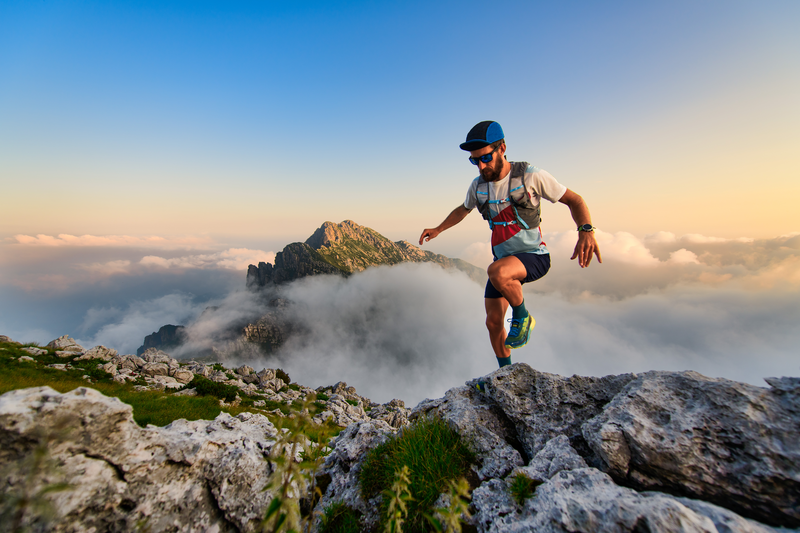
94% of researchers rate our articles as excellent or good
Learn more about the work of our research integrity team to safeguard the quality of each article we publish.
Find out more
ORIGINAL RESEARCH article
Front. Genet. , 11 August 2022
Sec. Genomics of Plants and the Phytoecosystem
Volume 13 - 2022 | https://doi.org/10.3389/fgene.2022.941287
This article is part of the Research Topic Functional Genomic Approaches in Molecular Breeding for Crop Improvement View all 7 articles
A marker-assisted backcrossing program initiated to transfer leaf rust resistance gene LrTrk from Triticum turgidum cv. Trinakria to hexaploid wheat variety HD2932 cotransferred a stripe rust resistance gene, YrTrk, along with LrTrk. The cross of hexaploid recurrent parent HD2932 with tetraploid donor parent Trinakria produced pentaploid F1 plants. F1s were backcrossed with recurrent parent HD2932 to produce BC1F1 generation. Foreground and background selection was conducted in each backcross generation to identify plants for backcrossing or selfing. While foreground selection for LrTrk was carried out with linked and validated molecular marker Xgwm234, for background selection, 86 polymorphic SSR markers from the A and B genomes were used. Single selected plants from BC1F1 and BC2F1 generations backcrossed and selfed to produce BC2F1and BC2F2 generations, respectively. Background selection resulted in 83.72%, 91.86%, and 98.25% of RPG recovery in BC1F1, BC2F1, and BC2F2 generations, respectively. A total of 27 plants with LrTrk in homozygous state were identified in BC2F2 generation and selfed to produce 27 BC2F3 NILs. All the NILs were tested for leaf and stripe rust resistance at the seedling stage using seven Puccinia triticina and one Puccinia striiformis f.sp. tritici rust pathotypes. All the 27 NILs were found to be resistant to both leaf and stripe rust pathotypes. So, these NILs are designated to carry leaf and stripe rust resistance genes LrTrk/YrTrk.
