- 1Animal Production Research Institute, Agriculture Research Center (ARC), Cairo, Egypt
- 2Agricultural Genetic Engineering Research Institute, Giza, Egypt
- 3African Genome Center, Mohammed VI Polytechnic University, Ben Guerir, Morocco
- 4International Center For Agricultural Research in the Dry Areas (ICARDA), Giza, Egypt
Heat stress caused by climatic changes is one of the most significant stresses on livestock in hot and dry areas. It has particularly adverse effects on the ability of the breed to maintain homeothermy. Developing countries are advised to protect and prepare their animal resources in the face of potential threats such as climate change. The current study was conducted in Egypt’s three hot and dry agro-ecological zones. Three local sheep breeds (Saidi, Wahati, and Barki) were studied with a total of 206 ewes. The animals were exercised under natural heat stress. The heat tolerance index of the animals was calculated to identify animals with high and low heat tolerance based on their response to meteorological and physiological parameters. Genomic variation in these breeds was assessed using 64,756 single nucleotide polymorphic markers (SNPs). From the perspective of comparative adaptability to harsh conditions, our objective was to investigate the genomic structure that might control the adaptability of local sheep breeds to environmental stress under hot and dry conditions. In addition, indices of population structure and diversity of local breeds were examined. Measures of genetic diversity showed a significant influence of breed and location on populations. The standardized index of association (rbarD) ranged from 0.0012 (Dakhla) to 0.026 (Assuit), while for the breed, they ranged from 0.004 (Wahati) to 0.0103 (Saidi). The index of association analysis (Ia) ranged from 1.42 (Dakhla) to 35.88 (Assuit) by location and from 6.58 (Wahati) to 15.36 (Saidi) by breed. The most significant SNPs associated with heat tolerance were found in the MYO5A, PRKG1, GSTCD, and RTN1 genes (p ≤ 0.0001). MYO5A produces a protein widely distributed in the melanin-producing neural crest of the skin. Genetic association between genetic and phenotypic variations showed that OAR1_18300122.1, located in ST3GAL3, had the greatest positive effect on heat tolerance. Genome-wide association analysis identified SNPs associated with heat tolerance in the PLCB1, STEAP3, KSR2, UNC13C, PEBP4, and GPAT2 genes.
1 Introduction
Heat stress caused by climate change is one of the most pressing problems in animal production, especially in hot and dry regions. Due to their rapid metabolic rate and growth, high production level, and species-specific traits such as rumen fermentation, sweating disability, and skin insulation, ruminants are vulnerable to heat stress (Gonzalez-Rivas et al., 2020). Heat stress disrupts homeostasis, affects biological balance, and limits animal production, e.g., through lower milk yields and quality, lower meat production, and lower fertility (Sejian et al., 2018). These impacts have become even more pronounced with global warming, increasing stress on animal species worldwide. Each year, heat stress costs countries such as the United States of America between $1.9 and $2.7 billion in lost economic output (Godde et al., 2021). As a result, food security based on livestock production is threatened in many parts of the world (Collier et al., 2019).
Global climate change has significant implications for the arid and semiarid regions of West Asia and North Africa (WANA), where expected climatic changes in the Near East will most likely result in a faster increase in average and maximum temperatures than the global rate (Change, 2014). Studying the population structure of livestock breeds found in WANA regions will help us understand the effects of geographic location on their adaptation to such harsh environments (Cloete et al., 2014; Gboshe and Osarenakhue, 2020). Sheep and goats are the most commonly kept livestock in the hot, arid areas of the Near East, where rural populations rely on them. Local breeds face the challenge of adapting their physiological traits to the increasingly hot and dry weather, as well as the decreasing availability of grass and water (Rahimi et al., 2021). It is well known that sheep and goats can survive and reproduce longer under hot, dry conditions than other livestock. They rely on their behavioral and physiological adaptive mechanisms to heat stress. Sheep can tolerate water deprivation for up to 7 days and survive without food for more than 4 days under hot summer conditions (Gaughan et al., 2008). Climatic factors such as air temperature, relative humidity, solar radiation, and precipitation patterns significantly influence animal survival, disease, parasite infestation, and availability of forage resources (Sejian et al., 2018).
In addition, SNP genotyping technology is being used to study animal breed diversity and population structure (Girish and Dubey, 2018; Ghoreishifar et al., 2019). Understanding this diversity may lead to inferences about the interactions between different breeds that share geographic regions, including further information about the genetic evolution of animals and their historical origins in ancient parts of the world. In rural areas where flock sizes are small and the implementation of recording schemes is nearly impossible, a number of global and local plans of action encourage the importance of diversity characterization that can support breeding and conservation efforts (Haile et al., 2019; Pilling et al., 2020). The Food and Agriculture Organization’s global strategic action plans are also intended to promote the importance of diversity characterization, monitoring indigenous breeds, utilization for sustainable agriculture, and conservation efforts. These programs aim to promote diversity representation within developing countries and awareness campaigns to ensure the characterization and use of indigenous breeds (Monau et al., 2020).
Using genotyping technologies can help identify animals with superior adaptive traits, such as the ability to withstand environmental stressors common in harsh environments, which will ensure the long-term viability of future breed improvement strategies. It can provide a genetic selection model for breeding programs to produce elite and well-adapted breeds. This study aimed to examine the existing genome architecture that may contribute to the adaptability of local sheep breeds to environmental stress under the prevailing hot, dry conditions in Egypt.
2 Methodology
2.1 Agroecological zones
Fieldwork was conducted from 2009 to 2019 in three agroecological zones with harsh environmental conditions (Figure 1); A) Coastal Zone of the Western Desert (CZWD), a region stretching from Alexandria (Egypt) in the east to Tripoli (Libya) in the west, with an annual rainfall of fewer than 150 ml, 3–4 months of poor quality winter, and sparse vegetation in the long summer; B) Desert Oasis in the New Valley (NV) in southwestern Egypt, from the Libyan border in the west to the Nile Valley in the east and from Matrouh Governorate in the north to the Sudanese border in the south. It includes three major desert oases: Dakhla, Kharga, and Farafra. Environmental conditions are hot in summer (ambient temperature under scales up to 50°C), sparse rainfall (2–10 mm/year), and very intense solar radiation; amutilizationrature varies greatly between day and night, often exceeding 20°C; C) Upper Egypt is a hot, arid region stretching from Giza in the north to the Sudanese border in the south (latitudes: 22° south to 29° north). It is characterized by intense sunshine, very hot summers, cold winter nights, and low rainfall (15 mm/year). The ambient temperature varies greatly between day and night. The predominant agroecology is intensive agriculture with more than one crop per year and a mixture of arable and livestock farming.
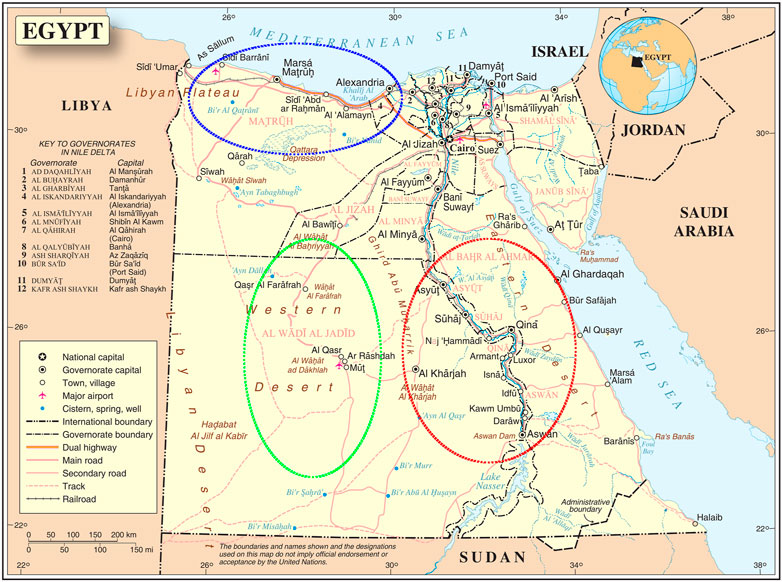
FIGURE 1. The three agroecological zones where local sheep breeds were collected. Western desert coastal zone (blue stroked), New Valley desert oasis (green stroked), and upper Egypt (red stroked) (map source united nations: www.un.org).
2.2 Breeds
Three different local Egyptian sheep breeds were included in our study, all of which were native to the three agroecological zones studied. The sheep breeds collected included Barki (83 animals), Wahati (55 animals) and Saidi (68 animals), totaling 206 animals. All the animals were non-lactating, non-pregnant females ranging in parity from 2 to 4. A) The Barki desert sheep, a native of the CZWD known for its adaptation to desert environments, is a fat-tailed sheep with open, coarse wool, a white body, and a colored head (Shebaita and El-Banna, 1982; Aboul-Naga, 1985; Agha et al., 2008; Aboul Naga et al., 2021). For centuries, they have been subject to natural selection in desert areas known for high temperatures, intense sunlight, frequent drought, and extensive grazing. B) Wahati sheep (also called Farafra) have a narrow, medium-sized body and a medium-sized head with a straight profile. Most rams are unhorned and have white fleece and small ears. The fat tail has a broad base that ends in a cylindrical part. The animals have a white body with a brown head or an all-white body (Elshazly and Youngs, 2019; Talaat Bashandy et al., 2020) and C) Saidi sheep, the native Upper Egyptian breed, are bred in small flocks; they are known for their tolerance to heat stress and the prevailing hot, dry environment (Galal et al., 2008). The Saidi sheep are considered the oldest Egyptian sheep breed and are characterized by good fertility but high mortality of the young animals (El-Hommosi and Abdel-Hafiz, 1982; Elshazly and Youngs, 2019). They are mostly dark in color and have an open, coarse fleece. Some specimens are cream or mixed colored. They have a Roman nose and dewlap under the neck, while the tail is long, thin, and covered with wool (Gaili et al., 1994). The studied populations were collected in six different geographical regions of Egypt (Borg Arab, Mallawy, Aswan, Matrouh, Assuit, Farafra, Kharga, and Dakhla). Egypt’s western, the west-east and south regions are represented by these geographical locations (Figure 1).
