- Department of Plastic Surgery, Zhejiang Hospital, Hangzhou, Zhejiang, China
Background: Until now, the relevance of the tanning response to sun exposure and skin diseases has incomplete and inconsistent epidemiological observations. In this case, it is valuable to find out the causality of tanning response to sun exposure and skin diseases, and take a step further toward developing effective therapies as well as prevention methods.
Methods: We investigated the causal effect of tanning response to sun exposure on 10 major skin diseases that have been studied in recent large-scale genome-wide association studies (GWASs). Significant independent genetic variants from large-scale GWAS on ease of skin tanning (N = 453,065) are selected as the effective instrumental variables (IVs). For each skin disease, we extracted the summary statistics of those IVs (or their proxies) from the corresponding skin disease-GWAS as the valid IVs. Mendelian randomization (MR) was further performed to evaluate the causal association of ease of skin tanning with each of the skin diseases using different statistical methods, including inverse-variance weighted (IVW), the weighted median, and MR-Egger. Sensitivity analysis was also conducted to evaluate the effect of horizontal pleiotropy and heterogeneity.
Results: We observe significant associations between six skin diseases with tanning response to sun exposure with adjusted p-value derived by IVW less than 0.05 and with nominal p value less than 0.05 at the same time derived by either MR-Egger or weighted median. The six skin diseases include actinic keratosis (IVW FDR = 1.71E-40, MR Egger p-value = 3.46E-22), seborrhoeic keratosis (IVW FDR = 2.97E-4, MR Egger p-value = 1.06E-3), blepharochalasis (IVW FDR = 1.30E-3, MR Egger p-value = 2.91E-4), seborrhoeic dermatitis (IVW FDR = 1.29E-2, MR Egger p-value = 1.23E-2), malignant melanoma of skin (IVW FDR = 2.95E-2, MR Egger p-value = 1.91E-2), and freckles (IVW FDR = 2.95E-2, weighted median p-value = 1.02E-3). Interestingly, we find increased trends of developing all of the six skin diseases with increased tanning response to sun exposure (beta values are positive using IVW, MR-egger, and weighted median methods). We also replicate the association on three skin diseases using an independent outcome GWAS cohort, including malignant melanoma of the skin (replication IVW p-value = 2.13E-39), actinic keratosis (replication IVW p-value = 4.64E-32), and seborrhoeic keratosis (replication IVW p-value = 1.79E-3).
Conclusion: Our observation shows that the tanning response to sun exposure is positively correlated with the development of skin diseases in people of European descent by Mendelian randomization studies. But randomized controlled trials are still needed to add proof to our observations.
1 Introduction
Skin disease is one of the most common human diseases across cultures, ages, and races. There is a significant public health problem associated with inadequate sun exposure. According to studies conducted over the past decade, insufficient sun exposure may be responsible for 340,000 deaths in the United States and 480,000 deaths in Europe each year, and an increased incidence of breast cancer, colorectal cancer, hypertension, cardiovascular disease, metabolic syndrome, multiple sclerosis, Alzheimer’s disease, autism, asthma, type 1 diabetes, and myopia (Alfredsson et al., 2020). It is widely acknowledged that many skin diseases are associated with tanning and respond to sun exposure (Visconti et al., 2018).
It has been reported that the change from a subarctic/temperate to a subtropical climate for 4 weeks improved significantly skin symptoms and quality of life in children, even for 3 months after the return. Until now, sun exposure has not been studied in adult patients with atopic dermatitis (Napolitano et al., 2021). However, the deterioration of the ozone layer and the increasing use of sun tanning beds have led to an increase in skin-damaging ultraviolet radiation (Zitás and Mészáros, 2016). Among the most probable mechanisms of contamination-induced dermatological hazards are oxidative stress, inflammation, and metabolic impairment. This stress will be further aggravated by the deleterious synergy between pollution and sunlight. Some experiments identified a few polycyclic aromatic hydrocarbons (PAHs) inducing huge toxic stress, at nanomolar concentrations, when exposed to long ultraviolet radiation A (UVA) wavelengths (Marrot, 2018). Tanning response to sun exposure is the process of melanin pigmentation, which is a skin-protecting response from DNA photodamage (Ortonne, 1990; Gilchrest and Eller, 1999). Nine major skin diseases or traits are under investigation in our study, including malignant melanoma of the skin, actinic keratosis, blepharochalasis, seborrhoeic keratosis, and melanocytic naevi, scne vulgaris, freckles, lichen simplex chronicus, and prurigo and chalazion. The World Health Organization(WHO) declared having sufficient evidence to classify exposure to ultraviolet radiation (sun exposure) as carcinogenic. Also, sun exposure is consistently associated with malignant melanoma (Lucas et al., 2014; Raimondi et al., 2020). Actinic keratosis (AK), a lesion that will easily develop into malignancy, is also caused by excessive exposure to solar radiation (Berman and Cockerell, 2013). Seborrhoeic keratosis is reported to associate with sun exposure and tanning (Del Rosso, 2017). Sunlights have some influence on the occurrence of Melanocytic naevi (Barsoum and Harrison, 2020). The common naevi distribution pattern over the skin is consistent with the sun exposure habit (Carli et al., 1998). As for Acne vulgaris, its pathogenesis is various and complicated but sunlight could affect acne vulgaris negatively (Amblard and Leccia, 1992). For freckles, the common name of ephelides and lentigines, the causes are not completely clear and influenced by both genetic and environmental factors, but for sure, sunlight is a trigger (Passeron and Picardo, 2018). While blepharochalasis, a rare eyelid disorder has no confirmed etiology, so does the sun exposure factor. Also, lichen simplex chronicus (LSC) is a multifactor induced skin disorder, and clinical manifestations are reginal skin thickening by frequently rubbing or scratching; sunlight factor is not reported to be associated with this disorder till now. Also, prurigo is an inflammatory skin disease characterized by intensely pruritic, causes unclear, but most likely related to hypersensitivity, neuropsychiatric factors, and genetic allergic constitution; sun exposure influence is not reported. Also, chalazia are caused by inflammation and obstruction of the eyelids’ sebaceous glands (Taylor and Sober, 1996).