Wheat (Triticum aestivum L.) is one of the most important food crops in the world. It is grown in nearly every region of the world and represents a main source of food for human population and means of livelihood for millions of farmers. India stands second after China in terms of total wheat production. As per the Third Advance Estimates, wheat production in India is estimated at a record 108.75 million metric tons (mmt) for the year 2020–2021 (https://pib.gov.in/PressReleaseIframePage.aspx?PRID=1721692). Although food grain production has increased over the years, India may need to produce 333 million tons of food grain which may include 140 million tons of wheat to feed a projected population of 1.7 billion people by 2050 (https://m.economictimes.com/news/economy/agriculture/india-needs-333-mt-grain-production-to-meet-demand-by-2050/articleshow/50033751.cms). To meet the demand of burgeoning population, wheat production needs to be enhanced. However, several biotic and abiotic factors adversely affect wheat production and it is important to develop varieties that are tolerant or resistant to biotic and abiotic stresses. Among several biotic factors, rust diseases caused by Puccinia spp. are of major economic importance. Three rusts of wheat, black or stem rust caused by Puccinia graminis f.sp. tritici, brown or leaf rust caused by Puccinia triticina (Syn: Puccinia recondita), and yellow or stripe rust caused by Puccinia striiformis f.sp. tritici are known to cause significant damage to wheat crop throughout the world (Line and Chen, 1995; Singh et al., 2002). Leaf rust is a major disease of wheat reducing wheat yields by 40–60% in its severe form on susceptible cultivars. However, over the years, judicious deployment of rust resistance genes has kept the rust diseases under control (Tomar et al., 2014).
Evolution of new virulent races of rust pathogens is a continuing phenomenon which renders resistance genes ineffective and thus necessitates deployment of newer genes. Leaf rust perpetuates for a longer period in wheat growing season as compared to the other two rusts at normal temperature ranging from 15 to 28°C. It occurs in all wheat growing zones of India at various intensities depending on the growth stage of crop and environmental conditions. The impact of leaf rust on yield reduction in wheat is well documented globally, which ranges from 10% under moderate to 65% under intense epidemic, depending on the growth stage of wheat crop when the initial rust infection occurs (Saari and Prescott, 1985). Use of diverse source of genes and development of genetic resistance is the best method to combat these diseases. With the availability of molecular markers, well distributed across all the 21 wheat chromosomes, marker-assisted backcross breeding has emerged as an excellent tool to transfer rust resistance gene(s) in any wheat variety (Mallick et al., 2021). In the past several rust resistance genes such as Lr19, Lr24, Lr28, Sr26, and Yr10 were transferred in wheat varieties, HD2687 (Bhawar et al., 2011), PBW343 (Sharma et al., 2021), HD2932 (Mallick et al., 2015), HD2733 (Singh et al., 2017), and DWR162 (Yadawad et al., 2017) using marker-assisted backcross breeding. One of the examples of wheat varietal improvement using marker-assisted backcross breeding is the development of wheat varieties Unnat PBW343 and Unnat PBW550 resistant to wheat rusts. The wheat variety PBW343 was improved for rust resistance by pyramiding linked resistance genes, Lr37/Yr17/Sr38 and Lr76/Yr70, originating from alien species Aegilops ventricosa and Aegilops umbellulata, respectively (Sharma et al., 2021). The wheat variety Unnat PBW550 is the stripe rust resistant version of old susceptible variety PBW550 and carries stripe rust resistance gene Yr15. A durum wheat variety HI8498 from central India was improved for stem rust resistance by pyramiding genes Sr2 and Sr36 (Prasad et al., 2013).
Several rust resistance genes have been identified and mapped from primary, secondary, and tertiary gene pools at ICAR-IARI, New Delhi (Gireesh et al., 2014, 2015; Niranjana et al., 2017; Nataraj et al., 2018; Dinkar et al., 2020; Rani et al., 2020). One such leaf rust resistance gene named as LrTrk was identified in durum wheat genotype Trinakria and mapped on chromosome 5BS. Leaf rust resistance gene LrTrk was recently transferred in popular wheat variety HD2967 (Mallick et al., 2022). In the current study, LrTrk was transferred into another hexaploid wheat variety HD2932 using marker-assisted selection. Along with LrTrk, stripe rust resistance (not yet mapped) was also transferred from Trinakria. HD2932 is a high-yielding variety developed by ICAR-Indian Agricultural Research Institute, New Delhi and was released for cultivation under late sown, irrigated conditions of central and peninsular zones in 2007.
