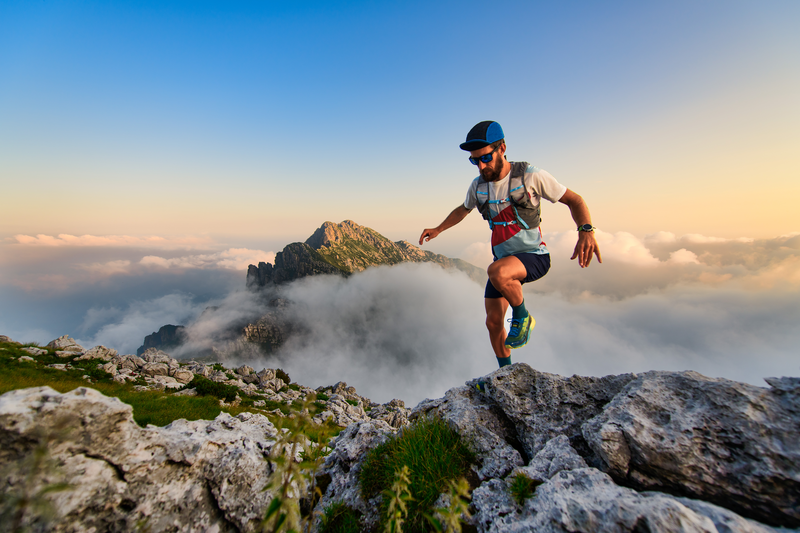
94% of researchers rate our articles as excellent or good
Learn more about the work of our research integrity team to safeguard the quality of each article we publish.
Find out more
ORIGINAL RESEARCH article
Front. Genet. , 24 October 2022
Sec. Livestock Genomics
Volume 13 - 2022 | https://doi.org/10.3389/fgene.2022.961474
This article is part of the Research Topic Transcriptional/Post-Transcriptional Regulations in Agricultural Species After Stresses View all 18 articles
Weaning represents one of the most critical periods in pig production associated with increase in disease risk, reduction in performance and economic loss. Physiological changes faced by piglets during the weaning period have been well characterised, however little is currently known about the underlying molecular pathways involved in these processes. As pig meat remains one of the most consumed sources of protein worldwide, understanding how these changes are mediated is critical to improve pig production and consequently sustainable food production globally. In this study, we evaluated the effect of weaning on transcriptomic changes in the colon of healthy piglets over time using an RNA-sequencing approach. The findings revealed a complex and coordinated response to weaning with the majority of genes found to be rapidly differentially expressed within 1 day post weaning. Multiple genes and pathways affected by weaning in the colon were associated with immune regulation, cell signalling and bacterial defence. NOD-like receptors, Toll-like receptor and JAK-STAT signalling pathways were amongst the pathways significantly enriched. Immune activation was evidenced by the enrichment of pathways involved in interferon response, cytokines interactions, oxidoreductase activities and response to microbial invasion. Biosynthesis of amino acids, in particular arginine, was also amongst the most enriched KEGG pathways in weaned pigs, reinforcing the critical role of arginine in gut homeostasis under stress conditions. Overall, transcriptomic and physiological results suggest that pigs going through the weaning transition undergo a transient period of inflammatory state with a temporary breakdown of barrier functions in the gut. These findings could provide valuable tools to monitor host response post weaning, and may be of particular relevance for the investigation and development of intervention strategies aimed to reduce antibiotic use and improve pig health and performance.
Livestock production is expected to produce more food than ever before. As the expanding world population is getting wealthier, the demand for safe and secure animal protein is increasing (Henchion et al., 2017). The challenge is to meet this demand in ways that are environmentally, socially and economically sustainable. Together with poultry, pork is one of the fastest growing livestock sectors and also one of the most consumed meats world-wide (FAO, 2019). Pig production is also widely recognised as one of the most efficient in terms of carbon footprint and climate change potential compared to other animal protein source (Macleod et al., 2013). Ensuring livestock animals can fulfil their full genetic potential is essential for sustainable food production.
As a result of abrupt dietary, social, and environmental changes, weaning is recognised as the most critical period in modern pig production associated with an increase in disease risk, reduction in performance and welfare leading to significant economic loss (Gresse et al., 2017; Nowland et al., 2019). At weaning, the pigs gastrointestinal tract (GIT) undergoes rapid changes in size, protein turnover rates, microbiome composition, and detrimental alterations in digestive and barrier functions (Pluske et al., 2018). Although the physiological changes faced by piglets over weaning have been well characterised, little is known about the underlying genes and pathways involved in these processes. Understanding how these changes are regulated or mediated is critical to improve pig production and consequently sustainable food production globally.
Furthermore, due to similarities in anatomy and physiology, the pig is widely recognised as a translational animal model to study human gastrointestinal diseases and to understand biological pathways related to mucosal function, development and nutritional regulation (Zhang et al., 2013; Roura et al., 2016; Sciascia et al., 2016). Previous studies have highlighted the importance of improving the knowledge on molecular mechanisms responsible for phenotypic differences especially at an early age with the dual purpose of improving production and providing adequate models for human studies (Ayuso et al., 2015).
