- 1Department of Molecular Medicine, University of Pavia, Pavia, Italy
- 2Department of Medicine and Surgery, University of Insubria, Varese, Italy
- 3Istituto di Ricerca Genetica e Biomedica, CNR, Milano, Italy
- 4Pediatric Hematology/Oncology, Fondazione IRCCS Policlinico S, Matteo, Pavia, Italy
- 5Centro Fibrosi Cistica, Azienda Ospedaliera Universitaria Integrata Verona, Verona, Italy
Background: Shwachman–Diamond syndrome (SDS) is a rare autosomal recessive ribosomopathy mainly characterized by exocrine pancreatic insufficiency, skeletal alterations, neutropenia, and a relevant risk of hematological transformation. At least 90% of SDS patients have pathogenic variants in SBDS, the first gene associated with the disease with very low allelic heterogeneity; three variants, derived from events of genetic conversion between SBDS and its pseudogene, SBDSP1, provided the alleles observed in about 62% of SDS patients.
Methods: We performed a reanalysis of the available WES files of a group of SDS patients with biallelic SBDS pathogenic variants, studying the results by next bioinformatic and protein structural analysis. Parallelly, careful clinical attention was given to the patient focused in this study.
Results: We found and confirmed in one SDS patient a germline heterozygous missense variant (c.100T>C; p.Phe34Leu) in the EIF6 gene. This variant, inherited from his mother, has a very low frequency, and it is predicted as pathogenic, according to several in silico prediction tools. The protein structural analysis also envisages the variant could reduce the binding to the nascent 60S ribosomal.
Conclusion: This study focused on the hypothesis that the EIF6 germline variant mimics the effect of somatic deletions of chromosome 20, always including the locus of this gene, and similarly may rescue the ribosomal stress and ribosomal dysfunction due to SBDS mutations. It is likely that this rescue may contribute to the stable and not severe hematological status of the proband, but a definite answer on the role of this EIF6 variant can be obtained only by adding a functional layer of evidence. In the future, these results are likely to be useful for selected cases in personalized medicine and therapy.
Introduction
Shwachman–Diamond syndrome (SDS) is a rare, autosomal recessive disease (OMIM #260400) included among inherited bone marrow failure syndrome (IBMFS). It is primarily characterized by neutropenia, exocrine pancreatic insufficiency, skeletal alterations, and an increased risk to develop hematological malignancies, including myelodysplastic syndrome (MDS) and acute myeloid leukemia (AML) (Dror et al., 2011; Nelson and Myers, 2018). The disease-causing gene is SBDS, localized at 7q11.21 (Boocock et al., 2003), and a large majority of patients carry biallelic pathogenic variants in SBDS, the detection of which confirms the clinical diagnosis. Typically, mutation analysis of exon 2 identifies, in at least 90% of SDS patients, one of three common mutations, (c.183_184delTAinsCT, c.258+2T>C, and c.258+2T>C in addition to c.183_184delTAinsCT in the same allele). Two of these common mutations are observed concomitantly in approximately 62% of SDS patients (Nelson and Myers, 2018).
The approach of whole-exome sequencing (WES) has disclosed genetic heterogeneity for SDS. Indeed, biallelic mutations in DNAJ homolog subfamily C member 21 (DNAJC21) (Tummala et al., 2016; Dhanraj et al., 2017), in GTPase Elongation Factor-like (EFL1) (Stepensky et al., 2017; Tan et al., 2018; Tan et al., 2019; Lee et al., 2021), or heterozygous mutations in the 54-kDa signal recognition particle (SRP54) (Carapito et al., 2017) have been reported in association with SDS or SDS-like phenotype in some patients.
When SRP54 is involved in the co-translation protein-targeting pathway, the other genes code for proteins involved in the ribosome biogenesis pathway as SBDS: 1) DNAJC21 is involved in the pre-rRNA processing, and it is implicated in the late 60S maturation in the cytoplasm; 2) EFL1 interacts with SBDS to evict the anti-association factor eIF6 from the ribosomal subunit 60S in the final step of ribosome maturation (Weis et al., 2015). The removal of eIF6 from the 60S subunit allows the 80S ribosome assembly (Ceci et al., 2003).
In SDS, because of the loss of SBDS activity, eIF6 remains bound to the 60S subunit and prevents its joining to the 40S subunit, resulting in decreased ribosomal subunit joining and reduced translation efficiency. The impairment of ribosome biogenesis links SDS to the group of bone marrow failure disorders, called ribosomopathies, associated with mutations in genes related to ribosomal biogenesis and function. Ribosomopathies are characterized by ribosome dysfunction producing marrow failure and cancer predisposition (De Keersmaecker et al., 2015).
Focusing on the SDS predisposition to hematological malignancies, a growing interest in eIF6 and its role in ribosome maturation prompted two different types of studies. The first concerns the interstitial deletion of the long arm of chromosome 20, del(20)(q), identified by periodic bone marrow monitoring of SDS patients; this clonal abnormality always includes the loss of the EIF6 locus (Pressato et al., 2015). Clinical follow-up of SDS patients showed that del(20)(q), without additional clonal chromosomal abnormalities, correlates with a lower risk of developing MDS and/or AML, suggesting that the loss of one copy of EIF6 gene restores ribosome homeostasis in the context of reduced SBDS activity (Valli et al., 2013; Khan et al., 2020; Khan et al., 2021). The del(20)(q) has also been associated with an increased number of burst-forming unit-erythroid (BFU-E) colonies in cases of paternal deletion (Nacci et al., 2017).
The second study monitored the mutational status on multiple bone marrow samples of patients with SDS. By using NGS sequencing, recent research demonstrated the frequent development of hematopoietic clones carrying a heterozygous mutation in EIF6 and TP53 genes. Functional studies observed the inactivation of eIF6 and the corresponding improvement of the less effective SDS translation, supporting the hypothesis of improved clone fitness (Kennedy et al., 2021; Tan et al., 2021).
All these studies unveiled that SDS represents another genetic disease in which a somatic rescue mechanism occurs, either through a del(20)(q), always encompassing the EIF6 locus, or through EIF6 heterozygous variants.
As a part of a larger study of whole-exome sequencing (WES) on SDS patients carrying biallelic causative SBDS pathogenic variants, aimed to understand if other germline variants could explain the large clinical variability among the patients (Morini et al., 2019), we identified a single case carrying a heterozygous germline variant (c.100T>C, p.Phe34Leu) in EIF. We postulate, with strong support from structural data, that this variant impairs protein activity, thus reducing the ribosomal dysfunction, similarly to deletions of chromosome 20, and, likely, resulting in a milder and stable hematological picture in the reported patient.
Materials and Methods
Case Report
Our patient, a 23-year-old male (born in 1998), identified as UPN 2 (unique patient number) in the previous work by our group (Valli et al., 2019), is the second child of healthy non-consanguineous Italian parents. UPN 2 was diagnosed as affected by SDS in 2001 based on the presence of neutropenia and pancreatic insufficiency.
Since diagnosis, an annual follow-up has been performed at the pediatric onco-hematology unit. Over the last 10 years, the patient has maintained a stable hematological picture.