Plant materials comprised of bread wheat variety HD2932 as recurrent parent and Triticum turgidum var. durum cv. Trinakria as donor parent for leaf and stripe rust resistance. HD2932 was crossed as female parent with Trinakria to produce F1 generation. The heterozygosity of F1 plants was confirmed with SSR marker Xgwm234, linked to leaf rust resistance gene LrTrk in Trinakria. The true hybrids were crossed with HD2932, as male parent to produce BC1F1 generation. Foreground selection in BC1F1 generation was also carried out with linked and validated molecular marker of LrTrk, Xgwm234. Plants carrying LrTrk were further selected for their phenotypic resemblance with HD2932. Ten plants with maximum phenotypic resemblance with HD2932 were used for background selection using SSR markers polymorphic between parents HD2932 and Trinakria. A plant with maximum recurrent parent genome (RPG) recovery was backcrossed with recurrent parent HD2932 to produce BC2F1 generation. Foreground and background selections were also carried out in BC2F1 generation, following the same procedure as it was done in BC1F1 generation. A plant with maximum RPG in BC2F1 generation was selfed to produce BC2F2 generation. Foreground selection was again carried out in BC2F2 generation to identify plants carrying LrTrk in homozygous state. All the plants carrying LrTrk in homozygous state were subjected to background selection and selfed to produce BC2F3 progeny for rust evaluation. The marker-assisted backcross breeding scheme is presented in Figure 1.
FIGURE 1. Schematic representation of marker-assisted backcrossing to introgress rust resistance gene LrTrk/YrTrk in wheat variety HD2932. RP, recurrent parent; DP, donor parent; FS, foreground selection; BS, background selection.
Leaf tissues for DNA isolation were collected from 3 to 4 weeks old plants. DNA was isolated by CTAB method as described by Murray and Thompson (1980). DNA samples were quantified using NanoDrop™ spectrophotometer and diluted to a final concentration of 25 ng/μl of DNA using TE buffer for PCR amplification. The SSR primers for foreground and background selection were diluted to an initial concentration of 1 nmol/µl and further diluted to a working concentration of 5 pmol/µl. While only one (Xgwm234) marker (F: 5′GAGTCCTGATGTGAAGCTGTTG 3′; R: 5′ CTCATTGGGGTGTGTACGTG 3′) was used for foreground selection of leaf rust resistance gene LrTrk, a total of 700 SSR markers belonging to A and B genome of wheat were used for background selection. The PCR amplification was performed in 10 µl reaction volume containing 2 μl of 25 ng/μl gDNA (50 ng), 1 μl of each forward and reverse primers (5 pmol/μl), 3 μl of 2× GoTaq PCR Master Mix (Promega, #M7122), and 3 μl of nuclease-free water in 96-well PCR plates with a thermal seal in an Eppendorf thermal cycler with the following thermal profile: one cycle of 4 min at 94°C (initial denaturation), followed by 35 cycles of 30 s at 94°C (denaturation), 30 s at 50–60°C (vary according to primer annealing, 55°C for Xgwm234), and 30 s at 72°C and 10 min at 72°C (a final extension). PCR products were resolved on 3.5% agarose gel and visualized on a UV trans-illuminator Gel Documentation System (G: Box, Syngene). The RPG percentage in each backcross generation was calculated using the following formula:
The near isogenic lines developed using marker-assisted selection were screened with P. triticina and P. striiformis f.sp. tritici pathotypes in BC2F3 generation. Seven leaf rust pathotypes viz. 77-2, 77-5, 77-3, 77-9, 104, 107-2, and 162-1 and one stripe rust pathotype110S119 were used at seedling stage for screening of NILs. Initial inoculums were obtained from the ICAR-Indian Institute of Wheat and Barley Research (IIWBR), Regional Station, Flowerdale, Shimla, and multiplied on the susceptible wheat cultivar Agra Local at ICAR-IARI, New Delhi for further use.
For leaf and stripe rust screening, the NILs along with parents HD2932 and Trinakria and susceptible check Agra Local were planted in aluminum trays of size 4 × 10 × 3 inches in the glasshouse. Ten-day-old seedlings were inoculated with individual rust pathotypes in isolation by spraying the inoculum with a hand sprayer. The inoculation mixture was prepared by adding uredospores in water with a drop of Tween 20. After inoculation, the trays were kept in humid glass chambers for 48 h and subsequently shifted to glasshouse benches under ambient light and temperature conditions. Rust response (infection type) was recorded 12 days after inoculation, as described by Stakman et al. (1962).