2.3 Experimental procedures
Animals were given physical activity under natural heat stress (PHS) during the study period, which consisted of walking approximately 7 km under direct sunlight in July and August (simulating summer grazing on poor pasture). During winter, animals grazed on ranges and received green fodder when available, and in summer, crop residues plus concentrates, depending on maintenance needs. Animals had full access to fresh water except during exercise.
2.3.1 Meteorological parameters
Dry bulb temperature (DBT) and relative humidity (RH) were calculated and recorded using an alcoholic thermometer and a hydrometer at rest, by 7 a.m., and during exposure. The Temperature-Humidity Index (THI) was calculated using the formula of Hahn et al. (Hahn et al., 2003) (Eq. 1). The THI ranged from 98.6 to 109.3 (Table 1), indicating that the animals were under severe heat stress.
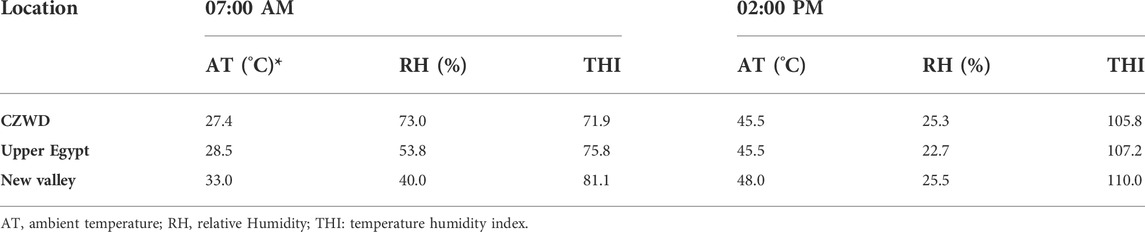
TABLE 1. Climatic parameters in the morning and afternoon for various locations on the day of sample collection.
2.3.2 Physiological parameters
Physiological measurements were taken before (07:00 a.m.) and after (02:00 p.m.) exposure to PHS. The thermal parameters were ear temperature (ET, °C), and rectal temperature (RT, °C), measured with infrared and clinic thermometer, respectively. The parameters used to calculate respiratory rate were gas volume (GV, L/min) and respiratory rate (RR, res./min), measured by tidal volume (TV, calculated as GV/RR) and a dry gas meter, respectively. Consumption of oxygen (VO2) and production of carbon dioxide (VCO2) were measured using the open-circuit technique (Yousef and Dill, 1969), and metabolic rate (MR) was calculated as kcal. BW 0.75/day (Brouwer, 1965).
Animal Heat Tolerant Index (ATHI) (Krupin, 2021) was calculated to identify high and low heat tolerant animals based on animal response in the leading five physiological parameters; RT, ET, RR, TV, and MR. Animals scored one if the change in the physiological parameter ≥2 standard deviation of its value at rest; and scored 0 if not. The sum of the five parameter scores was used as AHTI; "0" index is the most tolerant animal, and "5" index is the least tolerant one.
2.4 DNA extraction and SNP genotyping
At the time of exposure, blood samples were collected from each animal. DNA was extracted from whole blood samples using the Qiagen DNA Mini Kit (Qiagen GmbH, Germany) following the manufacturer’s protocol. DNA samples were genotyped using 64,756 single nucleotide polymorphic markers (SNPs) from the Ovine Infinium HD Bead Chip (Illumina, San Diego, CA, United States) by Neogen’s GeneSeek ⓒ (Lincoln, NE, United States) as a commercial service. The R package “poppr” (Kamvar et al., 2014) was used to perform the SNP quality control. SNPs were filtered for minor allele frequency (MAF) < 0.01, genotyping call rate <90%, and HWE p-value < 10−6.
2.5 Molecular data and population structure analyses
Diversity indices were used to investigate the richness and evenness of genotypic data and assess population structure. The genetic informativeness of the SNPs data was assessed using Hexp Nei’s unbiased gene diversity (Nei, 1978) (Eq. 2), the index of association Ia (Brown et al., 1980) (Eq. 3), and the standardized index of association (rbarD).
The rbarD (Brown et al., 1980) and the index of association analysis (Ia) (Agapow and Burt, 2001) were estimated to investigate the mode of reproduction and random mating. The rbarD and Ia expectations for a randomly mated population are both zero. Any significant deviation from the assumed value of zero would indicate clonal reproduction. We also calculated the heterozygosity index (Hexp), which indicates population diversity. Hexp, is widely used measure for assessing expected heterozygosity, underestimates true population diversity in samples with relatives (Simpson, 1949; Harris and DeGiorgio, 2017) (Table 2). These genetic indices were calculated using the summarization function “poppr” in the poppr (v2.8.6) R package. (Kamvar et al., 2014). Pairwise Fst values calculated between geographic locations using Weir and Cockerham’s method (Weir and Cockerham, 1984) were used in an analysis to estimate the degree of variation between samples and to construct Neighbor-Joining trees.
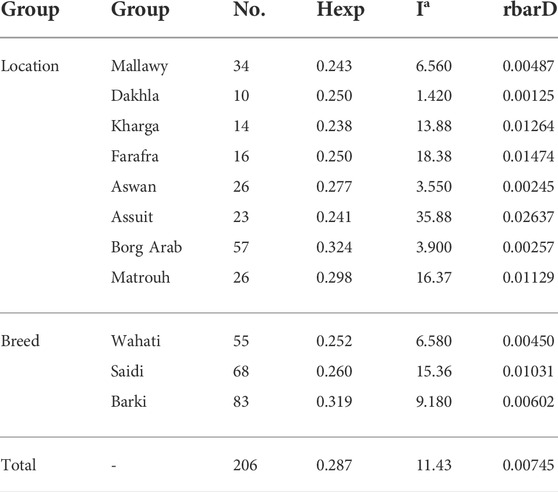
TABLE 2. The diversity statistics analysis, genetic richness, and evenness using Hexp Nei’s unbiased gene diversity (Nei, 1978), the index of association (Ia) (Agapow and Burt, 2001), and the standardized index of association (rbarD) (Brown et al., 1980).
The principal component analysis was used to reduce the complexity of genetic variation while retaining trends and patterns. This dimension reduction could generate feature summaries to identify specific clustering that may be influenced by geographic location and breed type. The discriminant analysis of principal components (DAPC) was performed on the sample for cluster analysis without prior information on individual populations using poppr v2.8.6 (Kamvar et al., 2014). Adegenet v2.1.3 (Jombart, 2008) was used for k-means clustering. Phylogenetic analysis and tree construction was performed using ape v5.4.1 (Paradis et al., 2004), and plotted using iToL software (Letunic and Bork, 2019).
The genetic structure of the population was determined using the LEA v3.2.0 R package (Gain and François, 2020) using a Bayesian model. The population structure analysis is used to evaluate genetic admixture, which is the process or result of interbreeding between two or more previously isolated populations within a species (Yuan et al., 2017). The admixture ancestry model was developed for several sub-populations (K) ranging from 1 to 10, with 100,000 iterations for each K-value. Using a cross-validation technique, the “snmf” function in the LEA v3.2.0 R package (Gain and François, 2020) was used to calculate an entropy criterion that evaluates the quality of fit of the statistical model to the data. Under the assumption of K ancestral populations, it provides least-squares estimates of ancestry proportions rather than maximum likelihood estimates (François and Durand, 2010).
2.6 Genome-wide association and gene ontology analyses
Genome-wide association analysis (GWAS) was performed using statgenGWAS (van Rossum et al., 2020). Using the Efficient Mixed Model Association (EMMA) model (Eq. 4), statgenGWAS was used to compute REML-estimates of the variance components (Kang et al., 2008). The significance threshold for SNP-trait associations was tested using the false discovery rate method (FDR) (p-value of ≤0.001) (Brzyski et al., 2017). The genetic kinship, which evaluates the degree of genetic relatedness or the coefficient of relationship between individuals, was calculated inside the statgenGWAS pipeline using “vanRaden” (VanRaden, 2008). The statgenGWAS package’s plot function was used to generate Manhattan and QQ plots with the default parameters. The iSheep online database was used to find genes close to or adjoin trait-associated SNPs located on the sheep genome (version of Oar v4.0.) (Wang Z.-H. et al., 2021). The gene enrichment analysis was used to identify biological pathways that are over-represented in trait-associated genes and may be related to the heat tolerance phenotype using ShinyGo online software (Ge et al., 2020).