At the latest clinical evaluation (2021), his height was 155.0 cm, his weight 50.0 kg, and his chest circumference 77.5 cm, all at 25–30 centiles of height and weight distribution of SDS male/female patients (personal communication by M. Cipolli and G. Tridello). At the same time, no pathological changes were observed in both lower limbs, and normal bone density was reported. Similarly, no structural changes in the thoracic cage were present. His school performance was low average. At the same clinical evaluation of 2021, hemoglobin and RBC counts were normal (14.9, normal value, nv: 13.2–17.3 g/dl and 4.35 × 10^6 per µl, nv 4.30–5.70 × 10^6 per µl, respectively). The neutrophil count was low (0.9 × 10^3, nv: 2.0–8.0 × 10^3 per µl, and ranging between 0.5 and 0.7 × 10^3 per µl in previous tests), lymphocyte count was normal (1.8 × 10^3µL, nv: 1.5–4.0 × 10^3 per µl), and platelets were slightly reduced (120 × 10^3 per µl, nv: 150–400 × 10^3 per µl, ranging between 100 and 130 × 10^3 per µl in previous tests). Bone marrow biopsy confirmed, as the previous controls, a stable cellularity (20%), and immunostaining with anti-p53 antibody was consistently negative. No evidence of any change suggesting myelodysplasia was reported.
Routine biochemical tests were within normal limits; serum amylase and pancreatic isoamylase were low, 14.0 mU/ml and 5.0 mU/ml, respectively (nv: 25.0–125.0 mU/ml and 8.0–53.0 mU/ml, respectively). Serum lipase was within normal limits: 36.0 mU/ml (nv: 8.0–58.0 mU/ml). Creon tablets as a treatment for the exocrine pancreatic insufficiency were prescribed. S-Parathyroid hormone (PTH) levels were slightly elevated during the last two checks, corresponding to 112.3 and 84.5 pg/ml values (nv: 12,0–72,0 pg/ml). Vitamin D, calcium, and potassium were at the normal levels. The patient had received his third dose of COVID-19 vaccine without any major complication.
Similarly, a recent survey carried out by an American SDS Registry, confirmed no worrying clinical effect in three SDS patients who have been vaccinated against COVID-19 (Galletta et al., 2022).
Cytogenetics
Repeated conventional and molecular cytogenetic analyses were performed on bone marrow yearly since 2002, almost regularly, up to 2021. The methods used included routine cytogenetics, Fluorescent In Situ Hybridization (FISH) on metaphases and nuclei with informative probes and libraries, and array-based Comparative Genomic Hybridization (a-CGH), according to the methods reported in Pressato et al. (2015).
Molecular and Bioinformatics Investigations
In 2015, we performed WES on 16 DNA samples obtained from blood samples of SDS patients after their acceptance to the project. Samples eligible for WES held as key features a concentration of 50 ng/ul, a ratio 260/280λ and 260/230λ, respectively, at least equal to 1.8 and 1.8–2.1, and a good integrity evaluated by agarose gel electrophoresis. Sequencing libraries of the samples were prepared using the exome enrichment kit Agilent SureSelectXT Human All Exon V5+UTRs. WES was performed on the HiSeq 1,000 (Illumina, 2 bp × 100 bp). The processing of sequencing data was based on the ISAAC pipeline (Raczy et al., 2013); for data alignment and for variants calling, we used, respectively, the ISAAC aligner and ISAAC variant caller. From the samples alignment we obtained a mean coverage of 65% and a coverage >20X corresponding to 91% of the complete exome sequences. For the annotation and the analysis of the variants in the variant call format (VCF) files, generated by WES for each sample, the expert variant interpreter (eVai) platform (https://www.engenome.com/evai/) (Nicora et al., 2022) was used.
We confirmed the relevant variants by Sanger sequencing. The primers used for the amplification and sequencing were designed using Primer3web (https://primer3.ut.ee/). PCRs were performed in 25 μL of reaction volume containing 50 ng of genomic DNA, 0.5 μm primers, 2 μl dNTP Mixture (2.5 mm each), 2.5 μl 5X PrimeSTAR GXL Buffer, and 1.25U PrimeSTAR GXL DNA Polymerase (TAKARA BIO INC.). PCR products were checked by 2% agarose gel electrophoresis and underwent clean-up prior to sequencing using the Applied 3500 DX Genetic Analyzer sequencer with BrilliantDye Terminator Cycle sequencing kit (NimaGen). The sequencing data were analyzed by Finch TV software.
Structural Analysis
For structural visualization and evaluation of the effect of the Phe34Leu mutation, the model of eIF6 was extracted from PDB code 6LSS (pre-60 ribosome). This model had originally been generated by SWISS-MODEL (Waterhouse et al., 2018) and docked into the electron density of human pre-60S ribosomal particles by rigid-body fitting, followed by extensive manual rebuilding in COOT with a final local resolution comprised between 3.1 and 4.8 Å (Liang et al., 2020).
Results
Cytogenetic Findings
Bone marrow karyotype analysis was normal up to 2007; then, from 2008 to 2010 a small clone was detected carrying an isochromosome for the long arm of chromosome 7, i(7)(q10), which is one of the most frequent clonal chromosome anomalies in the bone marrow of SDS patients: the abnormal cells ranged from 1.7 to 7.6%, according to different methods (conventional cytogenetics or FISH with different probes) or different times of analysis. On DNA from a sample with 2.9% of cells with the i(7)(q10), a-CGH was performed; it did not reveal the chromosome 7 abnormality due to the small size of the clone, nor any other abnormality. The i(7)(q10) clone then disappeared, and was not found in any subsequent analysis from 2011 to 2020; no structural abnormality of chromosome 20 was ever identified.
Molecular and Bioinformatics Analysis
The clinical diagnosis of SDS was confirmed by the presence of one of the common mutations [c.258+2T>C, p.Cys84fs3, paternal] and a second variant [c.356G>A, p.Cys119Tyr, maternal] in SBDS. The latter was included by Finch et al. (2011) among disease-associated missense variants since, on the basis of functional studies, it was evaluated as a mutation affecting SBDS protein stability.
When reviewing our WES file of UPN2 using the eVai platform, we first filtered the results searching for variants in other genes related to SDS or SDS-like phenotypes, DNAJC21, EFL1, and SRP54, no relevant variants were found. Then, checking for variants in EIF6 gene, we found a heterozygous single nucleotide substitution causing a missense change (c.100T>C; p.Phe34Leu). Sanger sequencing confirmed the variant for the patient and assessed its maternal origin (Figure 1A). According to the Genome Aggregation Database (gnomAD), the variant is a rare one as its frequency is 0.0002002.