Marker-assisted transfer of leaf rust resistance gene LrTrk in wheat variety HD2932 resulted in identification of NILs (HD2932 + LrTrk/YrTrk) resistant to both leaf and stripe rusts. Crossing of HD2932 with Trinakria produced F1 generation. All the F1 plants were expected to be pentaploids (2n = 5x = 35) as it is a cross between hexaploid wheat variety HD2932 and a tetraploid durum genotype Trinakria. The F1 plants were grown in a net house and were screened with co-dominant SSR marker Xgwm234 linked to leaf rust resistance gene LrTrk. All the F1 plants were found to be heterozygous. These F1 plants were backcrossed to recurrent parent HD2932 to produce BC1F1generation. The marker-assisted backcrossing scheme is presented in Figure 1. The BC1F1 seeds were found to be mixture of completely shriveled seeds to medium and normal filled seeds. One hundred and fifty-six normal looking seeds were planted to raise BC1F1 generation. Foreground selection in BC1F1 generation with Xgwm234 identified 72 plants carrying LrTrk in heterozygous condition (Table 1). Of these 72 plants, 10 plants looking phenotypically similar to recurrent parent HD2932 were selected for background analysis. Parental polymorphism survey with 700 SSR markers was carried out. Of these 700 markers, 86 markers were found to be polymorphic between parents HD2932 and Trinakria. These 86 polymorphic SSR markers were used for marker-assisted background analysis of 10 BC1F1 plants selected phenotypically. Background analysis showed that the RPG recovery in 10 BC1F1 plants varied from 79.65% to 83.72% (Table 1). The plant with maximum RPG recovery (83.72%) in BC1F1 generation was backcrossed with HD2932 to produce BC2F1 generation. The BC2F1 seeds were all normally filled. Foreground analysis of 163 BC2F1 plants with Xgwm234 identified 78 plants with LrTrk in heterozygous state (Table 1). Marker-assisted background analysis of 10 plants looking phenotypically similar to HD2932 in BC2F1 generation showed that RPG recovery varied from 88.37% to 91.86% (Table 1). The plant with 91.86% of RPG was selfed to produce BC2F2 generation. Foreground selection in BC2F2 generation identified 27 plants homozygous for LrTrk and 65 plants, heterozygous for LrTrk (Table 1). Representative gel picture of foreground selection is presented in Figure 2. Background analysis of 27 plants carrying LrTrk in homozygous state revealed that RPG ranged from 95.93% to 98.25% (Table 1). Thus with foreground, background, and phenotypic selection, NILs of wheat variety HD2932 with more than 95% RPG were developed.
TABLE 1. Number of plants identified of carrying leaf rust resistance gene LrTrk in each backcross generations and their maximum genome recovery percentage.
FIGURE 2. Representative gel picture of foreground selection for leaf rust resistance gene LrTrk with linked marker Xgwm234 in BC2F2 generation. P1: HD2932 (RP), P2: Trinakria (DP), 1-15: BC2F2 plants, *plants carrying LrTrk in homozygous state.
The near isogenic lines of HD2932 carrying LrTrk, and the parental lines HD2932 and Trinakria along with susceptible check Agra Local were screened for leaf and stripe rust resistance. All the 27 NILs were found to be resistant to both leaf and stripe rust pathotypes used in this study. The leaf rust pathotype 77-9 produced IT “0” in all the NILs, while all other leaf rust pathotypes 77-3, 107-2, 77-2, 104, 162-1, and 77-5 produced IT “;” in all the NILs (Figure 3; Table 2). The recurrent parent HD2932 and susceptible check Agra Local produced susceptible reaction with IT “3” against all the leaf rust pathotypes, whereas the donor parent Trinakria showed IT of “;” with six pathotypes (107-2, 77-2, 104, 162-1, 77-5, and 77-9) and “; 1” with pathotype 77-3 (Table 2). The results showed that all the 27 F2 plants selected based on linked marker Xgwm234 were resistant to seven leaf rust pathotypes. Screening of 27 NILs with single stripe rust pathotype 110S119 showed resistant IT of “0” in all the NILs (Figure 4; Table 2). The parents and susceptible check Agra Local when tested with stripe rust pathotype 110S119 produced IT “3” in HD2932 and Agra Local and “;“ in donor parent Trinakria (Figure 4; Table 2).