3 Results
3.1 Genetic diversity and population structure
Genetic diversity was measured in different breed types and geographic locations. Various population statistics were used to examine the genetic diversity of the sheep population studied (Table 2). The rbarD values for the sheep sample ranged from 0.0012 (Dakhla) to 0.026 (Assuit), while for the breed they ranged from 0.004 (Wahati) to 0.0103 (Saidi) and totaled 0.007 (Table 2). The genetic parameter of Ia, on the other hand, ranged from 1.42 (Dakhla) to 35.88 (Assuit) by location and from 6.58 (Wahati) to 15.36 (Saidi) by breed. Borg Arab (0.324) and Barki (0.319) had the highest Hexp values depending on location and breed (Table 2). Phylogenetic analysis was performed to determine the genetic influence of sampling location and breed on the genetic variability of the sheep collection studied (Figure 2A). The phylogenetic tree constructed from the SNPs data reflected both breed type and geographic location (Figure 2A). The collected samples were divided into clusters reflecting the three main breeds, Wahati, Saidi, and Braki. Most of the Barki breed was clustered into one group, while the Saidi were divided into two groups. One of these groups differed greatly, while the other group showed some similarity to other breeds. Most of the Wahati group was grouped together, while the remaining members were scattered throughout the phylogenetic tree. The phylogenetic tree revealed some associations with breed regions depending on the geographic locations of the collected sheep samples (Figure 2A). The samples from the Borg Arab region were strongly clustered in one group. In addition, samples from Mallawy, Farafra, Assuit, Kharga, and Dakhla were mostly clustered in a few major groups (Figure 2A). Samples from western Egypt, such as Farafra, Kharga, Dakhla, and Borg Arab (Figure 1), were mostly collected in the upper part of the phylogenetic tree (Figure 2A). Samples collected from southern Egypt, such as Mallawy, Assuit, and Aswan, were clustered in the lower part of the phylogenetic tree (Figure 2A). The highest possible K for the studied sheep population is three according to the population structure analysis (Figures 2B,C). Depending on the breed, the first population is dominated by Wahati, the second by the Barki breed, and the third by the Saidi breed (Figure 2C). Depending on the sampling location, most of the samples from Mallawy, Kharga, and Dakhla were grouped in the first population, Borg Arab in the second population, and Aswan, Assuit, and Matrouh were grouped in the third population (Figure 2C).
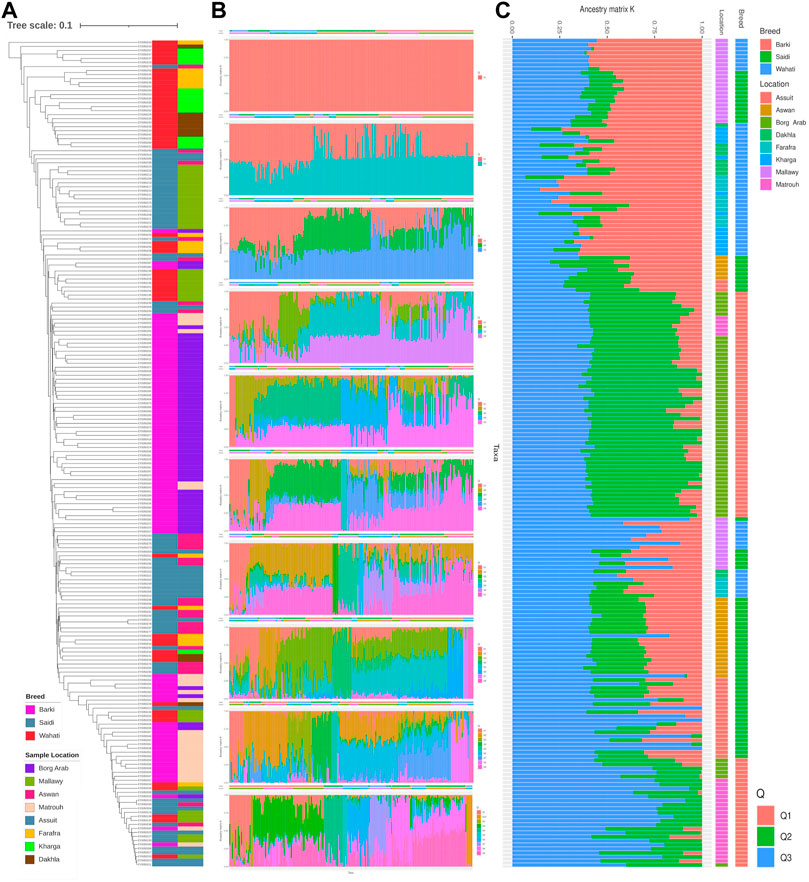
FIGURE 2. The diversity analysis of the studied sheep population. (A) Phylogenetic tree of the sheep population studied, colored by breed and location. (B) Population structure based on the optimal number of clusters (k-means) solutions for K = 1–10. (C) Population structure based on the optimal K of 3.
Principal component analysis (PCA) and discriminant analysis of principal components (DAPC) were used to cluster sheep populations as a function of breed and sampling location using principal component and reduction algorithms. PCA analysis showed a low ability to discriminate genetic variance among the samples studied based on breed level (Figure 3A). PCA revealed that it could explain 2.8 and 1.6% of the detected variance on the first and second axes, respectively. It showed that there were crosses between samples of Saidi and Wahati breeds, and some of these samples were very different from the two breeds. On the other hand, the majority of Barki samples were easily distinguishable (Figure 3A). Compared with PCA, DAPC analysis gave more precise and efficient explanation, explaining 75.2 and 24.8% on the first and second axes, respectively. Breeds were highly distinguishable and clustered in large groups with a small number of intercrossing (Figure 3B). At the sampling location level, PCA analysis explained 2.7 and 1.8% on the first axis, with sites grouped into major groups primarily influenced by geographic location (Figure 3C). Asuit and Aswan (southern Egypt), Farafra, Kharga, and Dakhla (southwestern Egypt), and Borg Arab and Matrouh (northwestern Egypt) were grouped into clusters. This can be clearly seen in the DAPC analysis, which explains a higher variation on the first (56.5%) and second (22.1%) axes compared to the PCA analysis (Figure 3D). Mallawy samples were clearly clustered in the DPAC analysis and slightly clustered in the PCA (Figures 3C,D).
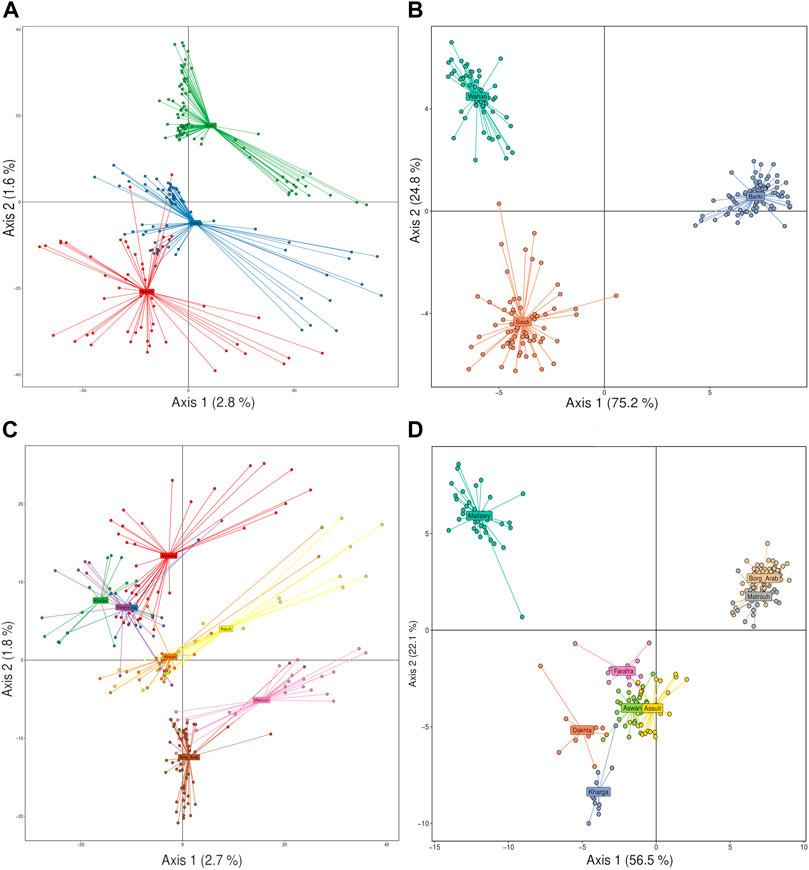
FIGURE 3. Discriminant analysis of principal components (DAPC) and PCA of the Egyptian sheep breed studied performed based on SNP genotyping data. Breed data was clustered using DAPC (A) and PCA (B), and geographical location data was clustered using PCA (C) and DAPC (D).
DAPC analysis was used to determine the population structure of the breeds under evaluation (Figure 4). When comparing the number of cluster solutions (k-means) using the Bayesian Information Criterion, the optimal K (number of populations) was determined to be two or 3, which corresponded to the population structure (Figure 4A). Linear discriminant analysis (LDA) generated using the DPAC reduction algorithm classified the studied breed into three distinct groups: one large group and two smaller groups (Figure 4B). The population structure diagrams generated using DPAC analysis show considerable overlap between the Saidi and Barki breeds, with only a small proportion of Saidi differing. The Wahati group differs significantly at k = 3 and k = 4 (Figure 4C). Kinship and Weir-Cockerham fixation index analyses were used to evaluate the interbreeding, and intrabreeding levels of the populations studied (Figure 5).