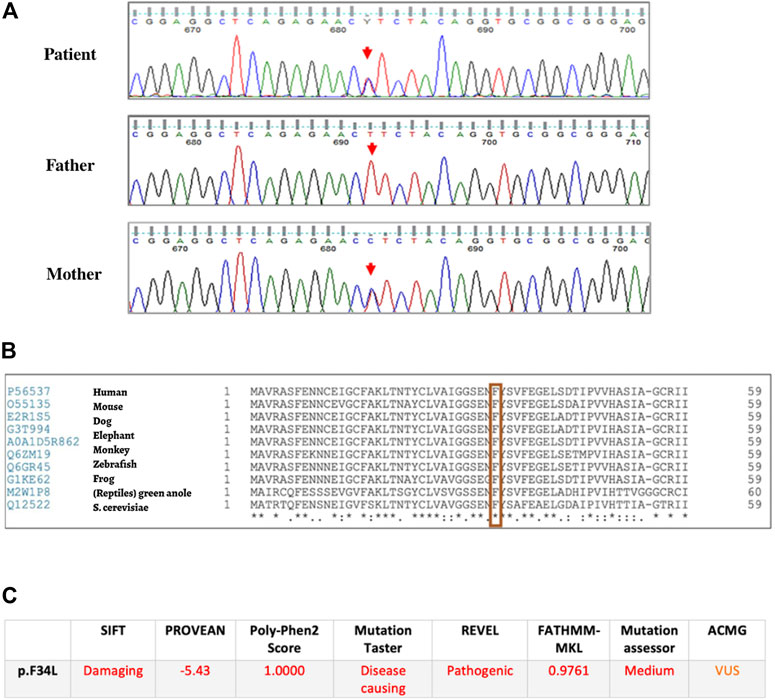
FIGURE 1. Sequencing confirmation of the EIF6 variant. (A) Part of electropherogram from the proband and their parents, showing the substitution T>C in the heterozygous status at the position c.100 maternal inherited; (B) protein sequence alignment using UniProt shows absolute conservation of F34 throughout different species (human, mouse, dog, elephant, monkey, zebrafish, frog, reptiles, and S. cerevisiae); (C) in silico analysis of the p.Phe43Leu variant using different prediction tools.
These results were obtained in DNA from a blood sample, but we checked the variant in DNAs also from eight bone marrow specimens obtained at regular follow-ups (the latest one in 2021): all of these showed the stable presence of the heterozygous variant, with a ratio 1:1 to normal allele.
Protein sequence alignment using UniProt (www.uniprot.org) showed that the amino acid Phenylalanine in position 34 is highly conserved among different species such as human, mouse, dog, elephant, monkey, zebrafish, frog, reptiles, and S. cerevisiae (Figure 1B). The variant functional predictions are entered in Figure 1C.
We then filtered the WES results for the presence of variants in genes related to the development of MDS or leukemia, as reported by several authors (Lindsley, 2017; Steensma, 2017; Armstrong et al., 2018; Bluteau et al., 2018; Obrochta and Godley, 2018; Kennedy et al., 2021). The variants with a frequency ≤0.01 were validated by Sanger sequencing in the patient and his parents. These variants are entered in Table1 with their genome position, amino acid change, zygosity, allele frequency, and pathogenicity prediction, according to various specific tools and parental origin.
Structural Analysis
The protein eIF6 consists of five quasi-identical alpha/beta subdomains arrayed about a five-fold axis of pseudosymmetry (Figure 2A). Residue F34 (blue sticks) is the core part of a chain of hydrophobic interactions mediated by a cluster of five aromatic residues (F7, F16, F34, Y35, and F38) (pink sticks) belonging to the N-terminal domain and one, F205, belonging to the C-terminal domain (Figure 2B). This residue arrangement, involving helix 2A and β-strand 2E, where the group of four residues, F34, Y35, F38, and F205, are located, respectively (nomenclature according to (Groft et al., 2000), contributes to stabilize the “velcro” strategy adopted in the pentein fold of eIF6 (Fülöp and Jones, 1999), where β-strand 2E at the C-terminus hydrogen bonds with a β-strand from the N-terminal subdomain. The flat protein surfaces, thus, formed are necessary for interaction with ribosomal protein L23, a component of the 60S subunit, on one side, and with GTPBP4 (nucleolar GTP-binding protein 1), also involved in the 60S biogenesis, on the other (Figures 2C,D). The F34L variant lacks the central phenylalanine of the cluster, interrupts this regular pattern and introduces clashes, represented by solid red disks in Figures 2E,F, where both leucine rotamers are considered. Despite the similar hydrophobic nature of the two residues, the reduction of the side chain size and the interruption of the aromatic stack explain why analysis by I-mutant predicts a Gibbs free energy difference (ΔΔG) between the mutant and the wild-type of −0.87 kcal/mol. This result is evidence of a large decrease in protein stability. We can, therefore, envisage that the F34L variant could impair eIF6 folding, with a reduction in binding to the nascent 60S ribosomal subunit through protein L23 and a possible consequent reduction in binding of protein GTPBP4, which is also involved in ribosomal biogenesis.
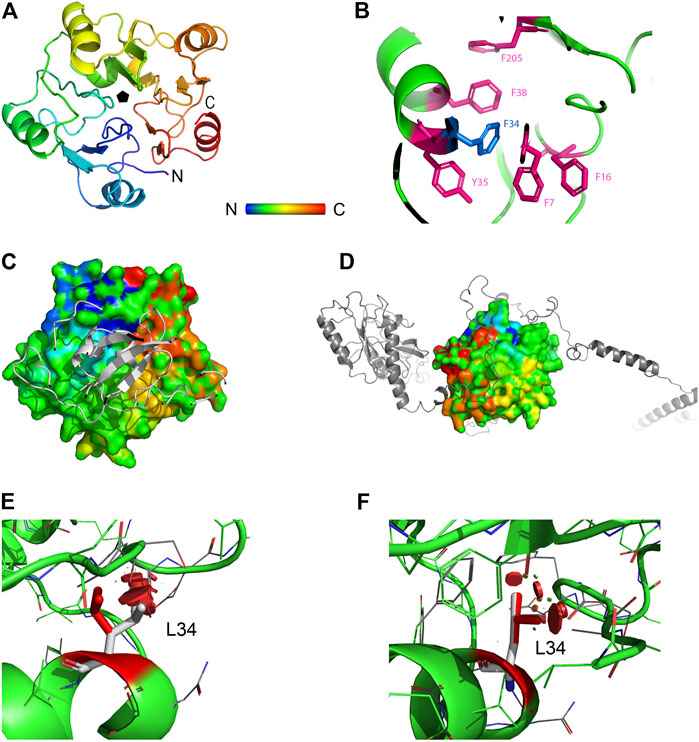
FIGURE 2. Structural analysis of eIF6. (A) Rainbow-colored (blue: N-terminus, red: C-terminus) ribbon representation of eIF6, consisting of five quasi-identical alpha/beta subdomains arrayed about a five-fold axis of pseudosymmetry. (B) Residue F34 (blue sticks) is the core part of a chain of hydrophobic interactions mediated by a cluster of five aromatic residues (F7, F16, F34, F38, and Y35) belonging to the N-terminal domain and one, F205, belonging to the C-terminal domain (pink sticks). (C) Surface representation of eIF6 interacting with ribosomal protein L23 (gray ribbon). (D) Surface representation of eIF6 interacting with ribosomal protein GTPBP4 (gray ribbon). (E,F) Effect of the F34L mutation: red solid disks represent clashes between the two rotamers of L34 and neighboring residues.
Discussion
Three main features characterize SDS: bone marrow failure, pancreatic exocrine dysfunction, and skeletal abnormalities (Dror et al., 2011). In addition, a significant percentage of SDS patients may develop serious hematological complications, requiring, in some cases, hematopoietic stem cell transplantation, a procedure associated with a requirement for specific protocols for this disease (Donadieu et al., 2012). A challenge for the surveillance of the disease is the early identification of risk factors relevant to the evolution of the hematological picture (Furutani et al., 2022). An extensive study on a French cohort of 102 patients postulated that the combination of a young age at diagnosis with low values of specific hematologic parameters, at the time of diagnosis and later constitutes a negative prognostic factor. This combination was a risk factor stronger than any of the other nine single variables taken into consideration alone, as transient cytopenia, low value of neutrophils, hemoglobin, platelets, or SBDS genotype. The latter was confirmed not to be linked to significant differences in hematologic parameters and other nonhematologic features, both in patients harboring two common mutations or in patients with one common and one rare mutation (Donadieu et al., 2012).