FIGURE 3. Representative figure showing infection type (IT) of HD2932 NILs carrying LrTrk/YrTrk with leaf rust pathotype 77-5.
TABLE 2. Response of recurrent parent HD2932, donor parent Trinakria, NILs (HD2932 + LrTrk/YrTrk), and susceptible check Agra Local toward leaf and stripe rust pathotypes.
FIGURE 4. Phenotyping of parents, Trinakria and HD2932, susceptible check Agra Local, and NILs of HD2932 carrying LrTrk/YrTrk with stripe rust pathotype 110S119.
The present investigation demonstrates the successful transfer of leaf and stripe rust resistance gene LrTrk/YrTrk into hexaploid wheat variety HD2932 using marker-assisted backcross breeding. The cross of hexaploids with tetraploid wheat generally produces viable F1s when a genotype with higher ploidy level is used as a maternal parent (Kalous et al., 2015). Here, also F1s were produced by taking hexaploid wheat variety HD2932 as female parent and tetraploid T. turgidum var. durum cv. Trinakria as male parent. The pentaploid F1s (2n = 5x = 35) produced from this type of crosses can be used in backcrossing to either of the parents (Martin et al., 2013; Kalous et al., 2015) but as female parents, as they have low pollen viability because of aneuploidy (Kihara, 1982; Sharma and Gill, 1983). In our study also, the pentaploid F1s were backcrossed with hexaploid recurrent parent HD2932 by taking pentaploid F1s as female parent. The BC1F1 seeds varied for their appearance. This is because of variation in number of D genome chromosomes in plants resulting in aneuploidy causing poorly developed endosperm (Mallick et al., 2022). In BC2F1 generation, seeds were normal with well-developed endosperm.
Foreground selection of leaf rust resistance gene LrTrk was carried out with linked and validated molecular marker Xgwm234. The marker Xgwm234 was mapped at a distance of 6.3 cm from LrTrk on chromosome 5BS (Gireesh et al., 2014).
Background selection in BC1F1, BC2F1, and BC2F2 generations identified plants with maximum RPG of 83.72%, 91.86%, and 98.25%, respectively. Phenotypic selection coupled with marker-assisted background selection resulted in rapid and higher recovery of HD2932 background than the expected average recovery percentage of each backcross generation. The efficacy of phenotypic plus background selection in achieving higher genome recovery with two backcrosses only has been demonstrated in major crops like rice (Ellur et al., 2016; Grover et al., 2020), wheat (Xu et al., 2017; Sharma et al., 2021; Mallick et al., 2022), and maize (Hossain et al., 2018; Zunjare et al., 2018).
The near isogenic lines developed in this program were phenotyped with seven leaf and one stripe rust pathotypes to know the efficacy of marker-assisted selection. As expected, the recurrent parent HD2932 showed susceptible reaction with all the leaf rust pathotypes with IT “3” while the donor parent Trinakria showed resistant reaction (ITs “;” and “; 1”). All the NILs showed high degree of leaf rust resistance with IT “0” and “;” when tested with different leaf rust pathotypes. The susceptible check Agra Local showed susceptible response with IT “3” similar to HD2932. Although marker-assisted foreground selection was carried out for leaf rust resistance gene LrTrk, the NILs developed in this study also showed resistance to stripe rust as well. This is because the durum wheat genotype Trinakria carries resistance to stripe rust also (Mishra et al., 2011). The stripe rust resistance gene in Trinakria is not yet mapped, therefore, it is not possible to say that it is linked with LrTrk, but it seems that in the NILs developed in this study, leaf and stripe rust resistance genes are co-transferred. Therefore, NILs also showed resistant infection type with stripe rust pathotype 110S119. The transfer of stripe rust resistance along with leaf rust resistance gene LrTrk was also observed in some of the NILs of HD2967 (Mallick et al., 2022). The leaf and stripe rust-resistant NILs of HD2932 developed in this study can be used as replacement of wheat variety HD2932. It can also provide rust resistance genes in the superior genetic background for use in wheat breeding programs.
