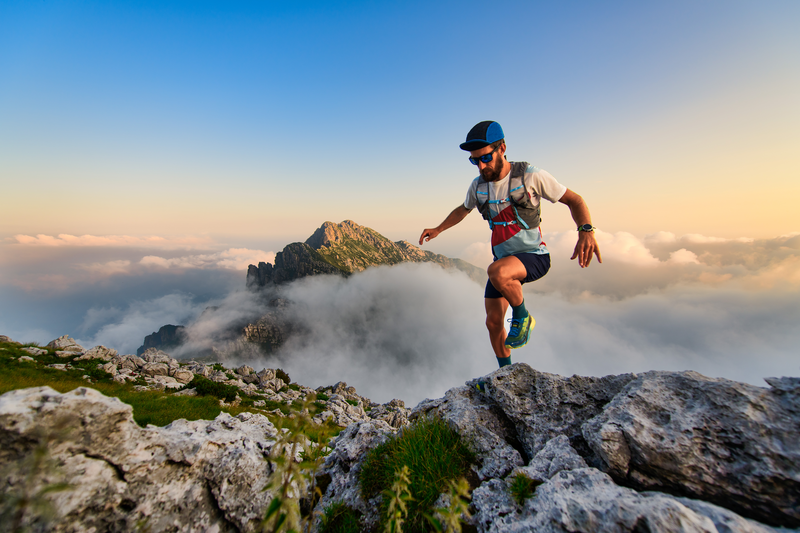
94% of researchers rate our articles as excellent or good
Learn more about the work of our research integrity team to safeguard the quality of each article we publish.
Find out more
EDITORIAL article
Front. Genet. , 15 June 2022
Sec. Livestock Genomics
Volume 13 - 2022 | https://doi.org/10.3389/fgene.2022.942747
This article is part of the Research Topic Epigenetic Variation Influences on Livestock Production and Disease Traits View all 24 articles
Editorial on the Research Topic
Epigenetic Variation Influences on Livestock Production and Disease Traits
The epigenome source of variation modulates gene expression without involving changes to the DNA sequence and is an underutilized source of information that may contribute to improved disease management, reproduction, productivity, and environmental adaptation of livestock. The epigenome which comprises DNA methylation, histone tail modifications, chromatin remodeling, and non-coding RNA species that can transmit epigenetic information (e.g. microRNAs and long non-coding RNAs, etc.) responds to environmental factors (nutrition, pathogens, and climate, etc.) to influence the expression of genes and the emergence of specific phenotypes. Increasing evidence indicates that phenotypic expression results from multi-level interactions between the genome, epigenome, environmental factors, and other non-genetic factors. Furthermore, numerous lines of evidence suggest the influence of epigenome variation on livestock production (e.g. milk production), disease traits, reproduction, and environmental adaptation. Given that livestock breeding depends heavily on the interaction between host genetics, environment (e.g. cold or warm climate, nutrition, pathogens, etc.), physiology (e.g. age) and management practices (e.g. type of feed material, form/quantity of feed), many of which impact the epigenome, it is imperative that attention should be given to epigenome characterization and application in livestock breeding and disease management. Therefore, the aim of this Research Topic is to collect research data on the influences of epigenome alterations (DNA methylation, RNA methylation, histone tail modifications and chromatin remodeling) on livestock production traits, to facilitate gainful use of this important source of variation to support continued improvement in livestock traits and disease management. Non-coding RNA data on livestock is already covered by other Research Topics and is excluded from this topic.
This Research Topic collates four review articles and 19 original research articles covering major topics in epigenetics (DNA methylation, RNA methylation, and histone modification) in relation to livestock health, reproduction, production and response to stress and environmental perturbations in many livestock species, including cattle (seven articles), chicken (five articles), sheep (three articles), pig (two articles), yak (one article), and pearl oyster (one article). Although, many livestock species and a broad range of epigenomics alterations are covered, there is a bias towards DNA methylation alterations and more concentration on cattle, highlighting the need to widen/intensify research efforts to include all livestock species as well as address more issues of health and productivity, which is vital for the effective exploitation of epigenetic information for livestock trait improvement and health management.