Although the risk for developing MDS and AML is not related to the type of SBDS mutations, it can be favorably modified by other genetic factors as the presence of specific chromosomal abnormalities, in particular, the i(7)(q10) and del(20)(q) if they are the only abnormality present (Minelli et al., 2009; Khan et al., 2021).
When del(20)(q) is considered, cytogenetic studies, made over a long period of time on the SDS Italian cohort, confirmed the benign prognostic significance of this abnormality. Indeed, the patients carrying del(20)(q) consistently showed a mild hypoplastic bone marrow picture, no or mild neutropenia, anemia, and thrombocytopenia (Pressato et al., 2012; Valli et al., 2019).
In all cases, del(20)(q) includes the loss of the EIF6 locus and, consequently, in the bone marrow clones with the chromosomal abnormality, only one allele expressing EIF6 is present in the bone marrow clones with the chromosomal abnormality. This condition has been studied in vivo on mice demonstrating that knockout heterozygous animals presented reduced eIF6 levels as expected, while preserving sufficient nucleolar eIF6 and regular ribosome biogenesis. In the liver cells, a larger amount of 80S in polysomal profiles suggested a lower efficiency in initiation of translation (Gandin et al., 2008).
Common events in SDS patients are the development of many somatic clones in the bone marrow with variants in either EIF6 or TP53 genes, producing different effects: i) variants in EIF6 have a compensatory role on the impact of SBDS deficiency, in turn reducing the risk of developing MDS and AML by improving the ribosome maturation, enhancing translation, and reducing p53 upregulation (Kennedy et al., 2021), ii) variants in TP53 act in a different pathway and decrease tumor suppressor checkpoint activation. Clones acquiring only variants in both TP53 alleles are associated with the development of hematological malignancies (Kennedy et al., 2021).
Furthermore, higher p53 protein levels, linked to inhibition of osteogenesis, have been found in SDS patient’s osteoblasts, in correlation with the skeletal anomalies of patients (Frattini et al., 2021).
Relevantly, the EIF6 clonal variants in SDS patients were not observed in the presence of leukemic evolution or serious bone marrow dysfunction, confirming a positive impact on the hematological alterations determined by the SBDS genotype (Kennedy et al., 2021).
The results on humans fit the yeast model proposed by Menne et al. (2007) who demonstrated that missense variants in Tif6 can bypass the fitness defect resulting from a lack of Sdo1 through the reduction of Tif6 binding to the 60S ribosome subunit.
Here, we report a patient, UPN 2, in whom we found a heterozygous germline variant, c.T>C (p.Phe34Leu), in EIF6. This variant is rare according to gnomAD and the amino acid Phe34 is extensively conserved (Figure 1B). To the best of our knowledge, this is the first patient in whom a constitutional pathogenic EIF6 variant is associated with biallelic SBDS mutations. In UPN 2, the missense variant in EIF6 is a germline variant thus, at least in theory, its effect in ameliorating the consequences of SBDS mutations is expected to have started to act from zygote and to be widespread to all tissues.
Available clinical data suggest that stature (the patient is at low centiles for SDS patients), pancreatic function (the patient is still on therapy with pancreatic enzymes), and cognitive development are not strongly and favorably influenced. As far as the skeletal features are concerned, osteoblasts from UPN 2 did not differ with respect to osteoblasts from a group of 13 SDS patients studied by Frattini et al. (2021) who demonstrated lower mineralization capacity and lower expression of genes responsible for osteoblastogenesis.
Conversely, hematological data and bone marrow picture, over the last 10 years, have been stable; hemoglobin, platelets, and lymphocytes have consistently been within normal limits and the patient never needed any kind of therapy. The patient has moderate cytopenia, which does not appear to have ever required transfusions or the use of hematopoietic growth factors.
Only mild bone marrow alterations were present, and no evidence of any worsening has been reported so far, during the course of the disease. In addition, negative anti-p53 antibody reactivity has been repeatedly observed.
Similarly, Tan et al. (2021) reported an SBDS-mutated patient with a somatic bone marrow variant in EIF6 (c.182G>T, p.Arg61Leu), showing stable and mild hematological features. When studying this patient, they interpreted the variant as an example of a somatic genetic rescue. The Arg61Leu variant in fact rescued the defect of Sdo1-deficient yeast cells and the larval lethality of Sbds-deficient Drosophila (Tan et al., 2021). We expected that the variant p.Phe34Leu, identified in our patient, could have a similar effect as Arg61Leu, predicting a reduced binding to the nascent 60S ribosome, but it needs to be proven.
Our findings, on a germinal level, might agree with the hypothesis that somatic EIF6 mutations, observed by Kennedy and others, produce a compensatory effect for the pre-existing germline SBDS mutations, thus reducing the risk of developing malignant neoplasia. Among these somatic mutations, missense ones were predominantly located in regions encoding conserved secondary structure motives, exactly like the p.Phe34Leu variant described here, which is a newly described one (Kennedy et al., 2021).
In all SDS patients, the diagnosis prompts compulsory monitoring, over time, of the most relevant symptoms, including exocrine pancreatic dysfunction, especially bone marrow failure (Valli et al., 2017). In our case, this program of surveillance will produce a large amount of useful information not only to manage the clinical problems of the patient but also to compare him with the group of other SDS patients, whose exome analysis did not reveal any significant EIF6 variants.
If the periodic clinical check will confirm a stable and positive situation, particularly concerning the hematological picture, we can confirm that we have observed a human example of somatic genetic rescue through EIF6 mutations comparable to yeast and Drosophila models.
Undoubtedly, our observations, supported by genetic considerations and in silico simulations, may be of actual use for clinical interpretation only when experimentally validated.
The study of the effect of p.Phe34Leu variant could also be improved by using the molecular dynamics simulation approach, as previously performed for EFL1 (Delre et al., 2020).
To check the presence, in addition to the EIF6 variant, of any other variants in genes involved in MDS or AML (Lindsley, 2017; Steensma, 2017; Armstrong et al., 2018; Bluteau et al., 2018; Obrochta and Godley, 2018; Kennedy et al., 2021), we analyzed the exome file of UPN 2 and three genes, ASXL1, JAK2, and GNAS, were identified as bearing a mutation which might be relevant for the risk of MDS/AML (Table1).
ASXL1: Several studies showed that ASXL1 somatic mutations are among the most frequent variants in all subtypes of myeloid malignancies including MDS (11–21% cases), AML (5–11%), myeloproliferative neoplasm (MPN), and chronic myelomonocytic leukemia (CMML) (40–50%) (Boultwood et al., 2010; Thol et al., 2011; Fujino and Kitamura, 2020). ASXL1 mutations accelerate myeloid malignancies and are always associated with poor prognosis (Gelsi-Boyer et al., 2012).