This study demonstrates successful transfer of leaf and stripe rust resistance genes LrTrk/YrTrk from a tetraploid T. turgidum var. durum cv. Trinakria to hexaploid wheat variety HD2932 using marker-assisted backcrossing scheme. The marker Xgwm234 was originally used for transferring the linked leaf rust resistance gene LrTrk, but in the process a stripe rust resistance YrTrk also got transferred. Although these two genes are not tightly linked, as suggested from a previous study, all the NILs developed in the current study showed resistance to both leaf and stripe rusts. These NILs with more than 95% genomic similarity with recurrent parent can be released as such to replace the susceptible variety HD2932 (or) can be used in breeding programs as these genes are present in a superior genetic background.
The original contributions presented in the study are included in the article/Supplementary Material; further inquiries can be directed to the corresponding author.
The experimental research on plants complied with relevant institutional, national, and international guidelines and legislation.
Vinod, NMa, and SKJ contributed to conceptualization and design of the study. MS and JS contributed in rust phenotyping. PA, AM, MC, and SB carried out genotyping. PA, NM, and AC performed the statistical analysis. Vinod, NMa, SKJ, and PA analyzed the results. NMa and Vinod wrote the first draft of the manuscript. All authors contributed to manuscript revision and read and approved the submitted version.
This research work was funded by the Indian Council of Agricultural Research (ICAR) under the project “CRP on Molecular Breeding” (Project Code: 12-143-B).
The authors are grateful to the ICAR-Indian Institute of Wheat and Barley Research, Regional Station, Shimla, for providing pure inoculums of leaf and stripe rust pathogens and the ICAR-Indian Agricultural Research Institute, New Delhi, for facilitating the experiments.
The authors declare that the research was conducted in the absence of any commercial or financial relationships that could be construed as a potential conflict of interest.
All claims expressed in this article are solely those of the authors and do not necessarily represent those of their affiliated organizations, or those of the publisher, the editors, and the reviewers. Any product that may be evaluated in this article, or claim that may be made by its manufacturer, is not guaranteed or endorsed by the publisher.
Bhawar, K. B., Sharma, J. B., Singh, A. K., Sivasamy, M., Singh, M., Prabhu, K. V., et al. (2011). Molecular marker assisted pyramiding of leaf rust resistance genes Lr19 and Lr28 in bread wheat (Triticum aestivum L.) variety HD2687. Indian J. Genet. Plant Breed. 71, 304.
Dinkar, V., Jha, S. K., Mallick, N., Niranjana, M., Agarwal, P., Sharma, J. B., et al. (2020). Molecular mapping of a new recessive wheat leaf rust resistance gene originating from Triticum spelta. Sci. Rep. 10, 22113. doi:10.1038/s41598-020-78679-3
Ellur, R. K., Khanna, A., Bhowmick, P. K., Vinod, K. K., Nagarajan, M., Mondal, K. K., et al. (2016). Marker-aided incorporation of Xa38, a novel bacterial blight resistance gene, in PB1121 and comparison of its resistance spectrum with xa13+ Xa21. Sci. Rep. 6, 29188. doi:10.1038/srep29188
Gireesh, C., Vinod, Sharma, J. B., and Prabhu, K. V. (2015). Genetics and molecular mapping of stem rust resistance in bread wheat line WR95. Euphytica 205, 869–875. doi:10.1007/s10681-015-1428-x
Gireesh, C., Vinod, Sharma, J. B., and Prabhu, K. V. (2014). Inheritance and molecular mapping of leaf rust resistance in Triticum turgidum var. durum cv. Trinakria. Indian J. Genet. Plant Breed. 74, 10. doi:10.5958/j.0975-6906.74.1.002
Grover, N., Kumar, A., Yadav, A. K., Krishnan, S. G., Ellur, R. K., Bhowmick, P. K., et al. (2020). Marker assisted development and characterization of herbicide tolerant near isogenic lines of a mega Basmati rice variety, “Pusa Basmati 1121. Rice 13, 68. doi:10.1186/s12284-020-00423-2
Hossain, F., Muthusamy, V., Pandey, N., Vishwakarma, A. K., Baveja, A., Zunjare, R. U., et al. (2018). Marker-assisted introgression of opaque2 allele for rapid conversion of elite hybrids into quality protein maize. J. Genet. 97, 287–298. doi:10.1007/s12041-018-0914-z
Kalous, J. R., Martin, J. M., Sherman, J. D., Heo, H.-Y., Blake, N. K., Lanning, S. P., et al. (2015). Impact of the D genome and quantitative trait loci on quantitative traits in a spring durum by spring bread wheat cross. Theor. Appl. Genet. 128, 1799–1811. doi:10.1007/s00122-015-2548-3
Kihara, H. (1982). Importance of cytoplasm in plant genetics. Cytol. (Tokyo) 47, 435–450. doi:10.1508/cytologia.47.435
Line, R. F., and Chen, X. (1995). Successes in breeding for and managing durable resistance to wheat rusts. Plant Dis. 79, 1254–1255.