Recent advances in sequencing technologies now provides novel opportunities to comprehensively explore the complex gut ecosystem of humans and animals. RNA-sequencing (RNA-Seq) is a powerful high-throughput approach to profile gene expression that, in contrast with microarray-based technologies, allows for the characterisation and quantification of both known and unknown transcripts (Mach et al., 2014). Fundamental understanding of the host response to stress is paramount to develop tools or best practices to improve pig health, productivity and welfare. To date, RNA-Seq has been used to study production traits of livestock animals but transcriptomic studies in pigs using this technology is relatively scarce and have mainly focused on disease response or regulation mechanism of fat deposition and muscle development to evaluate growth and meat quality between pig genotypes (Piórkowska et al., 2018; Xu et al., 2019).
Much attention and focus has been given to the gut microbiome and its taxonomic and metabolic changes through pig development and weaning (Frese et al., 2015; Bian et al., 2016; Guevarra et al., 2018a) but we are still lacking understanding about the host gene expression change in response to weaning. The current study aims to investigate the transcriptomic changes in the pig gut through weaning over time.
All animals were treated in accordance with the University of Nottingham ethical guidelines and codes of practice applying to care and management of animals. Twelve litters (Landrace x Large White) over three batches (4 litters per batch) were used in the study and housed at the School of Biosciences, Sutton Bonington Campus, University of Nottingham, United Kingdom. For the phenotypic data, all 3 batches (12 litters) were used. For the transcriptomic data, only one batch (the first batch) was used (4 litters).
For prevention of iron deficiency and coccidiosis, all piglets received a 1 ml IM injection of Gleptosil (Alstoe Ltd., York, United Kingdom) 24 h after birth, and 0.7 ml of Baycox (Bayer, Newbury, United Kingdom) orally 3 days after birth. At 21 days of age, 6 weight-matched piglets per litter were randomly allocated by random selection of coded balls to treatment (baseline average weight at day 19: 6.77 ± 0.189 kg). Piglets allocated to the weaned treatment were separated from their dam, moved and mixed with non-littermates in pens of 4 individuals and received ad lib commercial diet (wheat, whey powder and soya based) containing: 21.25% protein, 7.50% fat, 2.00% fibre, 5.00% ash, 1.70% lysine, 13.80% moisture). Weaned piglets did not receive creep feed supplementation before weaning. Piglets allocated to the unweaned treatment as control remained with their dam and littermate up to 35 days of age with access to creep feed from day 25 days of age (same commercial diet as above). No antibiotic or anthelmintic treatment were used during the trial. At day 1, 4, and 14 post weaning: one weaned and one unweaned piglet from each litter were weighed euthanised by intraperitoneal injection of Dolethal (1 ml.kg-1 body weight; 20% w/v Pentobarbitone Sodium, Vétoquinol, Buckingham, United Kingdom). At slaughter, body lesion was scored for each pig on a 3-point scale with 1 for no lesion, 2 for moderate scratches on back, flank, head, ear and tail, and 3 for intense, deep or bleeding scratches on back, flank, head, ear and tail. Graphic visualisation of the trial design can be found in Supplementary Figure S1.
All sample processing and analysis was blinded using randomly generated numerical codes. Tissue samples from the 0.5 section of the colon were immediately collected post-slaughter, rinsed in sterile buffered saline solution and preserved in RNA later (Ambion, CA United States ) at 4°C for 24–48 h to allow tissue penetration then stored at −80°C.
Tissue samples of the 0.5 small intestine (as proportions) along from the gastric pylorus to the ileocecal valve were fixed in Bouin’s solution, embedded in paraffin and cut in 5 µm transverse sections. Histological section were stained with H&E for histometric measurement of villus length and crypt depth, with Periodic Acid Schiff stain for goblet cell counts (Matsuo et al., 1997) and with Toluidine Blue for quantification of mast cells (Moeser et al., 2007).
Secretory IgA (sIgA) was measured in ileal flushes using the methods previously described by Lessard et al. (2009). At slaughter, a 20 cm segment of ileum taken upstream from the cecum was flushed using 5 ml of sterile PBS, and centrifuged for 10 min at 500 g. The supernatant was collected and stored at −80°C. Secretory IgA was measured in duplicate using a sandwich Porcine IgA ELISA Quantitation Kit (Bethyl Laboratories, TX, United States ) according to the manufacturer protocol.
Total RNA was extracted using NucleoSpin® RNA II kit (Macherey-Nagel, Düren · Germany), briefly 10–30 mg of tissue was placed in a 2 ml round bottomed tube (Fisher Scientific, United Kingdom) with 350 µl of guanidine thiocyate buffer, 3.5 µl of 0.1 mM β-mercaptoethanol and a 5 mm stainless steel bead for homogenisation for 2 × 2 min at 3,000 rpm using a Retsch MM200 homogeniser (Retsch, Haan, Germany). The extraction process was then followed according to the manufacturer instruction until the last step where final elution volume was 40 µl of RNAse-free water instead of 60 µl. All samples were quantified and tested for RNA integrity using the Nanodrop 1,000 spectrophotometer (NanoDrop Technologies, DE, United States ) and Agilent 2,100 Bioanalyzer (Agilent Technologies, CA, United States ). Mean (±SE) of RNA concentration was 306.5 ± 29 ng/ml and RNA integrity number was 9.21 ± 0.13.
