak amputation (beak trimming) in poultry. Br. Poult. Sci. 30, 479–488. doi:10.1080/00071668908417172
Fagerberg, L., Hallström, B. M., Oksvold, P., Kampf, C., Djureinovic, D., Odeberg, J., et al. (2014). Analysis of the human tissue-specific expression by genome-wide integration of transcriptomics and antibody-based proteomics. Mol. Cell. Proteomics. 13, 397–406. doi:10.1074/mcp.M113.035600
Falker-Gieske, C., Bennewitz, J., and Tetens, J. (2021). The light response in chickens divergently selected for feather pecking behavior reveals mechanistic insights towards psychiatric disorders. Mol. Biol. Rep. 49 (2), 1649–1654. doi:10.1007/s11033-021-07111-4
Falker-Gieske, C., Iffland, H., Preuß, S., Bessei, W., Drögemüller, C., Bennewitz, J., et al. (2020a). Meta-analyses of genome wide association studies in lines of laying hens divergently selected for feather pecking using imputed sequence level genotypes. BMC Genet. 21, 114. doi:10.1186/s12863-020-00920-9
Falker-Gieske, C., Mott, A., Preuß, S., Franzenburg, S., Bessei, W., Bennewitz, J., et al. (2020b). Analysis of the brain transcriptome in lines of laying hens divergently selected for feather pecking. BMC Genomics 21, 595. doi:10.1186/s12864-020-07002-1
Fanis, P., Gillemans, N., Aghajanirefah, A., Pourfarzad, F., Demmers, J., Esteghamat, F., et al. (2012). Five friends of methylated chromatin target of protein-arginine-methyltransferaseprmt-1 (chtop), a complex linking arginine methylation to desumoylation. Mol. Cell. Proteomics. 11, 1263–1273. doi:10.1074/mcp.M112.017194
Fichtman, B., Harel, T., Biran, N., Zagairy, F., Applegate, C. D., Salzberg, Y., et al. (2019). Pathogenic variants in NUP214 cause "plugged" nuclear pore channels and acute febrile encephalopathy. Am. J. Hum. Genet. 105, 48–64. doi:10.1016/j.ajhg.2019.05.003
Föcking, M., Lopez, L. M., English, J. A., Dicker, P., Wolff, A., Brindley, E., et al. (2015). Proteomic and genomic evidence implicates the postsynaptic density in schizophrenia. Mol. Psychiatry 20, 424–432. doi:10.1038/mp.2014.63
Gandal, M. J., Zhang, P., Hadjimichael, E., Walker, R. L., Chen, C., Liu, S., et al. (2018). Transcriptome-wide isoform-level dysregulation in ASD, schizophrenia, and bipolar disorder. Science 362, eaat8127. doi:10.1126/science.aat8127
Gearing, L. J., Cumming, H. E., Chapman, R., Finkel, A. M., Woodhouse, I. B., Luu, K., et al. (2019). CiiiDER: a tool for predicting and analysing transcription factor binding sites. PLoS ONE 14, e0215495. doi:10.1371/journal.pone.0215495
Gentle, M. J., Waddington, D., Hunter, L. N., and Jones, R. (1990). Behavioural evidence for persistent pain following partial beak amputation in chickens. Appl. Anim. Behav. Sci. 27, 149–157. doi:10.1016/0168-1591(90)90014-5
Gilad, Y., Rifkin, S. A., and Pritchard, J. K. (2008). Revealing the architecture of gene regulation: The promise of eQTL studies. Trends Genet. 24, 408–415. doi:10.1016/j.tig.2008.06.001
Grams, V., Wellmann, R., Preuß, S., Grashorn, M. A., Kjaer, J. B., Bessei, W., et al. (2015). Genetic parameters and signatures of selection in two divergent laying hen lines selected for feather pecking behaviour. Genet. Sel. Evol. 47, 77. doi:10.1186/s12711-015-0154-0
Haas, E. N. de, and van der Eijk, J. A. J. (2018). Where in the serotonergic system does it go wrong? Unravelling the route by which the serotonergic system affects feather pecking in chickens. Neurosci. Biobehav. Rev. 95, 170–188. doi:10.1016/j.neubiorev.2018.07.007
Hannon, E., Dempster, E. L., Mansell, G., Burrage, J., Bass, N., Bohlken, M. M., et al. (2021). DNA methylation meta-analysis reveals cellular alterations in psychosis and markers of treatment-resistant schizophrenia. Elife 10, e58430. doi:10.7554/eLife.58430
Hoffmeyer, I. (1969). Feather pecking in pheasants: An ethological approach to the problem. Kalø Denmark: Vildtbiologisk Station.
