- 1Comparative Orthopaedic and Genetics Research Laboratory, School of Veterinary Medicine, University of Wisconsin-Madison, Madison, WI, United States
- 2Department of Animal and Dairy Sciences, College of Agricultural and Life Sciences, University of Wisconsin-Madison, Madison, WI, United States
- 3Department of Veterinary Integrative Biosciences, College of Veterinary Medicine and Biomedical Sciences, Texas A&M University, College Station, TX, United States
Here, we report the use of genome-wide association study (GWAS) for the analysis of canine whole-genome sequencing (WGS) repository data using breed phenotypes. Single-nucleotide polymorphisms (SNPs) were called from WGS data from 648 dogs that included 119 breeds from the Dog10K Genomes Project. Next, we assigned breed phenotypes for hip dysplasia (Orthopedic Foundation for Animals (OFA) HD, n = 230 dogs from 27 breeds; hospital HD, n = 279 dogs from 38 breeds), elbow dysplasia (ED, n = 230 dogs from 27 breeds), and anterior cruciate ligament rupture (ACL rupture, n = 279 dogs from 38 breeds), the three most important canine spontaneous complex orthopedic diseases. Substantial morbidity is common with these diseases. Previous within- and between-breed GWAS for HD, ED, and ACL rupture using array SNPs have identified disease-associated loci. Individual disease phenotypes are lacking in repository data. There is a critical knowledge gap regarding the optimal approach to undertake categorical GWAS without individual phenotypes. We considered four GWAS approaches: a classical linear mixed model, a haplotype-based model, a binary case-control model, and a weighted least squares model using SNP average allelic frequency. We found that categorical GWAS was able to validate HD candidate loci. Additionally, we discovered novel candidate loci and genes for all three diseases, including FBX025, IL1A, IL1B, COL27A1, SPRED2 (HD), UGDH, FAF1 (ED), TGIF2 (ED & ACL rupture), and IL22, IL26, CSMD1, LDHA, and TNS1 (ACL rupture). Therefore, categorical GWAS of ancestral dog populations may contribute to the understanding of any disease for which breed epidemiological risk data are available, including diseases for which GWAS has not been performed and candidate loci remain elusive.
Introduction
In recent years, organized efforts have assembled large repositories of whole-genome sequencing (WGS) data, such as the international Dog10K Consortium (Ostrander et al., 2019). The Dog10K Consortium aims to generate ∼20x coverage WGS data from 10,000 dogs and use this large dataset to investigate domestication, breed formation, aging, behavior, and morphologic variation. Breed and sex are the only phenotypic data recorded. The number of dogs from each breed in the repository varies from a few individuals to more than 50 dogs. To maximize the use of WGS repository data for the investigation of canine polygenic disease, new statistical approaches are needed for the analysis of breed phenotypes with an unbalanced number of individuals per breed.
In dogs, breed phenotypes rather than individual case-control or quantitative phenotypes have been used to study height, chondrodysplasia, body size, muscling, skull shape, ear type, snout length, fur length and quality, life expectancy, cognition, behavior, cesarean section rate, litter size, stillbirth rate, gestation length, and cancer mortality (Jones et al., 2008; Sutter et al., 2008; Parker et al., 2009; Bannasch et al., 2010; Boyko et al., 2010; Vaysse et al., 2011; Hoopes et al., 2012; Schoenebeck et al., 2012; Hayward et al., 2016; Plassais et al., 2017; Plassais et al., 2019; Smith et al., 2019; Bannasch et al., 2020; Doherty et al., 2020; Gnanadesikan et al., 2020). Domestication has produced dog breeds with substantial phenotypic variation. Weight and height can vary substantially (Supplementary Figure S1). Over 400 breeds exist, defined by behavioral and physical characteristics. Strong artificial selection and population bottlenecks have resulted in breeds with fixed phenotypes, such as chondrodysplasia (Parker et al., 2017).
Disease risk variants have undergone inadvertent selection within breeds due to genetic correlation caused by random fixation, linkage disequilibrium (LD), and pleiotropy (Supplementary Figure S2). High disease prevalence in a breed reflects the enrichment of disease risk alleles during modern breed creation and domestication (Sargan, 2004; Karlsson and Lindblad-Toh 2008). Disease risk variants overrepresented within a breed allow genome-wide association study (GWAS) to be performed using breed phenotypes for the discovery of large effect variants associated with reproductive traits (Smith et al., 2019), longevity (Plassais et al., 2019; Doherty et al., 2020), and cancer (Doherty et al., 2020).
Although single-nucleotide polymorphism (SNP) array data can detect some fixed large-effect genetic variants, such data are underpowered compared to WGS SNPs for the discovery of all potential associations that may exist across many breeds (Hayward et al., 2016). Genetic risk for many complex diseases is also influenced by numerous small to moderate effect loci responsible for within-breed heterogeneity (Supplementary Figure S3) (Hayward et al., 2016; Bannasch et al., 2020). Within a breed, heterogeneity is best captured by within-breed GWAS with individual-level phenotypes (Hayward et al., 2016). Disease-associated variants with lesser effects that are responsible for within-breed phenotypic heterogeneity are not captured by across-breed GWAS with breed phenotypes. Across-breed GWAS with WGS SNPs and breed phenotypes is a useful approach to discover fixed large-effect variants that are shared across breeds that would otherwise go undiscovered. However, this approach has not been investigated as an approach for the genetic discovery of common complex diseases using repository data.
Previous research has used a classical linear mixed model (LMM) approach and removed individuals from overrepresented breeds to balance breed representation (Plassais et al., 2019; Doherty et al., 2020). However, LMMs do not properly support multilevel categorical phenotypes. For this study, we developed a new statistical approach for analyzing repository sequence data with breed phenotypes and unbalanced breed representation. We performed the first GWAS using WGS SNPs and breed phenotypes to discover large-effect genetic variants shared across breeds for the three major economically important canine orthopedic diseases, namely hip dysplasia (HD), elbow dysplasia (ED), and anterior cruciate ligament (ACL) rupture (Johnson et al., 1994; Wilke et al., 2005; Michelsen, 2013). HD, ED, and ACL rupture are complex polygenic diseases with moderate heritability, where disease risk varies considerably between breeds (Witsberger et al., 2008; Oberbauer et al., 2017). Heritability estimates of HD range between 0.1–0.6 (Fries and Remedios 1995; Ginja et al., 2009; Krotscheck and Todhunter 2010; Lewis et al., 2013). For ED, heritability estimates range from 0.10–0.77 (Guthrie and Pidduck, 1990; Beuing et al., 2000; Mäki et al., 2000; Soo et al., 2018) and for ACL rupture, heritability estimates range from 0.27–0.89 (Nielen et al., 2001; Wilke et al., 2006; Baker et al., 2017; Cook et al., 2020). Previous within- and between-breed GWAS for HD, ED, and ACL rupture have identified candidate loci using array SNPs (Pfahler and Distl 2012; Baird et al., 2014; Hayward et al., 2016; Baker et al., 2017; Mikkola et al., 2019). Studies investigating HD and ACL rupture have found associations in genes influencing morphology (Fealey et al., 2017; Baker et al., 2018; Healey et al., 2019; Baker et al., 2021). The discovery of shared across-breed genetic variants for these diseases has not been previously undertaken.
To study WGS SNPs using breed phenotypes and unbalanced breed representation, we used four association models for GWAS of HD, ED, and ACL rupture. We were able to detect new biologically relevant ED associations and validate previously reported associations for HD. We also report HD’s association with genes that have been under selection since domestication. These domestication genes influence traits such as body size, ear phenotype, and behavior. This suggests that HD is a complex trait that may have been directly or indirectly influenced by the selection of morphological traits during canine domestication.
Materials and methods
Whole-genome sequencing samples
High-depth WGS data from 648 canids, including 562 dogs from 119 breeds, were obtained from the Dog10K Consortium (Ostrander et al., 2019). Dog10K performed alignment to CanFam3.1 and called 45,165,129 variants (Ostrander et al., 2019). Variant mean depth, variant missingness, variant quality, individual mean depth, and individual missingness analyses were performed using vcftools (Danecek et al., 2011) and tidyverse in R (Wickham et al., 2019).
Breed filtering
In an across-breed GWAS with breed phenotypes, it is critical that breed assignments are correct. To detect samples with inaccurate breed assignment, we performed neighbor-joining phylogeny analysis. We compared the 562 dogs with a breed assignment to a previously published dataset of 1,110 dogs from 129 breeds with SNP array data (accession: GSE123368, GSE70454, GSE83160, GSE90441, and GSE96736) (Vaysse et al., 2011; Decker et al., 2015; Dreger et al., 2016a; 2016b; Parker et al., 2017; Plassais et al., 2019). VCFtools was used to filter WGS SNPs to retain biallelic SNPs, remove indels, and remove variants with a quality score under 10 (Danecek et al., 2011). SNP locations were extracted from WGS data and merged with SNP array data. 143,706 SNPs were used to calculate 100 bootstrapped pairwise identity-by-state (IBS) distance matrices using PLINK with the –genome and –cluster commands (Purcell et al., 2007; Parker et al., 2017). The neighbor program in PHYLIP drew 100 trees from the bootstrapped IBS matrices (Rambaut 2006; Parker et al., 2017). The consensus program in PHYLIP then combined the 100 trees to build a consensus tree by majority rule (Felsenstein, 1989). FigTree v1.4.4 was used to plot the consensus tree (Rambaut 2006). Any of the 562 dogs with WGS SNPs that did not cluster within their breed as expected were excluded from subsequent analysis.
Breed phenotypes for hip dysplasia, elbow dysplasia, and anterior cruciate ligament rupture
Breed phenotypes for HD, ED, and ACL rupture were assigned based on epidemiological data describing disease prevalence (Witsberger et al., 2008; Oberbauer et al., 2017). Because both publications reported HD prevalence for different breeds and used different methods, we analyzed them separately (Orthopedic Foundation for Animals (OFA) HD prevalence (Oberbauer et al., 2017) and hospital HD prevalence from 27 veterinary teaching hospitals in North America from 1964 to 2003 (Witsberger et al., 2008)).
Individual and variant filtering
VCFtools was used to retain biallelic SNPs, remove indels, and remove variants with a quality score under 20 (Danecek et al., 2011). The quality score threshold of 20 was selected based on earlier work (Plassais et al., 2019). Dogs within the filtered Dog10K dataset were retained if that breed had a reported prevalence for HD, ED, or ACL rupture. Samples and SNPs were then filtered in PLINK (Purcell et al., 2007). Dogs were removed if they had a genotyping rate
Literature search for loci associated with hip dysplasia, elbow dysplasia, and anterior cruciate ligament rupture
A literature search was performed to create a comprehensive list of all previously reported associated loci with HD, ED, and ACL rupture in dogs.
Genome-wide association study
Linear mixed model
A centered relatedness matrix was calculated using GEMMA v0.94.1 for classical LMM association analysis with sex as a covariate (Zhou and Stephens 2012). The Wald test was used to determine P values, and Bonferroni correction (0.05/number of variants) was used to identify significant associations for OFA HD and ED (7,586,942 SNPs) (p < 6.59E-9) (Oberbauer et al., 2017) and hospital HD and ACL rupture (7,026,774 SNPs) (p < 7.12E-9) (Witsberger et al., 2008). All Quantile–Quantile (QQ) and Manhattan plots were constructed using R. For comparison to Bonferroni correction of LMM results, a separate false discovery rate (FDR) correction of P values used the fdrtool in R (Strimmer 2008).
Binary case-control model
A binary association was performed by selecting a group of breeds that are strongly protected from the trait (low-risk controls) and a group of breeds that are strongly predisposed (high-risk cases) for the association. Two different scenarios were compared. Initially, we used a stringent scenario with a full separation of cases and controls to ensure the prevalence cutoffs were disparate. Since a limited number of dogs from low- and high-risk breeds for HD, ED, and ACL rupture were available, we also tested a less stringent scenario where the threshold values produced case and control groups with a larger sample group size. The case-control binary association was performed using GEMMA with a genomic relationship matrix and sex as a covariate (Zhou and Stephens 2012). As described in the GEMMA software, all of the controls are regarded as zero, while all of the cases are regarded as one. When the SNP effect size is small, this approximation is relatively accurate (Zhou et al., 2013). The Wald test was used to determine P values, and Bonferroni correction was used to identify significant associations for OFA HD and ED (stringent binary case-control p < 6.68E-9) (lenient binary case-control p < 6.64E-9) (Oberbauer et al., 2017) and hospital HD and ACL rupture (stringent binary case-control p < 7.26E-9) (lenient binary case-control p < 7.13E-9) (Witsberger et al., 2008).
Haplotype-based model
Fixed width haplotype-based association was performed with PLINK (Purcell et al., 2007). A fixed 4 SNP window was selected based on a preliminary comparison of differing window sizes. The centered relatedness matrices previously generated were used to draw scree plots in R to determine the optimum number of eigenvectors to correct for the population structure after assessment using Catell’s scree test (Cangelosi and Goriely 2007). For the OFA HD and ED datasets, the first six eigenvectors and for the hospital HD and ACL rupture datasets the first eight eigenvectors were incorporated as covariates. When the haplotype-based analysis was performed with a fixed 4 SNP window, 7,586,822 and 7,026,651 sliding windows were analyzed for the OFA (HD and ED) and veterinary hospital (HD and ED) datasets, respectively. A Bonferroni correction was used to identify significant associations for OFA HD and ED (p < 1.68E-9) (Oberbauer et al., 2017) and hospital HD and ACL rupture (p < 1.82E-9) (Witsberger et al., 2008). For comparison to Bonferroni correction of haplotype-based results, a separate FDR correction of P values was performed with fdrtool in R (Strimmer 2008).