The review articles critically examine available epigenetics research data and impacts on livestock health, productivity, reproduction, stress adaptation, and reprograming during somatic cell nuclear transfer. Wang and Ibeagha-Awemu (Wang and Ibeagha-Awemu) present the recent discoveries and evidence on how the epigenetic processes due to DNA methylation, n6 methyladenosine (m6A) RNA methylation, histone modification, and chromatin remodeling impacts the health and productivity of farm animals, particularly cattle, pig, goat, sheep, and poultry. The investigations collated, examined DNA-methylation alterations mostly at the genome-wide scale and to a lesser extent at specific gene regions and addressed animal response to environmental stressors, nutrition, disease pathogens, and developmental processes. They further discuss the application of epigenetics data in livestock health and production and present gaps in livestock epigenetics research. Given increasing evidence that supports the concept that some acquired traits result from environmental exposure during early embryonic and fetal development (i.e., fetal programming), and which can be stamped in the germline as epigenetic information and transmitted to subsequent generations, Zhu et al., discuss the effects of parental environmental exposures on the epigenetics (DNA methylation, histone modification, chromatin remodeling, and non-coding RNAs regulation) of gametes and the early embryo, and available evidence for transgenerational ineritance in livestock (Zhu et al.). In the same light, Wu and Sirard (Wu and Sirard) present available information on the parental non-genetic effects retained in gametes on the development of the early embryo of cows (dairy), paying particular attention to the metabolism of the mother at the time of conception, in vitro culture conditions, and parental age relative to puberty. Examining an assisted reproduction technology, somatic cell nuclear transfer, that is commonly used for the production of cloned animals but with the limitation of low cloning efficiency, Wang et al. presents available data proving that incomplete epigenetic reprogramming contributes to the low developmental potential of cloned embryos, and also describes the regulation of epigenetic reprogramming by long non-coding RNAs (Wang et al.).
A majority of the original research articles (65%) investigate how DNA methylation or m6A RNA methylation alterations play essential roles in the regulation of various livestock traits. Two articles present DNA methylation alterations in response to disease pathogens in cattle. Wang et al. present evidence of significant DNA methylation alterations in mammary gland quarters of Chinese Holsteins challenged with Staphylococcus aureus compared with non-challenged quarters of the same animals, as well as significant enrichment of several differentially methylated and expressed genes with immune functions (e.g. IL6R, TNF, BTK, IL1R2, and TNFSF8) in several immune-related pathways indicative of their involvement in cow’s response to Staphylococcus aureus infection (Wang et al.). Meanwhile, the role of Mycobacterium avium subsp paratuberculosis (MAP) infection on the epigenetic modeling of gut immunity during the progression of Johne’s Disease (JD) is presented with evidence of tissue-specific responses to MAP infection with more DNA methylation alterations (more differentially methylated cytosines and differentially methylated regions) in the ileum lymph node tissue than in the ileum tissue of cows with subclinical JD (Ibeagha-Awemu et al.). Five articles discuss the effects of different stressors or environment change on DNA methylation patterns in livestock, such as differential DNA methylation changes due to the effect of different incubation temperatures and CO2 levels in chicken cardiac muscle (Corbett et al.); or due to weaning stress in piglet peripheral blood mononuclear cells (Corbett et al.); or due to heat stress in cattle (Del Corvo et al.); or due to long term stress in chicken red blood cells (Pértille et al.); or due to water depth variation (environment change) in pearl oyster (Pinctada margaritifera var. cumingii) resulting in shell color variation (Stenger et al.). With regards to product quality, Zhao et al. present the impact of DNA methylation alterations on beef tenderness as well as novel epigenetic data associated with beef quality and further insights into meat science and muscle biology (Zhao et al.). DNA methylation alterations known to influence reproduction traits is the subject of three articles. Perrier et al. report the effects of early life plane of nutrition of Holstein calves on sperm DNA methylation patterns post-puberty (Perrier et al.); while Khezri et al. examine the effect of age and sperm cell condition (fresh or frozen-thawed), demonstrating a significant decrease in the percentage of DNA fragmented sperm cells, and more sperm DNA methylation with increasing age of the bull at differentially methylated regions found between 14 and 17 months of age (Khezri et al.). Examining sheep reproduction traits, Yao et al. demonstrate significant DNA methylation and gene expression changes between high prolificacy (FecBB) and low prolificacy (FecB+) Hu sheep, thereby demonstrating that DNA methylation influences prolificacy capacity by modulating gene expression in the ovaries (Yao et al.). Deviating from a focus on single DNA sites/regions or tissues, Hazard and colleagues show that global DNA methylation rate (GDMR) in blood varies among animals, but is significantly higher in female lambs compared to male lambs, and is moderately heritable and associates significantly with quantitative trait loci harboring genes with known active roles in gene expression (Hazard et al.). Meanwhile, Drouilhet et al. demonstrate significant GDMR variability in sheep blood due to sampling date and breed, and between blood and various tissues (12 somatic tissues and 6 reproductive tissues) (Drouilhet et al.).