The causal link between tanning response to sun exposure and developing skin diseases remains incomplete and inconsistent. Therefore, in this study, we investigated the association between sun exposure and 10 major skin diseases or traits using Mendelian randomization (MR). Mendelian randomization design has been widely applied in recent years to determine the causal inferences of its significant advantage of overcoming the methodological limitations of observational studies. In this study, we used MR study to explore the causal association between tanning response to sun exposure (exposure) and skin diseases (outcomes) in European people of descent by multiple large-scale genome-wide association study (GWAS) datasets. The outcomes under investigation include acne vulgaris, actinic keratosis, blepharochalasis, chalazion, freckles, malignant melanoma of the skin, melanocytic naevi, prurigo nodularis, seborrhoeic dermatitis, and seborrhoeic keratosis.
2 Materials and methods
2.1 Study design
MR analysis used a group of single nucleotide polymorphisms (SNPs) as instrumental variables (IVs) to infer the causal effect of an exposure on an interesting outcome. The genome-wide association study (GWAS) summaries of exposure and various outcomes were downloaded from the MRC IEU OpenGWAS database. We extracted GWAS summary statistics of ease of skin tanning trait as the exposure data and 10 skin diseases as outcomes including acne vulgaris, actinic keratosis, blepharochalasis, chalazion, freckles, malignant melanoma of the skin, melanocytic naevi, prurigo nodularis, seborrhoeic dermatitis, and seborrhoeic keratosis.
In general, MR analysis needs to meet three assumptions: 1) the IVs should be significantly associated with the exposure; 2) the IVs should be independent. and 3) the IVs should not affect the outcome except through exposure. The overall design of this study is shown in Figure 1.
2.2 Selection of genetic variants associated with tanning response to sun exposure
The genetic associations on tanning response to sun exposure are derived from a large genome-wide association study of ease of skin tanning in 453,065 subjects of European ancestry (https://gwas.mrcieu.ac.uk/datasets/).
The cohort used in the GWAS analysis is from the UK Biobank (UKBB), which is a prospective cohort study that covered more than 500,000 individuals from the United Kingdom, aged from 40 to 70. Biological samples, including blood, urine, and saliva were collected and every participant accepted an extensive questionnaire investigation about health conditions and lifestyle. In accordance with the Declaration of Helsinki, every participant signed informed consent.
The UKBB study collected individual self-report about the ease of skin tanning of European ethnicity. The individuals who reported never tanned only burn, or get mildly or occasionally tanned, were included in the group of low tan responses. On the contrary, people who reported showing moderate or severe tanning were included in the group of high tan responses. Logistic regression was using PLINK assuming an additive genetic model and taking sex as a covariate, as well as the first five principal components evaluated on the genomic data, to control the potential population stratification.
We retrieved the GWAS dataset of ease of skin tanning from the MRC IEU GWAS database, where 9,851,867 SNPs across the genome were used for association analysis. The LD score regression analysis was used to estimate the SNP heritability for tanning response to sun exposure, and the total observed scale H2 is 0.1917 (se = 0.06), with lambda GC equal to 1.43. The GWAS variants with a p-value less than 5E-8 were used as potential instruments in the following MR studies after LD pruning.
2.3 GWAS datasets on skin diseases in discovery and replication phases
We investigated the causal effect of sun exposure on 10 major skin diseases, including acne vulgaris, actinic keratosis, blepharochalasis, chalazion, freckles, malignant melanoma of the skin, melanocytic naevi, prurigo nodularis, seborrhoeic dermatitis, and seborrhoeic keratosis. The demographic profiles of GWAS cohorts related to these diseases in the discovery phase are shown in the Results section. The GWASs on all skin diseases were performed in the European population, except that GWAS on freckles was performed in the East Asian population because the freckles-related GWAS for the European population is not available in the IEU database. For each skin disease, we extracted the summary statistics of those IVs (or their proxies) from the corresponding skin disease-GWAS to perform MR analysis.