Confirmatory evidence of ASXL1 relevance in hematological malignancies was produced by Wang et al. (2014) who showed that heterozygous genetic ASXL1-knockout mice (Asxl1+/−) developed MDS/MPN and by Abdel-Wahab et al. (2013), who reported that hematopoietic cell-specific deletions of Asxl1 induced an MDS-like disease. Germinal de novo nonsense or frameshift mutations in ASXL1 cause the Bohring–Opitz syndrome (Bedoukian et al., 2018), and a few reports describe missense germline mutations, in association with familial recurrence of different hematological malignancies.
Hamadou and coworkers reported the mutation p.Arg402Gln in two Tunisian sisters affected by NHL (tonsil large B-cell lymphoma and gastric MALT lymphoma) (Hamadou et al., 2016); Seiter and coworkers reported a father and son, both carrying the p.Asn968Ser mutation, who developed MDS then evolving in AML at age 75 and 46, respectively (Seiter et al., 2018).
Zebisch and coworkers described a family in which a 60-year-old female affected with MDS was transplanted from her 58-year-old apparently healthy sister; the graft function was poor, and laboratory investigations demonstrated the presence of the same ASXL1 mutation, p.Pro808His, in the donor sister (Zebisch et al., 2020).
An ASXL1 mutation p.Gly704Arg is present in a family (a 2-year-old child and her 54-year-old paternal uncle, both affected with primary myelofibrosis) included in the study of Andres-Zayas and coworkers on germline predisposition for leukemia (Andrés-Zayas et al., 2021).
In our patient, the missense variant p.Asp1211Asn is interpreted as pathogenic only by SIFT (https://sift.bii.a-star.edu.sg) (Vaser et al., 2016) (Table1), and the previously reported germinal ASXL1 mutations were reported as damaging or disease causing by SIFT and MutationTaster, respectively (https://www.mutationtaster.org) (Schwarz et al., 2014), while other tools classified them as benign.
Based on available evidence, missense germinal mutations in ASXL1 might act as a risk factor for developing hematological malignancies; topics such as penetrance or anticipation can be discussed only when a much larger number of cases have been studied (Seiter et al., 2018). At present hematological surveillance in carriers of germinal missense mutations in ASXL1 seems a reasonable management of the problem, even if the functional significance of the mutation is not fully defined.
JAK2: The JAK2 variant, p.Ser15Phe, is interpreted as pathogenic only in one out of the seven prediction tools used (Table 1); it falls at the N-terminus outside the most functionally relevant domains reported by Benton and coworkers (Benton et al., 2019). Reports about germinal JAK2 mutations are rare (Goldin et al., 2009; Park et al., 2020) and quoted mutations are described to be pathogenic when present in bone marrow as somatic mutations (Klampfl et al., 2013; Tefferi, 2016).
GNAS: The GNAS variant p.Glu717Ter, found in our patient and paternally inherited, is interpreted as pathogenic by all four applicable prediction tools (Table1). Also, for GNAS reported evidence underlines its involvement in both genetic and hematological diseases.
Activating gain-of-function mutations are known to cause Mendelian disorders as polyostotic fibrous dysplasia and McCune–Albright syndrome (Robinson et al., 2016). GNAS somatic mutations have been recurrently found in hematological disorders such as MDS and lymphoma (Bejar et al., 2011; Xie et al., 2014). In addition, GNAS germline mutations are related to different types of pseudohypoparathyroidism (PHP-Ia,-Ib,-Ic) and pseudopseudohypoparathyroidism (PPHP). PHP and PPHP are autosomal dominant conditions and are associated with a lack of expression of Gsα if the pathogenic variant inactivates maternal or paternal alleles, respectively (Haldeman-Englert et al., 1993). As the clinical data on our patient include increased PTH, a careful evaluation for endocrinological condition was suggested to the family, and it is still in progress.
Conclusion
UPN 2 was diagnosed with SDS by targeted mutation analysis. WES studies for variants known in hematological disorders identified a variant in EIF6 which is likely to be associated with a favorable bone marrow condition, a variant in ASXL1 which warrants hematological surveillance, already scheduled in any SDS patient, and a variant in GNAS, which may explain the endocrinological abnormalities found, which are not part of the description of the syndrome.
These types of data, especially if it is supported by functional studies, clearly suggest that the use of extended genome analysis can provide useful data for better patient management.
This was demonstrated here for SDS, but it may be extended to other disorders. Certainly, a consistent portion of phenotypic variability in patients sharing the same monogenic disease is caused by additional genomic alterations. The progress in identifying these changes will help walk on the path of personalized medicine.
Data Availability Statement
The datasets for this article are not publicly available due to concerns regarding participant/patient anonymity. Requests to access the datasets should be directed to the corresponding author.
Ethics Statement
Ethical review and approval were not required for the study on human participants in accordance with the local legislation and institutional requirements. The patients/participants provided their written informed consent to participate in this study.
Author Contributions
Molecular investigation and Sanger sequencing: IT, SF, AF, and AM; bioinformatic work and writing the manuscript: IT, AM, CS, and CD; clinical data: MZ, EB, and MC; modelling of proteins: CS; cytogenetic data: RV, PR, GP, and FP.
Funding
We gratefully acknowledge the financial support by the AISS (Associazione Italiana Sindrome di Shwachman) to AM. This research was supported by a Grant from the Italian Ministry of Education, University and Research (MIUR) to the Department of Molecular Medicine of the University of Pavia under the initiative “Dipartimenti di Eccellenza (2018–2022)”. This work was supported by the grants from Ricerca Corrente to Fondazione IRCCS Policlinico San Matteo (RCR 08045818).
Conflict of Interest
The authors declare that the research was conducted in the absence of any commercial or financial relationships that could be construed as a potential conflict of interest.
Publisher’s Note
All claims expressed in this article are solely those of the authors and do not necessarily represent those of their affiliated organizations, or those of the publisher, the editors, and the reviewers. Any product that may be evaluated in this article, or claim that may be made by its manufacturer, is not guaranteed or endorsed by the publisher.
Acknowledgments
The authors thank enGenome for improving the bioinformatics analysis. The authors specially thank the Italian Agency for Development and Cooperation (AICS), the Center of International Cooperation and Development (CICOPS) at University of Pavia, and Partnership for Knowledge (PfK) initiative for offering a Ph. D scholarship in Translational medicine to IT. This work is part of the Ph. D thesis of IT.