In addition to presenting the effects of DNA methylation, the effect of RNA methylation, especially m6A RNA methylation, also known to participate in post-transcriptional gene regulation, is discussed. Combining methylated RNA immunoprecipitation sequencing (MeRIP-seq) and RNA sequencing (RNA-Seq) data, Zhang and colleagues show that genes with up-regulated m6A peaks but with decreased expression, are mainly enriched in the Wnt signaling pathway, suggesting that this pathway may be modified by m6A methylation in Clostridium perfringens beta2 toxin-induced porcine intestinal epithelial (IPEC-J2) cells (Zhang et al.). Similarly, Zhang et al. present a positive association between m6A methylation abundance (MeRIP-seq) and levels of gene expression (RNA-Seq), as well as a vital role of m6A methylation in the modulation of gene expression during yak adipocyte differentiation (Zhang et al.).
The effects of histone modifications, the second most studied epigenetic mechanism, is presented in relation to heat stress, disease/disease pathogens and thermal manipulation. Addressing two active histone modifications, H3K4me3 (predominantly marks active promoters) and H3K27ac (marks both active promoters and enhancers), Chanthavixay et al. present higher differences in histone modifications in the bursal of Leghorns compared to Fayoumis chickens in response to treatment with Newcastle disease virus while under chronic heat stress (Chanthavixay et al.), while David and colleagues show that histone marks (H3K4me3 and H3K27me3) known to contribute to environmental memory in eukaryotes, presents higher numbers of differential peaks in the hypothalamus of 35 days old (slaughter age) chickens with implicated peaks found on genes with metabolic, neurodevelopmental, and gene regulation functions, but fewer differences between peaks of the histone marks in muscle tissue in response to thermal stress during embryogenesis (David et al.). Examining liver tissue between healthy and fatty liver hemorrhagic syndrome (FLHS) chickens, Zhu et al. present H3K27ac differential peaks association with multiple known FLHS risk genes with roles in the immune response, and lipid and energy metabolism (Zhu et al.). On a more general note, Zheng et al. present transcriptional changes and the taxonomic status of a new Chinese native cattle known as Wandong (Zheng et al.)
These reports unequivocally present evidence of the involvement of epigenetic marks in defining livestock trait expression and their importance in modulating economically important livestock traits, products, and environmental adaptation. Concerted efforts and more targeted epigenetic research in livestock spanning the exposome (nutrition, disease pathogens, microbiota, chemicals, pollutants, and environmental stress [heat, cold, and climate chane], parental stress, maternal behavior and management practices), species, breeds (genetic variation), and under various conditions will provide a holistic view and understanding of the epigenetic influence on phenotypic variation in livestock production, reproduction, health and environmental adaptation, and gainful exploitation for production and health management.
EMI-A initiated the Research Topic. HK, SM, and GL co-edited the Research Topic with EMI-A and all authors participated in the editorial process. EMI-A drafted the manuscript. All authors revised and approved the manuscript.
The authors declare that the research was conducted in the absence of any commercial or financial relationships that could be construed as a potential conflict of interest.
All claims expressed in this article are solely those of the authors and do not necessarily represent those of their affiliated organizations, or those of the publisher, the editors and the reviewers. Any product that may be evaluated in this article, or claim that may be made by its manufacturer, is not guaranteed or endorsed by the publisher.