Huber-eicher, B., and Wechsler, B. (1997). Feather pecking in domestic chicks: Its relation to dustbathing and foraging. Anim. Behav. 54, 757–768. doi:10.1006/anbe.1996.0506
Hughes, B. O., and Duncan, I. J. (1972). The influence of strain and environmental factors upon feather pecking and cannibalism in fowls. Br. Poult. Sci. 13, 525–547. doi:10.1080/00071667208415981
Iffland, H., Wellmann, R., Preuß, S., Tetens, J., Bessei, W., Piepho, H.-P., et al. (2020a). A novel model to explain extreme feather pecking behavior in laying hens. Behav. Genet. 50, 41–50. doi:10.1007/s10519-019-09971-w
Iffland, H., Wellmann, R., Schmid, M., Preuß, S., Tetens, J., Bessei, W., et al. (2020b). Genomewide mapping of selection signatures and genes for extreme feather pecking in two divergently selected laying hen lines. Animals. 10, 262. doi:10.3390/ani10020262
Johnsson, M., Williams, M. J., Jensen, P., and Wright, D. (2016). Genetical Genomics of behavior: a novel chicken genomic model for anxiety behavior. Genetics 202, 327–340. doi:10.1534/genetics.115.179010
Kippe, J. M., Mueller, T. M., Haroutunian, V., and Meador-Woodruff, J. H. (2015). Abnormal N-acetylglucosaminyltransferase expression in prefrontal cortex in schizophrenia. Schizophr. Res. 166, 219–224. doi:10.1016/j.schres.2015.06.002
Kjaer, J. B. (2017). Divergent selection on home pen locomotor activity in a chicken model: selection program, genetic parameters and direct response on activity and body weight. PLoS ONE 12, e0182103. doi:10.1371/journal.pone.0182103
Kjaer, J. B., and Sørensen, P. (1997). Feather pecking behaviour in White Leghorns, a genetic study. Br. Poult. Sci. 38, 333–341. doi:10.1080/00071669708417999
Knuesel, I., Mastrocola, M., Zuellig, R. A., Bornhauser, B., Schaub, M. C., and Fritschy, J. M. (1999). Short communication: altered synaptic clustering of GABAA receptors in mice lacking dystrophin (mdx mice). Eur. J. Neurosci. 11, 4457–4462. doi:10.1046/j.1460-9568.1999.00887.x
Konopaske, G. T., Subburaju, S., Coyle, J. T., and Benes, F. M. (2015). Altered prefrontal cortical MARCKS and PPP1R9A mRNA expression in schizophrenia and bipolar disorder. Schizophr. Res. 164, 100–108. doi:10.1016/j.schres.2015.02.005
Leblond, C. S., Cliquet, F., Carton, C., Huguet, G., Mathieu, A., Kergrohen, T., et al. (2019). Both rare and common genetic variants contribute to autism in the Faroe Islands. NPJ Genom. Med. 4, 1. doi:10.1038/s41525-018-0075-2
Liang, Z., Wen, C., Jiang, H., Ma, S., and Liu, X. (2021). Protein arginine methyltransferase 5 functions via interacting proteins. Front. Cell. Dev. Biol. 9, 725301. doi:10.3389/fcell.2021.725301
Lutz, V., Stratz, P., Preuß, S., Tetens, J., Grashorn, M. A., Bessei, W., et al. (2017). A genome-wide association study in a large F2-cross of laying hens reveals novel genomic regions associated with feather pecking and aggressive pecking behavior. Genet. Sel. Evol. 49, 18. doi:10.1186/s12711-017-0287-4
Marchler-Bauer, A., Derbyshire, M. K., Gonzales, N. R., Lu, S., Chitsaz, F., Geer, L. Y., et al. (2015). Cdd: NCBI's conserved domain database. Nucleic Acids Res. 43, D222–D226. doi:10.1093/nar/gku1221
Mashimo, M., Iwasaki, Y., Inoue, S., Saito, S., Kawashima, K., and Fujii, T. (2017). Acetylcholine released from T cells regulates intracellular Ca2+, IL-2 secretion and T cell proliferation through nicotinic acetylcholine receptor. Life Sci. 172, 13–18. doi:10.1016/j.lfs.2016.12.015