RNA samples were sent to ARK Genomics (Edinburgh, United Kingdom) for library preparation and sequencing of the whole transcript expression. Illumina TruSeq mRNA library prep kit was used, and paired end sequencing was performed on an Illumina HiSeq2000 sequence analyser (Illumina, Inc. San Diego, United States ). Animal details and metadata are provided in Supplementary Table S3 and Supplementary Table S4. Mean number of raw reads per sample was 101.4 M (min = 66.1M, max = 133.6 M), equivalent to 5,139 Mbp per sample on average. Reads in fastq format are available at the European Nucleotide Archive (ENA) with the accession number PRJEB54752 https://www.ebi.ac.uk/ena/browser/view/PRJEB54752.
Raw fastq reads were processed with Cutadapt (Version 1.12) (Martin, 2011) to remove adapters and low-quality bases (3′ quality cutoff of 10, -q 10), paired reads with a minimum length of 20 bases were retained prior to quantitation (−m 20). Sequence alignment and read quantification was performed using the pseudo-alignment-based tool Kallisto v0.43.0 (Bray et al., 2016). Differential expression of transcripts between weaned and unweaned animals was determined using Sleuth v0.28.1 (Pimentel et al., 2017). Identification of genes enriched in specific functions were determined with reference to the Kyoto Encyclopedia of Genes and Genomes (KEGG) and gene ontology (GO), using “NIPA” software available on https://github.com/ADAC-UoN/NIPA to conduct hypergeometric tests for enrichment with Benjamini-Hochberg multiple testing correction.
For phenotypic data, statistical analysis was performed in IBM SPSS v24 to determine the effect of weaning treatment at different time point treatment on pig weight, intestinal and blood measurement using linear mixed model analysis. In the mixed model, time point*treatment was used as the fixed effect and trial batch and litter as random effects.
Gene expression cluster analysis was performed and revealed that sex was the only factor that showed a grouping effect. Therefore sex was included in the model as a confounder for the gene expression analysis. Sequence alignment and read quantification was performed using the pseudo-alignment-based tool Kallisto v0.43. (Bray et al., 2016). Differential expression was determined using the Wald test in Sleuth v0.28.1 (Pimentel et al., 2017) with sex as a confounder in the model. Transcripts with a false detection rate corrected p-value < 0.1 and a log2 fold change (log2FC) greater than 1 and less than minus 1 were considered to be differentially expressed. False detection rate correction was performed using the Benjamini-Hochberg method (Benjamini and Hochberg, 1995).
All piglets were found in good health during the trial and displayed no clinical signs of disease or scour. As expected, weaning caused a number of physiological changes which were found to be time-dependant. Significant differences were observed in pig weight, blood and plasma measurements, body lesion scores and intestinal measurement (Table 1). These results also indicate the significant effect of time on almost all variables measured between day 1 and day 14 post weaning. This highlights that the pigs are undergoing rapid period of development at this age and the importance of designing studies using age-matched controls when evaluating the effect of weaning in pigs as opposed to using pre-weaning values as controls.
TABLE 1. Phenotypic measurements between weaned and unweaned pigs at 1, 4, and 14 days post weaning. Data shown are means and the pooled SEM for weaned and unweaned pigs for each time points.
Plasma analysis revealed that cortisol levels remained stable over time in the unweaned group but increased almost 4-fold at day 1 and 2-fold at day 4 post weaning in the weaned pigs suggesting an activation of the HPA axis under weaning stress. Interestingly, while most parameters showed a rapid spike followed by a progressive return to the unweaned level by day 14, cortisol level still remained significantly higher at 14 days post weaning but to a lesser magnitude.
Haematology and biochemistry profiles are used as indicators of health status to evaluate the metabolic, nutritional and energy state of the pig. In blood, circulating granulocyte levels, haemoglobin and haematocrit were significantly increased in weaned pigs, while platelet counts decreased. In plasma, cholesterol, triglycerides and globulin levels decreased at day 4 and day 14 in the weaned group compared to unweaned pigs.
Measurement of intestinal architecture were also affected by weaning, with significant reduction in jejunal villus height and villus/crypt ratio. However, villus width remained unaffected by weaning and crypt depth was increased. Goblet cell numbers in crypt and in villus remained unaffected by weaning. Mucosal mast cells decreased at day 4 with an overall statistical trend for weaned pigs to show reduced mast cell count compared to unweaned controls.
Ileal sIgA was also greatly reduced at all time points post weaning, suggesting a decrease in immune protection from maternal milk. sIgA is considered one of the first line of defence and a critical factor for piglet growth and survival. Removal of the piglets from the sow at weaning causes dramatic drop in gut IgA levels increasing the immune vulnerability of piglets post weaning.
RNAseq (HiSeq) was used to identify differentially expressed transcripts (DET) between weaned and unweaned pigs in colonic tissue. An average of 52.3 million trimmed paired reads were obtained for each sample. Reads mapped as pairs (83.0%–85.3%) to the porcine genome ref sequence (sus scrofa 10.2). The Wald test was used on TPM to establish the total number of DET between weaned and unweaned pigs for each time point and identified a total of 239 transcripts at q value ≤0.1 and FC ≥ 2. The volcano plots visually represent significant DET for each time point and show an even distribution between up- and down-regulated genes (Figure 1).
FIGURE 1. Volcano plots of differentially expressed transcripts between weaned and unweaned pigs at 1, 4, and 14 days post weaning. Red points indicates genes significant at Qval ≤0.1.