Chanthavixay, G., Kern, C., Wang, Y., Saelao, P., Lamont, S. J., Gallardo, R. A., et al. (2020). Integrated Transcriptome and Histone Modification Analysis Reveals NDV Infection under Heat Stress Affects Bursa Development and Proliferation in Susceptible Chicken Line. Front. Genet. 11, 567812. doi:10.3389/fgene.2020.567812
Corbett, R. J., Luttman, A. M., Wurtz, K. E., Siegford, J. M., Raney, N. E., Ford, L. M., et al. (2021). Weaning Induces Stress-dependent DNA Methylation and Transcriptional Changes in Piglet PBMCs. Front. Genet. 12, 633564. doi:10.3389/fgene.2021.633564
Corbett, R. J., te Pas, M. F. W., van den Brand, H., Groenen, M. A. M., Crooijmans, R. P. M. A., Ernst, C. W., et al. (2020). Genome-Wide Assessment of DNA Methylation in Chicken Cardiac Tissue Exposed to Different Incubation Temperatures and CO2 Levels. Front. Genet. 11, 558189. doi:10.3389/fgene.2020.558189
David, S-A., Vitorino Carvalho, A., Gimonnet, C., Brionne, A., Hennequet-Antier, C., Piégu, B., et al. 2019. Thermal Manipulation during Embryogenesis Impacts H3K4me3 and H3K27me3 Histone Marks in Chicken Hypothalamus. NIH 10:01207. doi:10.3389/fgene.2019.01207
Del Corvo, M., Lazzari, B., Capra, E., Zavarez, L., Milanesi, M., Utsunomiya, Y. T., et al. (2021). Methylome Patterns of Cattle Adaptation to Heat Stress. Front. Genet. 12, 633132. doi:10.3389/fgene.2021.633132
Drouilhet, L., Moreno, C., Plisson-Petit, F., Marcon, D., Fabre, S., and Hazard, D. (2022). Variability in Global DNA Methylation Rate across Tissues and over Time in Sheep. Front. Genet. 13, 791283. doi:10.3389/fgene.2022.791283
Hazard, D., Plisson-Petit, F., Moreno-Romieux, C., Fabre, S., and Drouilhet, L. (2020). Genetic Determinism Exists for the Global DNA Methylation Rate in Sheep. Front. Genet. 11, 616960. doi:10.3389/fgene.2020.616960
Ibeagha-Awemu, E. M., Bissonnette, N., Bhattarai, S., Wang, M., Dudemaine, P-L., McKay, S., et al. (2021). Whole Genome Methylation Analysis Reveals Role of DNA Methylation in Cow’s Ileal and Ileal Lymph Node Responses to Mycobacterium avium Subsp. Paratuberculosis Infection. Front. Genet. 12.135 doi:10.3389/fgene.2021.797490
Khezri, A., Narud, B., Stenseth, E.-B., Zeremichael, T. T., Myromslien, F. D., Wilson, R. C., et al. (2020). Sperm DNA Hypomethylation Proximal to Reproduction Pathway Genes in Maturing Elite Norwegian Red Bulls. Front. Genet. 11, 922. doi:10.3389/fgene.2020.00922
Perrier, J.-P., Kenny, D. A., Chaulot-Talmon, A., Byrne, C. J., Sellem, E., Jouneau, L., et al. (2020). Accelerating Onset of Puberty through Modification of Early Life Nutrition Induces Modest but Persistent Changes in Bull Sperm DNA Methylation Profiles Post-puberty. Front. Genet. 11, 945. doi:10.3389/fgene.2020.00945
Pértille, F., Ibelli, A. M. G., Sharif, M. E., Poleti, M. D., Fröhlich, A. S., Rezaei, S., et al. (2020). Putative Epigenetic Biomarkers of Stress in Red Blood Cells of Chickens Reared across Different Biomes. Front. Genet. 11, 508809. doi:10.3389/fgene.2020.508809
Stenger, P.-L., Ky, C.-L., Reisser, C. M. O., Cosseau, C., Grunau, C., Mege, M., et al. (2021). Environmentally Driven Color Variation in the Pearl Oyster Pinctada Margaritifera Var. Cumingii (Linnaeus, 1758) Is Associated with Differential Methylation of CpGs in Pigment- and Biomineralization-Related Genes. Front. Genet. 12, 630290. doi:10.3389/fgene.2021.630290
Wang, M., and Ibeagha-Awemu, E. M. (2021). Impacts of Epigenetic Processes on the Health and Productivity of Livestock. Front. Genet. 11, 1812. doi:10.3389/fgene.2020.613636
Wang, M., Liang, Y., Ibeagha-Awemu, E. M., Li, M., Zhang, H., Chen, Z., et al. (2020). Genome-Wide DNA Methylation Analysis of Mammary Gland Tissues from Chinese Holstein Cows with Staphylococcus aureus Induced Mastitis. Front. Genet. 11, 550515. doi:10.3389/fgene.2020.550515