References
Abdel-Wahab, O., Gao, J., Adli, M., Dey, A., Trimarchi, T., Chung, Y. R., et al. (2013). Deletion of Asxl1 Results in Myelodysplasia and Severe Developmental Defects In Vivo. J. Exp. Med. 210 (12), 2641–2659. doi:10.1084/jem.20131141
Andrés-Zayas, C., Suárez-González, J., Rodríguez-Macías, G., Dorado, N., Osorio, S., Font, P., et al. (2021). Clinical Utility of Targeted Next-Generation Sequencing for the Diagnosis of Myeloid Neoplasms with Germline Predisposition. Mol. Oncol. 15 (9), 2273–2284. doi:10.1002/1878-0261.12921
Armstrong, R. N., Steeples, V., Singh, S., Sanchi, A., Boultwood, J., and Pellagatti, A. (2018). Splicing Factor Mutations in the Myelodysplastic Syndromes: Target Genes and Therapeutic Approaches. Adv. Biol. Regul. 67, 13–29. doi:10.1016/j.jbior.2017.09.008
Bedoukian, E., Copenheaver, D., Bale, S., and Deardorff, M. (2018). Bohring‐Opitz Syndrome Caused by an ASXL1 Mutation Inherited from a Germline Mosaic Mother. Am. J. Med. Genet. 176 (5), 1249–1252. doi:10.1002/ajmg.a.38686
Bejar, R., Stevenson, K., Abdel-Wahab, O., Galili, N., Nilsson, B., Garcia-Manero, G., et al. (2011). Clinical Effect of Point Mutations in Myelodysplastic Syndromes. N. Engl. J. Med. 364 (26), 2496–2506. doi:10.1056/nejmoa1013343
Benton, C. B., Boddu, P. C., DiNardo, C. D., Bose, P., Wang, F., Assi, R., et al. (2019). Janus Kinase 2 Variants Associated with the Transformation of Myeloproliferative Neoplasms into Acute Myeloid Leukemia. Cancer 125 (11), 1855–1866. doi:10.1002/cncr.31986
Bluteau, O., Sebert, M., Leblanc, T., Peffault de Latour, R., Quentin, S., Lainey, E., et al. (2018). A Landscape of Germ Line Mutations in a Cohort of Inherited Bone Marrow Failure Patients. Blood 131 (7), 717–732. doi:10.1182/blood-2017-09-806489
Boocock, G. R. B., Morrison, J. A., Popovic, M., Richards, N., Ellis, L., Durie, P. R., et al. (2003). Mutations in SBDS Are Associated with Shwachman-Diamond Syndrome. Nat. Genet. 33 (1), 97–101. doi:10.1038/ng1062
Boultwood, J., Perry, J., Pellagatti, A., Fernandez-Mercado, M., Fernandez-Santamaria, C., Calasanz, M. J., et al. (2010). Frequent Mutation of the Polycomb-Associated Gene ASXL1 in the Myelodysplastic Syndromes and in Acute Myeloid Leukemia. Leukemia 24 (5), 1062–1065. doi:10.1038/leu.2010.20
Carapito, R., Konantz, M., Paillard, C., Miao, Z., Pichot, A., Leduc, M. S., et al. (2017). Mutations in Signal Recognition Particle SRP54 Cause Syndromic Neutropenia with Shwachman-Diamond-like Features. J. Clin. Invest. 127 (11), 4090–4103. doi:10.1172/jci92876
Ceci, M., Gaviraghi, C., Gorrini, C., Sala, L. A., Offenhäuser, N., Carlo Marchisio, P., et al. (2003). Release of eIF6 (p27BBP) from the 60S Subunit Allows 80S Ribosome Assembly. Nature 426 (6966), 579–584. doi:10.1038/nature02160
De Keersmaecker, K., Sulima, S. O., and Dinman, J. D. (2015). Ribosomopathies and the Paradox of Cellular Hypo- to Hyperproliferation. Blood 125 (9), 1377–1382. doi:10.1182/blood-2014-10-569616
Delre, P., Alberga, D., Gijsbers, A., Sánchez-Puig, N., Nicolotti, O., Saviano, M., et al. (2020). Exploring the Role of Elongation Factor-like 1 (EFL1) in Shwachman-Diamond Syndrome through Molecular Dynamics. J. Biomol. Struct. Dyn. 38 (17), 5219–5229. doi:10.1080/07391102.2019.1704883
Dhanraj, S., Matveev, A., Li, H., Lauhasurayotin, S., Jardine, L., Cada, M., et al. (2017). Biallelic Mutations in DNAJC21 Cause Shwachman-Diamond Syndrome. Blood 129 (11), 1557–1562. doi:10.1182/blood-2016-08-735431
Donadieu, J., Fenneteau, O., Beaupain, B., Beaufils, S., Bellanger, F., Mahlaoui, N., et al. (2012). Classification of and Risk Factors for Hematologic Complications in a French National Cohort of 102 Patients with Shwachman-Diamond Syndrome. Haematologica 97 (9), 1312–1319. doi:10.3324/haematol.2011.057489
Dror, Y., Donadieu, J., Koglmeier, J., Dodge, J., Toiviainen-Salo, S., Makitie, O., et al. (2011). Draft Consensus Guidelines for Diagnosis and Treatment of Shwachman-Diamond Syndrome. alAnn N. Y. Acad Sci 1242 (1), 40–55. doi:10.1111/j.1749-6632.2011.06349.x
Finch, A. J., Hilcenko, C., Basse, N., Drynan, L. F., Goyenechea, B., Menne, T. F., et al. (2011). Uncoupling of GTP Hydrolysis from eIF6 Release on the Ribosome Causes Shwachman-Diamond Syndrome. Genes Dev. 25 (9), 917–929. doi:10.1101/gad.623011
Frattini, A., Bolamperti, S., Valli, R., Cipolli, M., Pinto, R. M., Bergami, E., et al. (2021). Enhanced P53 Levels Are Involved in the Reduced Mineralization Capacity of Osteoblasts Derived from Shwachman-Diamond Syndrome Subjects. Ijms 22 (24), 13331. doi:10.3390/ijms222413331
Fujino, T., and Kitamura, T. (2020). ASXL1 Mutation in Clonal Hematopoiesis. Exp. Hematol. 83, 74–84. doi:10.1016/j.exphem.2020.01.002
Fülöp, V., and Jones, D. T. (1999). β Propellers: Structural Rigidity and Functional Diversity. Curr. Opin. Struct. Biol. 9 (6), 715–721. doi:10.1016/s0959-440x(99)00035-4
Furutani, E., Liu, S., Galvin, A., Steltz, S., Malsch, M. M., Loveless, S. K., et al. (2022). Hematologic Complications with Age in Shwachman-Diamond Syndrome. Blood Adv. 6 (1), 297–306. doi:10.1182/bloodadvances.2021005539
Galletta, T. J., Loveless, S. K., Malsch, M. M., Shimamura, A., and Myers, K. C. (2022). Coronavirus Disease 2019 and Vaccination in Patients with Shwachman-Diamond Syndrome. Pediatr. Blood Cancer 69 (5), e29647. doi:10.1002/pbc.29647
Gandin, V., Miluzio, A., Barbieri, A. M., Beugnet, A., Kiyokawa, H., Marchisio, P. C., et al. (2008). Eukaryotic Initiation Factor 6 Is Rate-Limiting in Translation, Growth and Transformation. Nature 455 (7213), 684–688. doi:10.1038/nature07267
Gelsi-Boyer, V., Brecqueville, M., Devillier, R., Murati, A., Mozziconacci, M.-J., and Birnbaum, D. (2012). Mutations in ASXL1 Are Associated with Poor Prognosis across the Spectrum of Malignant Myeloid Diseases. J. Hematol. Oncol. 5, 12. doi:10.1186/1756-8722-5-12
Goldin, L. R., Björkholm, M., Kristinsson, S. Y., Samuelsson, J., and Landgren, O. (2009). Germline and Somatic JAK2 Mutations and Susceptibility to Chronic Myeloproliferative Neoplasms. Genome Med. 1 (5), 55. doi:10.1186/gm55
Groft, C. M., Beckmann, R., Sali, A., and Burley, S. K. (2000). Crystal Structures of Ribosome Anti-association Factor IF6. Nat. Struct. Biol. 7 (12), 1156–1164. doi:10.1038/82017
Haldeman-Englert, C. R., Hurst, A. C., and Levine, M. A. (1993). “Disorders of GNAS Inactivation,” in GeneReviews® [Internet]. M. P. Adam, H. H. Ardinger, R. A. Pagon, S. E. Wallace, L. J. Bean, K. W. Grippet al. (Seattle (WA): University of Washington, Seattle). Available from: http://www.ncbi.nlm.nih.gov/books/NBK459117/.