Matosin, N., Fernandez-Enright, F., Lum, J. S., Andrews, J. L., Engel, M., Huang, X.-F., et al. (2015). Metabotropic glutamate receptor 5, and its trafficking molecules norbin and tamalin, are increased in the CA1 hippocampal region of subjects with schizophrenia. Schizophr. Res. 166, 212–218. doi:10.1016/j.schres.2015.05.001
Mi, H., Huang, X., Muruganujan, A., Tang, H., Mills, C., Kang, D., et al. (2017). PANTHER version 11: Expanded annotation data from gene ontology and reactome pathways, and data analysis tool enhancements. Nucleic Acids Res. 45, D183–D189. doi:10.1093/nar/gkw1138
Parmentier, H. K., Rodenburg, T. B., Vries Reilingh, G. D., Beerda, B., and Kemp, B. (2009). Does enhancement of specific immune responses predispose laying hens for feather pecking? Poult. Sci. 88, 536–542. doi:10.3382/ps.2008-00424
Pasciuto, E., Burton, O. T., Roca, C. P., Lagou, V., Rajan, W. D., Theys, T., et al. (2020). Microglia require CD4 T cells to complete the fetal-to-adult transition. Cell. 182, 625–640.e24. e24. doi:10.1016/j.cell.2020.06.026
Pook, T., Mayer, M., Geibel, J., Weigend, S., Cavero, D., Schoen, C. C., et al. (2020). Improving imputation quality in BEAGLE for crop and livestock data. G3 (Bethesda) 10, 177–188. doi:10.1534/g3.119.400798
Reverter, A., and Chan, E. K. F. (2008). Combining partial correlation and an information theory approach to the reversed engineering of gene co-expression networks. Bioinformatics 24, 2491–2497. doi:10.1093/bioinformatics/btn482
Reverter, A., and Fortes, M. R. S. (2013). Association weight matrix: a network-based approach towards functional genome-wide association studies. Methods Mol. Biol. 1019, 437–447. doi:10.1007/978-1-62703-447-0_20
Rodenburg, T. B., Buitenhuis, A. J., Ask, B., Uitdehaag, K. A., Koene, P., van der Poel, J. J., et al. (2003). Heritability of feather pecking and open-field response of laying hens at two different ages. Poult. Sci. 82, 861–867. doi:10.1093/ps/82.6.861
Shannon, P., Markiel, A., Ozier, O., Baliga, N. S., Wang, J. T., Ramage, D., et al. (2003). Cytoscape: a software environment for integrated models of biomolecular interaction networks. Genome Res. 13, 2498–2504. doi:10.1101/gr.1239303
Sneeboer, M. A. M., van Mierlo, H. C., Stotijn, E., MacIntyre, D. J., Smith, C., Kahn, R. S., et al. (2019). Increased number of T-lymphocytes in post-mortem brain tissue of patients with schizophrenia. Schizophr. Res. 216, 526–528. doi:10.1016/j.schres.2019.10.032
Stowe, R. C., Sun, Q., Elsea, S. H., and Scaglia, F. (2018). LIPT1 deficiency presenting as early infantile epileptic encephalopathy, leigh disease, and secondary pyruvate dehydrogenase complex deficiency. Am. J. Med. Genet. A 176, 1184–1189. doi:10.1002/ajmg.a.38654
Tarantino, N., Leboyer, M., Bouleau, A., Hamdani, N., Richard, J. R., Boukouaci, W., et al. (2021). Natural killer cells in first-episode psychosis: an innate immune signature? Mol. Psychiatry 26, 5297–5306. doi:10.1038/s41380-020-01008-7
UniProt: The universal protein knowledgebase in 2021 (2021). UniProt: the universal protein knowledgebase in 2021. Nucleic Acids Res. 49, D480–D489. doi:10.1093/nar/gkaa1100