The majority of transcripts 171 (71.6%) were found to be differentially expressed after 1 days post weaning. After 4 days and 14 days post weaning only 67 (28.0%) and 1 (0.4%) genes were differentially expressed between weaned and unweaned pigs, respectively (Figure 2). Among DET with the cut off values of Qval ≤0.1 & FC ≥ 2, two transcripts were identified in common between day 1 and day 4 post weaning: ENSSSCT00000014128 and ENSSSCT00000017308 which are of unknown function (Figure 3). They were no DET common at all three time point or shared between day 4 and 14 or day 1 and 14 post weaning. Transcription according to time point and weaning treatment are shown in PCA plots and revealed distinct clusters between weaned and unweaned pigs across time points (Figure 4).
FIGURE 2. Number of up and downregulated transcripts between weaned and unweaned pigs at 1, 4, and 14 days post weaning (Qval ≤0.1 & FC ≥ 2).
FIGURE 3. Venn diagram of the number of differentially expressed transcripts between weaned and unweaned pigs for each time point (Qval ≤ 0.1 & FC ≥ 2).
FIGURE 4. Principal Component Analysis (PCA) of gene expression data from pigs labelled as time point (left panel) and weaning status (right panel).
The full list of differentially expressed transcripts at Qval ≤0.1 & FC ≥ 2 is provided in Supplementary Table S1. DETs with FC > 2 across all time points were subject to Gene Ontology (GO) and KEGG pathway analysis using NIPA https://github.com/richarddemes/NIPA to identify significantly enriched pathways between weaned and unweaned pigs at Qval ≤0.05 and minimum number of gene in term of two (Supplementary Table S2). Top 10 significantly enriched GO terms and KEGG pathways are shown in Figure 5.
FIGURE 5. Gene ontology (GO) and KEGG pathway enrichment analysis showing top 10 enriched terms between weaned and unweaned pigs (all time points). Size of circles represents number of gene in each term. (A) GO Biological Process, (B) GO Molecular Function, (C) GO Cellular Compartment, (D) KEGG.
In this study, the typical characteristics previously reported in other studies were observed validating our weaning model. Piglet weights were negatively affected by weaning at day 1 and day 4 but recovered at day 14. Post weaning growth check is a commonly reported problem in pigs with detrimental impact on life-time growth performances representing a large economic loss to the industry (Collins et al., 2017). Evidence of stress was also observed in this study with elevated plasma cortisol and increased lesion scores in the weaned pigs.
Measurements of intestinal structures such as villus and crypt have been commonly used to evaluate gut health as a measure of absorptive capacities for nutrients (Bontempo et al., 2006; Le Bon et al., 2010). As reported by others, the current study shows that villus morphometry is impaired by weaning. Villus atrophy during the first few days post weaning has been commonly reported and is often associated with loss in barrier function, decreased enzyme activities and decreased performance as observed in this study (Lallès, 2008).
This study has revealed a large number of genes up and down regulated as consequences of weaning in the pig colon highlighting the profound physiological impact of weaning on healthy pigs. The large majority of differentially expressed transcripts were found to peak on day 1 post weaning and progressively returned to unweaned level by day 14, emphasising the abrupt response of pigs to weaning (Lallès et al., 2007). There was little overlap in transcripts between time points suggesting a coordinated regulation of the host in response to weaning. These findings could inform selection of appropriate time points for further trials in weaned pigs.
Due to the large number of DET identified in this study, this discussion will mainly focus is on pathways significantly affected by weaning rather that individual genes. Metabolic, immune and barrier function activities have previously been implicated with weaning response in both the small and large intestine but the underlying mechanism for these changes have not been previously identified. In the current study, we found several pathways related to microbial response and immune functions activated post weaning; specifically, NOD-like receptors, Toll-like receptor and JAK-STAT signalling pathways are shown to be significantly activated. These signalling pathways indicate a cellular response to Microbial Associated Molecular Patterns (MAMPs) that drives the activation innate immune response. As such, we also observe positive regulation of Interferon alpha production and Interferon gamma mediated pathways.
In agreement with these results, Wang et al. (2008) also reported that weaning at 21 days of age showed an increase in expression of genes associated with oxidative stress and immune activation but decreased expression of genes related to nutrient utilisation and cell proliferation in the jejunum of pigs using microarray analysis. A previous study also reported that pigs weaned at a later age (28 days) showed increase in pro-inflammatory cytokines expression in the small intestine and colon during the first 2 days post weaning before returning to pre-weaned level after 8 days post weaning (Pié et al., 2004). The interferon response following weaning stress in pigs has previously been characterised and showed that weaning causes the release of IFN-α and the transient shut-off of the corresponding gene transcriptions in PBMC (Razzuoli et al., 2011).
In addition, Moeser et al. (2017) demonstrated that colonic and jejunal transepithelial resistance was increased resulting in impaired permeability of the gut barrier as a result of weaning, which may facilitate infiltration of luminal component such as bacteria or bacterial products. Here we see at least two GO biological terms that would agree with this hypothesis: “cellular responses to LPS” and “response to bacterium” where genes such as NOS2, CD274, IRF3, CXCL11, and ACOD1, are upregulated.
The immune regulation observed through our transcriptomic results also reflects the significant increase in granulocyte levels found in blood, suggesting both a local and systemic activation of immunity in response to weaning. The combined effect of immune and stress activation can lead to an energy cost whereby growing animals divert their energy resource towards these responses instead of growth which can explain reduced performance observed in weaned pigs. In growing pigs, transcriptomic multi-tissue analysis revealed that activated immune response, protein metabolism, defence against pathogens and oxidative stress were the main biological pathways associated with feed efficiencies (Gondret et al., 2017). The authors suggested that dietary intervention with anti-inflammatory or antioxidant properties could be evaluated to improve efficiencies in growing pigs. As feed accounts for more than 60% of the cost of food production, improving feed efficiency is a major target to improve profitability of the pig industry (Jing et al., 2015).