Weighted least squares model
We used a simple linear regression model with weights on the averaged genotypes as our final approach to perform categorical GWAS with breed phenotypes. Initially, we computed the average of the SNP dosage for each locus in each dog breed. So, we had an X matrix (n*p) for each disease phenotype where n = number of dog breeds and p = number of SNPs. We then computed the principal components from the genomic relationship matrix and included the first five important principal components in the model. For each breed, the weight was the proportion of the genotyped individuals for each breed in the whole sample population. Finally, we ran the following model and estimated SNP effects in the R environment:
where X = average SNP dosage matrix, y = disease prevalence in the breed, and Wt = proportion of individuals of each breed in the GWAS sample.
For each SNP within a breed, an SNP average allelic frequency was calculated. Because the confidence that the SNP average allelic frequency appropriately represents the true breed average is related to the number of individual dogs within a breed, the GWAS was weighted according to the number of individuals (repeats) within each breed. This model has the advantage of using all the SNP data and appropriately considers variation in the number of dogs in each breed. A Bonferroni correction was used to identify significant associations for OFA HD and ED (p < 6.59E-9) (Oberbauer et al., 2017) and hospital HD and ACL rupture (p < 7.11E-9) (Witsberger et al., 2008).
Gene annotation
The CanFam3.1 build on the UCSC and Ensembl genome browsers was used to identify genes contained within the top candidate loci. Due to the increased density of the WGS SNPs no flanking regions were added for gene annotation.
Results
Whole-genome sequencing, breed, and SNP filtering
Initial assessment of the unfiltered Dog10K variants from 648 canids (n = 5 wolves, n = 33 village/indigenous dogs, n = 562 domestic dogs (119 breeds), n = 14 mixed dogs/breeds, and n = 34 dogs of unknown breed) was performed (Supplementary Table S1, Supplementary Figure S4). Variant mean depth ranged from 0 to 1,541.1, with a mean of 18.8. Variant missingness ranged from 0.15% to 99.85%, with a mean of 7.54%. Variant quality ranged from 30 to 25,177,600, with a mean of 43,170. The minimum mean depth of the samples was 0.01, with a mean of 18.88 and a maximum of 43.19. The percent missingness of the samples ranged from 2.23% to 99.88%, with a mean of 7.54%.
Breed assignment of purebred dogs in the Dog10K dataset was verified by performing neighbor-joining phylogeny, except for 12 dogs which were removed from the dataset (Supplementary Figure S5). Purebred dogs with WGS in Dog10K were then compared to breeds with known prevalence for HD, ED, and ACL rupture (Witsberger et al., 2008; Oberbauer et al., 2017). For OFA HD and ED, there were 230 dogs from 27 breeds (169 males, 54 females, and 7 unknown) with WGS data (Table 1) (Oberbauer et al., 2017). For hospital HD and ACL rupture, there were 279 dogs from 38 breeds (204 males, 56 females, and 19 unknown) with WGS data (Witsberger et al., 2008) (Table 1).
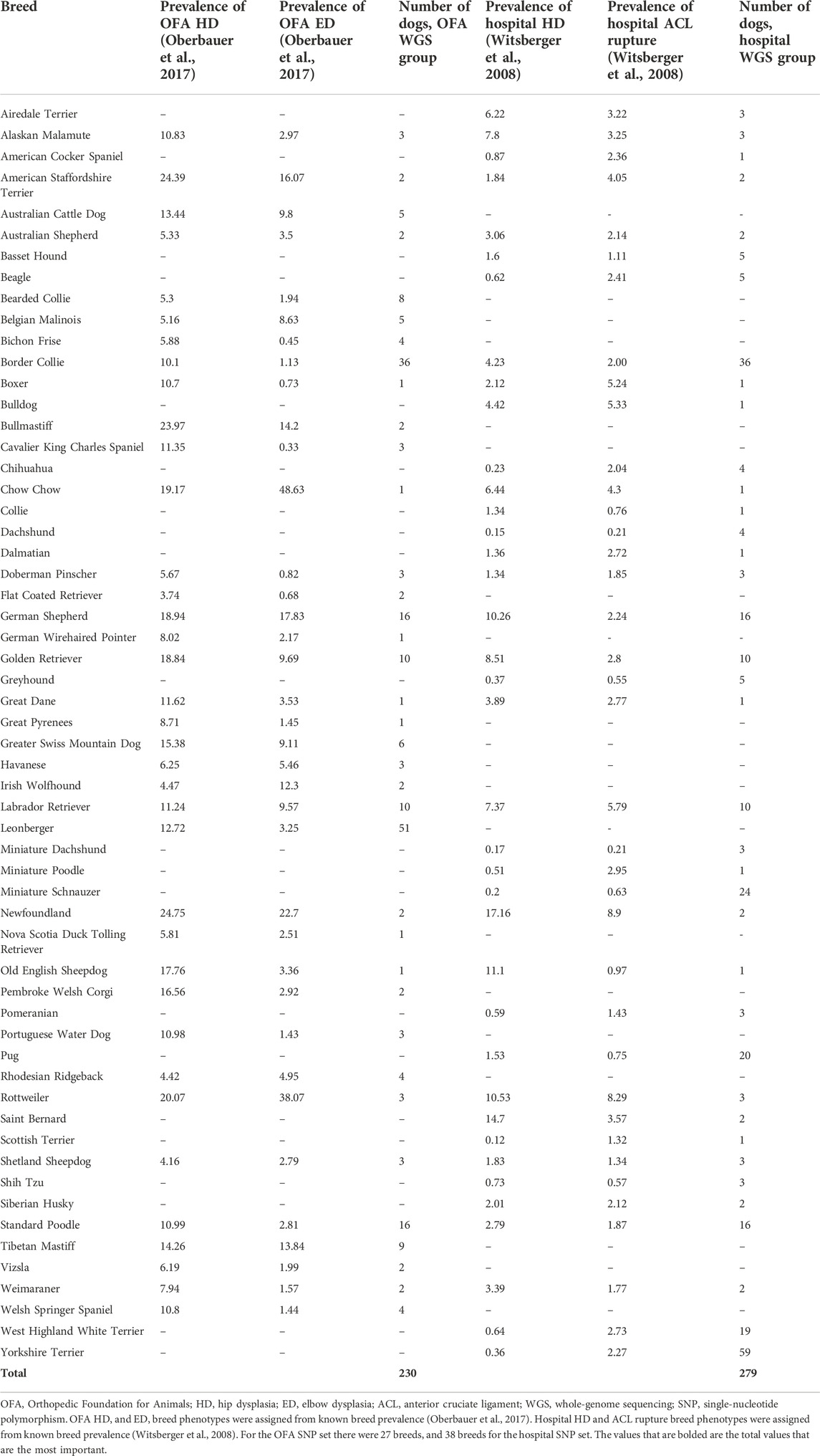
TABLE 1. Breed phenotypes for hip dysplasia, elbow dysplasia, and anterior cruciate ligament rupture.
The 45,165,129 variants in the raw VCF file were filtered to 33,414,513 SNPs for dogs with reported prevalence for HD, ED, and ACL rupture by removing indels and retaining biallelic SNPs with a minimum quality score of 20. After SNP filtering, 7,586,942 SNPs remained in the OFA SNP set, and 7,026,774 SNPs remained in the hospital SNP set (Table 2). No samples had a genotyping rate
Linear mixed genome-wide association study model
The analysis we performed was a classical generalized LMM that was corrected for population structure and sex (Figures 1–4). The number of SNPs that met genome-wide significance after Bonferroni correction was 16, 517, 195, and 219 for the OFA HD, ED, hospital HD, and ACL rupture datasets, respectively. For comparison, separate LMM FDR corrections resulted in 0, 83, 11, and 47 SNPs with significant associations for OFA HD, ED, hospital HD, and ACL rupture (Supplementary Figure S6). The SNP effect estimates are reported in Supplementary Data S5.
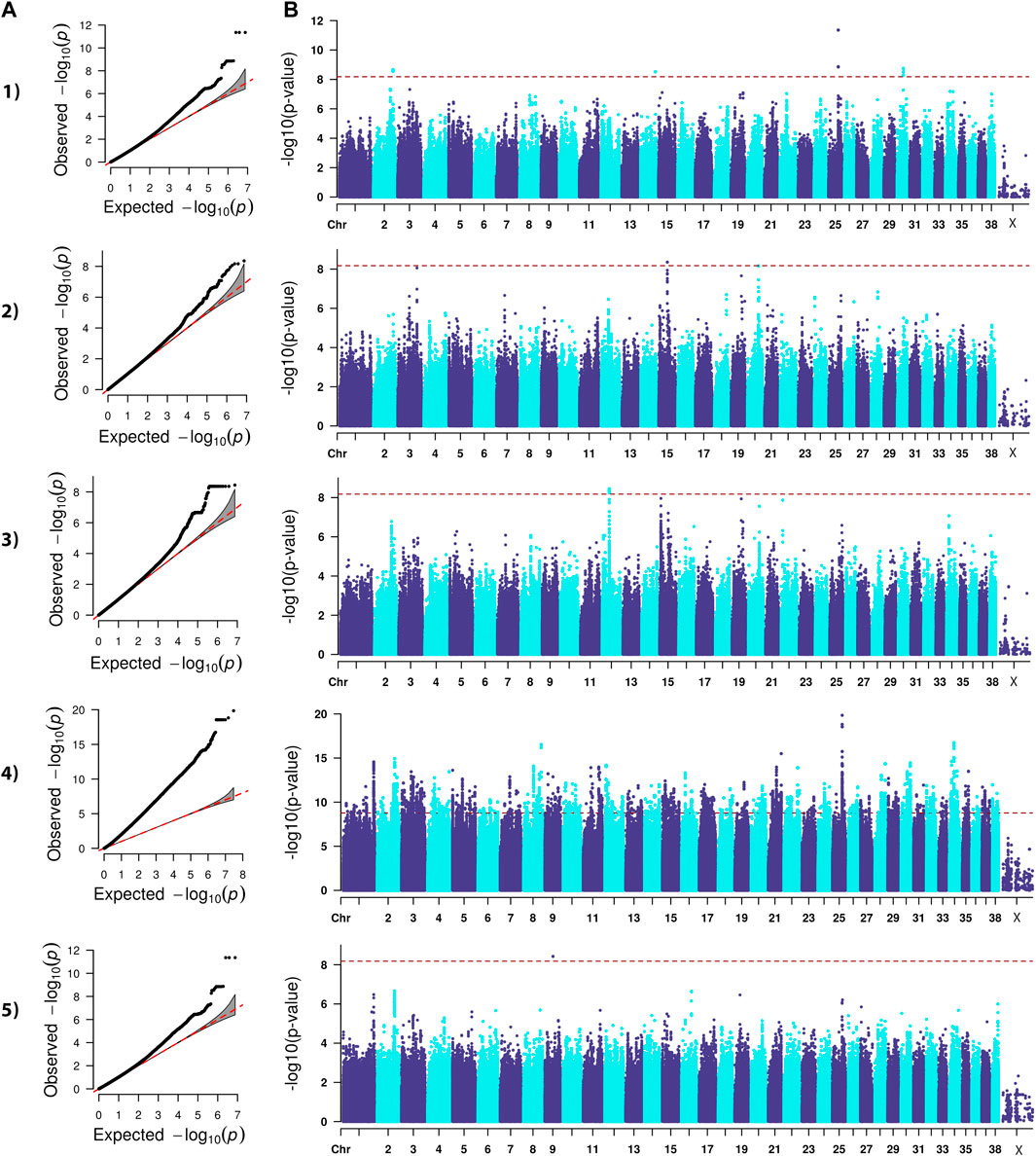
FIGURE 1. QQ (A) and Manhattan (B) plots. Comparison of four models for performing across-breed genome-wide association study using breed phenotypes for OFA hip dysplasia. 1) Linear mixed model, λ = 0.95, 16 significant single-nucleotide polymorphisms (SNPs) on chromosome 2, 14, 25, and 30 (p < 6.59E-9). 2) Stringent binary case-control model, λ = 1.034, 1 significant SNP on chromosome 15 (p < 6.68E-9). 3) Lenient binary case-control model, λ = 1.02, 21 significant SNPs on chromosome 12 (p < 6.64E-9). 4) Haplotype-based model, λ = 2.31, 5,687 significant SNPs with the strongest associations occurring on chromosomes 8, 21, 25, and 34 (p < 1.68E-9). 5) Weighted least squares model, λ = 1.54, 1 significant SNP on chromosome 9 met Bonferroni significance cutoff (p < 6.59E-9).