In the discovery phase, the GWASs of the 10 investigated skin diseases were derived from the FinnGen biobank (https://www.finngen.fi/fi), which has collected biological samples from 500,000 participants in Finland over 6 years. To ensure the robustness of our discovery, we replicated 5 MR studies using UKBB cohorts, including actinic keratosis, chalazion, malignant melanoma of the skin, melanocytic naevi, and seborrhoeic keratosisdata, while the other five skin diseases lack necessary GWAS summaries in the UKBB.
2.4 MR analysis
Three MR analysis methods were chosen in this study, including the inverse-variance weighted (IVW) (Bowden et al., 2017), the weighted median (Bowden et al., 2016), and the MR-Egger test (Bowden et al., 2015). The effect size (beta) corresponds to one standard deviation (SD) in tanning response to sun exposure. All statistical tests were performed with R Packages TwoSampleMR (Hemani et al., 2018). The resulting p values of IVW were corrected by Benjamini–Hochberg (BH) procedure. Under the threshold of IVW-adjusted p-value < 0.05, either nominal p-value of MR Egger <0.05 or nominal p-value of weighted median <0.05, we declared significant MR results. The technical details of the three methods are described below.
2.4.1 IVW method
The inverse-variance weighted (IVW) method uses a weighted linear regression of SNP-exposure coefficients and SNP-disease coefficients to estimate the effect of exposure on outcome. Suppose there are
In MR analysis, genetic variants need to meet the following three assumptions to be valid instrumental variables (IVs): 1) the IVs should be significantly associated with the exposure; 2) the IVs should be independent; and 3) the IVs should not affect the outcome except through the exposure.
The IVW method is efficient when all genetic variants are valid IVs, which implies that
Let
If the genetic variant is valid IV,
The causal effect can be estimated from GWAS using inverse-variance weighted (IVW) as follows:
where
2.4.2 MR-egger method
The MR-Egger method is an alternative method for Mendelian randomization analysis which is like the IVW method. In MR-Egger,
In comparison with the IVW method, MR-Egger has a more intercept term,
2.4.3 Weighted median method
Let
2.5 Pleiotropy analysis
The pleiotropy analysis is on the ground of the MR-Egger intercept test.
In addition, we performed a heterogeneity test with Cochran’s Q statistic (together with the
3 Results and discussion
We performed MR analyses to measure the causal effect of tanning response to sun exposure on 10 common skin diseases. The demographic profiles of GWAS cohorts related to these diseases in the discovery phase are shown in Table 1. The GWASs on all skin diseases were performed in the European population, except that GWAS on Freckles was performed in the East Asian population because the freckles-related GWAS for the European population is not available in the IEU database. For each skin disease, the number of IVs represents the number of SNPs as IVs (or as IVs’ proxies with LD R2 > 0.4) shown in the corresponding skin disease-GWAS. In the discovery phase, the GWASs of the nine investigated skin diseases were derived from the FinnGen biobank (https://www.finngen.fi/fi).
The MR analyses are performed using three approaches (inverse variance weighted approach, MR Egger approach, and weighted median approach), and the overall results are presented in Table 2. Under the threshold of IVW FDR <0.05, and either nominal p-value of MR Egger <0.05 or nominal p-value of weighted median <0.05, we declare significant MR results. As a result, six of ten skin diseases we examined are significantly associated with the tanning response to sun exposure. In addition, we conduct sensitivity analyses and the results are given in Table 3. In the following results, we will discuss the effect of tanning response to sun exposure on skin diseases that passed the significance threshold.
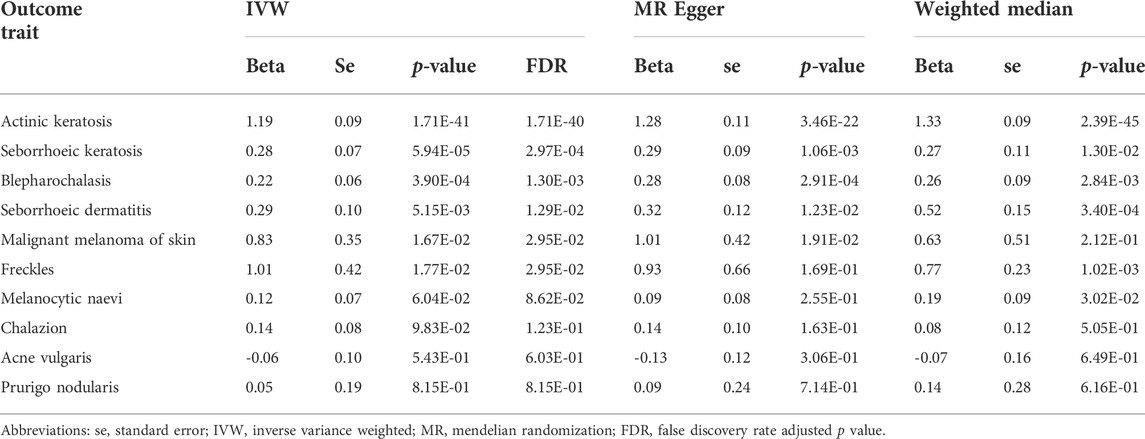
TABLE 2. MR results between tanning response to sun exposure and ten skin diseases in the discovery study.