The colon also harbours the richest and most diverse microbial population of the gut. In recent years, a large number of studies have documented the development of the gut microbiota of the pig over time (Frese et al., 2015; Mach et al., 2015; Guevarra et al., 2018b; Han et al., 2018; Ke et al., 2019; Wang et al., 2019). Weaning is typically associated with a decrease in gut microbial diversity and major shifts in bacterial taxa composition (Guevarra et al., 2019). In the current study, we observe activation of immune signalling pathways and pro-inflammatory cytokines in the early days post weaning, and we also observe regulation of pathways involved in oxido-reducatase activity. Amongst the activated pathways related to anti-microbial defence, genes involved in oxidative burst such as NOS2 (Nitric oxide synthesase 2) and NOX1 (NAPDH oxidase 1) are upregulated which are involved in the production of nitic oxide and superoxide radicals. These nitrogen rich compounds are rapidly converted into nitrate (NO3-) in the lumen providing favourable condition for the growth and proliferation of gut bacteria that carry nitrate-reductase genes such as Enterobacteriaceae (Winter et al., 2013). In addition, inflammatory conditions provide increased level of luminal oxygen due to elevated blood flow and haemoglobin, this favours aerobic respiration of Enterobacteriaceae while inhibiting the growth of obligate anaerobes such as Bacterioides and Clostridia (Zeng et al., 2017). Enterobacteriaceae have a detrimental effect on pig health and growth and is one of the leading cause of diarrhoea in pig production (Rhouma et al., 2017). E. coli is highly prevalent post weaning and can lead to mortality and zoonosis (Luppi, 2017). As a result, antibiotic usage is commonly used around weaning and have led to increasing reports in colistin-resistant E. coli in pigs (Rhouma et al., 2017). Tackling post weaning inflammatory response could represent a step towards reduction in antimicrobial use in pig production.
At weaning, pigs are abruptly transitioned from sow’s milk to a complex plant-based diet with distinct nutritional profile and as such transcriptomic modulation of pathways involved in nutritional metabolism would be expected. In the current study, biosynthesis of amino acids, in particular arginine biosynthesis was the second most significant KEGG pathway enriched in weaned pigs via the up regulation of argininosuccinate synthase 1 gene (ASS1). Young mammals, including piglets, have a particularly high requirement of arginine for growth and metabolic function (Flynn and Wu, 1997). L-Arginine is also the biological precursor of nitric oxide (NO), and alteration of arginine uptake and metabolism has been found to be associated with inflammatory bowel diseases, (Stuehr, 2004; Luiking et al., 2012; Coburn et al., 2016). A number of studies have reported that arginine administration significantly attenuate intestinal inflammation associated with down-regulation of the JAK-STAT signalling pathway and increased growth performance and survival in pigs (Wu et al., 2010; Zheng et al., 2018; Che et al., 2019: Luiking et al., 2012; Zhang et al., 2019). Reports of reduced arginine availability in conditions of acute and chronic stress, often associated with increase in NOS2 activity, aligns with the results observed in the current study. The specific mechanisms of regulation and interaction between cortisol, NOS, immune response, and arginine metabolism remain unknown, but could provide further evidence to suggest that arginine requirement should be carefully evaluated when designing diets to support pigs during the weaning transition.
Finally, although the current study has identified a large number of transcripts and pathways regulated at the mRNA level, post transcriptional and post translational mechanisms could also regulate the host response to weaning and should be investigated in future studies as a complementary approach to transcriptomic methods.
Weaning is a multifactorial event that results in complex interactions between gut, brain and metabolism. Understanding these responses and the molecular mechanisms that underpins these changes is critical to improve sustainable pig production. This study has identified multiple genes and pathways differentially regulated by weaning. These results revealed that pigs going through the weaning transition undergo a transient period of inflammatory state with temporary breakdown of barrier functions in the gut. The condition of the inflamed gut have been previously shown to provide favourable growth advantage for the expansion of Enterobacteriaceae, a leading cause of enteric disease in pigs. Under the experimental and controlled conditions of this trial, differential gene expression returned to unweaned control levels by day 14 post weaning. However, the translation of the study results to commercial production setting remains to be explored.
Indicators of weaning stress and response have previously been used including histology, systemic markers of immunity and characterisation of the microbiota composition. Here, we have identified a number of target genes and pathways that could also be used as biomarkers of intestinal inflammation to complement these measures. Together, these could provide valuable tools to monitor host response post weaning, especially in context of intervention strategies aimed to reduce antibiotic use and improve pig health and performance. Finally, as weaning in pigs have been used as a model for stress-related bowel dysfunction in humans, it would be of interest to investigate if the similar transcriptomic changes are involved in these disorders.
The datasets presented in this study can be found in online repositories. The names of the repository/repositories and accession number(s) can be found below: European Nucleotide Archive (ENA) with the accession number PRJEB54752.
All trials and procedures were reviewed and approved by the University of Nottingham ethics committee, the Animal Welfare and Ethical Review Body (AWERB).