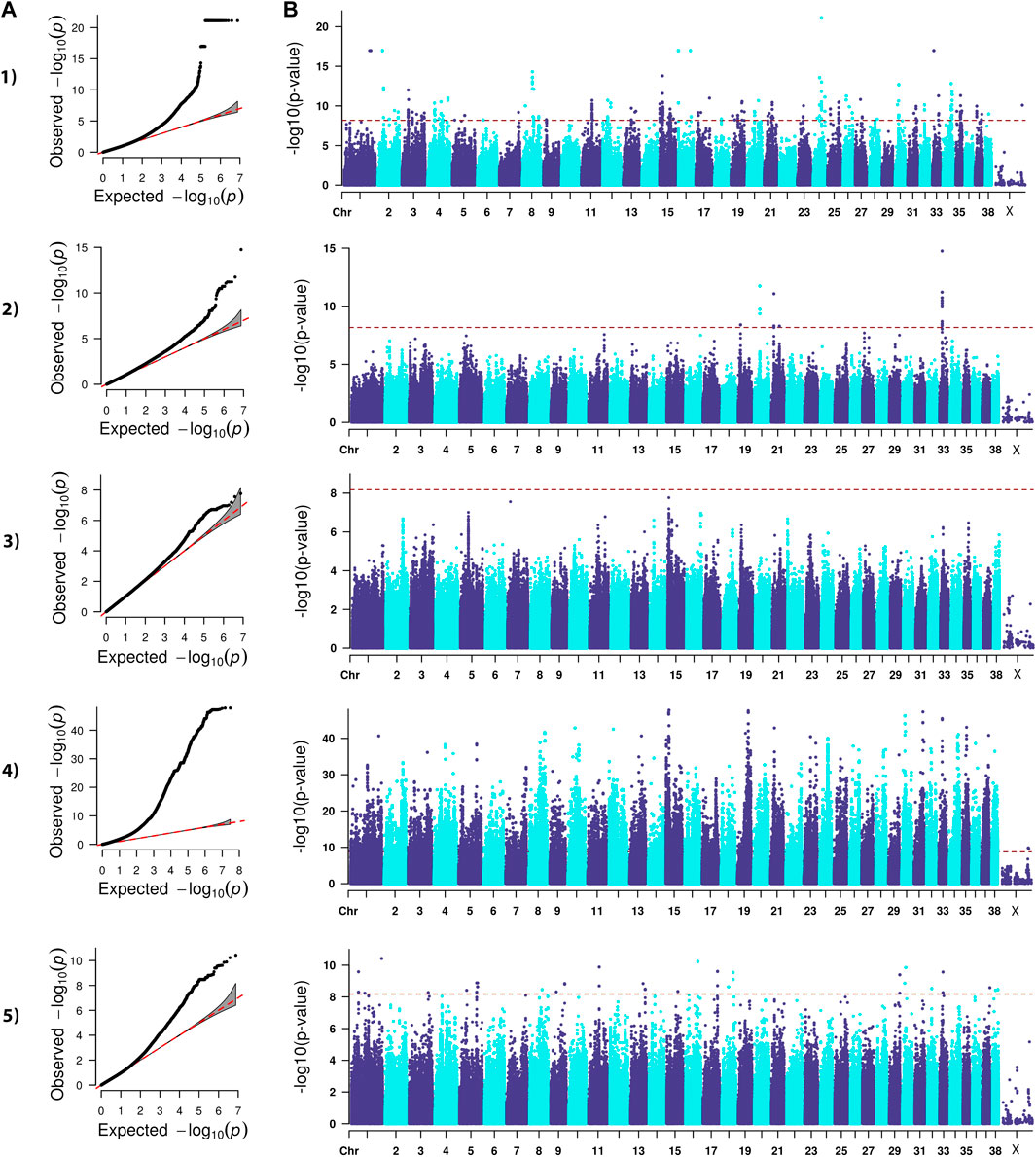
FIGURE 2. QQ (A) and Manhattan (B) plots. Comparison of four models for performing across-breed genome-wide association study using breed phenotypes for elbow dysplasia. 1) Linear mixed model, λ = 0.99, 517 significant single-nucleotide polymorphisms (SNPs) with the strongest associations on chromosome 1, 2, 8, 15, 16, 24, 30, and 33 (p < 6.59E-9). 2) Stringent binary case-control model, λ = 1.04, 30 significant SNPs on chromosome 19, 20, 21, 33 (p < 6.68E-9). 3) Lenient binary case-control model, λ = 1.01, no significant SNPs (p < 6.64E-9). 4) Haplotype-based model, λ = 1.84, 58,592 significant SNPs with the strongest associations on chromosomes 1, 8, 10, 12, 13, 15, 19, 21, 23, 24, 30, 31, 33, 34, 35, and 37 (p < 1.68E-9). 5) Weighted least squares model, λ = 0.96, 90 significant SNPs on chromosomes 1, 3, 5, 8, 9, 11, 13, 15, 16, 17, 18, 29, 30, 32, 33, 37, and 38 (p < 6.59E-9).
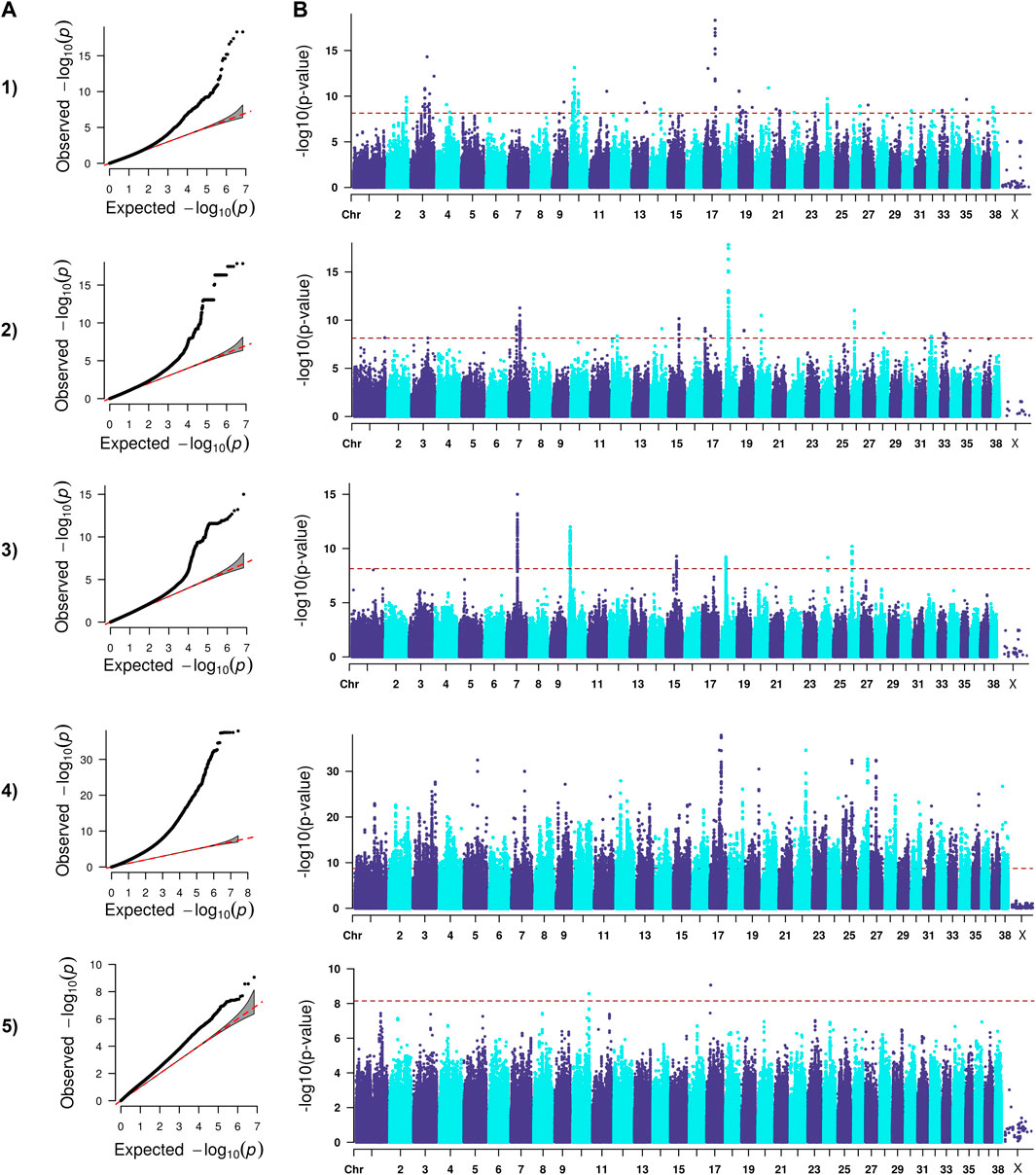
FIGURE 3. QQ (A) and Manhattan (B) plots. Comparison of four models for performing across-breed genome-wide association study using breed phenotypes for hospital hip dysplasia. 1) Linear mixed model, λ = 0.96, 195 significant single-nucleotide polymorphisms (SNPs) with the strongest associations on chromosome 3, 10, 11, 17, 19, and 20 (p < 7.12E-9). 2) Stringent binary case-control model, λ = 1.04, 364 significant SNPs with the strongest associations on chromosomes 7, 15, 18, 20, and 26 (p < 7.26.E-9). 3) Lenient binary case-control model, λ = 1.00, 376 significant SNPs on chromosomes 7, 10, 15, 18, 24, and 26 (p < 7.13E-9). 4) Haplotype-based model, λ = 1.97, 18,895 significant SNPs with the strongest associations on chromosomes 5, 7, 12, 17, 19, 22, 25, 26, and 27 (p < 1.82E-9). 5) Weighted least squares model, λ = 1.54, 3 significant SNPs on chromosomes 10 and 17 met Bonferroni significance cutoff (p < 7.11E-9).
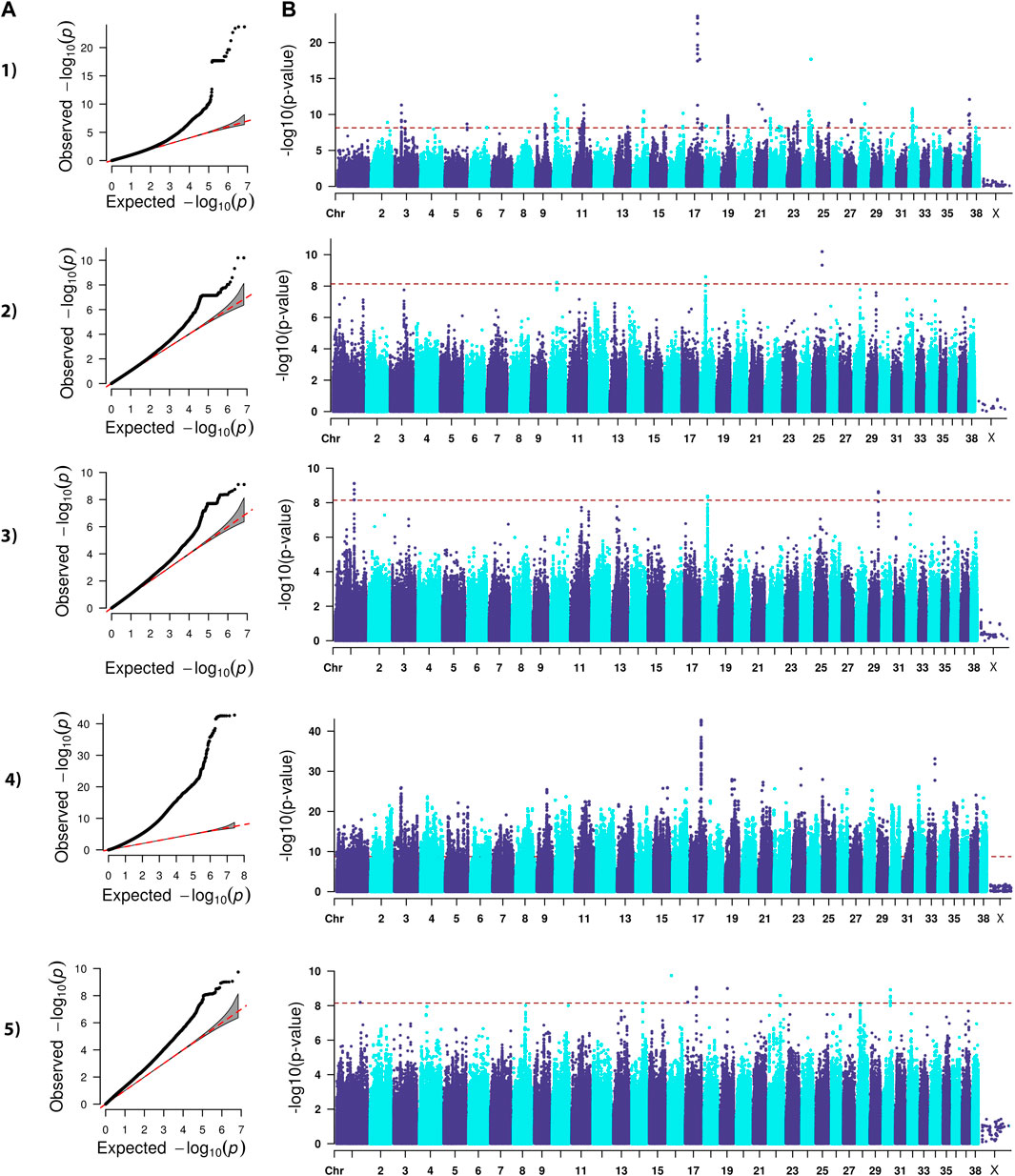
FIGURE 4. QQ (A) and Manhattan (B) plots. Comparison of four models for performing across-breed genome-wide association study using breed phenotypes anterior cruciate ligament rupture. 1) Linear mixed model, λ = 0.96, 219 significant single-nucleotide polymorphisms (SNPs) with the strongest associations on chromosomes 3, 10, 11, 14, 16, 17, 19, 21, 24, 28, 32, and 37 (p < 7.12E-9). 2) Stringent binary case-control model, λ = 1.05, 5 significant SNPs on chromosomes 10, 18, and 25 (p < 7.26E-9). 3) Lenient binary case-control model, λ = 1.03, 20 significant SNPs on chromosomes 1, 18, and 29 (p < 7.13E-9). 4) Haplotype-based model, λ = 2.30, 38,677 significant SNPs with the strongest associations on chromosomes 3, 9, 15, 16, 17, 19, 21, 22, 23, 25, 32, and 33 (p < 1.82E-9). 5) Weighted least squares model, λ = 1.58, 20 significant SNPs on chromosomes 1, 14, 16, 17, 19, 22, and 30 (p < 7.11E-9).