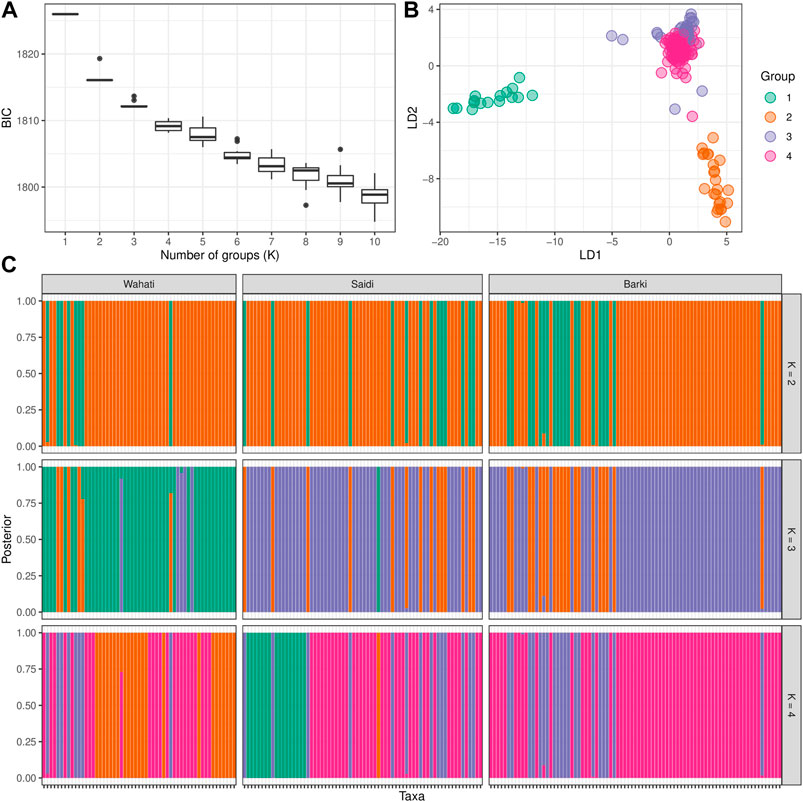
FIGURE 4. Population structure determined by the discriminant analysis of principal components (DAPC) algorithm. (A) The optimal number of clusters (k-means) solutions compared using the Bayesian Information Criterion (BIC). (B) The linear discriminant analysis (LDA) produced using dimension-reduction depending on DPAC algorithm. (C) The STRUCTURE-like plot of the studied population based on the best K ranges.
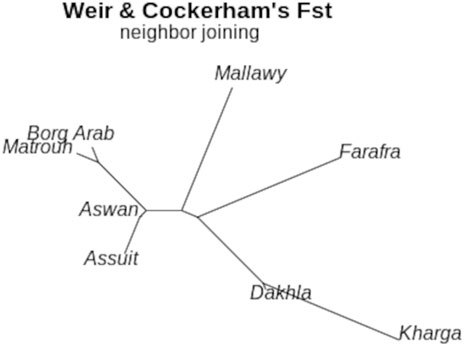
FIGURE 5. Neighbor-Joining tree for sheep subpopulations located in different geographical regions based on estimated Fst (theta) values calculated using the Weir-Cockerham fixation index method (Weir and Cockerham, 1984)
3.2 Genome-wide association analysis
GWAS analysis was used to identify SNPs associated with the heat tolerance phenotype in the studied sheep population, which originated from different Egyptian regions. The GWAS study revealed 46 SNPs showing genetic variation in the studied population and significantly associated with the heat tolerance phenotype (p-value of ≤0.001) (Figure 6). These SNPs are located on chromosomes Chr1 (6 SNPs), Chr7 (5 SNPs), ChrNA (4 SNPs), Chr2 (3 SNPs), Chr5 (3 SNPs), Chr3 (3 SNPs), Chr19 (3 SNPs), Chr6 (3 SNPs), Chr8 (2 SNPs), Chr16 (2 SNPs), Chr11 (2 SNPs), Chr4 (2 SNPs), Chr23 (2 SNPs), Chr13 (1 SNPs), Chr10 (1 SNPs), Chr12 (1 SNPs), Chr17 (1 SNPs), Chr22 (1 SNPs), and Chr21 (1 SNPs) (Figure 6A and Table 3). The variation of these SNPs in relation to the genomic reference of sheep is A>G (22 SNPs), G>A (13 SNPs), C>A (6 SNPs), A>C (4 SNPs), and T>A (1 SNPs). The correlation between SNPs and heat tolerance ranged from 2.88 logp-value (OAR7_60745094.1) to 4.51 logp-value (OAR7_60704536.1). Heat tolerance was associated with these SNPs, with significance ranging from 2.88 logp-value (OAR7_60745094.1) to 4.51 logp-value (OAR7_60745094.1). (OAR7_60704536.1) (Table 3). The effect of these SNPs on heat tolerance ranged from 0.57 to 1.47 units, with both positive and negative effects. The positive effect ranged from 0.57 units (OAR7_60745094.1 and OAR4_17771871.1) to 1.47 units (OAR1_18300122.1), whereas the negative effect ranged from -0.55 units (OAR7_58797217.1) to -1.18 units (OAR2_172930491.1). Gene annotation analysis revealed that these SNPs were located in 36 different genes, with ATP2C1 and MYO5A each having two SNPs (Table 3 and Figure 7). Gene ontology analysis identified genes involved in biological pathways such as calcium and manganese binding or transport, epidermal growth, and cell adhesion (Figure 7, Supplementary Figure S1 and Supplementary Table S2). In addition, EGF-domain related genes (EGFL6, NCAN, LTBP1, LAMA2, DSC2, and UNC13C genes), Kinase (KSR2, UNC13C, PRKG1, SH3BGR, PLCB1, and WWP1), growth (FGF9, NCAN, LTBP1, and EGFL6), and collagen-containing extracellular matri (COL28A1,LTBP1,LAMA2, and ERBB2IP) were detected.
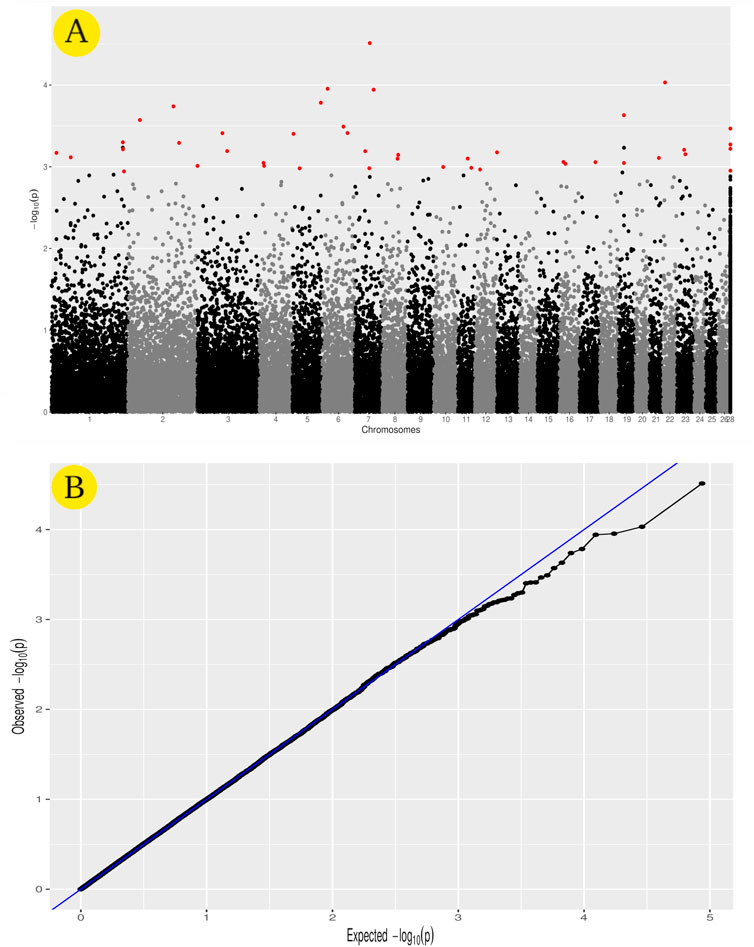
FIGURE 6. GWAS for heat tolerance in the studied Egyptian sheep breeds. The Manhattan plot of the studied genetic variation association with heat tolerance (A), as well as the Quantile–quantile plots and Manhattan plots for the GWAS (B).