3.1 Effect on actinic keratosis
To analyze the effect of tanning response to sun exposure on actinic keratosis, we extract 125 IVs from IEU databased (ID: finn-b-L12_ACTINKERA). A total of 4,817 actinic keratosis cases and 213,273 controls of the European population were involved in the original GWAS. The tanning response to sun exposure is significantly associated with increased risk of actinic keratosis according to the results of IVW (beta = 1.19, p-value = 1.71E-41, adjusted p-value = 1.71E-40), MR Egger (beta = 1.28, p-value = 3.46E-22) and weighted median (beta = 1.33, p-value = 2.39E-45). The results are shown in Figure 2.
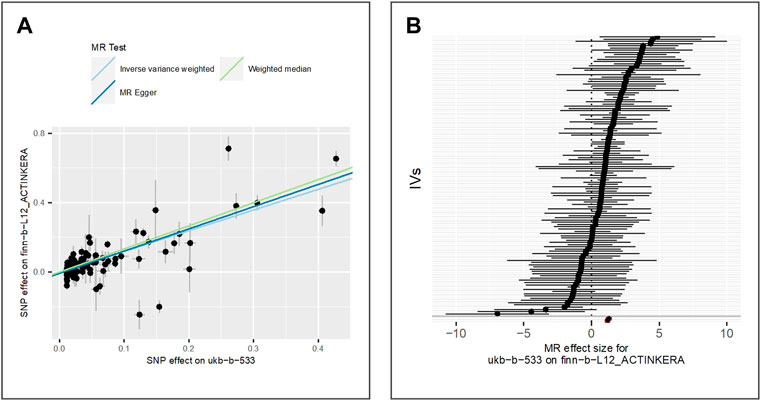
FIGURE 2. Comparison of MR methods and results associated with actinic keratosis. (A) Scatter plot reflects SNP effects on the actinic keratosis against SNP effects on the exposure; (B) forest plot represents the causal effect of exposure on the outcome using each SNP singly.
The Egger bias intercept is used to test the horizontal pleiotropy (intercept = -7.69E-03, p-value = 0.15) and the result indicates there is no horizontal pleiotropy. However, we find the presence of heterogeneity in the IVW analysis (p-value = 6.41E-26) and MR Egger analysis (p-value = 2.82E-25) using the Cochran Q test. Then we use the random effects model of IVW to estimate the effect of tanning response to sun exposure, and the results (beta = 1.19, p-value = 1.70558E-41) indicate there is still a positive correlation between exposure and actinic keratosis after considering the random noise. We performed a leave-one-out (LOO) sensitivity analysis to detect if the association was disproportionately influenced by a single SNP. We could see the LOO results in Supplementary Figure S1 and there was no bias caused by any single SNP.
3.2 Effect on seborrhoeic keratosis
We evaluate the effect of exposure on seborrhoeic keratosis using the GWAS dataset finn-b-L12_SEBORRKERAT. 2,434 cases and 207,482 controls of the European population were included in the GWAS. Also, we extract 125 valid IVs for downstream MR analysis. MR results of IVW (beta = 0.28, p-value = 5.94E-05, adjusted p-value = 2.97E-04), MR Egger (beta = 0.29, p-value = 1.06E-03) and weighted median (beta = 0.27, p-value = 1.30E-02) show evidence for the positive association between the exposure and seborrhoeic keratosis. Figure 3 shows the scatter plot for method comparison and forest plot indicating the MR effect of each IV.
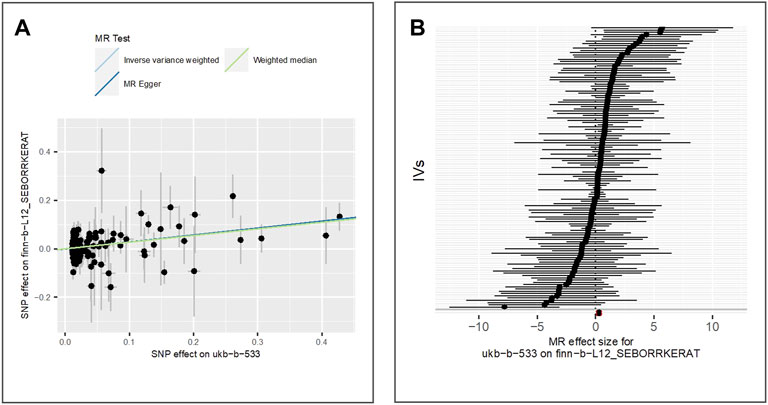
FIGURE 3. Comparison of MR methods and results associated with seborrhoeic keratosis: (A) scatter plot reflects SNP effects on the seborrhoeic keratosis against SNP effects on the exposure; (B) forest plot represents the causal effect of exposure on the outcome using each SNP singly.