van der Eijk, J. A. J., Verwoolde, M. B., Vries Reilingh, G. d., Jansen, C. A., Rodenburg, T. B., and Lammers, A. (2019). Chicken lines divergently selected on feather pecking differ in immune characteristics. Physiol. Behav. 212, 112680. doi:10.1016/j.physbeh.2019.112680
van Dijk, T. B., Gillemans, N., Pourfarzad, F., van Lom, K., Lindern, M. V., Grosveld, F., et al. (2010a). Fetal globin expression is regulated by friend of Prmt1. Blood 116, 4349–4352. doi:10.1182/blood-2010-03-274399
van Dijk, T. B., Gillemans, N., Stein, C., Fanis, P., Demmers, J., van de Corput, M., et al. (2010b). Friend of Prmt1, a novel chromatin target of protein arginine methyltransferases. Mol. Cell. Biol. 30, 260–272. doi:10.1128/MCB.00645-09
Vestergaard, K. S., Kruijt, J. P., and Hogan, J. A. (1993). Feather pecking and chronic fear in groups of red junglefowl: their relations to dustbathing, rearing environment and social status. Anim. Behav. 45, 1127–1140. doi:10.1006/anbe.1993.1137
Wang, L., Luo, J., Fang, M., Jiang, G., Zhang, X., Yu, W., et al. (2012). A new trick of INPP4A: Decreased expression of INPP4A in patients with temporal lobe epilepsy and pilocarpine-induced rat model. Synapse 66, 533–541. doi:10.1002/syn.21540
Wysocki, M., Bessei, W., Kjaer, J. B., and Bennewitz, J. (2010). Genetic and physiological factors influencing feather pecking in chickens. World's. Poult. Sci. J. 66, 659–672. doi:10.1017/S0043933910000644
Yang, J., Lee, S. H., Goddard, M. E., and Visscher, P. M. (2011). Gcta: a tool for genome-wide complex trait analysis. Am. J. Hum. Genet. 88, 76–82. doi:10.1016/j.ajhg.2010.11.011
Keywords: feather pecking, eQTL, AWM, genomics, transcriptomics, gene regulation, behavioral disorder
Citation: Mott AC, Mott A, Preuß S, Bennewitz J, Tetens J and Falker-Gieske C (2022) eQTL analysis of laying hens divergently selected for feather pecking identifies KLF14 as a potential key regulator for this behavioral disorder. Front. Genet. 13:969752. doi: 10.3389/fgene.2022.969752
Received: 15 June 2022; Accepted: 25 July 2022;
Published: 17 August 2022.
Edited by:
Aline Silva Mello Cesar, University of São Paulo, BrazilReviewed by:
Mona Franziska Giersberg, Utrecht University, NetherlandsCopyright © 2022 Mott, Mott, Preuß, Bennewitz, Tetens and Falker-Gieske. This is an open-access article distributed under the terms of the Creative Commons Attribution License (CC BY). The use, distribution or reproduction in other forums is permitted, provided the original author(s) and the copyright owner(s) are credited and that the original publication in this journal is cited, in accordance with accepted academic practice. No use, distribution or reproduction is permitted which does not comply with these terms.
*Correspondence: Clemens Falker-Gieske, Y2xlbWVucy5mYWxrZXItZ2llc2tlQHVuaS1nb2V0dGluZ2VuLmRl
Disclaimer: All claims expressed in this article are solely those of the authors and do not necessarily represent those of their affiliated organizations, or those of the publisher, the editors and the reviewers. Any product that may be evaluated in this article or claim that may be made by its manufacturer is not guaranteed or endorsed by the publisher.
Research integrity at Frontiers
Learn more about the work of our research integrity team to safeguard the quality of each article we publish.