Lambda ranged from 0.95 for OFA HD to 0.99 for ED (Figures 1–4). The 16 significant OFA HD SNPs were located on chromosomes 2, 14, 25, and 30 (Table 3). The strongest associations for ED were located on chromosomes 1, 2, 8, 15, 16, 24, 30, and 33 (Table 4). Hospital HD SNPs had strong associations with chromosomes 3, 10, 11, 17, 19, and 20 (Table 5), and ACL rupture SNPs had strong associations on chromosomes 3, 10, 11, 14, 16, 17, 19, 21, 24, 28, 32, and 37 (Table 6). IL26 and IL22 were in a chromosome 10 region located at 10,418,984–10,524,282 bp. CSMD1 was in a region on chromosome 16 and TNS1 was in a region on chromosome 37. The strongest association for hospital HD located on chromosome 17 at 44,162,612–44,172,578 bp overlapped with the strongest association for ACL rupture. Similarly, two significant regions on chromosome 24 at 25,363,325–25,384,175 bp and 32,828,822–32,908,498 bp were significant for both ED and ACL rupture and contained TGIF2 and LDHA respectively. The chromosome 15 region located at 10,683,929–10,712,309 bp that associated with ED contained FAF1, and a significant hospital HD association on chromosome 3 located at 91,241,141 bp contained LCORL.
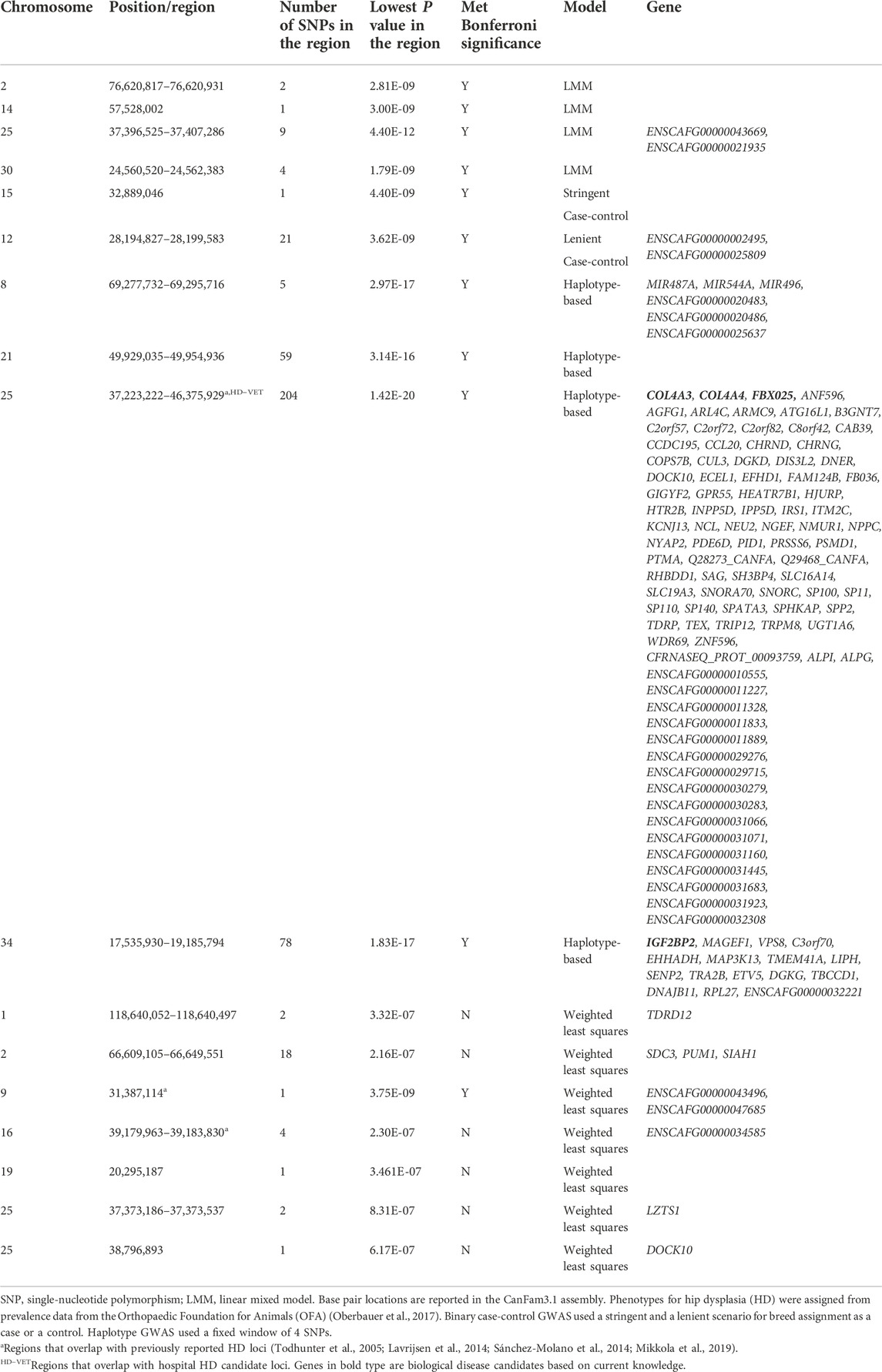
TABLE 3. Top candidate loci associated with OFA hip dysplasia in across-breed GWAS using breed phenotypes and four different association models.
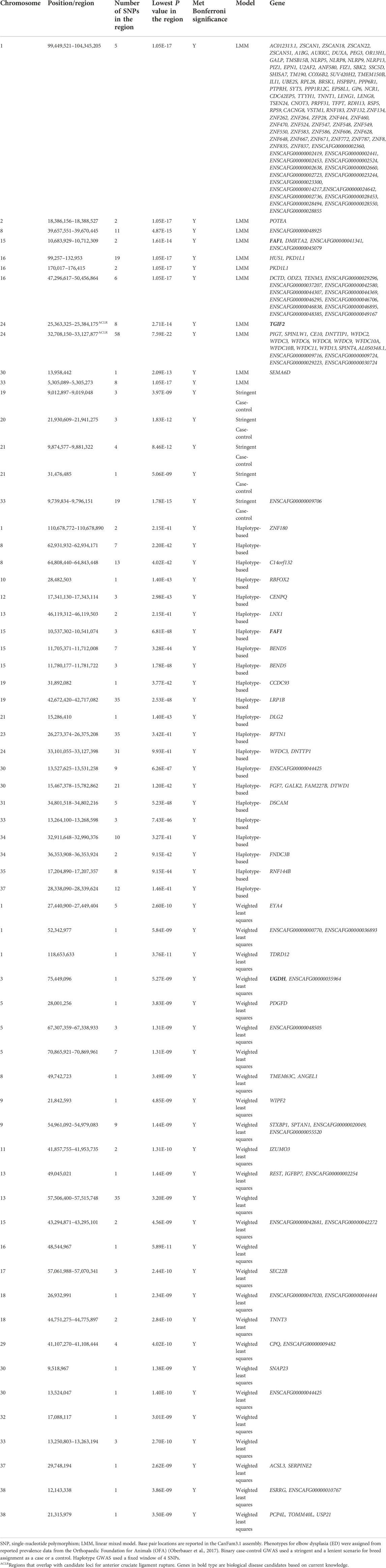
TABLE 4. Top candidate loci associated with elbow dysplasia in across-breed GWAS using breed phenotypes and four different association models.
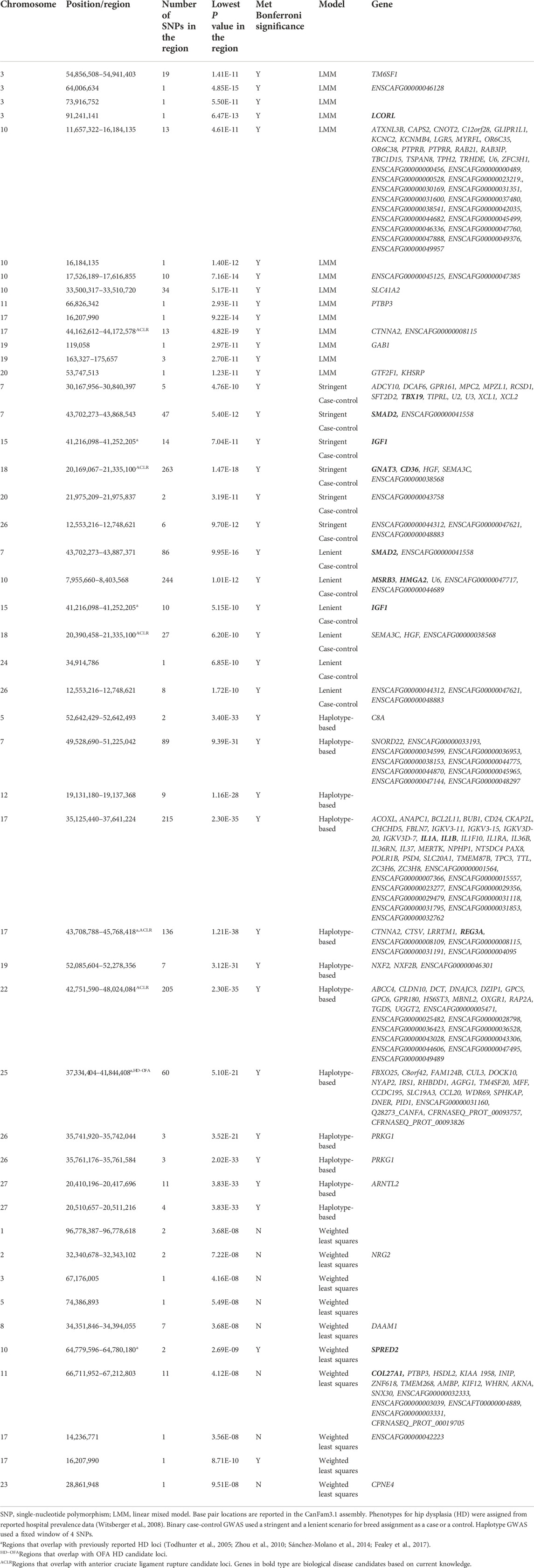
TABLE 5. Top candidate loci associated with hospital hip dysplasia in across-breed GWAS using breed phenotypes and four different association models.
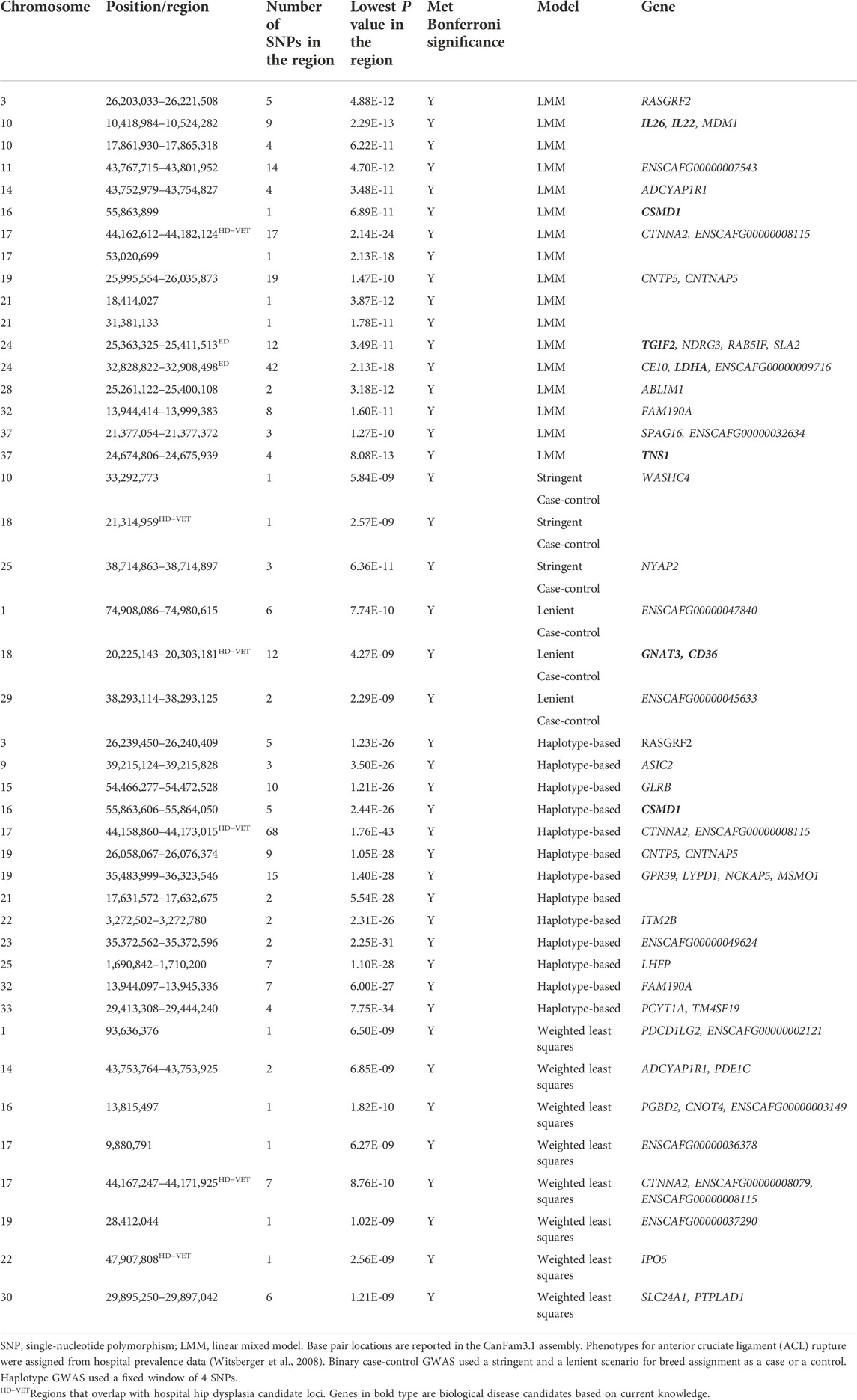
TABLE 6. Top candidate loci associated with anterior cruciate ligament rupture in across-breed GWAS using breed phenotypes and four different association models.