Mallick, N., Agarwal, P., Jha, S. K., Niranjana, M., and Vinod, (2021). Marker-assisted breeding for rust management in wheat. Indian Phytopathol. 74, 365–370. doi:10.1007/s42360-020-00317-9
Mallick, N., Jha, S. K., Agarwal, P., Mall, A., Kumar, S., Choudhary, M. K., et al. (2022). Marker-assisted improvement of bread wheat variety HD2967 for leaf and stripe rust resistance. Plants 11, 1152. doi:10.3390/plants11091152
Mallick, N., Sharma, J. B., Tomar, R. S., Sivasamy, M., and Prabhu, K. V. (2015). Marker-assisted backcross breeding to combine multiple rust resistance in wheat. Plant Breed. 134, 172–177. doi:10.1111/pbr.12242
Martin, A., Simpfendorfer, S., Hare, R. A., and Sutherland, M. W. (2013). Introgression of hexaploid sources of crown rot resistance into durum wheat. Euphytica 192, 463–470. doi:10.1007/s10681-013-0890-6
Mishra, A. N., Yadav, S. R., Shirsekar, G. S., Dubey, V. G., Kaushal, K., and Sai Prasad, S. V. (2011). Diversity for resistance to stem and leaf rusts in Indian wheat germplasm. Indian J. Plant Genet. Resour. 24, 283–291.
Murray, M. G., and Thompson, W. (1980). Rapid isolation of high molecular weight plant DNA. Nucleic Acids Res. 8, 4321–4325. doi:10.1093/nar/8.19.4321
Nataraj, V., Vinod, , Sharma, J. B., Chanwala, J., Mallick, N., Jha, S. K., et al. (2018). Molecular characterization of Triticum militinae derived introgression lines carrying leaf rust resistance. Genet. Resour. Crop Evol. 65, 787–796. doi:10.1007/s10722-017-0572-7
Niranjana, M., VinodSharma, J. B., Mallick, N., Tomar, S. M. S., and Jha, S. K. (2017). Cytogenetic analysis and mapping of leaf rust resistance in Aegilops speltoides Tausch derived bread wheat line Selection2427 carrying putative gametocidal gene (s). Genome 60, 1076–1085. doi:10.1139/gen-2017-0107
Prasad, S. V., Sai, S. S. K., Kumar, V., Kantwa, S. L., Dubey, V. G., Ambati, D., et al. (2013). International Symposium on Genetics and Breeding of Durum Wheat. Spain: Ciheam, 27–30.Pyramiding of resistance genes Sr36 and Sr2 in durum wheat background (HI 8498) through markerassisted selection for resistance to stem rust race 117-group pathotypes
Rani, K., Raghu, B. R., Jha, S. K., Agarwal, P., Mallick, N., Niranjana, M., et al. (2020). A novel leaf rust resistance gene introgressed from Aegilops markgrafii maps on chromosome arm 2AS of wheat. Theor. Appl. Genet. 133, 2685–2694. doi:10.1007/s00122-020-03625-w
Saari, E. E., and Prescott, J. M. (1985). “World distribution in relation to economic losses,” in Diseases, distribution, epidemiology, and control (Netherlands: Elsevier), 259–298.