Hamadou, W. S., Abed, R. E., Besbes, S., Bourdon, V., Fabre, A., Youssef, Y. B., et al. (2016). Familial Hematological Malignancies: ASXL1 Gene Investigation. Clin. Transl. Oncol. 18 (4), 385–390. doi:10.1007/s12094-015-1379-7
Kennedy, A. L., Myers, K. C., Bowman, J., Gibson, C. J., Camarda, N. D., Furutani, E., et al. (2021). Distinct Genetic Pathways Define Pre-malignant versus Compensatory Clonal Hematopoiesis in Shwachman-Diamond Syndrome. Nat. Commun. 12 (1), 1334. doi:10.1038/s41467-021-21588-4
Khan, A. W., Kennedy, A., Furutani, E., Myers, K., Frattini, A., Acquati, F., et al. (2021). The Frequent and Clinically Benign Anomalies of Chromosomes 7 and 20 in Shwachman-Diamond Syndrome May Be Subject to Further Clonal Variations. Mol. Cytogenet 14 (1), 54. doi:10.1186/s13039-021-00575-w
Khan, A. W., Minelli, A., Frattini, A., Montalbano, G., Bogni, A., Fabbri, M., et al. (2020). Microarray Expression Studies on Bone Marrow of Patients with Shwachman-Diamond Syndrome in Relation to Deletion of the Long Arm of Chromosome 20, Other Chromosome Anomalies or Normal Karyotype. Mol. Cytogenet 13, 1. doi:10.1186/s13039-019-0466-9
Klampfl, T., Gisslinger, H., Harutyunyan, A. S., Nivarthi, H., Rumi, E., Milosevic, J. D., et al. (2013). Somatic Mutations of Calreticulin in Myeloproliferative Neoplasms. N. Engl. J. Med. 369 (25), 2379–2390. doi:10.1056/nejmoa1311347
Lee, S., Shin, C. H., Lee, J., Jeong, S. D., Hong, C. R., Kim, J. D., et al. (2021). Somatic Uniparental Disomy Mitigates the Most Damaging EFL1 Allele Combination in Shwachman-Diamond Syndrome. bioRxiv. Available from: https://www.biorxiv.org/content/10.1101/483362v5.
Liang, X., Zuo, M. Q., Zhang, Y., Li, N., Ma, C., Dong, M. Q., et al. (2020). Structural Snapshots of Human pre-60S Ribosomal Particles before and after Nuclear Export. Nat. Commun. 11 (1), 3542. doi:10.1038/s41467-020-17237-x
Lindsley, R. C. (2017). Uncoding the Genetic Heterogeneity of Myelodysplastic Syndrome. Hematol. Am. Soc. Hematol. Educ. Program 2017 (1), 447–452. doi:10.1182/asheducation-2017.1.447
Menne, T. F., Goyenechea, B., Sánchez-Puig, N., Wong, C. C., Tonkin, L. M., Ancliff, P. J., et al. (2007). The Shwachman-Bodian-Diamond Syndrome Protein Mediates Translational Activation of Ribosomes in Yeast. Nat. Genet. 39 (4), 486–495. doi:10.1038/ng1994
Minelli, A., Maserati, E., Nicolis, E., Zecca, M., Sainati, L., Longoni, D., et al. (2009). The Isochromosome I(7)(q10) Carrying c.258+2t>c Mutation of the SBDS Gene Does Not Promote Development of Myeloid Malignancies in Patients with Shwachman Syndrome. Leukemia 23 (4), 708–711. doi:10.1038/leu.2008.369
Minelli, A., Nacci, L., Valli, R., Pietrocola, G., Ramenghi, U., Locatelli, F., et al. (2016). Structural Variation in SBDS Gene, with Loss of Exon 3, in Two Shwachman-Diamond Patients. Blood Cells, Mol. Dis. 60, 33–35. doi:10.1016/j.bcmd.2016.06.007
Morini, J., Nacci, L., Babini, G., Cesaro, S., Valli, R., Ottolenghi, A., et al. (2019). Whole Exome Sequencing Discloses Heterozygous Variants in the DNAJC 21 and EFL 1 Genes but Not in SRP 54 in 6 Out of 16 Patients with Shwachman‐Diamond Syndrome Carrying Biallelic SBDS Mutations. Br. J. Haematol. 185 (3), 627–630. doi:10.1111/bjh.15594
Nacci, L., Valli, R., Maria Pinto, R., Zecca, M., Cipolli, M., Morini, J., et al. (2017). Parental origin of the deletion del(20q) in Shwachman-Diamond patients and loss of the paternally derived allele of the imprintedL3MBTL1gene. Genes Chromosom. Cancer 56 (1), 51–58. doi:10.1002/gcc.22401
Nelson, A. S., and Myers, K. C. (2018). Diagnosis, Treatment, and Molecular Pathology of Shwachman-Diamond Syndrome. Hematology/Oncology Clin. N. Am. 32 (4), 687–700. doi:10.1016/j.hoc.2018.04.006
Nicora, G., Zucca, S., Limongelli, I., Bellazzi, R., and Magni, P. (2022). A Machine Learning Approach Based on ACMG/AMP Guidelines for Genomic Variant Classification and Prioritization. Sci. Rep. 12 (1), 2517. doi:10.1038/s41598-022-06547-3
Obrochta, E., and Godley, L. A. (2018). Identifying Patients with Genetic Predisposition to Acute Myeloid Leukemia. Best Pract. Res. Clin. Haematol. 31 (4), 373–378. doi:10.1016/j.beha.2018.09.014
Park, H. S., Son, B. R., Shin, K. S., Kim, H. K., Yang, Y., Jeong, Y., et al. (2020). Germline JAK2 V617F Mutation as a Susceptibility Gene Causing Myeloproliferative Neoplasm in First-Degree Relatives. Leukemia Lymphoma 61 (13), 3251–3254. doi:10.1080/10428194.2020.1802448
Pressato, B., Valli, R., Marletta, C., Mare, L., Montalbano, G., Curto, F. L., et al. (2015). Cytogenetic Monitoring in Shwachman-Diamond Syndrome. J. Pediatr. Hematol. Oncol. 37 (4), 307–310. doi:10.1097/mph.0000000000000268
Pressato, B., Valli, R., Marletta, C., Mare, L., Montalbano, G., Curto, F. L., et al. (2012). Deletion of Chromosome 20 in Bone Marrow of Patients with Shwachman-Diamond Syndrome, Loss of the EIF6 Gene and Benign Prognosis. Br. J. Haematol. 157 (4), 503–505. doi:10.1111/j.1365-2141.2012.09033.x
Raczy, C., Petrovski, R., Saunders, C. T., Chorny, I., Kruglyak, S., Margulies, E. H., et al. (2013). Isaac: Ultra-fast Whole-Genome Secondary Analysis on Illumina Sequencing Platforms. Bioinforma. Oxf Engl. 29 (16), 2041–2043. doi:10.1093/bioinformatics/btt314
Robinson, C., Collins, M. T., and Boyce, A. M. (2016). Fibrous dysplasia/McCune-Albright Syndrome: Clinical and Translational Perspectives. Curr. Osteoporos. Rep. 14 (5), 178–186. doi:10.1007/s11914-016-0317-0
Schwarz, J. M., Cooper, D. N., Schuelke, M., and Seelow, D. (2014). MutationTaster2: Mutation Prediction for the Deep-Sequencing Age. Nat. Methods 11 (4), 361–362. doi:10.1038/nmeth.2890