Binary case-control genome-wide association study model
A stringent and a less stringent scenario were used for breed assignment (Supplementary Table S2–S6; Supplementary Data S4). The stringent case-control scenario for OFA HD had 1 SNP on chromosome 15 that met genome-wide significance. With the less stringent scenario, 21 SNPs on chromosome 12 met genome-wide significance (Figure 1) (Table 3). With the stringent scenario, 30 significant ED SNPs were found on chromosomes 19, 20, 21, and 33. No significant SNPs were identified with the less stringent scenario (Figure 2) (Table 4). For hospital HD, 364 significant SNPs were found with the stringent scenario with the strongest associations located on chromosomes 7, 15, 18, 20, and 26 (Figure 3), and 376 significant SNPs on chromosomes 7, 10, 15, 18, 24, and 26 were found with the less stringent scenario. Genes within candidate regions for hospital HD included IGF1, TBX19, GNAT3, CD36, MSRB3, HMGA2, and SMAD2 (Table 5). For ACL rupture, 5 significant SNPs were found with the stringent scenario on chromosomes 10, 18, and 25, while the lenient scenario identified 20 SNPs on chromosomes 1, 18, and 29 (Figure 4). Two ACL rupture candidate loci on chromosome 18 located at 20,225,143–20,303,181bp and 21,314,959bp overlapped with candidate loci for hospital HD. The SNP effect estimates are reported in Supplementary Data S5.
Haplotype-based genome-wide association study model
Scree plots (Supplementary Figure S7) were used to determine the optimum number of eigenvectors for the correction of population structure. For the OFA HD and ED SNPs, the first six eigenvectors were used as covariates, and the first eight eigenvectors were used for the hospital HD and ACL rupture SNPs. When the haplotype-based analysis was performed with a fixed 4 SNP window, 7,586,822 and 7,026,651 sliding windows were analyzed for the OFA and hospital datasets, respectively. Bonferroni genome-wide significance was set at (cutoff = -log10 (0.05/number of haplotypes) = 8.77 (OFA dataset) and 8.74 (veterinary hospital dataset). Lambda values ranged from 1.84 (ED) to 2.31 (OFA HD) (Figures 1–4). For OFA HD there were 5,687 significant SNPs, with the strongest associations located on chromosomes 8, 21, 25, and 34 (Figure 1). The locus on chromosome 34 contained IGF2BP2, a gene known to influence body size. For ED, there were 58,592 significant SNPs, with the strongest associations located on chromosomes 1, 8, 10, 12, 13, 15, 19, 21, 23, 24, 30, 31, 33, 34, 35, and 37 (Figure 2) (Table 4). The chromosome 15 loci 10,537,302–10,541,074bp contained the gene FAF1. For hospital HD there were 18,895 significant SNPs with the strongest associations on chromosomes 5, 7, 12, 17, 19, 22, 25, 26, and 27 (Figure 3). The chromosome 17 locus, which contained REG3A, overlapped with an ACL rupture locus from the LMM, haplotype, and weighted least square models. The chromosome 25 locus also overlapped with an OFA HD locus determined by the haplotype model. The chromosome 17 locus 35,125,440–37,641,224 bp contained the IL1A and IL1B genes. For ACL rupture, there were 38,677 significant SNPs, with the strongest associations located on chromosomes 3, 9, 15, 16, 17, 19, 21, 22, 23, 25, 32, and 33. The chromosome 17 locus at 44,158,860–44,173,015bp overlapped with the hospital HD candidate loci. Separate haplotype-based analysis with FDR correction still had evident genomic inflation (Supplementary Figure S8). After FDR correction, 36 and 23,598 SNPs met the same Bonferroni significance thresholds for OFA HD and ED analysis. The hospital HD and ACL rupture analysis had 4,294 and 10,403 SNPs meet Bonferroni significance thresholds after FDR correction. The SNP effect estimates are reported in Supplementary Data S5.
Weighted least squares genome-wide association study model
The final model performed for comparison was a novel weighted least squares approach. This association analysis calculated the average allelic frequency for each SNP within a breed and then weighted it according to the number of individuals (repeats) within a breed in the dataset. This model was developed to address the challenges of unbalanced breed representation in the repository data rather than excluding individuals to balance the number of dogs per breed. Genome-wide significance was set at Bonferroni correction of p < 6.59E-9 for the OFA HD and ED datasets and p < 7.11E-9 for the hospital HD and ACL rupture datasets.
Lambda values ranged from 0.96 (OFA ED) to 1.58 (ACL rupture) (Figures 1–4). 1 SNP on chromosome 9 met genome-wide significance when the OFA HD dataset was analyzed (Figure 1). This chromosome 9 SNP is an established HD locus (Table 3) (Mikkola et al., 2019). One locus on chromosome 16 that was approaching significance overlapped with an established HD locus (Table 3) (Todhunter et al., 2005). Another region approaching genome-wide significance on chromosome 25, at 37,373,186–37,373,537bp, was reported as significant with the LMM and haplotype-based GWAS. The weighted least squares analysis of the ED data resulted in 90 SNPs located on chromosomes 1, 3, 5, 8, 9, 11, 13, 15, 16, 17, 18, 29, 30, 32, 33, 37, and 38 that met genome-wide significance (Figure 2) (Table 4). The chromosome 3 locus contained the gene UGDH. The chromosome 16 locus was also reported as significant with the LMM GWAS. 2 SNPs on chromosome 10 and 1 SNP on chromosome 17 met genome-wide significance for the hospital HD data (Figure 3). The region on chromosome 10 (Table 5) is a known HD associated locus and contained SPRED2 (Todhunter et al., 2005). Of the SNPs that were approaching genome-wide significance, a chromosome 11 region contained COL27A1 and was reported as significant with the LMM GWAS. 20 SNPs located on chromosomes 1, 14, 16, 17, 19, 22, and 30, met genome-wide significance for the weighted least squares analysis of the ACL rupture dataset (Table 6). The significant locus on chromosome 14 was also reported as significant with the LMM GWAS. The chromosome 17 locus at 44,167,247–44,171,925bp was also reported as significant with the LMM and haplotype GWAS, and this region also overlapped with a hospital HD candidate locus. The SNP effect estimates are reported in Supplementary Data S5.
Discussion
To investigate large-effect disease-associated variants for three major canine orthopedic diseases, we performed association analysis with LMM, binary case-control, haplotype-based, and weighted least squares methods with breed phenotypes. We selected the diseases to study, in part, because there is existing genetic discovery literature, particularly for HD. This body of work allowed us to compare previously reported candidate variants with the loci and genes identified in the present study.
In total, we identified six HD candidate loci on chromosomes 9, 10, 15, 16, 17, and 25 that have been previously reported in the literature. A chromosome 25 region containing the COL4A3 and COL4A4 genes was associated with Norberg Angle (NA), a measurement of hip joint laxity, in a population of British Labrador Retrievers (Sánchez-Molano et al., 2014), and had some overlap with another chromosome 25 region with suggestive association of HD in Dutch Labrador Retrievers (Lavrijsen et al., 2014). Portions of these regions were identified with OFA HD LMM analysis, both OFA and hospital HD haplotype analyses, and approached significance with the OFA HD weighted least squares analysis. The F-box protein 25 (FBX025) gene that controls ubiquitin-mediated proteolysis is within the chromosome 25 HD region of interest (Lykkjen et al., 2010). F-box proteins are important in cell signaling, transcription, and cell cycle signaling. FBX025 can influence osteoblast differentiation (He et al., 2019). GWAS of nontraumatic osteonecrosis of the femoral head in humans reported an association with a FBX025 SNP (Zhang et al., 2020). Subtle changes to hip endochondral ossification, femoral head shape, or subchondral bone and articular cartilage healing could all be mechanisms by which FBX025 influences HD risk (Schachner and Lopez 2015).
A chromosome 9 locus identified by the weighted least squares OFA HD GWAS overlapped with a region previously associated with hip joint incongruity, femoral head positioning, and NA in German Shepherds (Mikkola et al., 2019). A locus on chromosome 16 with no annotated genes approached significance in the weighted least squares OFA HD GWAS and has been associated with NA in the offsprings of Greyhounds crossed with HD Labrador Retrievers (Todhunter et al., 2005). Similarly, a chromosome 10 region containing SPRED2 was detected in the weighted least squares hospital HD GWAS and overlapped an HD quantitative trait locus in the same Greyhound–Labrador Retriever crosses (Todhunter et al., 2005). SPRED2 functions in chondrocyte and bone development, with promoter activity noted in the acetabulum and femoral head (Bundschu et al., 2005).
With the hospital HD SNPs, haplotype GWAS found a chromosome 17 locus containing REG3A (regenerating islet derived gamma 3). This candidate locus is strongly associated with hip osteoarthritis (OA), suggesting REG3A may influence canine hip OA through MAPK pathway signaling (Zhou et al., 2010). REG3A plays a role in adhesion, cell recognition, and protection from oxidative stress-induced-apoptosis; REG3A may affect OA progression by regulating chondrocyte response to injury (Ortiz et al., 1998; Zhou et al., 2010).
The locus on chromosome 15 that was previously reported in the literature was found through both stringent and lenient case-control binary analysis of hospital HD data (Fealey et al., 2017). Interestingly, both stringent and lenient case-control GWAS identified a candidate locus on chromosome 15 containing IGF1, the growth hormone that influences body size and lifespan in dogs (Plassais et al., 2019). A previous across-breed GWAS identified IGF1 as a candidate gene influencing pelvis size and risk of HD, as dogs with larger pelves tend to have smaller NA and increased HD risk (Fealey et al., 2017). IGF1 was not the only domestication gene found within HD candidate loci. IGF1, LCORL, SMAD2, HMGA2, TBX19, IGF2BP2, MSRB3, GNAT3, and CD36 are all genes that have been under selection during domestication (Cadieu et al., 2009; Zapata et al., 2016; Plassais et al., 2019). LCORL, SMAD2, HMGA2, TBX19, and IGF2BP2 are well-documented regulators of dog size and are associated with domestication (Sutter et al., 2007; Sutter et al., 2008; Boyko et al., 2010; Hoopes et al., 2012; Hayward et al., 2016; Plassais et al., 2017; Plassais et al., 2019). These results suggest that the risk of HD is influenced by morphological trait selection during domestication. However, additional analysis should be pursued to correct for breed size to confirm the association with HD that is not confounded by breed differences in body size.
Other evidence that HD has been influenced by domestication is that MSRB3, GNAT3, and CD36 are also genes responsible for diverse phenotypic and behavioral traits associated with domestication. MSRB3 is associated with a dropped ear morphology (Vaysse et al., 2011; Webster et al., 2015). GNAT3 and CD36 have been associated with behavioral traits undergoing selection, including touch sensitivity, fear, and aggression (Zapata et al., 2016). Although most of these associations with domestication were detected using case-control binary GWAS, LCORL, and, IGF2BP2 were highlighted by LMM and haplotype GWAS, respectively.
Haplotype GWAS identified a novel association between hospital HD and a candidate locus on chromosome 17 that contains IL1A (interleukin 1α) and IL1B (interleukin 1β). IL1A and IL1B polymorphisms have been associated with human hip OA patients (Cai et al., 2015). One proposed mechanism is that IL1 transcription and synthesis promotes cartilage breakdown, the release of arachidonic acid, and prostanoid and eicosanoid production (Solovieva et al., 2009). The hospital HD weighted least squares analysis revealed a novel chromosome 11 region containing COL27A1 that approached significance. Mutations in COL27A1 are associated with human orthopedic disease, Steel syndrome, which includes bilateral congenital HD (Gonzaga-Jauregui et al., 2015).
LMM and haplotype GWAS identified an interesting association between ED and a locus on chromosome 15 containing FAF1 (fas-associated factor 1), an apoptosis enhancer that modulates osteoblast differentiation (Chu et al., 1995; Zhang et al., 2011). FAF1 has been associated with osteochondrosis of the proximal radius and ulna and the distal humerus in pigs (Rangkasenee et al., 2013). In addition, the weighted least squares ED analysis detected a chromosome 3 region containing UGDH. UGDH is involved in the biosynthesis of glycosaminoglycans. A structural variant affecting UGDH has been associated with disproportional dwarfism in cats (Buckley et al., 2020; Struck et al., 2020). Interestingly, affected dwarf cats have rotational abnormalities present in their thoracic limbs resulting in moderate elbow incongruity (Struck et al., 2020).
We identified candidate loci for both HD and ACL rupture on chromosomes 17, 18, and 22. The chromosome 17 locus, containing CTNNA2, was the most significant haplotype GWAS association for both HD and ACL rupture. GNAT3 and CD36 are genes associated with behavioral domestication traits residing in the chromosome 18 locus (Zapata et al., 2016). Further investigation is warranted to explore shared variants across breeds and diseases. Two chromosome 24 loci were associated with both ED and ACL rupture and included TGIF2, a gene that influences transcription and cellular proliferation. Suppressed TGIF2 expression results in decreased cellular proliferation, increased synovial cell apoptosis, and associated OA (Luo et al., 2019). Interestingly, we did not identify shared loci for HD and ED.