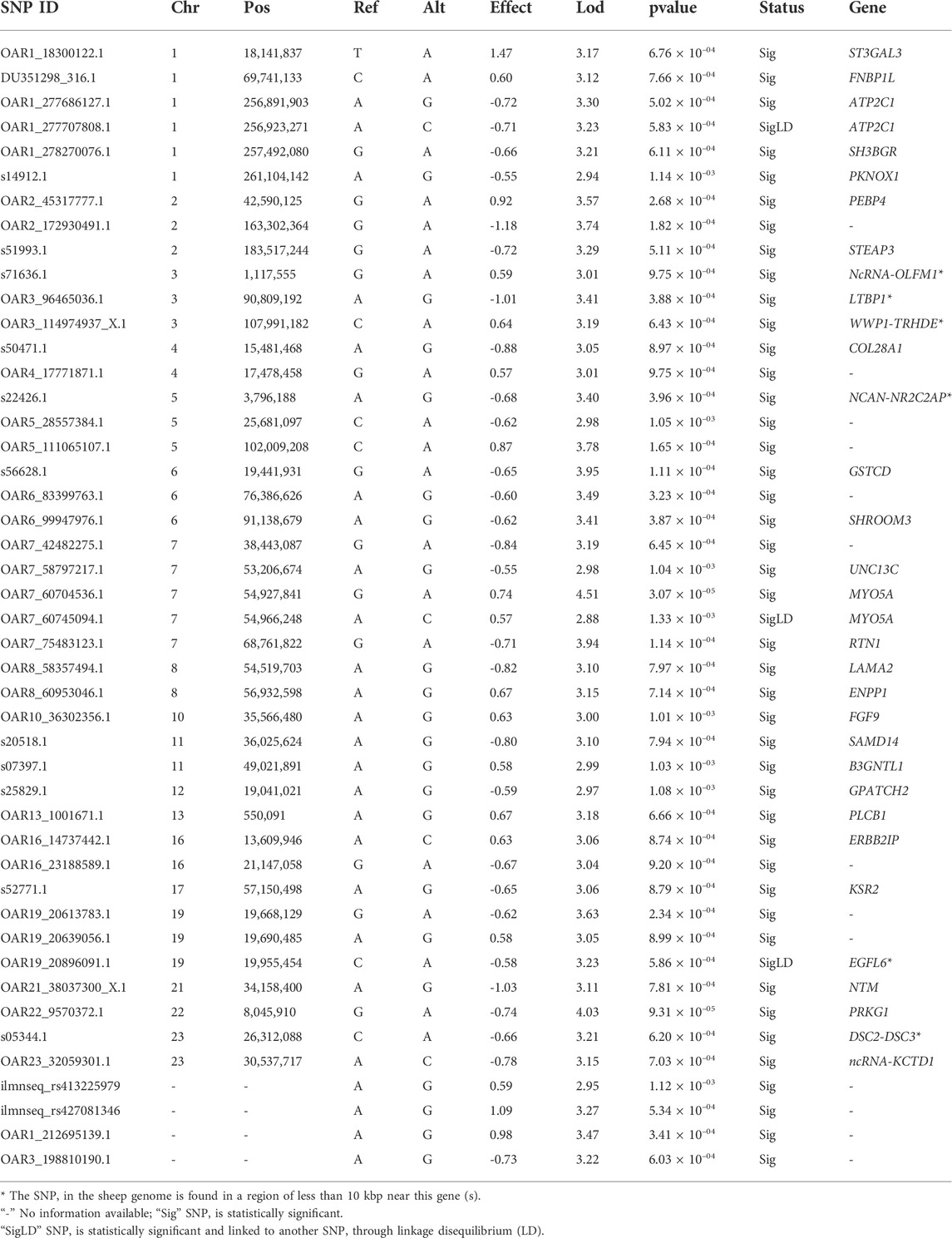
TABLE 3. List of candidate genes associated with the heat tolerance trait in the Egyptian bres studied. The status column indicates the state of SNP significance and whether it is linked to other SNPs via linkage disequilibrium.
4 Discussion
Climate change is raising global temperatures, resulting in drastic changes in ambient temperature. As a result, the animals will be unable to produce enough heat to maintain homeothermy, putting them at risk of severe heat stress (Daramola et al., 2012). Developing countries must conserve resources and plan for future threats to protect their livestock. Our study investigated the genomic variation of 206 sheep from three Egyptian breeds (Wahati, Saidi, and Braki) collected from six different geographical locations in Egypt (Borg Arab, Mallawy, Aswan, Matrouh, Assuit, Farafra, Kharga, Dakhla). These samples were genotyped for 64,756 SNP markers. The genetic diversity and population structure of these breeds were assessed, and a possible association between heat stress indicators (phenotypes) and genome-wide SNPs was investigated.
The diversity analysis indices were useful in demonstrating the impact of location and breed. The rbarD (Brown et al., 1980) and Ia (Agapow and Burt, 2001) parameters show low values for isolated geographical locations such as Dakhla, Aswan, and Borg Arab, which are located on the outskirts of Egypt (Figures 1, 2). Breeds found in remote areas, such as Wahati, produced similar results 2). Hexp values, on the other hand, were slightly higher for isolated locations such as Borg Arab 2). According to the phylogenetic tree and the population structure (Figure 2) constructed from SNP genotyping data, the influence of breed is more evident than that of sampling location. The highest possible K for the studied sheep population is three according to the population structure analysis (Figures 2B,C). Even though the breed clearly influenced some populations, the Saidi breed has crosses with other breeds. The population structure generated by the DAPC algorithm (Jombart and Collins, 2015) confirmed the population number determined by the LEA tool (Frichot and François, 2015). PCA analysis could not distinguish between the Saidi and Wahati, resulting in a low explanation on both axes. According to the DAPC, Wahati and Barki are very different breeds, differing greatly on both axes (Figure 3B). Both Wahati and Barki sub-populations are well adapted to harsh desert conditions such as feed shortages and high ambient temperatures. They can produce large amounts of meat, wool, and milk under these conditions (El-Wakil et al., 2008; Aboulnaga et al., 2021). The effect of geographic location on group clustering was highlighted by DAPC analysis (Figure 3D). Samples from Aswan and Assuti (southern Egypt), Borg Arab and Matrouh (northwestern coastal zone of Egypt), and Dakhla, Farafra, and Kharga (western desert) were divided into different groups. The different population structures of the desert breeds studied were also described in previous reports (Othman et al., 2016). The ability of the DAPC method to outperform PCA in achieving a clear difference in variance between populations and producing an appropriate group score is consistent with previous reports (Azizi et al., 2017). FST coefficient was calculated to assess genetic differentiation and relatedness among individuals. It provided additional information on the breeds studied and confirmed the influence of geographic location (Figure 5). The phylogenetic tree constructed with Weir and Cockerham fixation index showed the proximity between the desert breeds (Dakhla and Kharga).
When an animal begins to suffer heat exhaustion, food intake decreases, and metabolism slows, leading to hypothyroidism and significant economic losses (West, 2003). GWAS analysis was used to identify SNPs associated with the heat tolerance phenotype in the sheep population studied (Figure 1). It revealed 46 SNPs showing genetic variation in the studied population and significantly associated (p-value of ≤0.001) with the heat tolerance phenotype (Figure 6). Gene ontology analysis identified genes involved in biological pathways such as calcium and manganese binding or transport, epidermal growth, and cell adhesion (Figure 7 and Supplementary Figure S1). The most significant SNPs associated with heat tolerance were found in the genes MYO5A, PRKG1, GSTCD, and RTN1 (p-value of ≤0.0001) (Table 3 and Figure 7). The SNP located in the MYO5A gene (OAR7_60704536.1) had a 0.74 per measuring unit effect on the trait value of heat tolerance in the studied sheep population (Table 3).
The gene MYO5A belongs to the myosin gene superfamily and is one of the three heavy-chain myosin V genes. It belongs to the family of actin-based motor proteins involved in cytoplasmic vesicle transport and anchoring, as well as spindle-pole alignment and mRNA translocation. This gene produces a protein found in melanocytes and neurons related to melanin-producing cells from the neural crest in the epidermis of the skin (Zhang et al., 2021). Several SNPs within the MYO5A gene have been linked to heat tolerance in cattle (Jia et al., 2019; Rajawat et al., 2022). The link between MYO5A and heat tolerance may be due to its role in pigmentation. PRKG1 (cGMP-dependent protein kinase 1) functions as an essential mediator in the nitric oxide/cGMP signaling pathway and is involved in various signaling activities in different cell types. PRKG1 is known for its association with tick resistance in cattle, as it is one of the genes activated by the immune system (Mapholi et al., 2016; Vajana et al., 2018). In addition, the gene PRKG1 controls blood vessel constriction and smooth muscle contraction, which helps reduce heat loss. The role of PRKG1 in body thermoregulation is essential for adaptation to heat stress and has been reported in horses and cattle (Cardona et al., 2014; Librado et al., 2015). In sheep, it has been reported to be associated with a plateau in the adaptation mechanisms (Yang et al., 2016). GSTCD (Glutathione S-Transferase C-Terminal Domain Containing) is found in various airway cell types and is associated with lung functionality (Liu et al., 2017). The link between genes associated with the respiratory system and heat tolerance in sheep may be because high air temperatures primarily cause heat stress, resulting in low oxygen levels and increased carbon dioxide concentrations (Morrison, 1983). RTN1 is a reticulon encoding gene family member. Reticulons are endoplasmic reticulum-associated organelles that play a role in neuroendocrine secretion and membrane trafficking in neuroendocrine cells and are closely associated with ER stress (Chiurchiu et al., 2014). Tissue hypoxia and angiogenic programs may be activated in response to low oxygen levels in areas of high temperature to compensate for the perceived lack of oxygen and metabolites, resulting in a high rate of ER stress (Binet and Sapieha, 2015; Walczyńska and Sobczyk, 2017).