The results of the Egger bias intercept (intercept: 5.50E-04, p-value = 0.90) show that there was no horizontal pleiotropy in the MR analysis. The results of IVW analysis (p-value = 0.30) and MR Egger analysis (p-value = 0.28) using the Cochran Q statistic show that there was no heterogeneity either. LOO analysis in Supplementary Figure S2 showed that results were not biased by a single SNP.
3.3 Effect on blepharochalasis
To estimate the effect of tanning response to sun exposure on blepharochalasis, we extract 125 IVs from finn-b-H7_BLEPHAROCHALASIS GWAS dataset which is derived from a cohort of 4,135 cases and 203,231 controls of the European population. The results of IVW (beta = 0.22, p-value = 3.90E-04, adjusted p-value = 1.30E-03), MR Egger (beta = 0.28, p-value = 2.91E-04) and weighted median (beta = 0.26, p-value = 2.84E-03) (as shown in Figure 4) indicate that tanning response to sun exposure is positively associated with the risk of blepharochalasis.
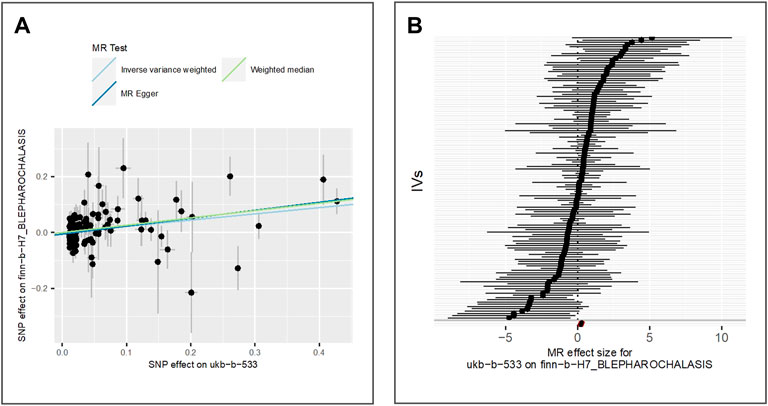
FIGURE 4. Comparison of MR methods and results associated with blepharochalasis: (A) scatter plot reflects SNP effects on the blepharochalasis against SNP effects on the exposure; (B) forest plot represents the causal effect of exposure on the outcome using each SNP singly.
The results of the Egger bias intercept (intercept: 5.45E-03, p-value = 0.16) show that there was no horizontal pleiotropy. The results of IVW analysis (p-value = 4.77E-03) and MR Egger analysis (p-value = 5.99E-03) using the Cochran Q statistic indicate the presence of significant heterogeneity. We applied the random effect model of IVW to re-estimate the MR effect, and the results under the IVW-random effect (beta = 0.22, p-value = 3.90E-04) indicate a statistically significant positive relationship between exposure and outcome considering the random noises. LOO analysis in Supplementary Figure S3 showed that results were not biased by a single SNP.
3.4 Effect on seborrhoeic dermatitis
There are 1,253 cases and 198,740 controls of the European population in the GWAS of seborrhoeic dermatitis (IEU ID: finn-b-L12_SEBORRHOEIC). We extract 125 IVs to perform MR analyses. The results of IVW (beta = 0.29, p-value = 5.15E-03, adjusted p-value = 1.29E-02), MR Egger (beta = 0.32, p-value = 1.23E-02) and weighted median (beta = 0.52, p-value = 3.40E-04) (as shown in Figure 5), indicate positive significant association between tanning response to sun exposure and seborrhoeic dermatitis.
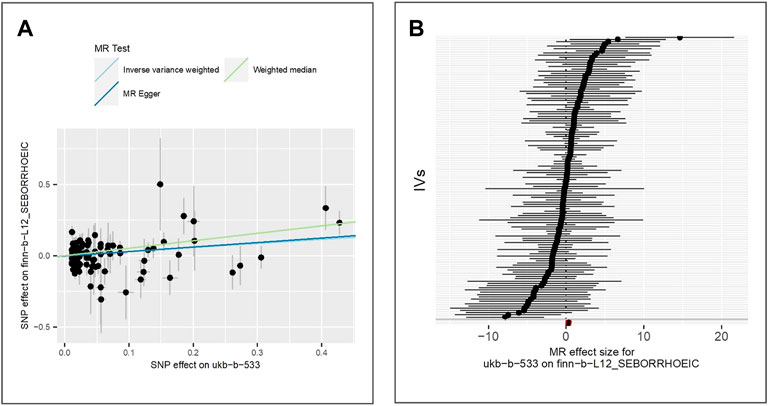
FIGURE 5. Comparison of MR methods and results associated with seborrhoeic dermatitis: (A) scatter plot reflects SNP effects on seborrhoeic dermatitis against SNP effects on the exposure; (B) forest plot represents the causal effect of exposure on the outcome using each SNP singly.