Several interesting OA genes were identified from the ACL rupture LMM GWAS. The chromosome 10 locus contained two interleukin genes, IL26 and IL22. IL22 has been positively correlated with synovitis in knee OA, suggesting a functional role (Deligne et al., 2015). A chromosome 16 locus containing CSMD1 was detected by LMM and haplotype GWAS. A CSMD1 SNP influences height and risk of OA in humans (Elliott et al., 2013). In another study, a CSMD1 SNP was associated with CTX-II levels, a urinary biomarker of cartilage degradation (Ramos et al., 2014). Another ACL rupture locus on chromosome 24 contains LDHA (lactate dehydrogenase A), which catalyzes the final step of anaerobic glycolysis. In chondrocytes under inflammatory stress, LDHA binds NAHD and increases oxidation and free radical generation, thereby augmenting the inflammatory response and OA severity (Arra et al., 2020). Lastly, the chromosome 37 locus contained TNS1 (tensin 1), which is essential for extracellular fibronectin, collagen matrix formation, and myofibroblast differentiation (Bernau et al., 2017). TNS1 expression is decreased in ruptured ACL in patients with a meniscal tear compared to patients without meniscal injury (Brophy et al., 2018).
Classic LMMs perform a series of single SNP association tests and examine phenotype associations independently. LMMs are generally used to analyze balanced datasets with quantitative traits for phenotypes. Quantitative traits are traits that vary among individuals over a range of continuous distributions of phenotypes, such as height or weight. Haplotype-based models perform a similar analysis of quantitative traits, but rather than perform association analysis with individual SNPs, the association analysis is performed on a neighboring group of SNPs on a chromosome. Haplotype-based methods can increase statistical power to detect lower frequency variants by constructing groups of SNPs based on the principle that SNPs in close proximity are more likely to be inherited together. Binary case-control models are used to analyze dichotomous phenotypes. Classic LMM, haplotype-based, and binary case-control models can perform poorly when datasets are unbalanced, which can inflate type 1 error rates for rare phenotypic variants. In addition to performing poorly with unbalanced datasets, these methods are optimized for quantitative (LMM and haplotype-based) and dichotomous phenotypes (binary case-control). Breed phenotypes are ordinal categorical phenotypes. Using categorical phenotypes with models optimized to handle quantitative or dichotomous traits may result in lost information and decreased power (Lane et al., 2019).
Biologically relevant associations were identified by all GWAS methods with no evidence that one method is advantageous. No methodology performed consistently better across all datasets. LMM, haplotype-based, case-control binary, and weighted least squares models all reported biologically relevant associations. No two methods had a constant overlap of significant findings over all diseases analyzed. Currently, there is little literature documenting candidate loci for ED and ACL rupture, limiting validation findings and hindering complete performance assessment of the models. Many significant associations do not contain annotated genes. This could be due to imperfect annotation of the reference genome. However, such regions could contain quantitative trait loci that explain variation in gene expression and associated disease risk.
Several study limitations were identified. Genomic inflation with high lambda values was a consistent feature of haplotype GWAS. This could be due to the use of a fixed SNP window exacerbating allele frequency differences with the large, fixed haplotypes that exist across breeds of dogs (Lindblad-Toh et al., 2005). Although elevated lambda values can reflect disease polygenicity, in an across-breed GWAS using breed phenotypes, inflated values are more likely a consequence of population structure. Despite the application of FDR correction to the haplotype GWAS, genomic inflation was still evident. The fixed SNP window haplotype GWAS likely had the highest rate of reporting type 1 errors under the parameters tested in this study. Refinement of the haplotype GWAS approach appears warranted. Rather than fixed haplotypes, the use of dynamically defined haplotypes (Hamazaki and Iwata, 2020) could help address this problem.
Overall, the weighted least squares method was able to identify one SNP that was previously reported or detected by another model for all diseases. The weighted least squares method most often identified a moderate number of SNPs, but the Bonferroni correction appeared too conservative as even loci that had been previously reported did not meet genome-wide significance, such as the chromosome 16 locus identified from OFA HD SNPs. The advantage of the weighted least squares method is that it was developed to handle unbalanced datasets to allow the analysis of all sample data. A disadvantage to the weighted least squares model is if most of the samples of a particular breed did not have the large effect variant at a particular SNP, then that variant may go undetected during analysis because average allelic frequencies are calculated per SNP per breed. There is growing scientific interest in ordinal categorial GWAS approaches optimized for repository SNP data (Bi et al., 2021), and alternative future approaches may yield improved performance. In a recent study that performed LMM analysis with breed phenotypes, breed groups were fixed at n = 4 (Plassais et al., 2019). More work is needed to study the effect of variation of breed class number on the association model results.
Defining genome-wide significance dynamically for each model would also be helpful. Bonferroni correction may be too conservative for the weighted least squares method, as supported by the chromosome 16 locus identified from OFA HD SNPs that did not meet genome-wide significance but overlapped with a previous HD candidate locus. Conversely, a more stringent cutoff may be needed for haplotype GWAS to reduce the risk of false positives. FDR correction was not sufficient by itself to dampen the extreme genomic inflation of the haplotype-based analysis. The FDR correction of the LMM did limit the number of significant loci to fewer genomic regions and even had no reported significant loci for the OFA HD dataset.
There were also limitations to the binary case-control GWAS regarding stringency parameters for breed classification as excessively stringent classification reduced sample size. Reduced sample size may increase the likelihood of a false-positive association that reflects a contrast of other breed phenotypes different from the complex disease of interest. This problem should self-correct as the number of genomes in the Dog10K project expands. Overall, the dichotomous case-control GWAS had the smallest number of significant SNPs detected for each disease revealing that information is lost when an ordinal categorical phenotype is arbitrarily made into a dichotomous binary case-control phenotype. Case-control GWAS may allow important large-effect disease-associated genetic variants of breeds considered moderate risk, not classified as high or low risk, to go undetected.
Although SNP and dog filtering was robustly considered, including confirming the phylogeny of individual samples using a cladogram, while aiming to preserve as many dogs as possible in each breed group, further data filtering could have been considered, such as excluding individuals with very low sequencing depth. However, despite differences between HD breed prevalence (Witsberger et al., 2008; Oberbauer et al., 2017) and differences in the number of dogs in each breed in our dataset, we were able to identify both novel and previously reported large effect variants. The hospital HD and ACL rupture datasets consistently had more genomic inflation than the OFA datasets. Excess genomic inflation in the hospital dataset may be attributable to specific breeds containing large numbers of dogs, which was not the case in the OFA dataset.
In conclusion, we investigated four approaches for across-breed GWAS with breed phenotypes for three major canine orthopedic diseases. The use of multiple GWAS methods helped us to detect significant associations. For HD, strong associations with genes that regulate morphology were found, suggesting that the intense selection that gave rise to diverse breeds of dog has also influenced breed risk for HD. We also discovered a strong association between ED and a biologically relevant gene, FAF1, that warrants validation. Further investigation is needed to explore alternative methods for haplotype GWAS. Here, use of a moving window to dynamically define haplotype may be beneficial. Further optimization of the methods for the estimation of genome-wide significance appropriate for each model is also needed to appropriately control the false discovery rate. Categorical GWAS of ancestral populations may contribute to the understanding of any disease for which epidemiological risk data are available for the breed or ancestral population, including diseases for which GWAS has not been performed and candidate loci remain elusive.
Data availability statement
The genome sequence accessions and metadata are available from the Dog Biomedical Variant Database Consortium (Jagannathan et al., 2019). The variants are available at the European Variant Archive under project ID PRJEB32865 at https://www.ebi.ac.uk/eva/?eva-study=PRJEB32865. Our SNP set was a newer version of the variants in this repository. The current DBVDC dataset is available by request from the consortium.
Ethics statement
Ethical review and approval was not required for the animal study because the study uses repository data from the Dog 10K Genome Project.
Author contributions
EB carried out the study and wrote the first draft of the manuscript. MM contributed to data analysis and preparation of figures and contributed to writing and editing the manuscript. GR contributed to method development for data analysis. BD helped with dataset preparation, study design, and bioinformatics approach and contributed to manuscript editing. PM designed the experiment, obtained funding for the experiment, supervised the study, and revised the manuscript. All authors have read and approved the final version of the manuscript.
Funding
EB was supported by a T32 training grant (T32OD010423) from the National Institutes of Health. The funder had no role in the study design, data collection and analysis, decision to publish, or preparation of the manuscript.
Acknowledgments
The authors gratefully acknowledge the support of the Dog10K Genomes Project (www.dog10kgenomes.org) for this study.
Conflict of interest
The authors declare that the research was conducted in the absence of any commercial or financial relationships that could be construed as a potential conflict of interest.
Publisher’s note
All claims expressed in this article are solely those of the authors and do not necessarily represent those of their affiliated organizations, or those of the publisher, the editors, and the reviewers. Any product that may be evaluated in this article, or claim that may be made by its manufacturer, is not guaranteed or endorsed by the publisher.
Supplementary material
The Supplementary Material for this article can be found online at: https://www.frontiersin.org/articles/10.3389/fgene.2022.913354/full#supplementary-material
References
Arra, M., Swarnkar, G., Ke, K., Otero, J. E., Ying, J., Duan, X., et al. (2020). LDHA-mediated ROS generation in chondrocytes is a potential therapeutic target for osteoarthritis. Nat. Commun. 11, 3427. doi:10.1038/s41467-020-17242-0
Baird, A. E., Carter, S. D., Innes, J. F., Ollier, W., and Short, A. (2014). Genome-wide association study identifies genomic regions of association for cruciate ligament rupture in Newfoundland dogs. Anim. Genet. 45, 542–549. doi:10.1111/age.12162
Baker, L. A., Kirkpatrick, B., Rosa, G. J., Gianola, D., Valente, B., Sumner, J. P., et al. (2017). Genome-wide association analysis in dogs implicates 99 loci as risk variants for anterior cruciate ligament rupture. PLoS One 12, e0173810. doi:10.1371/journal.pone.0173810
Baker, L. A., Momen, M., McNally, R., Berres, M. E., Binversie, E. E., Sample, S. J., et al. (2021). Biologically enhanced genome-wide association study provides further evidence for candidate loci and discovers novel loci that influence risk of anterior cruciate ligament rupture in a dog model. Front. Genet. 12, 593515. doi:10.3389/fgene.2021.593515
Baker, L. A., Rosa, G. J., Hao, Z., Piazza, A., Hoffman, C., Binversie, E. E., et al. (2018). Multivariate genome-wide association analysis identifies novel and relevant variants associated with anterior cruciate ligament rupture risk in the dog model. BMC Genet. 19, 39. doi:10.1186/s12863-018-0626-7
Bannasch, D. L., Baes, C. F., and Leeb, T. (2020). Genetic variants affecting skeletal morphology in domestic dogs. Trends Genet. 36, 598–609. doi:10.1016/j.tig.2020.05.005
Bannasch, D., Young, A., Myers, J., Truvé, K., Dickinson, P., Gregg, J., et al. (2010). Localization of canine brachycephaly using an across breed mapping approach. PLoS One 5, e9632. doi:10.1371/journal.pone.0009632
Bernau, K., Torr, E. E., Evans, M. D., Aoki, J. K., Ngam, C. R., and Sandbo, N. (2017). Tensin 1 is essential for myofibroblast differentiation and extracellular matrix formation. Am. J. Respir. Cell. Mol. Biol. 56, 465–476. doi:10.1165/rcmb.2016-0104OC
Beuing, R., Mues, C. H., Tellhelm, B., and Erhardt, G. (2000). Prevalence and inheritance of canine elbow dysplasia in German Rottweiler. J. Anim. Breed. Genet. 117, 375–383. doi:10.1046/j.1439-0388.2000.00267.x
Bi, W., Zhou, W., Dey, R., Mukherjee, B., Sampson, J. N., and Lee, S. (2021). Efficient mixed model approach for large-scale genome-wide association studies of ordinal categorical phenotypes. Am. J. Hum. Genet. 108, 825–839. doi:10.1016/j.ajhg.2021.03.019
Bleedorn, J. A., Greuel, E. N., Manley, P. A., Schaefer, S. L., Markel, M. D., Holzman, G., et al. (2011). Synovitis in dogs with stable stifle joints and incipient cranial cruciate ligament rupture: A cross-sectional study. Vet. Surg. 40, 531–543. doi:10.1111/j.1532-950X.2011.00841.x
Bolormaa, S., Pryce, J. E., Reverter, A., Zhang, Y., Barendse, W., Kemper, K., et al. (2014). A multi-trait, meta-analysis for detecting pleiotropic polymorphisms for stature, fatness and reproduction in beef cattle. PLoS Genet. 10, e1004198. doi:10.1371/journal.pgen.1004198
Boyko, A. R., Quignon, P., Li, L., Schoenebeck, J. J., Degenhardt, J. D., Lohmueller, K. E., et al. (2010). A simple genetic architecture underlies morphological variation in dogs. PLoS Biol. 8, e1000451. doi:10.1371/journal.pbio.1000451
Brenner, S., Miller, J. H., and Broughton, W. (2002). Encyclopedia of genetics. Cambridge, MA: Academic Press.