According to the genetic association between genetic and phenotypic variations, OAR1_18300122.1 (ST3GAL3) had the most positive effect on the heat tolerance trait (Table 3 and Figure 7). ST3GAL3 encodes a membrane protein type II, which catalyzes sialic acid transfer from CMP sialic acid to galactose-containing substrates. Some mutations in this gene affect the development of higher cognitive functions as well as airway inflammation, and its role in animals is still being explored (Smith et al., 2018). SNPs in or near the genes of NTM (OAR21_38037300.1), LTBP1 (OAR3_96465036.1), COL28A1 (s50471.1), LAMA2 (OAR8_58357494.1), and SAMD14 (s20518.1) (Table 3 and Figure 7). Neurotrimin (NTM) is a protein that plays a role in the development of nerves. The presence of NTM in the placenta and adult bovine tissues suggests that it may play a role in early nervous system development (Xu et al., 2018). LTBP1 (Latent Transforming Growth Factor Beta Binding Protein 1) is a protein-coding gene associated with growth traits in sheep breeds (Wang S. et al., 2021). LAMA2 (Laminin Subunit Alpha 2) is a protein-coding gene that has shown some genetic variations associated with reproductive traits, such as the number of lambs weaned (Bolormaa et al., 2017). When studying muscle growth and development in sheep offspring, the protein-coding gene SAMD14 (Sterile Alpha Motif Domain Containing 14) was found to have differential expression (Gauvin et al., 2020).
GWAS analysis identified heat tolerance-related SNPs in genes such as PLCB1, STEAP3, KSR2, UNC13C, PEBP4, and GPAT2 (Table 3 and Figure 7). PLCB1 (Phospholipase C Beta 1) encoded by the protein plays an important role in the intracellular transduction of many extracellular signals. PLCB1 has been associated with environmental factors and tissue-specific expression patterns in sheep and has been identified as a potential selection target in several studies (Li et al., 2020; Wiener et al., 2021). STEAP3 (STEAP3 Metalloreductase) encodes a multipass membrane protein that functions as an iron transporter. According to reports, some members of the STEAP gene family are involved in lipid storage or fat cell regulation and have been linked to fat deposition in sheep tails (Xu et al., 2017; Bakhtiarizadeh and Alamouti, 2020). KSR2 (Kinase Suppressor Of Ras 2), often known as “The Fat Gene,” was identified in a Chr17 selection signature. In humans, KSR2 variants have been demonstrated to play a key role in energy homeostasis and obesity (Pearce et al., 2013). In sheep, genetic variants within the KSR2 gene have been linked to body size and muscle development (Purfield et al., 2017). Gene expression analysis and single-nucleotide polymorphisms in UNC13C (Unc-13 Homolog C) genes linked to the thoracic vertebral number and the immune system in sheep (Mousel et al., 2021; Zhong et al., 2021). PEBP4 (Phosphatidylethanolamine Binding Protein 4) is a member of an evolutionarily conserved family of proteins that perform important biological functions such as lipid binding and inhibition of serine proteases. According to new research, GWAS associations show genetic links between fertility and growth in cattle (Kour et al., 2021). GPAT2 (Mitochondrial Glycerol-3-Phosphate Acyltransferase 2) has been linked to lipid composition because of its role in the biosynthesis of triglycerides and glycerophospholipids (Dircks and Sul, 1997). Genetic variation in the GPAT2 gene region has been associated with indicator traits of carcass composition in Nellore cattle.
5 Conclusion
Expected climatic changes in ambient temperature, which will cause animal heat stress, will affect the breed’s inability to maintain homeothermy (Daramola et al., 2012). Developing countries dependent on livestock production must conserve and prepare their animal genetic resources for future threats (Jombart, 2008). The lack of diversity in the Egyptian sheep breeds studied, which may be due to inbreeding pressure, may result in genetic disorders and decreased animal fitness. The increased use of reproductive technologies such as artificial insemination and the overuse of high-impact rams could be the primary cause of higher levels of inbreeding. GWAS analysis uncovered several potential regions that could be used to study genetic factors controlling sheep adaptation to heat stress. It revealed significant SNPs associated with heat tolerance performance (AHTI) in the genes MYO5A, PRKG1, GSTCD, and RTN1 (p ≤ 0.0001). MYO5A had an effect of 0.74 on the heat tolerance trait in the sheep population studied, which belongs to the actin-based motor protein related to melanin production in the skin. The role of PRKG1 in body thermoregulation is known for adaptation to heat stress in horses, cattle, and sheep with adaptation mechanisms to high mountain environments. GSTCD is associated with the respiratory system, where sheep control heat stress through the respiratory function (Aboulnaga et al., 2021). This may explain why there is such a strong link between these genes and heat tolerance, as they are essential in maintaining the biological system. Gene ontology analysis revealed a strong link between heat tolerance genes involved in lipid storage and fat deposition in sheep tails (STEAP3 and GPAT2) and muscle formation (KSR2). The findings of genes associated with pigmentation and tail fat deposition with heat tolerance in the studied Egyptian breeds could explain why Near East sheep breeds perform better than temperate breeds under heat stress in the prevailing hot, dry areas where they are raised. Furthermore, our findings may provide more robust genetic markers for ongoing and future breeding programs.
Data availability statement
The datasets presented in this study can be found in online repositories. The name of the repository and link to the data can be found below: Zenodo; https://doi.org/10.5281/zenodo.6371316.
Ethics statement
The animal study was reviewed and approved by the Research Evaluation and Ethics Committee, Animal Production Research Institute. Written informed consent was obtained from the owners for the participation of their animals in this study.
Author contributions
AA-N, ME, EA, TAbdlk, TAbdls, and LM contributed to conception and design of the study, collected samples and performed filed experiments. AlA, AH, and AcA organized the database and performed the statistical analysis. AH, and AlA wrote the first draft of the manuscript. All authors contributed to manuscript revision, read, and approved the submitted version”.
Funding
The genomics lab analysis was funded by “Centre de Coopération Internationale en Recherche Agronomique pour le Développement" (CIRAD), within the activity of “PERFORM”, ARIMNET project and L’Agence nationale de la recherche (ANR) organisation, which is highly appreciated.
Acknowledgments
The authors acknowledge the African Supercomputing Center at Mohamed VI Polytechnic University for the supercomputing resources (https://ascc.um6p.ma/) made available for conducting the research reported in this paper. The authors would like to express their gratitude to all of the donors and organisations who contributed to the CGIAR system and helped in funding the CGIAR Research Program on Livestock.
Conflict of interest
The authors declare that the research was conducted in the absence of any commercial or financial relationships that could be construed as a potential conflict of interest.
Publisher’s note
All claims expressed in this article are solely those of the authors and do not necessarily represent those of their affiliated organizations, or those of the publisher, the editors and the reviewers. Any product that may be evaluated in this article, or claim that may be made by its manufacturer, is not guaranteed or endorsed by the publisher.
Supplementary material
The Supplementary Material for this article can be found online at: https://www.frontiersin.org/articles/10.3389/fgene.2022.898522/full#supplementary-material
References
Aboul Naga, A., Khalek, T. A., Osman, M., Elbeltagy, A., Abdel-Aal, E., Abou-Ammo, F., et al. (2021). Physiological and genetic adaptation of desert sheep and goats to heat stress in the arid areas of Egypt. Small Ruminant Res. 203, 106499. doi:10.1016/j.smallrumres.2021.106499
Aboul-Naga, A. (1985). Crossbreeding for fecundity in subtropical sheep. Genet. Reproduction Sheep 55–62.
Aboulnaga, A. M., Abdel Khalek, T., Hayder, M., Hamdon, H., Abozed, G., Abdel Sabour, T., et al. (2021). Adaptive capability of wahati sheep and goats flocks to desert oasis conditions in the new valley of Egypt. Egypt. J. Animal Prod. 58, 71–77. doi:10.21608/ejap.2021.65502.1013
Agapow, P.-M., and Burt, A. (2001). Indices of multilocus linkage disequilibrium. Mol. Ecol. Notes 1, 101–102. doi:10.1046/j.1471-8278.2000.00014.x
Agha, S., Pilla, F., Galal, S., Shaat, I., D’andrea, M., Reale, S., et al. (2008). Genetic diversity in Egyptian and Italian goat breeds measured with microsatellite polymorphism. J. Animal Breed. Genet. 125, 194–200. doi:10.1111/j.1439-0388.2008.00730.x
Azizi, Z., Moradi Shahrbabak, H., and Moradi Shahrbabak, M. (2017). Comparison of pca and dapc methods for analysis of iranian buffalo population structure using snpchip90k data. Iran. J. Animal Sci. 48, 153–161.
Bakhtiarizadeh, M. R., and Alamouti, A. A. (2020). RNA-Seq based genetic variant discovery provides new insights into controlling fat deposition in the tail of sheep. Sci. Rep. 10, 13525–13613. doi:10.1038/s41598-020-70527-8
Binet, F., and Sapieha, P. (2015). Er stress and angiogenesis. Cell. Metab. 22, 560–575. doi:10.1016/j.cmet.2015.07.010
Bolormaa, S., Brown, D., Swan, A., van der Werf, J., Hayes, B., and Daetwyler, H. (2017). Genomic prediction of reproduction traits for merino sheep. Anim. Genet. 48, 338–348. doi:10.1111/age.12541
Brouwer, E. (1965). The energy cost of standing and lying in adult cattle. Publs Eur. Ass. Anim. Prod. 11, 441.