The results of the Egger bias intercept (intercept: 2.78E-03, p-value = 0.66) show that there is no horizontal pleiotropy. We perform heterogeneity analyses through IVW analysis (p-value = 5.44E-02) and MR Egger analysis (p-value = 4.93E-02) using the Cochran Q test. The IVW random effect results (beta = 0.29, p-value = 5.15E-03) show a significant positive relation between tanning response to sun exposure and seborrhoeic dermatitis. LOO analysis in Supplementary Figure S4 showed that results were not biased by a single SNP.
3.5 Effect on malignant melanoma of the skin
We extract the summary statistics from GWAS of malignant melanoma of skin (IEU ID: finn-b-C3_MELANOMA_SKIN) and find 125 valid IVs. We observe a positive association between exposure and the malignant melanoma of skin using all three methods, for example. IVW (beta = 0.83, p-value = 1.67E-02, adjusted p-value = 2.95E-02), MR Egger (beta = 1.01, p-value = 1.91E-02), and weighted median (beta = 0.63, p-value = 2.12E-01). The scatter plot for method comparison and forest plot indicating the MR effect of each IV is shown in Figure 6.
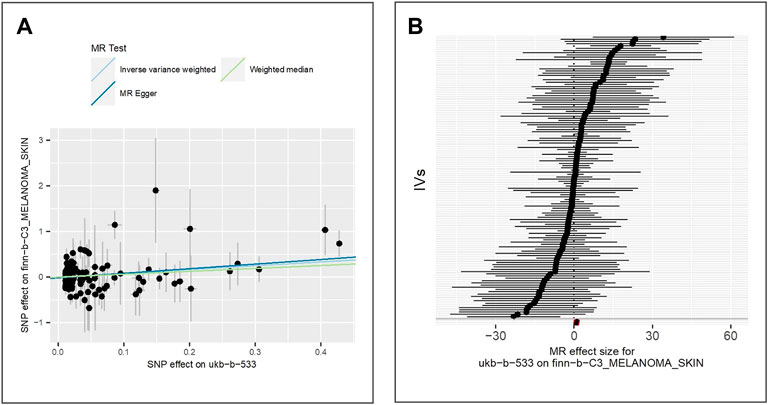
FIGURE 6. Comparison of MR methods and results associated with malignant melanoma of the skin: (A) scatter plot reflects SNP effects on the malignant melanoma of skin against SNP effects on the exposure; (B) forest plot represents the causal effect of exposure on the outcome using each SNP singly.
The results of the Egger bias intercept (intercept: 1.56E-02, p-value = 0.47) show that there was no horizontal pleiotropy. The results of IVW analysis (p-value = 0.177) and MR Egger analysis (p-value = 0.170) using the Cochran Q statistic neither indicate heterogeneity in the MR analysis. LOO analysis in Supplementary Figure S5 showed that results were not biased by a single SNP.
3.6 Effect on freckles
We extract 35 IVs from GWAS of freckles (IEU ID: ebi-a-GCST006091). This GWAS included 7,148 freckles cases and 4,034 controls of the East Asian population. We find that tanning response to sun exposure is positively associated with elevated risk of freckles using the two measuring methods IVW (beta = 1.01, p-value = 1.77E-02, adjusted p-value = 2.95E-02) and weighted median (beta = 0.77, p-value = 1.02E-03), while the result of MR Egger is not significant (beta = 0.93, p-value = 1.69E-01). The scatter plot for method comparison and the forest plot indicating the MR effect of each IV is shown in Figure 7.
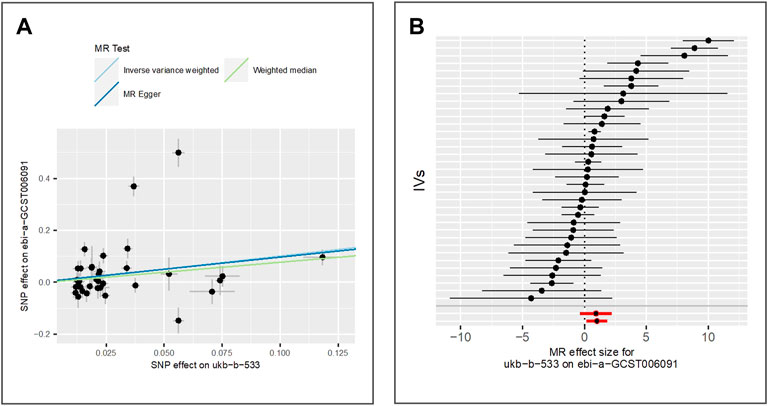
FIGURE 7. Comparison of MR methods and results associated with freckles: (A) scatter plot reflects SNP effects on the freckles against SNP effects on the exposure; (B) forest plot represents the causal effect of exposure on the outcome using each SNP singly.