Brophy, R. H., Rothermich, M. A., Tycksen, E. D., Cai, L., and Rai, M. F. (2018). Presence of meniscus tear alters gene expression profile of anterior cruciate ligament tears. J. Orthop. Res. 36, 2612–2621. doi:10.1002/jor.24025
Buckley, R. M., Davis, B. W., Brashear, W. A., Farias, F. H., Kuroki, K., Graves, T., et al. (2020). A new domestic cat genome assembly based on long sequence reads empowers feline genomic medicine and identifies a novel gene for dwarfism. PLoS Genet. 22, e1008926. doi:10.1371/journal.pgen.1008926
Bundschu, K., Knobeloch, K. P., Ullrich, M., Schinke, T., Amling, M., Engelhardt, C. M., et al. (2005). Gene disruption of Spred-2 causes dwarfism. J. Biol. Chem. 280, 28572–28580. doi:10.1074/jbc.M503640200
Cadieu, E., Neff, M. W., Quignon, P., Walsh, K., Chase, K., Parker, H. G., et al. (2009). Coat variation in the domestic dog is governed by variants in three genes. Science 326, 150–153. doi:10.1126/science.1177808
Cai, H., Sun, H. J., Wang, Y. H., and Zhang, Z. (2015). Relationships of common polymorphisms in IL-6, IL-1A, and IL-1B genes with susceptibility to osteoarthritis: A meta-analysis. Clin. Rheumatol. 34, 1443–1453. doi:10.1007/s10067-014-2708-x
Cangelosi, R., and Goriely, A. (2007). Component retention in principal component analysis with application to cDNA microarray data. Biol. Direct 2, 2–21. doi:10.1186/1745-6150-2-2
Cheverud, J. M. (1988). A comparison of genetic and phenotypic correlations. Evolution 42, 958–968. doi:10.1111/j.1558-5646.1988.tb02514.x
Chu, K., Niu, X. I., and Williams, L. T. (1995). A Fas-associated protein factor, FAF1, potentiates Fas-mediated apoptosis. Proc. Natl. Acad. Sci. U. S. A. 92, 11894–11898. doi:10.1073/pnas.92.25.11894
Chuang, C., Ramaker, M. A., Kaur, S., Csomos, R. A., Kroner, K. T., Bleedorn, J. A., et al. (2014). Radiographic risk factors for contralateral rupture in dogs with unilateral cranial cruciate ligament rupture. PLoS One 9, e106389. doi:10.1371/journal.pone.0106389
Cook, S. R., Conzemius, M. G., McCue, M. E., and Ekenstedt, K. J. (2020). SNP-based heritability and genetic architecture of cranial cruciate ligament rupture in Labrador Retrievers. Anim. Genet. 51, 824–828. doi:10.1111/age.12978
Danecek, P., Auton, A., Abecasis, G., Albers, C. A., Banks, E., DePristo, M. A., et al. (2011). The variant call format and VCFtools. Bioinformatics 27, 2156–2158. doi:10.1093/bioinformatics/btr330
Decker, B., Davis, B. W., Rimbault, M., Long, A. H., Karlins, E., Jagannathan, V., et al. (2015). Comparison against 186 canid whole-genome sequences reveals survival strategies of an ancient clonally transmissible canine tumor. Genome Res. 25, 1646–1655. doi:10.1101/gr.190314.115
Deligne, C., Casulli, S., Pigenet, A., Bougault, C., Campillo-Gimenez, L., Nourissat, G., et al. (2015). Differential expression of interleukin-17 and interleukin-22 in inflamed and non-inflamed synovium from osteoarthritis patients. Osteoarthr. Cartil. 23, 1843–1852. doi:10.1016/j.joca.2014.12.007
Doherty, A., Lopes, I., Ford, C. T., Monaco, G., Guest, P., and de Magalhães, J. P. (2020). A scan for genes associated with cancer mortality and longevity in pedigree dog breeds. Mamm. Genome 31, 215–227. doi:10.1007/s00335-020-09845-1
Dreger, D. L., Davis, B. W., Cocco, R., Sechi, S., Di Cerbo, A., Parker, H. G., et al. (2016a). Commonalities in development of pure breeds and population isolates revealed in the genome of the Sardinian Fonni's Dog. Genetics 204, 737–755. doi:10.1534/genetics.116.192427
Dreger, D. L., Rimbault, M., Davis, B. W., Bhatnagar, A., Parker, H. G., and Ostrander, E. A. (2016b). Whole-genome sequence, SNP chips and pedigree structure: Building demographic profiles in domestic dog breeds to optimize genetic-trait mapping. Dis. Model. Mech. 9, 1445–1460. doi:10.1242/dmm.027037
Elliott, K. S., Chapman, K., Day-Williams, A., Panoutsopoulou, K., Southam, L., Lindgren, C. M., et al. (2013). Evaluation of the genetic overlap between osteoarthritis with body mass index and height using genome-wide association scan data. Ann. Rheum. Dis. 72, 935–941. doi:10.1136/annrheumdis-2012-202081
Fealey, M. J., Li, J., Todhunter, R. J., Krotscheck, U., Hayashi, K., McConkey, M. J., et al. (2017). Genetic mapping of principal components of canine pelvic morphology. Canine Genet. Epidemiol. 4, 4. doi:10.1186/s40575-017-0043-7
Fels, L., and Distl, O. (2014). Identification and validation of quantitative trait loci (QTL) for canine hip dysplasia (CHD) in German shepherd dogs. PLoS One 9, e96618. doi:10.1371/journal.pone.0096618
Fries, C. L., and Remedios, A. M. (1995). The pathogenesis and diagnosis of canine hip dysplasia: A review. Can. Vet. J. 36, 494–502. PMID: 7585436.
Ginja, M. M., Silvestre, A. M., Colaço, J., Gonzalo-Orden, J. M., Melo-Pinto, P., Orden, M. A., et al. (2009). Hip dysplasia in estrela mountain dogs: Prevalence and genetic trends 1991–2005. Vet. J. 182, 275–282. doi:10.1016/j.tvjl.2008.06.014
Ginja, M. M., Silvestre, A. M., Gonzalo-Orden, J. M., and Ferreira, A. J. (2010). Diagnosis, genetic control and preventive management of canine hip dysplasia: A review. Vet. J. 184, 269–276. doi:10.1016/j.tvjl.2009.04.009
Gnanadesikan, G. E., Hare, B., Snyder-Mackler, N., Call, J., Kaminski, J., Miklósi, Á., et al. (2020). Breed differences in dog cognition associated with brain-expressed genes and neurological functions. Integr. Comp. Biol. 60, 976–990. doi:10.1093/icb/icaa112
Gonzaga-Jauregui, C., Gamble, C. N., Yuan, B., Penney, S., Jhangiani, S., Muzny, D. M., et al. (2015). Mutations in COL27A1 cause Steel syndrome and suggest a founder mutation effect in the Puerto Rican population. Eur. J. Hum. Genet. 23, 342–346. doi:10.1038/ejhg.2014.107
Guthrie, S., and Pidduck, H. G. (1990). Heritability of elbow osteochondrosis within a closed population of dogs. J. Small Anim. Pract. 31, 93–96. doi:10.1111/j.1748-5827.1990.tb00730.x
Hamann, H., Kirchhoff, T., and Distl, O. (2003). Bayesian analysis of heritability of canine hip dysplasia in German Shepherd Dogs. J. Anim. Breed. Genet. 120, 258–268. doi:10.1046/j.1439-0388.2003.00395.x
Hamazaki, K., and Iwata, H. (2020). Rainbow: Haplotype-based genome-wide association study using a novel SNP-set method. PLoS Comput. Biol. 16, e1007663. doi:10.1371/journal.pcbi.1007663
Hayward, J. J., Castelhano, M. G., Oliveira, K. C., Corey, E., Balkman, C., Baxter, T. L., et al. (2016). Complex disease and phenotype mapping in the domestic dog. Nat. Commun. 7, 10460. doi:10.1038/ncomms10460
He, S., Yang, S., Zhang, Y., Li, X., Gao, D., Zhong, Y., et al. (2019). LncRNA ODIR1 inhibits osteogenic differentiation of hUC-MSCs through the FBXO25/H2BK120ub/H3K4me3/OSX axis. Cell. Death Dis. 10, 947. doi:10.1038/s41419-019-2148-2
Healey, E., Murphy, R. J., Hayward, J. J., Castelhano, M., Boyko, A. R., Hayashi, K., et al. (2019). Genetic mapping of distal femoral, stifle, and tibial radiographic morphology in dogs with cranial cruciate ligament disease. PLoS One 14, e0223094. doi:10.1371/journal.pone.0223094
Hoopes, B. C., Rimbault, M., Liebers, D., Ostrander, E. A., and Sutter, N. B. (2012). The insulin-like growth factor 1 receptor (IGF1R) contributes to reduced size in dogs. Mamm. Genome 23, 780–790. doi:10.1007/s00335-012-9417-z
Huang, M., Hayward, J. J., Corey, E., Garrison, S. J., Wagner, G. R., Krotscheck, U., et al. (2017). A novel iterative mixed model to remap three complex orthopedic traits in dogs. PLoS One 12, e0176932. doi:10.1371/journal.pone.0176932
Jagannathan, V., Drögemüller, C., and Leeb, T. Dog Biomedical Variant Database Consortium (DBVDC) (2019). A comprehensive biomedical variant catalogue based on whole genome sequences of 582 dogs and eight wolves. Anim. Genet. 50, 695–704. doi:10.1111/age.12834
Janutta, V., Hamann, H., and Distl, O. (2006). Complex segregation analysis of canine hip dysplasia in German shepherd dogs. J. Hered. 97, 13–20. doi:10.1093/jhered/esi128
Johnson, J. A., Austin, C. C., and Breur, G. J. (1994). Incidence of canine appendicular musculoskeletal disorders in 16 veterinary teaching hospitals from 1980 through 1989. Vet. Comp. Orthop. Traumatol. 7, 56–69. doi:10.1055/s-0038-1633097
Jones, P., Chase, K., Martin, A., Davern, P., Ostrander, E. A., and Lark, K. G. (2008). Single-nucleotide-polymorphism-based association mapping of dog stereotypes. Genetics 179, 1033–1044. doi:10.1534/genetics.108.087866
Karlsson, E. K., and Lindblad-Toh, K. (2008). Leader of the pack: Gene mapping in dogs and other model organisms. Nat. Rev. Genet. 9, 713–725. doi:10.1038/nrg2382
King, M. D. (2017). Etiopathogenesis of canine hip dysplasia, prevalence, and genetics. Vet. Clin. North Am. Small Anim. Pract. 47, 753–767. doi:10.1016/j.cvsm.2017.03.001
Krontveit, R. I., Nødtvedt, A., Sævik, B. K., Ropstad, E., and Trangerud, C. (2012). Housing-and exercise-related risk factors associated with the development of hip dysplasia as determined by radiographic evaluation in a prospective cohort of Newfoundlands, Labrador Retrievers, Leonbergers, and Irish Wolfhounds in Norway. Am. J. Vet. Res. 73, 838–846. doi:10.2460/ajvr.73.6.838
Krotscheck, U., and Todhunter, R. J. (2010). “Pathogenesis of hip dysplasia,” in Mechanisms of disease in small animal surgery. Editors M. J. Bojrab, and E. Monnet. 3rd Edition (Jackson WY: Teton NewMedia), 636–645.
Lane, J. M., Jones, S. E., Dashti, H. S., Wood, A. R., Aragam, K. G., van Hees, V. T., et al. (2019). Biological and clinical insights from genetics of insomnia symptoms. Nat. Genet. 51, 387–393. doi:10.1038/s41588-019-0361-7
Lavrijsen, I. C., Leegwater, P. A., Martin, A. J., Harris, S. J., Tryfonidou, M. A., Heuven, H. C., et al. (2014). Genome wide analysis indicates genes for basement membrane and cartilage matrix proteins as candidates for hip dysplasia in Labrador Retrievers. PLoS One 9, e87735. doi:10.1371/journal.pone.0087735
Lewis, T. W., Blott, S. C., and Woolliams, J. A. (2013). Comparative analyses of genetic trends and prospects for selection against hip and elbow dysplasia in 15 UK dog breeds. BMC Genet. 14, 16. doi:10.1186/1471-2156-14-16
Lindblad-Toh, K., Wade, C. M., Mikkelsen, T. S., Karlsson, E. K., Jaffe, D. B., Kamal, M., et al. (2005). Genome sequence, comparative analysis and haplotype structure of the domestic dog. Nature 438, 803–819. doi:10.1038/nature04338
Luo, C., Liang, J. S., Gong, J., Zhang, H. L., Feng, Z. J., Yang, H. T., et al. (2019). The function of microRNA-34a in osteoarthritis. Bratisl. Lek. Listy 120, 386–391. doi:10.4149/BLL_2019_063
Lust, G., Williams, A. J., Burton-Wurster, N., Pijanowski, G. J., Beck, K. A., Rubin, G., et al. (1993). Joint laxity and its association with hip dysplasia in Labrador Retrievers. Am. J. Vet. Res. 54, 1990–1999.
Lykkjen, S., Dolvik, N. I., McCue, M. E., Rendahl, A. K., Mickelson, J. R., and Roed, K. H. (2010). Genome-wide association analysis of osteochondrosis of the tibiotarsal joint in Norwegian Standardbred trotters. Anim. Genet. 41, 111–120. doi:10.1111/j.1365-2052.2010.02117.x
Mäki, K., Janss, L. L., Groen, A. F., Liinamo, A. E., and Ojala, M. (2004). An indication of major genes affecting hip and elbow dysplasia in four Finnish dog populations. Heredity 92, 402–408. doi:10.1038/sj.hdy.6800434
Mäki, K., Liinamo, A. E., and Ojala, M. (2000). Estimates of genetic parameters for hip and elbow dysplasia in Finnish Rottweilers. J. Anim. Sci. 78, 1141–1148. doi:10.2527/2000.7851141x
Michelsen, J. (2013). Canine elbow dysplasia: Aetiopathogenesis and current treatment recommendations. Vet. J. 196, 12–19. doi:10.1016/j.tvjl.2012.11.009
Mikkola, L., Holopainen, S., Pessa-Morikawa, T., Lappalainen, A. K., Hytönen, M. K., Lohi, H., et al. (2019). Genetic dissection of canine hip dysplasia phenotypes and osteoarthritis reveals three novel loci. BMC Genomics 20, 1027. doi:10.1186/s12864-019-6422-6
Muir, P. (1997). Physical examination of lame dogs. Compend. Continuing Educ. Pract. Veterinarian 19, 1149–1161.