Seiter, K., Htun, K., Baskind, P., and Liu, Z. (2018). Acute Myeloid Leukemia in a Father and Son with a Germline Mutation of ASXL1. Biomark. Res. 6 (1), 7. doi:10.1186/s40364-018-0121-3
Steensma, D. P. (2017). The Evolving Role of Genomic Testing in Assessing Prognosis of Patients with Myelodysplastic Syndromes. Best Pract. Res. Clin. Haematol. 30 (4), 295–300. doi:10.1016/j.beha.2017.09.009
Stepensky, P., Chacón-Flores, M., Kim, K. H., Abuzaitoun, O., Bautista-Santos, A., Simanovsky, N., et al. (2017). Mutations inEFL1, anSBDSpartner, Are Associated with Infantile Pancytopenia, Exocrine Pancreatic Insufficiency and Skeletal Anomalies in aShwachman-Diamond like Syndrome. J. Med. Genet. 54 (8), 558–566. doi:10.1136/jmedgenet-2016-104366
Tan, Q. K.-G., Cope, H., Spillmann, R. C., Stong, N., Jiang, Y.-H., McDonald, M. T., et al. (2018). Further Evidence for the Involvement of EFL1 in a Shwachman-Diamond-like Syndrome and Expansion of the Phenotypic Features. Cold Spring Harb. Mol. Case Stud. 4 (5), a003046. doi:10.1101/mcs.a003046
Tan, S., Kermasson, L., Hilcenko, C., Kargas, V., Traynor, D., Boukerrou, A. Z., et al. (2021). Somatic Genetic Rescue of a Germline Ribosome Assembly Defect. Nat. Commun. 12, 5044. doi:10.1038/s41467-021-24999-5
Tan, S., Kermasson, L., Hoslin, A., Jaako, P., Faille, A., Acevedo-Arozena, A., et al. (2019). EFL1 Mutations Impair eIF6 Release to Cause Shwachman-Diamond Syndrome. Blood 134 (3), 277–290. doi:10.1182/blood.2018893404
Tefferi, A. (2016). Somatic JAK2 Mutations and Their Tumor Phenotypes. Blood 128 (6), 748–749. doi:10.1182/blood-2016-06-722645
Thol, F., Friesen, I., Damm, F., Yun, H., Weissinger, E. M., Krauter, J., et al. (2011). Prognostic Significance of ASXL1 Mutations in Patients with Myelodysplastic Syndromes. Jco 29 (18), 2499–2506. doi:10.1200/jco.2010.33.4938
Tummala, H., Walne, A. J., Williams, M., Bockett, N., Collopy, L., Cardoso, S., et al. (2016). DNAJC21 Mutations Link a Cancer-Prone Bone Marrow Failure Syndrome to Corruption in 60S Ribosome Subunit Maturation. Am. J. Hum. Genet. 99 (1), 115–124. doi:10.1016/j.ajhg.2016.05.002
Valli, R., De Paoli, E., Nacci, L., Frattini, A., Pasquali, F., and Maserati, E. (2017). Novel Recurrent Chromosome Anomalies in Shwachman-Diamond Syndrome. Pediatr. Blood Cancer 64 (8), e26454. doi:10.1002/pbc.26454
Valli, R., Minelli, A., Galbiati, M., D'Amico, G., Frattini, A., Montalbano, G., et al. (2019). Shwachman-Diamond Syndrome with Clonal Interstitial Deletion of the Long Arm of Chromosome 20 in Bone Marrow: Haematological Features, Prognosis and Genomic Instability. Br. J. Haematol. 184 (6), 974–981. doi:10.1111/bjh.15729
Valli, R., Pressato, B., Marletta, C., Mare, L., Montalbano, G., Curto, F. L., et al. (2013). Different Loss of Material in Recurrent Chromosome 20 Interstitial Deletions in Shwachman-Diamond Syndrome and in Myeloid Neoplasms. Mol. Cytogenet 6 (1), 56. doi:10.1186/1755-8166-6-56
Vaser, R., Adusumalli, S., Leng, S. N., Sikic, M., and Ng, P. C. (2016). SIFT Missense Predictions for Genomes. Nat. Protoc. 11 (1), 1–9. doi:10.1038/nprot.2015.123
Wang, J., Li, Z., He, Y., Pan, F., Chen, S., Rhodes, S., et al. (2014). Loss of Asxl1 Leads to Myelodysplastic Syndrome-like Disease in Mice. Blood 123 (4), 541–553. doi:10.1182/blood-2013-05-500272
Waterhouse, A., Bertoni, M., Bienert, S., Studer, G., Tauriello, G., Gumienny, R., et al. (2018). SWISS-MODEL: Homology Modelling of Protein Structures and Complexes. Nucleic Acids Res. 46 (W1), W296–W303. doi:10.1093/nar/gky427
Weis, F., Giudice, E., Churcher, M., Jin, L., Hilcenko, C., Wong, C. C., et al. (2015). Mechanism of eIF6 Release from the Nascent 60S Ribosomal Subunit. Nat. Struct. Mol. Biol. 22 (11), 914–919. doi:10.1038/nsmb.3112
Xie, M., Lu, C., Wang, J., McLellan, M. D., Johnson, K. J., Wendl, M. C., et al. (2014). Age-related Mutations Associated with Clonal Hematopoietic Expansion and Malignancies. Nat. Med. 20 (12), 1472–1478. doi:10.1038/nm.3733
Keywords: Shwachman–Diamond syndrome, EIF6, SBDS, whole-exome sequencing, case report
Citation: Taha I, Foroni S, Valli R, Frattini A, Roccia P, Porta G, Zecca M, Bergami E, Cipolli M, Pasquali F, Danesino C, Scotti C and Minelli A (2022) Case Report: Heterozygous Germline Variant in EIF6 Additional to Biallelic SBDS Pathogenic Variants in a Patient With Ribosomopathy Shwachman–Diamond Syndrome. Front. Genet. 13:896749. doi: 10.3389/fgene.2022.896749
Received: 09 May 2022; Accepted: 23 June 2022;
Published: 12 August 2022.
Edited by:
Tommaso Pippucci, Sant'Orsola-Malpighi Polyclinic, ItalyReviewed by:
Wenhao Zhou, Fudan University, ChinaDritan Siliqi, National Research Council (IC-CNR), Italy
Steven Ellis, University of Louisville, United States
Copyright © 2022 Taha, Foroni, Valli, Frattini, Roccia, Porta, Zecca, Bergami, Cipolli, Pasquali, Danesino, Scotti and Minelli. This is an open-access article distributed under the terms of the Creative Commons Attribution License (CC BY). The use, distribution or reproduction in other forums is permitted, provided the original author(s) and the copyright owner(s) are credited and that the original publication in this journal is cited, in accordance with accepted academic practice. No use, distribution or reproduction is permitted which does not comply with these terms.
*Correspondence: Antonella Minelli, antonella.minelli@unipv.it
†These authors have contributed equally to this work