Wang, X., Qu, J., Li, J., He, H., Liu, Z., and Huan, Y. (2020). Epigenetic Reprogramming during Somatic Cell Nuclear Transfer: Recent Progress and Future Directions. Front. Genet. 11, 205. doi:10.3389/fgene.2020.00205
Wu, C., and Sirard, M.-A. (2020). Parental Effects on Epigenetic Programming in Gametes and Embryos of Dairy Cows. Front. Genet. 11, 557846. doi:10.3389/fgene.2020.557846
Yao, X., Li, F., Wei, Z., Ei-Samahy, M. A., Feng, X., Yang, F., et al. (2022). Integrative Genome-wide DNA Methylome and Transcriptome Analysis of Ovaries from Hu Sheep with High and Low Prolific. Front. Cell Dev. Biol. 10, 820558. doi:10.3389/fcell.2022.820558
Zhang, J., Yang, Q., Yang, J., Gao, X., Luo, R., Huang, X., et al. (2021). Comprehensive Analysis of Transcriptome-wide m6A Methylome upon Clostridium perfringens Beta2 Toxin Exposure in Porcine Intestinal Epithelial Cells by m6A Sequencing. Front. Genet. 12, 689748. doi:10.3389/fgene.2021.689748
Zhang, Y., Liang, C., Wu, X., Pei, J., Guo, X., Chu, M., et al. (2021). Integrated Study of Transcriptome-wide m6A Methylome Reveals Novel Insights into the Character and Function of m6A Methylation during Yak Adipocyte Differentiation. Front. Cell Dev. Biol. 9, 689067. doi:10.3389/fcell.2021.689067
Zhao, C., Ji, G., Carrillo, J. A., Li, Y., Tian, F., Baldwin, R. L., et al. (2020). The Profiling of DNA Methylation and its Regulation on Divergent Tenderness in Angus Beef Cattle. Front. Genet. 11, 939. doi:10.3389/fgene.2020.00939
Zheng, X.-D., Cheng, J., Qin, W.-J., Balsai, N., Shang, X.-J., Zhang, M.-T., et al. (2020). Whole Transcriptome Analysis Identifies the Taxonomic Status of a New Chinese Native Cattle Breed and Reveals Genes Related to Body Size. Front. Genet. 11, 562855. doi:10.3389/fgene.2020.562855
Zhu, L., Marjani, S. L., and Jiang, Z. (2021). The Epigenetics of Gametes and Early Embryos and Potential Long-Range Consequences in Livestock Species-Filling in the Picture with Epigenomic Analyses. Front. Genet. 12, 557934. doi:10.3389/fgene.2021.557934
Keywords: livestock, DNA/RNA methylation, histone tail modifications, production, reproduction, nutrition, pathogens/health, stress
Citation: Ibeagha-Awemu EM, Kiefer H, McKay S and Liu GE (2022) Editorial: Epigenetic Variation Influences on Livestock Production and Disease Traits. Front. Genet. 13:942747. doi: 10.3389/fgene.2022.942747
Received: 12 May 2022; Accepted: 16 May 2022;
Published: 15 June 2022.
Edited and reviewed by:
Martino Cassandro, University of Padua, ItalyCopyright © 2022 Kiefer, McKay, Liu and Her Majesty the Queen in Right of Canada, as represented by the Minister of Agriculture and Agri-Food Canada for the contribution of Ibeagha-Awemu. This is an open-access article distributed under the terms of the Creative Commons Attribution License (CC BY). The use, distribution or reproduction in other forums is permitted, provided the original author(s) and the copyright owner(s) are credited and that the original publication in this journal is cited, in accordance with accepted academic practice. No use, distribution or reproduction is permitted which does not comply with these terms.
*Correspondence: Eveline M. Ibeagha-Awemu, ZXZlbGluZS5pYmVhZ2hhLWF3ZW11QGFnci5nYy5jYQ==
Disclaimer: All claims expressed in this article are solely those of the authors and do not necessarily represent those of their affiliated organizations, or those of the publisher, the editors and the reviewers. Any product that may be evaluated in this article or claim that may be made by its manufacturer is not guaranteed or endorsed by the publisher.
Research integrity at Frontiers
Learn more about the work of our research integrity team to safeguard the quality of each article we publish.