Nap, R. C. (1995). “Pathophysiology and clinical aspects of canine elbow dysplasia,” in Proceedings of the 7th international elbow working group meeting, constance, Germany, 6–8.
Nielen, A. L., Janss, L. L., and Knol, B. W. (2001). Heritability estimations for diseases, coat color, body weight, and height in a birth cohort of Boxers. Am. J. Vet. Res. 62, 1198–1206. doi:10.2460/ajvr.2001.62.1198
Oberbauer, A. M., Keller, G. G., and Famula, T. R. (2017). Long-term genetic selection reduced prevalence of hip and elbow dysplasia in 60 dog breeds. PLoS One 12, e0172918. doi:10.1371/journal.pone.0172918
Ortiz, E. M., Dusetti, N. J., Vasseur, S., Malka, D., Bödeker, H., Dagorn, J. C., et al. (1998). The pancreatitis-associated protein is induced by free radicals in AR4-2J cells and confers cell resistance to apoptosis. Gastroenterology 114, 808–816. doi:10.1016/s0016-5085(98)70595-5
Ostrander, E. A., Wang, G. D., Larson, G., vonHoldt, B. M., Davis, B. W., Jagannathan, V., et al. (2019). Dog10K: An international sequencing effort to advance studies of canine domestication, phenotypes and health. Natl. Sci. Rev. 6, 810–824. doi:10.1093/nsr/nwz049
Parker, H. G., Dreger, D. L., Rimbault, M., Davis, B. W., Mullen, A. B., Carpintero-Ramirez, G., et al. (2017). Genomic analyses reveal the influence of geographic origin, migration, and hybridization on modern dog breed development. Cell. Rep. 19, 697–708. doi:10.1016/j.celrep.2017.03.079
Parker, H. G., VonHoldt, B. M., Quignon, P., Margulies, E. H., Shao, S., Mosher, D. S., et al. (2009). An expressed fgf4 retrogene is associated with breed-defining chondrodysplasia in domestic dogs. Science 325, 995–998. doi:10.1126/science.1173275
Pfahler, S., and Distl, O. (2012). Identification of quantitative trait loci (QTL) for canine hip dysplasia and canine elbow dysplasia in Bernese Mountain Dogs. PLoS One 7, e49782. doi:10.1371/journal.pone.0049782
Plassais, J., Kim, J., Davis, B. W., Karyadi, D. M., Hogan, A. N., Harris, A. C., et al. (2019). Whole genome sequencing of canids reveals genomic regions under selection and variants influencing morphology. Nat. Commun. 10, 1489. doi:10.1038/s41467-019-09373-w
Plassais, J., Rimbault, M., Williams, F. J., Davis, B. W., Schoenebeck, J. J., and Ostrander, E. A. (2017). Analysis of large versus small dogs reveals three genes on the canine X chromosome associated with body weight, muscling and back fat thickness. PLoS Genet. 13, e1006661. doi:10.1371/journal.pgen.1006661
Purcell, S., Neale, B., Todd-Brown, K., Thomas, L., Ferreira, M. A., Bender, D., et al. (2007). Plink: A tool set for whole-genome association and population-based linkage analyses. Am. J. Hum. Genet. 81, 559–575. doi:10.1086/519795
Rambaut, A. (2006). FigTree. Edinburgh, United Kingdom: Institute of Evolutionary Biology, University of Edinburgh. Available at: http://tree.bio.ed.ac.uk/software/figtree (Accessed June 2, 2020).
Ramos, Y. F., Metrustry, S., Arden, N., Bay-Jensen, A. C., Beekman, M., de Craen, A. J., et al. (2014). Meta-analysis identifies loci affecting levels of the potential osteoarthritis biomarkers sCOMP and uCTX-II with genome wide significance. J. Med. Genet. 51, 596–604. doi:10.1136/jmedgenet-2014-102478
Rangkasenee, N., Murani, E., Brunner, R., Schellander, K., Cinar, M. U., Scholz, A. M., et al. (2013). KRT8, FAF1 and PTH1R gene polymorphisms are associated with leg weakness traits in pigs. Mol. Biol. Rep. 40, 2859–2866. doi:10.1007/s11033-012-2301-9
Richardson, D. C. (1992). The role of nutrition in canine hip dysplasia. Vet. Clin. North Am. Small Anim. Pract. 22, 529–540. doi:10.1016/s0195-5616(92)50053-5
Sánchez-Molano, E., Woolliams, J. A., Pong-Wong, R., Clements, D. N., Blott, S. C., and Wiener, P. (2014). Quantitative trait loci mapping for canine hip dysplasia and its related traits in UK Labrador Retrievers. BMC Genomics 15, 833. doi:10.1186/1471-2164-15-833
Sargan, D. R. (2004). Idid: Inherited diseases in dogs: Web-based information for canine inherited disease genetics. Mamm. Genome 15, 503–506. doi:10.1007/s00335-004-3047-z
Schachner, E. R., and Lopez, M. J. (2015). Diagnosis, prevention, and management of canine hip dysplasia: A review. Vet. Med. 6, 181–192. doi:10.2147/VMRR.S53266
Schoenebeck, J. J., Hutchinson, S. A., Byers, A., Beale, H. C., Carrington, B., Faden, D. L., et al. (2012). Variation of BMP3 contributes to dog breed skull diversity. PLoS Genet. 8, e1002849. doi:10.1371/journal.pgen.1002849
Smith, G. K. (1998). Canine hip dysplasia: Pathogenesis, diagnosis, and genetic control. Vet. Q. 20, S22–S24. doi:10.1080/01652176.1998.10807390
Smith, S. P., Phillips, J. B., Johnson, M. L., Abbot, P., Capra, J. A., and Rokas, A. (2019). Genome-wide association analysis uncovers variants for reproductive variation across dog breeds and links to domestication. Evol. Med. Public Health 1, 93–103. doi:10.1093/emph/eoz015
Solovieva, S., Kämäräinen, O. P., Hirvonen, A., Hämäläinen, S., Laitala, M., Vehmas, T., et al. (2009). Association between interleukin 1 gene cluster polymorphisms and bilateral distal interphalangeal osteoarthritis. J. Rheumatol. 36, 1977–1986. doi:10.3899/jrheum.081238
Soo, M., Lopez-Villalobos, N., and Worth, A. J. (2018). Heritabilities and genetic trends for elbow score as recorded by the New Zealand Veterinary Association Elbow Dysplasia Scheme (1992–2013) in four breeds of dog. N. Z. Vet. J. 66, 154–161. doi:10.1080/00480169.2018.1440652
Strimmer, K. (2008). fdrtool: a versatile R package for estimating local and tail area-based false discovery rates. Bioinformatics 24, 1461–1462. doi:10.1093/bioinformatics/btn209
Struck, A. K., Braun, M., Detering, K. A., Dziallas, P., Neβler, J., Fehr, M., et al. (2020). A structural UGDH variant associated with standard Munchkin cats. BMC Genet. 21, 67–10. doi:10.1186/s12863-020-00875-x
Sutter, N. B., Bustamante, C. D., Chase, K., Gray, M. M., Zhao, K., Zhu, L., et al. (2007). A single IGF1 allele is a major determinant of small size in dogs. Science 316, 112–115. doi:10.1126/science.1137045
Sutter, N. B., Mosher, D. S., Gray, M. M., and Ostrander, E. A. (2008). Morphometrics within dog breeds are highly reproducible and dispute Rensch’s rule. Mamm. Genome 19, 713–723. doi:10.1007/s00335-008-9153-6
Tinga, S., Kim, S. E., Banks, S. A., Jones, S. C., Park, B. H., Pozzi, A., et al. (2018). Femorotibial kinematics in dogs with cranial cruciate ligament insufficiency: A three-dimensional in-vivo fluoroscopic analysis during walking. BMC Vet. Res. 14, 85. doi:10.1186/s12917-018-1395-2
Todhunter, R. J., Bliss, S. P., Casella, G., Wu, R., Lust, G., Burton-Wurster, N. I., et al. (2003). Genetic structure of susceptibility traits for hip dysplasia and microsatellite informativeness of an outcrossed canine pedigree. J. Hered. 94, 39–48. doi:10.1093/jhered/esg006
Todhunter, R. J., Mateescu, R., Lust, G., Burton-Wurster, N. I., Dykes, N. L., Bliss, S. P., et al. (2005). Quantitative trait loci for hip dysplasia in a crossbreed canine pedigree. Mamm. Genome 16, 720–730. doi:10.1007/s00335-005-0004-4
Vaysse, A., Ratnakumar, A., Derrien, T., Axelsson, E., Pielberg, G. R., Sigurdsson, S., et al. (2011). Identification of genomic regions associated with phenotypic variation between dog breeds using selection mapping. PLoS Genet. 7, e1002316. doi:10.1371/journal.pgen.1002316
Vermote, K. A., Bergenhuyzen, A. L., Gielen, I., van Bree, H., Duchateau, L., and Van Ryssen, B. (2010). Elbow lameness in dogs of six years and older: Arthroscopic and imaging findings of medial coronoid disease in 51 dogs. Vet. Comp. Orthop. Traumatol. 23, 43–50. doi:10.3415/VCOT-09-03-0032
Webster, M. T., Kamgari, N., Perloski, M., Hoeppner, M. P., Axelsson, E., Hedhammar, Å., et al. (2015). Linked genetic variants on chromosome 10 control ear morphology and body mass among dog breeds. BMC Genomics 16, 474. doi:10.1186/s12864-015-1702-2
Wickham, H., Averick, M., Bryan, J., Chang, W., McGowan, L. D., François, R., et al. (2019). Welcome to the tidyverse. J. Open Source Softw. 4, 1686. doi:10.21105/joss.01686
Wilke, V. L., Conzemius, M. G., Kinghorn, B. P., Macrossan, P. E., Cai, W., and Rothschild, M. F. (2006). Inheritance of rupture of the cranial cruciate ligament in Newfoundlands. J. Am. Vet. Med. Assoc. 228, 61–64. doi:10.2460/javma.228.1.61
Wilke, V. L., Robinson, D. A., Evans, R. B., Rothschild, M. F., and Conzemius, M. G. (2005). Estimate of the annual economic impact of treatment of cranial cruciate ligament injury in dogs in the United States. J. Am. Vet. Med. Assoc. 227, 1604–1607. doi:10.2460/javma.2005.227.1604
Witsberger, T. H., Villamil, J. A., Schultz, L. G., Hahn, A. W., and Cook, J. L. (2008). Prevalence of and risk factors for hip dysplasia and cranial cruciate ligament deficiency in dogs. J. Am. Vet. Med. Assoc. 232, 1818–1824. doi:10.2460/javma.232.12.1818
Zapata, I., Serpell, J. A., and Alvarez, C. E. (2016). Genetic mapping of canine fear and aggression. BMC Genomics 17, 572. doi:10.1186/s12864-016-2936-3
Zhang, L., Zhou, F., van Laar, T., Zhang, J., van Dam, H., and Ten Dijke, P. (2011). Fas-associated factor 1 antagonizes Wnt signaling by promoting β-catenin degradation. Mol. Biol. Cell. 22, 1617–1624. doi:10.1091/mbc.E10-12-0985
Zhang, Y., Bowen, T. R., Lietman, S. A., Suk, M., Williams, M. S., and Lee, M. T. (2020). PPARGC1B is associated with nontraumatic osteonecrosis of the femoral head: A genomewide association study on a chart-reviewed cohort. J. Bone Jt. Surg. Am. 102, 1628–1636. doi:10.2106/JBJS.19.01335
Zhou, X., Carbonetto, P., and Stephens, M. (2013). Polygenic modeling with bayesian sparse linear mixed models. PLoS Genet. 9, e1003264. doi:10.1371/journal.pgen.1003264
Zhou, X., and Stephens, M. (2012). Genome-wide efficient mixed-model analysis for association studies. Nat. Genet. 44, 821–824. doi:10.1038/ng.2310
Zhou, Z., Sheng, X., Zhang, Z., Zhao, K., Zhu, L., Guo, G., et al. (2010). Differential genetic regulation of canine hip dysplasia and osteoarthritis. PLoS One 5, e13219. doi:10.1371/journal.pone.0013219
Keywords: dog, GWAS, breed phenotypes, whole-genome sequencing SNPs, spontaneous orthopedic disease, weighted least squares regression
Citation: Binversie EE, Momen M, Rosa GJM, Davis BW and Muir P (2022) Across-breed genetic investigation of canine hip dysplasia, elbow dysplasia, and anterior cruciate ligament rupture using whole-genome sequencing. Front. Genet. 13:913354. doi: 10.3389/fgene.2022.913354
Received: 05 April 2022; Accepted: 07 November 2022;
Published: 02 December 2022.
Edited by:
Yuan-Ming Zhang, Huazhong Agricultural University, ChinaReviewed by:
Ottmar Distl, University of Veterinary Medicine Hannover, GermanyHailiang Song, Beijing Academy of Agricultural and Forestry Sciences, China
Copyright © 2022 Binversie, Momen, Rosa, Davis and Muir. This is an open-access article distributed under the terms of the Creative Commons Attribution License (CC BY). The use, distribution or reproduction in other forums is permitted, provided the original author(s) and the copyright owner(s) are credited and that the original publication in this journal is cited, in accordance with accepted academic practice. No use, distribution or reproduction is permitted which does not comply with these terms.
*Correspondence: Peter Muir, peter.muir@wisc.edu