Brown, A., Feldman, M., and Nevo, E. (1980). Multilocus structure of natural populations of hordeum spontaneum. Genetics 96, 523–536. doi:10.1093/genetics/96.2.523
Brzyski, D., Peterson, C. B., Sobczyk, P., Candès, E. J., Bogdan, M., and Sabatti, C. (2017). Controlling the rate of gwas false discoveries. Genetics 205, 61–75. doi:10.1534/genetics.116.193987
Cardona, A., Pagani, L., Antao, T., Lawson, D. J., Eichstaedt, C. A., Yngvadottir, B., et al. (2014). Genome-wide analysis of cold adaptation in indigenous siberian populations. PloS one 9, e98076. doi:10.1371/journal.pone.0098076
Chiurchiu, V., Maccarrone, M., and Orlacchio, A. (2014). The role of reticulons in neurodegenerative diseases. Neuromolecular Med. 16, 3–15. doi:10.1007/s12017-013-8271-9
Cloete, S., Olivier, J., Sandenbergh, L., and Snyman, M. (2014). The adaption of the South Africa sheep industry to new trends in animal breeding and genetics: A review. S. Afr. J. Anim. Sci. 44, 307–321. doi:10.4314/sajas.v44i4.1
Collier, R. J., Baumgard, L. H., Zimbelman, R. B., and Xiao, Y. (2019). Heat stress: Physiology of acclimation and adaptation. Anim. Front. 9, 12–19. doi:10.1093/af/vfy031
Daramola, J. O., Abioja, M. O., and Onagbesan, O. M. (2012). “Heat stress impact on livestock production,” in Environmental stress and amelioration in livestock production (Springer), 53–73.
Dircks, L. K., and Sul, H. S. (1997). Mammalian mitochondrial glycerol-3-phosphate acyltransferase. Biochim. Biophys. Acta 1348, 17–26. doi:10.1016/s0005-2760(97)00106-9
El-Hommosi, F., and Abdel-Hafiz, G. (1982). Reproductive performance of ossimi and saidi sheep under two pre-pubertal planes of nutrition. Assiut Vet. Med. J. 10, 61–66.
El-Wakil, S. I., Shemeis, A., Ahmed, A., and Abdallah, O. (2008). Genetic and phenotypic relationships involving body weight, degree of maturity and measurer of gain rate of barki sheep without having recourse to fitting growth curves. J. Agric. Sci. Mansoura Univ. 33, 4835–4848.
Elshazly, A., and Youngs, C. R. (2019). Feasibility of utilizing advanced reproductive technologies for sheep breeding in Egypt. part 1. genetic and nutritional resources. Egypt. J. Sheep Goats Sci. 14, 39–52.
François, O., and Durand, E. (2010). Spatially explicit bayesian clustering models in population genetics. Mol. Ecol. Resour. 10, 773–784. doi:10.1111/j.1755-0998.2010.02868.x
Frichot, E., and François, O. (2015). Lea: An r package for landscape and ecological association studies. Methods Ecol. Evol. 6, 925–929. doi:10.1111/2041-210x.12382
Gaili, E., Islam, A., and El-Naiem, A. (1994). Comparative lambing performance of najdi, awassi and hejazi ewes. J. Appl. Animal Res. 5, 29–33. doi:10.1080/09712119.1994.9705994
Gain, C., and François, O. (2020). Lea 3: Factor models in population genetics and ecological genomics with r. Mol. Ecol. Resour. 21, 2738–2748. doi:10.1111/1755-0998.13366
Galal, S., Gürsoy, O., and Shaat, I. (2008). Awassi sheep as a genetic resource and efforts for their genetic improvement-a review. Small Ruminant Res. 79, 99–108. doi:10.1016/j.smallrumres.2008.07.018
Gaughan, J., Mader, T. L., Holt, S., and Lisle, A. (2008). A new heat load index for feedlot cattle. J. Anim. Sci. 86, 226–234. doi:10.2527/jas.2007-0305
Gauvin, M. C., Pillai, S. M., Reed, S. A., Stevens, J. R., Hoffman, M. L., Jones, A. K., et al. (2020). Poor maternal nutrition during gestation in sheep alters prenatal muscle growth and development in offspring. J. Anim. Sci. 98, skz388. doi:10.1093/jas/skz388
Gboshe, P., and Osarenakhue, E. (2020). The effect of concentrated feed supplementation regimes on the nutrients digestibility of grasscutters (thryonomys swinderianus) fed pennisetum purpureum as basal feed. Highlights Biosci. 3. doi:10.36462/h.biosci.20214
Ge, S. X., Jung, D., and Yao, R. (2020). Shinygo: A graphical gene-set enrichment tool for animals and plants. Bioinformatics 36, 2628–2629. doi:10.1093/bioinformatics/btz931
Ghoreishifar, S. M., Moradi-Shahrbabak, H., Parna, N., Davoudi, P., and Khansefid, M. (2019). Linkage disequilibrium and within-breed genetic diversity in iranian zandi sheep. Arch. Anim. Breed. 62, 143–151. doi:10.5194/aab-62-143-2019
Girish, K., and Dubey, S. (2018). Eukaryotic molecular biology databases: An overview. Highlights Biosci. 1, 1–7. doi:10.36462/h.biosci.20184
Godde, C., Mason-D’Croz, D., Mayberry, D., Thornton, P. K., and Herrero, M. (2021). Impacts of climate change on the livestock food supply chain; a review of the evidence. Glob. Food Sec. 28, 100488. doi:10.1016/j.gfs.2020.100488
Gonzalez-Rivas, P. A., Chauhan, S. S., Ha, M., Fegan, N., Dunshea, F. R., and Warner, R. D. (2020). Effects of heat stress on animal physiology, metabolism, and meat quality: A review. Meat Sci. 162, 108025. doi:10.1016/j.meatsci.2019.108025
Hahn, G., Mader, T., and Eigenberg, R. (2003). Perspective on development of thermal indices for animal studies and management. EAAP Tech. Ser. 7, 31–44.
Haile, A., Gizaw, S., Getachew, T., Mueller, J. P., Amer, P., Rekik, M., et al. (2019). Community-based breeding programmes are a viable solution for ethiopian small ruminant genetic improvement but require public and private investments. J. Animal Breed. Genet. 136, 319–328. doi:10.1111/jbg.12401
Harris, A. M., and DeGiorgio, M. (2017). An unbiased estimator of gene diversity with improved variance for samples containing related and inbred individuals of any ploidy. G3 7, 671–691. doi:10.1534/g3.116.037168
Jia, P., Cai, C., Qu, K., Chen, N., Jia, Y., Hanif, Q., et al. (2019). Four novel snps of myo1a gene associated with heat-tolerance in Chinese cattle. Animals. 9, 964. doi:10.3390/ani9110964
Jombart, T. (2008). adegenet: a r package for the multivariate analysis of genetic markers. Bioinformatics 24, 1403–1405. doi:10.1093/bioinformatics/btn129
Jombart, T., and Collins, C. (2015). A tutorial for discriminant analysis of principal components (dapc) using adegenet 2.0. 0. London: Imperial College London, MRC Centre for Outbreak Analysis and Modelling.
Kamvar, Z. N., Tabima, J. F., and Gr"unwald, N. J. (2014). Poppr: An r package for genetic analysis of populations with clonal, partially clonal, and/or sexual reproduction. PeerJ 2, e281. doi:10.7717/peerj.281
Kang, H. M., Zaitlen, N. A., Wade, C. M., Kirby, A., Heckerman, D., Daly, M. J., et al. (2008). Efficient control of population structure in model organism association mapping. Genetics 178, 1709–1723. doi:10.1534/genetics.107.080101
Kour, A., Deb, S. M., Nayee, N., Niranjan, S. K., Raina, V. S., Mukherjee, A., et al. (2021). Novel insights into genome-wide associations in bos indicus reveal genetic linkages between fertility and growth. Anim. Biotechnol., 1–17. doi:10.1080/10495398.2021.1932520
Krupin, E. (2021). Heat stress assessment based on analysis of external environmental factors and microclimate in livestock housing facilities. Russ. Agric. Sci. 47, 425–429. doi:10.3103/s1068367421040108
Letunic, I., and Bork, P. (2019). Interactive tree of life (itol) v4: Recent updates and new developments. Nucleic Acids Res. 47, W256-W259–W259. doi:10.1093/nar/gkz239
Li, R., Li, C., Chen, H., Li, R., Chong, Q., Xiao, H., et al. (2020). Genome-wide scan of selection signatures in dehong humped cattle for heat tolerance and disease resistance. Anim. Genet. 51, 292–299. doi:10.1111/age.12896
Librado, P., Der Sarkissian, C., Ermini, L., Schubert, M., Jónsson, H., Albrechtsen, A., et al. (2015). Tracking the origins of yakutian horses and the genetic basis for their fast adaptation to subarctic environments. Proc. Natl. Acad. Sci. U. S. A. 112, E6889–E6897. doi:10.1073/pnas.1513696112
Liu, B., Azimi, S., Henry, A., Miller, S., Billington, C., Lee, J., et al. (2017). “Gstcd knockout mice show a decreased contraction of small airways following lps treatment,” in B30. Mechanisms of airway smooth muscle contractility and relaxation: Potential therapeutic targets (American Thoracic Society), A3157.