All authors listed have made a substantial, direct, and intellectual contribution to the work and approved it for publication.
We thank the Nottingham Trent University and Lallemand for funding.
We acknowledge the team at Bio-Support Unit at the University of Nottingham for help in running the trial. We thank Prof. Ian Connerton for critical reading and review of the manuscript.
The authors declare that the research was conducted in the absence of any commercial or financial relationships that could be construed as a potential conflict of interest.
All claims expressed in this article are solely those of the authors and do not necessarily represent those of their affiliated organizations, or those of the publisher, the editors and the reviewers. Any product that may be evaluated in this article, or claim that may be made by its manufacturer, is not guaranteed or endorsed by the publisher.
The Supplementary Material for this article can be found online at: https://www.frontiersin.org/articles/10.3389/fgene.2022.961474/full#supplementary-material
Ayuso, M., Fernández, A., Núñez, Y., Benitez, R., Isabel, B., Barragán, C., et al. (2015). Comparative analysis of muscle transcriptome between pig genotypes identifies genes and regulatory mechanisms associated to growth, Fatness and metabolism. PLoS One 10, e0145162. doi:10.1371/journal.pone.0145162
Benjamini, Y., and Hochberg, Y. (1995). Controlling the false discovery rate: A practical and powerful approach to multiple testing. J. R. Stat. Soc. Ser. B 57, 289–300. doi:10.1111/j.2517-6161.1995.tb02031.x
Bian, G., Ma, S., Zhu, Z., Su, Y., Zoetendal, E. G., Mackie, R., et al. (2016). Age, introduction of solid feed and weaning are more important determinants of gut bacterial succession in piglets than breed and nursing mother as revealed by a reciprocal cross-fostering model. Environ. Microbiol. 18, 1566–1577. doi:10.1111/1462-2920.13272
Bontempo, V., Di Giancamillo, A., Savoini, G., Dell’Orto, V., and Domeneghini, C. (2006). Live yeast dietary supplementation acts upon intestinal morpho-functional aspects and growth in weanling piglets. Anim. Feed Sci. Technol. 129, 224–236. doi:10.1016/j.anifeedsci.2005.12.015
Bray, N. L., Pimentel, H., Melsted, P., and Pachter, L. (2016). Near-optimal probabilistic RNA-seq quantification. Nat. Biotechnol. 34, 525–527. doi:10.1038/nbt.3519
Che, D., Adams, S., Zhao, B., Qin, G., and Jiang, H. (2019). Effects of dietary L-arginine supplementation from conception to post- weaning in piglets. Curr. Protein Pept. Sci. 20, 736–749. doi:10.2174/1389203720666190125104959
Coburn, L. A., Horst, S. N., Allaman, M. M., Brown, C. T., Williams, C. S., Hodges, M. E., et al. (2016). L-arginine availability and metabolism is altered in ulcerative colitis. Inflamm. Bowel Dis. 22, 1847–1858. doi:10.1097/MIB.0000000000000790
Collins, C. L., Pluske, J. R., Morrison, R. S., McDonald, T. N., Smits, R. J., Henman, D. J., et al. (2017). Post-weaning and whole-of-life performance of pigs is determined by live weight at weaning and the complexity of the diet fed after weaning. Anim. Nutr. (Zhongguo xu mu shou yi xue hui) 3, 372–379. doi:10.1016/j.aninu.2017.01.001
Flynn, N. E., and Wu, G. (1997). Glucocorticoids play an important role in mediating the enhanced metabolism of arginine and glutamine in enterocytes of postweaning pigs. J. Nutr. 127, 732–737. doi:10.1093/jn/127.5.732
Frese, S. A., Parker, K., Calvert, C. C., and Mills, D. A. (2015). Diet shapes the gut microbiome of pigs during nursing and weaning. Microbiome 3, 28. doi:10.1186/s40168-015-0091-8
Gondret, F., Vincent, A., Houée-Bigot, M., Siegel, A., Lagarrigue, S., Causeur, D., et al. (2017). A transcriptome multi-tissue analysis identifies biological pathways and genes associated with variations in feed efficiency of growing pigs. BMC Genomics 18, 244. doi:10.1186/s12864-017-3639-0
Gresse, R., Chaucheyras-Durand, F., Fleury, M. A., Van de Wiele, T., Forano, E., and Blanquet-Diot, S. (2017). Gut microbiota dysbiosis in postweaning piglets: Understanding the keys to health. Trends Microbiol. 25, 851–873. doi:10.1016/j.tim.2017.05.004
Guevarra, R. B., Hong, S. H., Cho, J. H., Kim, B. R., Shin, J., Lee, J. H., et al. (2018a). The dynamics of the piglet gut microbiome during the weaning transition in association with health and nutrition. J. Anim. Sci. Biotechnol. 9, 54–59. doi:10.1186/s40104-018-0269-6
Guevarra, R. B., Hong, S. H., Cho, J. H., Kim, B. R., Shin, J., Lee, J. H., et al. (2018b). The dynamics of the piglet gut microbiome during the weaning transition in association with health and nutrition. J. Anim. Sci. Biotechnol. 9, 54. doi:10.1186/s40104-018-0269-6
Guevarra, R. B., Lee, J. H., Lee, S. H., Seok, M. J., Kim, D. W., Kang, B. N., et al. (2019). Piglet gut microbial shifts early in life: Causes and effects. J. Anim. Sci. Biotechnol. 10, 1. doi:10.1186/s40104-018-0308-3
Han, G. G., Lee, J. Y., Jin, G. D., Park, J., Choi, Y. H., Kang, S. K., et al. (2018). Tracing of the fecal microbiota of commercial pigs at five growth stages from birth to shipment. Sci. Rep. 8, 6012. doi:10.1038/s41598-018-24508-7
Henchion, M., Hayes, M., Mullen, A., Fenelon, M., and Tiwari, B. (2017). Future protein supply and demand: Strategies and factors influencing a sustainable equilibrium. Foods 6, E53. doi:10.3390/foods6070053
Jing, L., Hou, Y., Wu, H., Miao, Y., Li, X., Cao, J., et al. (2015). Transcriptome analysis of mRNA and miRNA in skeletal muscle indicates an important network for differential Residual Feed Intake in pigs. Sci. Rep. 5, 11953. doi:10.1038/srep11953
Ke, S., Fang, S., He, M., Huang, X., Yang, H., Yang, B., et al. (2019). Age-based dynamic changes of phylogenetic composition and interaction networks of health pig gut microbiome feeding in a uniformed condition. BMC Vet. Res. 15, 172–185. doi:10.1186/s12917-019-1918-5
Lallès, J.-P. (2008). Nutrition and gut health of the young pig around weaning : What news. Arch. Zootech. 11, 5–15.