The results of the Egger bias intercept (intercept: 3.52E-03, p-value = 0.88) show that there is no horizontal pleiotropy in the MR study. The results of IVW analysis (p-value = 1.91E-30) and MR Egger analysis (p-value = 7.68E-31) using the Cochran Q statistic indicate that heterogeneity exists. We then re-analyze the association using the IVW random effect model, and the results (beta = 1.01, p-value = 1.77E-02) show a significant positive association between tanning response to sun exposure and freckles. LOO analysis in Supplementary Figure S6 shows that results were not biased by a single SNP.
3.7 The replication study
To replicate the associations found between the ease of skin tanning and skin diseases, we perform MR using independent outcome GWAS datasets derived from the UKBB. Five of ten skin diseases are available in the UKBB, including actinic keratosis, chalazion, malignant melanoma of the skin, melanocytic naevi, and seborrhoeic keratosisdata. The summary of relative GWASs derived from UKBB is detailed in Table 4. The MR results and sensitivity results are shown in Tables 5 and 6, respectively. We observe similar associations as in the discovery phase, indicating the robustness of our findings.
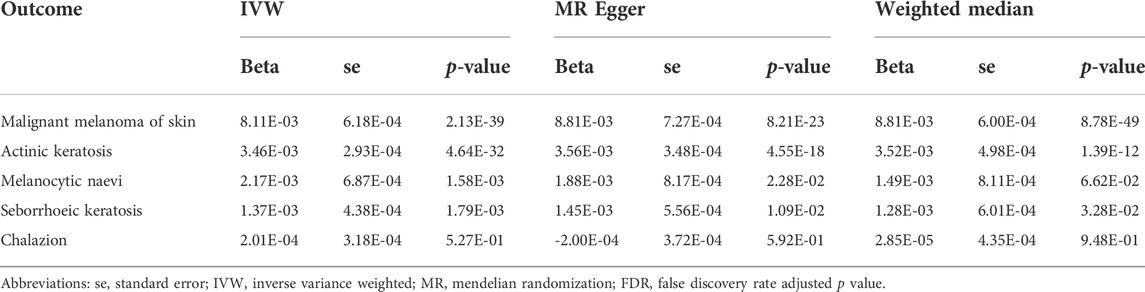
TABLE 5. MR results between tanning response to sun exposure and five skin diseases in the replication study.
4 Conclusion
Most skin changes are caused by sun exposure. To discover the causal relationship between sun exposure and tanning, as well as to develop effective therapies and preventative measures, we used a Mendelian randomization study to explore the causal association between tanning response to sun exposure and skin disease development based on multiple large-scale genome-wide association study datasets of the European population. Using Mendelian randomization studies, we found a positive correlation between tanning and skin diseases in the European population. There is a significant correlation between six skin conditions and tanning response to exposure to sunlight, including actinic keratosis, seborrhoeic keratosis, blepharochalasis, seborrhoeic dermatitis, malignant melanoma of skin, and freckles.
Data availability statement
The original contributions presented in the study are included in the article/Supplementary Material, and further inquiries can be directed to the corresponding author.
Author contributions
Conceptualization, WP and XH; methodology, QZ; writing—original draft preparation, WP; writing—review and editing, WP, QZ, SG, XW, FL; visualization, QZ; supervision, XH; project administration, XH. All authors have read and agreed to the published version of the manuscript.
Acknowledgments
We would like to thank the reviewers for their helpful remarks.
Conflict of interest
The authors declare that the research was conducted in the absence of any commercial or financial relationships that could be construed as a potential conflict of interest.
Publisher’s note
All claims expressed in this article are solely those of the authors and do not necessarily represent those of their affiliated organizations, or those of the publisher, the editors, and the reviewers. Any product that may be evaluated in this article, or claim that may be made by its manufacturer, is not guaranteed or endorsed by the publisher.
Supplementary material
The Supplementary Material for this article can be found online at: https://www.frontiersin.org/articles/10.3389/fgene.2022.967696/full#supplementary-material
Abbreviations
GWAS, Genome-wide association study; IVW, inverse-variance weighted; LSC, lichen simplex chronicus; MR, Mendelian randomization; MR-PRESSO, MR pleiotropy residual sum and outlier; SD, standard deviation; UKBB, United Kingdom Biobank.
References
Alfredsson, L., Armstrong, B. K., Butterfield, D. A., Chowdhury, R., de Gruijl, F. R., Feelisch, M., et al. (2020). Insufficient sun exposure has become a real public health problem. Int. J. Environ. Res. Public Health 17 (14), 5014. doi:10.3390/ijerph17145014
Amblard, P., and Leccia, M. T. (1992). Skin diseases with photosensitivity. Rev. Prat. 42 (11), 1365–1368.