Sharma, A., Srivastava, P., Mavi, G. S., Kaur, S., Kaur, J., Bala, R., et al. (2021). Resurrection of wheat cultivar PBW343 using marker-assisted gene pyramiding for rust resistance. Front. Plant Sci. 12, 570408. doi:10.3389/fpls.2021.570408
Sharma, H. C., and Gill, B. S. (1983). Current status of wide hybridization in wheat. Euphytica 32, 17–31. doi:10.1007/bf00036860
Singh, M., Mallick, N., Chand, S., Kumari, P., Sharma, J. B., Sivasamy, M., et al. (2017). Marker-assisted pyramiding of Thinopyrum-derived leaf rust resistance genes Lr19 and Lr24 in bread wheat variety HD2733. J. Genet. 96, 951–957. doi:10.1007/s12041-017-0859-7
Singh, R. P., Huerta-Espino, J., Roelfs, A. P., and Curtis, B. C. (2002). The wheat rusts. Growth 2, 35.
Stakman, E. C., Stewart, D. M., and Loegering, W. Q. (1962). Identification of physiologic races of Puccinia graminis var. tritici. Washingt. DC: USDA Agricultural Research Service E617.
Tomar, S. M. S., Singh, S. K., Sivasamy, M., and Vinod, (2014). Wheat rusts in India: Resistance breeding and gene deployment-A review. Indian J. Genet. Plant Breed. 74, 129. doi:10.5958/0975-6906.2014.00150.3
Xu, H., Cao, Y., Xu, Y., Ma, P., Ma, F., Song, L., et al. (2017). Marker-assisted development and evaluation of near-isogenic lines for broad-spectrum powdery mildew resistance gene Pm2b introgressed into different genetic backgrounds of wheat. Front. Plant Sci. 8, 1322. doi:10.3389/fpls.2017.01322
Yadawad, A., Gadpale, A., Hanchinal, R. R., Nadaf, H. L., Desai, S. A., Biradar, S., et al. (2017). Pyramiding of leaf rust resistance genes in bread wheat variety DWR 162 through marker assisted backcrossing. Indian J. Genet. Plant Breed. 77, 251. doi:10.5958/0975-6906.2017.00033.5
Keywords: leaf rust, stripe rust, marker-assisted backcrossing, gene introgression, wheat
Citation: Mallick N, Jha SK, Agarwal P, Kumar S, Mall A, M N, Choudhary MK, Chandra AK, Bansal S, Saharan MS, Sharma JB and Vinod (2022) Marker-assisted transfer of leaf and stripe rust resistance from Triticum turgidum var. durum cv. Trinakria to wheat variety HD2932. Front. Genet. 13:941287. doi: 10.3389/fgene.2022.941287
Received: 11 May 2022; Accepted: 04 July 2022;
Published: 11 August 2022.
Edited by:
Philomin Juliana, International Maize and Wheat Improvement Center, MexicoReviewed by:
Ziya Dumlupinar, Kahramanmaras Sütçü Imam University, TurkeyCopyright © 2022 Mallick, Jha, Agarwal, Kumar, Mall, M, Choudhary, Chandra, Bansal, Saharan, Sharma and Vinod. This is an open-access article distributed under the terms of the Creative Commons Attribution License (CC BY). The use, distribution or reproduction in other forums is permitted, provided the original author(s) and the copyright owner(s) are credited and that the original publication in this journal is cited, in accordance with accepted academic practice. No use, distribution or reproduction is permitted which does not comply with these terms.
*Correspondence: Vinod, dmlub2QuZ2VuZXRpY3NAZ21haWwuY29t
Disclaimer: All claims expressed in this article are solely those of the authors and do not necessarily represent those of their affiliated organizations, or those of the publisher, the editors and the reviewers. Any product that may be evaluated in this article or claim that may be made by its manufacturer is not guaranteed or endorsed by the publisher.
Research integrity at Frontiers
Learn more about the work of our research integrity team to safeguard the quality of each article we publish.