Lallès, J. P., Bosi, P., Smidt, H., and Stokes, C. R. (2007). Nutritional management of gut health in pigs around weaning. Proc. Nutr. Soc. 66, 260–268. doi:10.1017/S0029665107005484
Le Bon, M., Davies, H. E., Glynn, C., Thompson, C., Madden, M., Wiseman, J., et al. (2010). Influence of probiotics on gut health in the weaned pig. Livest. Sci. 133, 179–181. doi:10.1016/j.livsci.2010.06.058
Lessard, M., Dupuis, M., Gagnon, N., Nadeau, É., Matte, J. J., Goulet, J., et al. (2009). Administration of Pediococcus acidilactici or Saccharomyces cerevisiae boulardii modulates development of porcine mucosal immunity and reduces intestinal bacterial translocation after Escherichia coli challenge. J. Anim. Sci. 87, 922–934. doi:10.2527/jas.2008-0919
Luiking, Y. C., Ten Have, G. A. M., Wolfe, R. R., and Deutz, N. E. P. (2012). Arginine de novo and nitric oxide production in disease states. Am. J. Physiol. Endocrinol. Metab. 303, E1177–E1189. doi:10.1152/ajpendo.00284.2012
Luppi, A. (2017). Swine enteric colibacillosis: Diagnosis, therapy and antimicrobial resistance. Porc. Health Manag. 3, 16. doi:10.1186/s40813-017-0063-4
Mach, N., Berri, M., Esquerré, D., Chevaleyre, C., Lemonnier, G., Billon, Y., et al. (2014). Extensive expression differences along porcine small intestine evidenced by transcriptome sequencing. PLoS One 9, e88515. doi:10.1371/journal.pone.0088515
Mach, N., Berri, M., Estellé, J., Levenez, F., Lemonnier, G., Denis, C., et al. (2015). Early-life establishment of the swine gut microbiome and impact on host phenotypes. Environ. Microbiol. Rep. 7, 554–569. doi:10.1111/1758-2229.12285
Macleod, M., Gerber, P., Mottet, A., Tempio, G., Falcucci, A., Opio, C., et al. (2013). Greenhouse gas emissions from pig and chicken supply chains - a global life cycle assessment. Rome, Italy: Food and Agriculture Organization of the United Nations FAO.
Martin, M., 2011. Cutadapt removes adapter sequences from high-throughput sequencing reads. EMBnet. J. 17, 10, doi:10.14806/ej.17.1.200
Matsuo, K., Ota, H., Akamatsu, T., Sugiyama, A., and Katsuyama, T. (1997). Histochemistry of the surface mucous gel layer of the human colon. Gut 40, 782–789. doi:10.1136/gut.40.6.782
Moeser, A. J., Pohl, C. S., and Rajput, M. (2017). Weaning stress and gastrointestinal barrier development: Implications for lifelong gut health in pigs. Anim. Nutr. 3, 313–321. doi:10.1016/j.aninu.2017.06.003
Moeser, A. J., Ryan, K. A., Nighot, P. K., and Blikslager, A. T. (2007). Gastrointestinal dysfunction induced by early weaning is attenuated by delayed weaning and mast cell blockade in pigs. Am. J. Physiol. Gastrointest. Liver Physiol. 293, G413–G421. doi:10.1152/ajpgi.00304.2006
Nowland, T. L., Plush, K. J., Barton, M., and Kirkwood, R. N. (2019). Development and function of the intestinal microbiome and potential implications for pig production. Animals. 9, E76. doi:10.3390/ani9030076
Pié, S., Lallès, J. P., Blazy, F., Laffitte, J., Sève, B., and Oswald, I. P. (2004). Weaning is associated with an upregulation of expression of inflammatory cytokines in the intestine of piglets. J. Nutr. 134, 641–647. doi:10.1093/jn/134.3.641
Pimentel, H., Bray, N. L., Puente, S., Melsted, P., and Pachter, L. (2017). Differential analysis of RNA-seq incorporating quantification uncertainty. Nat. Methods 14, 687–690. doi:10.1038/nmeth.4324
Piórkowska, K., Żukowski, K., Ropka-Molik, K., Tyra, M., and Gurgul, A. (2018). A comprehensive transcriptome analysis of skeletal muscles in two Polish pig breeds differing in fat and meat quality traits. Genet. Mol. Biol. 41, 125–136. doi:10.1590/1678-4685-gmb-2016-0101
Pluske, J. R., Turpin, D. L., and Kim, J. C. (2018). Gastrointestinal tract (gut) health in the young pig. Anim. Nutr. 4, 187–196. doi:10.1016/j.aninu.2017.12.004
Razzuoli, E., Villa, R., Sossi, E., and Amadori, M. (2011). Characterization of the interferon-α response of pigs to the weaning stress. J. Interferon Cytokine Res. 31, 237–247. doi:10.1089/jir.2010.0041