Barsoum, R., and Harrison, S. L. (2020). Clinical characteristics in early childhood associated with a nevus-prone phenotype in adults from tropical Australia: two decades of follow-up of the townsville preschool cohort study. Int. J. Environ. Res. Public Health 17 (22), 8680. doi:10.3390/ijerph17228680
Berman, B., and Cockerell, C. J. (2013). Pathobiology of actinic keratosis: ultraviolet-dependent keratinocyte proliferation. J. Am. Acad. Dermatol. 68, S10–S19. doi:10.1016/j.jaad.2012.09.053
Bowden, J., Davey Smith, G., and Burgess, S. (2015). Mendelian randomization with invalid instruments: effect estimation and bias detection through egger regression. Int. J. Epidemiol. 44, 512–525. doi:10.1093/ije/dyv080
Bowden, J., Davey Smith, G., Haycock, P. C., and Burgess, S. (2016). Consistent estimation in mendelian randomization with some invalid instruments using a weighted median estimator. Genet. Epidemiol. 40, 304–314. doi:10.1002/gepi.21965
Bowden, J., Del Greco, M. F., Minelli, C., Davey Smith, G., Sheehan, N., and Thompson, J. (2017). A framework for the investigation of pleiotropy in two-sample summary data Mendelian randomization. Stat. Med. 36, 1783–1802. doi:10.1002/sim.7221
Carli, P., Biggeri, A., Nardini, P., De Giorgi, V., and Giannotti, B. (1998). Sun exposure and large numbers of common and atypical melanocytic naevi: an analytical study in a southern European population. Br. J. Dermatol. 138 (3), 422–425. doi:10.1046/j.1365-2133.1998.02118.x
Del Rosso, J. Q. (2017). A closer look at seborrheic keratoses: Patient perspectives, clinical relevance, medical necessity, and implications for management. J. Clin. Aesthet. Dermatol. 10 (3), 16–25.
Gilchrest, B. A., and Eller, M. S. (1999). DNA photodamage stimulates melanogenesis and other photoprotective responses. J. Investig. Dermatol. Symp. Proc. 4 (1), 35–40. doi:10.1038/sj.jidsp.5640178
Hemani, G., Zheng, J., Elsworth, B., Wade, K. H., Haberland, V., Baird, D., et al. (2018). The MR-Base platform supports systematic causal inference across the human phenome. elife 7, e34408. doi:10.7554/eLife.34408
Lucas, R. M., Norval, M., Neale, R. E., Young, A. R., de Gruijl, F. R., Takizawa, Y., et al. (2014). The consequences for human health of stratospheric ozone depletion in association with other environmental factors. Photochem. Photobiol. Sci. 14, 53–87. doi:10.1039/c4pp90033b
Marrot, L. (2018). Pollution and sun exposure: a deleterious synergy. Mechanisms and opportunities for skin protection. Curr. Med. Chem. 25 (40), 5469–5486. doi:10.2174/0929867324666170918123907
Napolitano, M., Monfrecola, G., Fabbrocini, G., Fattore, D., Patrì, A., and Patruno, C. (2021). Impact of sun exposure on adult patients affected by atopic dermatitis. Ital. J. Dermatol. Venerol. 156 (5), 558–561. doi:10.23736/S2784-8671.20.06582-7
Ortonne, J. P. (1990). The effects of ultraviolet exposure on skin melanin pigmentation. J. Int. Med. Res. 18 (3), 8C–17C.
Passeron, T., and Picardo, M. (2018). Melasma, a photoaging disorder. Pigment. Cell Melanoma Res. 31, 461–465. doi:10.1111/pcmr.12684
Raimondi, S., Suppa, M., and Gandini, S. (2020). Melanoma epidemiology and sun exposure. Acta Derm. Venereol. 100 (11), adv00136. doi:10.2340/00015555-3491
Visconti, A., Duffy, D. L., Liu, F., Zhu, G., Wu, W., Chen, Y., et al. (2018). Genome-wide association study in 176, 678 Europeans reveals genetic loci for tanning response to sun exposure. Nat. Commun. 9, 1684. doi:10.1038/s41467-018-04086-y
Keywords: tanning, sun exposure, skin diseases, Mendelian randomization, melanin pigmentation
Citation: Ping W, Zhao Q, Ge S, Wang X, Li F and Huang X (2022) Evaluating the effect of tanning response to sun exposure on the risk of skin diseases through Mendelian randomization. Front. Genet. 13:967696. doi: 10.3389/fgene.2022.967696
Received: 13 June 2022; Accepted: 03 August 2022;
Published: 02 September 2022.
Edited by:
Zhen Tian, Zhengzhou University, ChinaReviewed by:
Yanshuo Chu, University of Texas MD Anderson Cancer Center, United StatesDonghua Yu, Shaoxing University, China
Copyright © 2022 Ping, Zhao, Ge, Wang, Li and Huang. This is an open-access article distributed under the terms of the Creative Commons Attribution License (CC BY). The use, distribution or reproduction in other forums is permitted, provided the original author(s) and the copyright owner(s) are credited and that the original publication in this journal is cited, in accordance with accepted academic practice. No use, distribution or reproduction is permitted which does not comply with these terms.
*Correspondence: Xiaoxiang Huang, insomnianhxx@163.com
†These authors have contributed equally to this work