Mapholi, N. O., Maiwashe, A., Matika, O., Riggio, V., Bishop, S., MacNeil, M., et al. (2016). Genome-wide association study of tick resistance in south african nguni cattle. Ticks Tick. Borne. Dis. 7, 487–497. doi:10.1016/j.ttbdis.2016.02.005
Monau, P., Raphaka, K., Zvinorova-Chimboza, P., and Gondwe, T. (2020). Sustainable utilization of indigenous goats in southern Africa. Diversity 12, 20. doi:10.3390/d12010020
Morrison, S. (1983). Ruminant heat stress: Effect on production and means of alleviation. J. Anim. Sci. 57, 1594–1600. doi:10.2527/jas1983.5761594x
Mousel, M. R., White, S. N., Herndon, M. K., Herndon, D. R., Taylor, J. B., Becker, G. M., et al. (2021). Genes involved in immune, gene translation and chromatin organization pathways associated with mycoplasma ovipneumoniae presence in nasal secretions of domestic sheep. Plos one 16, e0247209. doi:10.1371/journal.pone.0247209
Nei, M. (1978). Estimation of average heterozygosity and genetic distance from a small number of individuals. Genetics 89, 583–590. doi:10.1093/genetics/89.3.583
Othman, O. E. M., Payet-Duprat, N., Harkat, S., Laoun, A., Maftah, A., Lafri, M., et al. (2016). Sheep diversity of five Egyptian breeds: Genetic proximity revealed between desert breeds. Small Ruminant Res. 144, 346–352. doi:10.1016/j.smallrumres.2016.10.020
Paradis, E., Claude, J., and Strimmer, K. (2004). Ape: Analyses of phylogenetics and evolution in r language. Bioinformatics 20, 289–290. doi:10.1093/bioinformatics/btg412
Pearce, L. R., Atanassova, N., Banton, M. C., Bottomley, B., van der Klaauw, A. A., Revelli, J.-P., et al. (2013). Ksr2 mutations are associated with obesity, insulin resistance, and impaired cellular fuel oxidation. Cell. 155, 765–777. doi:10.1016/j.cell.2013.09.058
Pilling, D., Bélanger, J., and Hoffmann, I. (2020). Declining biodiversity for food and agriculture needs urgent global action. Nat. Food 1, 144–147. doi:10.1038/s43016-020-0040-y
Purfield, D. C., McParland, S., Wall, E., and Berry, D. P. (2017). The distribution of runs of homozygosity and selection signatures in six commercial meat sheep breeds. PLoS One 12, e0176780. doi:10.1371/journal.pone.0176780
Rahimi, J., Mutua, J. Y., Notenbaert, A. M., Marshall, K., and Butterbach-Bahl, K. (2021). Heat stress will detrimentally impact future livestock production in east Africa. Nat. Food 2, 88–96. doi:10.1038/s43016-021-00226-8
Rajawat, D., Panigrahi, M., Kumar, H., Nayak, S. S., Parida, S., Bhushan, B., et al. (2022). Identification of important genomic footprints using eight different selection signature statistics in domestic cattle breeds. Gene 816, 146165. doi:10.1016/j.gene.2021.146165
Sejian, V., Bhatta, R., Gaughan, J., Dunshea, F., and Lacetera, N. (2018). Review: Adaptation of animals to heat stress. Animal 12, s431–s444. doi:10.1017/S1751731118001945
Shebaita, M., and El-Banna, I. (1982). “Heat load and heat dissipation in sheep and goats under environmental heat stress,” in Proceedings of the sixth international conference on animal and poultry production, held at university of zagazig, zagazig, Egypt, 21-23 september 1982/Egyptian society of animal production (Zagazig, Egypt: The Society), 459–469.
Smith, A. D., Panickar, K. S., Urban, J. F., and Dawson, H. D. (2018). Impact of micronutrients on the immune response of animals. Annu. Rev. Anim. Biosci. 6, 227–254. doi:10.1146/annurev-animal-022516-022914
Talaat Bashandy, A. H., Solma, M., Kassab, A., and Hamdon, H. (2020). Molecular evaluation of three populations of farafra sheep in comparison to ossimi and rahmani sheep breeds. Acta Univ. Agric. Silvic. Mendel. Brun. 68, 929–936. doi:10.11118/actaun202068060929
Vajana, E., Barbato, M., Colli, L., Milanesi, M., Rochat, E., Fabrizi, E., et al. (2018). Combining landscape genomics and ecological modelling to investigate local adaptation of indigenous ugandan cattle to East Coast fever. Front. Genet. 9, 385. doi:10.3389/fgene.2018.00385
van Rossum, B.-J., Kruijer, W., van Eeuwijk, F., Boer, M., Malosetti, M., Bustos-Korts, D., et al. (2020). Package ’statgengwas’.
VanRaden, P. M. (2008). Efficient methods to compute genomic predictions. J. Dairy Sci. 91, 4414–4423. doi:10.3168/jds.2007-0980
Walczyńska, A., and Sobczyk, Ł. (2017). The underestimated role of temperature–oxygen relationship in large-scale studies on size-to-temperature response. Ecol. Evol. 7, 7434–7441. doi:10.1002/ece3.3263
Wang, S., Yi, X., Wu, M., Zhao, H., Liu, S., Pan, Y., et al. (2021a). Detection of key gene indels in tgf-β pathway and its relationship with growth traits in four sheep breeds. Anim. Biotechnol. 32, 194–204. doi:10.1080/10495398.2019.1675682
Wang, Z.-H., Zhu, Q.-H., Li, X., Zhu, J.-W., Tian, D.-M., Zhang, S.-S., et al. (2021b). isheep: an integrated resource for sheep genome, variant and phenotype. Front. Genet. 12, 714852. doi:10.3389/fgene.2021.714852
Weir, B. S., and Cockerham, C. C. (1984). Estimating f-statistics for the analysis of population structure. evolution 38, 1358–1370. doi:10.1111/j.1558-5646.1984.tb05657.x
West, J. W. (2003). Effects of heat-stress on production in dairy cattle. J. Dairy Sci. 86, 2131–2144. doi:10.3168/jds.S0022-0302(03)73803-X
Wiener, P., Robert, C., Ahbara, A., Salavati, M., Abebe, A., Kebede, A., et al. (2021). Whole-genome sequence data suggest environmental adaptation of ethiopian sheep populations. Genome Biol. Evol. 13, evab014. doi:10.1093/gbe/evab014
Xu, D., Li, J., Zhang, C., Chen, W., Li, D., Li, S., et al. (2018). The analysis of splice variants and genomic imprinting status of ntm gene in cattle (bos taurus). J. Agric. Biotechnol. 26, 1707–1713.
Xu, S.-S., Ren, X., Yang, G.-L., Xie, X.-L., Zhao, Y.-X., Zhang, M., et al. (2017). Genome-wide association analysis identifies the genetic basis of fat deposition in the tails of sheep (ovis aries). Anim. Genet. 48, 560–569. doi:10.1111/age.12572
Yang, J., Li, W.-R., Lv, F.-H., He, S.-G., Tian, S.-L., Peng, W.-F., et al. (2016). Whole-genome sequencing of native sheep provides insights into rapid adaptations to extreme environments. Mol. Biol. Evol. 33, 2576–2592. doi:10.1093/molbev/msw129
Yousef, M., and Dill, D. (1969). Resting energy metabolism and cardiorespiratory activity in the burro equus asinus. J. Appl. Physiol. 27, 229–232. doi:10.1152/jappl.1969.27.2.229
Yuan, K., Zhou, Y., Ni, X., Wang, Y., Liu, C., and Xu, S. (2017). Models, methods and tools for ancestry inference and admixture analysis. Quant. Biol. 5, 236–250. doi:10.1007/s40484-017-0117-2
Zhang, H., Wu, Z., Yang, L., Zhang, Z., Chen, H., and Ren, J. (2021). Novel mutations in the myo5a gene cause a dilute coat color phenotype in mice. FASEB J. 35, e21261. doi:10.1096/fj.201903141RR
Keywords: sheep, dry areas, Egypt, GWAS, SNP genotyping, heat tolerance
Citation: Aboul-Naga AM, Alsamman AM, El Allali A, Elshafie MH, Abdelal ES, Abdelkhalek TM, Abdelsabour TH, Mohamed LG and Hamwieh A (2022) Genome-wide analysis identified candidate variants and genes associated with heat stress adaptation in Egyptian sheep breeds. Front. Genet. 13:898522. doi: 10.3389/fgene.2022.898522
Received: 17 March 2022; Accepted: 05 September 2022;
Published: 03 October 2022.
Edited by:
Joanna Szyda, Wroclaw University of Environmental and Life Sciences, PolandReviewed by:
Mohsen Gholizadeh, Sari Agricultural Sciences and Natural Resources University, IranSimon Frederick Lashmar, University of Pretoria, South Africa
Copyright © 2022 Aboul-Naga, Alsamman, El Allali, Elshafie, Abdelal, Abdelkhalek, Abdelsabour, Mohamed and Hamwieh. This is an open-access article distributed under the terms of the Creative Commons Attribution License (CC BY). The use, distribution or reproduction in other forums is permitted, provided the original author(s) and the copyright owner(s) are credited and that the original publication in this journal is cited, in accordance with accepted academic practice. No use, distribution or reproduction is permitted which does not comply with these terms.
*Correspondence: Adel M. Aboul-Naga, YWRlbG1hYm91bG5hZ2FAZ21haWwuY29t; Alsamman M. Alsamman, c21haG1vdWRAYWdlcmkuc2NpLmVn