Rhouma, M., Fairbrother, J. M., Beaudry, F., and Letellier, A. (2017). Post weaning diarrhea in pigs: Risk factors and non-colistin-based control strategies. Acta Vet. Scand. 59, 31. doi:10.1186/s13028-017-0299-7
Roura, E., Koopmans, S. J., Lallès, J. P., Le Huerou-Luron, I., De Jager, N., Schuurman, T., et al. (2016). Critical review evaluating the pig as a model for human nutritional physiology. Nutr. Res. Rev. 29, 60–90. doi:10.1017/S0954422416000020
Sciascia, Q., Daş, G., and Metges, C. C. (2016). Review: The pig as a model for humans: Effects of nutritional factors on intestinal function and health. J. Animal Sci. 94, 441–452. doi:10.2527/jas2015-9788
Stuehr, D. J. (2004). Enzymes of the L-arginine to nitric oxide pathway. J. Nutr. 134, 2748S–2751S. doi:10.1093/jn/134.10.2748S
Wang, J., Chen, L., Li, P., Li, X., Zhou, H., Wang, F., et al. (2008). Gene expression is altered in piglet small intestine by weaning and dietary glutamine supplementation. J. Nutr. 138, 1025–1032. doi:10.1093/jn/138.6.1025
Wang, W., Hu, H., Zijlstra, R. T., Zheng, J., and Gänzle, M. G. (2019). Metagenomic reconstructions of gut microbial metabolism in weanling pigs. Microbiome 7, 48. doi:10.1186/s40168-019-0662-1
Wu, X., Ruan, Z., Gao, Y., Yin, Y., Zhou, X., Wang, L., et al. (2010). Dietary supplementation with L-arginine or N-carbamylglutamate enhances intestinal growth and heat shock protein-70 expression in weanling pigs fed a corn-and soybean meal-based diet. Amino Acids 39, 831–839. doi:10.1007/s00726-010-0538-y
Xu, X., Mishra, B., Qin, N., Sun, X., Zhang, S., Yang, J., et al. (2019). Differential transcriptome analysis of early postnatal developing longissimus dorsi muscle from two pig breeds characterized in divergent myofiber traits and fatness. Anim. Biotechnol. 30, 63–74. doi:10.1080/10495398.2018.1437045
Zeng, M. Y., Inohara, N., and Nuñez, G. (2017). Mechanisms of inflammation-driven bacterial dysbiosis in the gut. Mucosal Immunol. 10, 18–26. doi:10.1038/mi.2016.75
Zhang, B., Gan, L., Shahid, M. S., Lv, Z., Fan, H., Liu, D., et al. (2019). In vivo and in vitro protective effect of arginine against intestinal inflammatory response induced by Clostridium perfringens in broiler chickens. J. Anim. Sci. Biotechnol. 10, 73. doi:10.1186/s40104-019-0371-4
Zhang, Q., Widmer, G., and Tzipori, S. (2013). A pig model of the human gastrointestinal tract. Gut Microbes 4, 193–200. doi:10.4161/gmic.23867
Keywords: pig, weaning, RNA-sequencing, transcriptomic, gut, immune response
Citation: Le Bon M, Tötemeyer S, Emes RD and Mellits KH (2022) Gut transcriptome reveals differential gene expression and enriched pathways linked to immune activation in response to weaning in pigs. Front. Genet. 13:961474. doi: 10.3389/fgene.2022.961474
Received: 04 June 2022; Accepted: 24 August 2022;
Published: 24 October 2022.
Edited by:
Suxu Tan, Qingdao University, ChinaReviewed by:
Stafford Vigors, University College Dublin, IrelandCopyright © 2022 Le Bon, Tötemeyer, Emes and Mellits. This is an open-access article distributed under the terms of the Creative Commons Attribution License (CC BY). The use, distribution or reproduction in other forums is permitted, provided the original author(s) and the copyright owner(s) are credited and that the original publication in this journal is cited, in accordance with accepted academic practice. No use, distribution or reproduction is permitted which does not comply with these terms.
*Correspondence: K. H. Mellits, a2VuLm1lbGxpdHNAbm90dGluZ2hhbS5hYy51aw==
Disclaimer: All claims expressed in this article are solely those of the authors and do not necessarily represent those of their affiliated organizations, or those of the publisher, the editors and the reviewers. Any product that may be evaluated in this article or claim that may be made by its manufacturer is not guaranteed or endorsed by the publisher.
Research integrity at Frontiers
Learn more about the work of our research integrity team to safeguard the quality of each article we publish.