- 1Department of Medical Genetics, Changzhi Maternal and Child Health Care Hospital, Changzhi, China
- 2School of Life Sciences, Fudan University, Shanghai, China
- 3Department of Pediatrics, Changzhi Maternal and Child Health Care Hospital, Changzhi, China
- 4Obstetrics Department, Changzhi Maternal and Child Health Care Hospital, Changzhi, China
- 5Human Phenome Institute, Zhangjiang Fudan International Innovation Center, MOE Key Laboratory of Contemporary Anthropology, Fudan University, Shanghai, China
Objective: To evaluate positive rate and accuracy of non-invasive prenatal testing (NIPT) combining Z-score and maternal copy number variation (CNV) analysis. To assess the relationship between Z-score and positive predictive value (PPV).
Methods: This prospective study included 61525 pregnancies to determine the correlation between Z-scores and PPV in NIPT, and 3184 pregnancies to perform maternal CNVs analysis. Positive results of NIPT were verified by prenatal diagnosis and/or following-up after birth. Z-score grouping, logistic regression analysis, receiver operating characteristic (ROC) curves, and S-curve trends were applied to correlation analysis of Z-scores and PPV. The maternal CNVs were classified according to the technical standard for the interpretation of ACMG. Through genetic counseling, fetal and maternal phenotypes and family histories were collected.
Results: Of the 3184 pregnant women, 22 pregnancies were positive for outlier Z-scores, suggesting fetal aneuploidy. 12 out of 22 pregnancies were true positive (PPV = 54.5%). 17 pregnancies were found maternal pathogenic or likely pathogenic CNVs (> 0.5 Mb) through maternal CNV analysis. Prenatal diagnosis revealed that 7 out of 11 fetuses carried the same CNVs as the mother. Considering the abnormal biochemical indicators during pregnancy and CNV-related clinical phenotypes after birth, two male fetuses without prenatal diagnosis were suspected to carry the maternally-derived CNVs. Further, we identified three CNV-related family histories with variable phenotypes. Statistical analysis of the 61525 pregnancies revealed that Z-scores of chromosomes 21 and 18 were significantly associated with PPV at 3 ≤ Z ≤ 40. Notably, three pregnancies with Z > 40 were both maternal full aneuploidy. At Z < -3, fetuses carried microdeletions instead of monosomies. Sex chromosome trisomy was significantly higher PPV than monosomy.
Conclusion: The positive rate of the NIPT screening model combining Z-score and maternal CNV analysis increased from 6.91‰ (22/3184) to 12.25‰ (39/3184) and true positives increased from 12 to 21 pregnancies. We found that this method could improve the positive rate and accuracy of NIPT for aneuploidies and CNVs without increasing testing costs. It provides an early warning for the inheritance of pathogenic CNVs to the next generation.
Introduction
Non-invasive prenatal testing (NIPT) based on high-throughput sequencing can detect fetal common chromosomal aneuploidies. The existence of placental cell-free fetal DNA (cff-DNA) fragments in the peripheral blood of pregnant women provide a basis for NIPT technology (Lo et al., 1997). Mostly, NIPT result was calculated by Z-score in which the individual sample is compared with a control group of normal (diploid) samples (Chiu et al., 2008). Numerous studies have shown that the model’s accuracy is higher than that of serological screening technology, regardless of single and twin pregnancies (Zhang et al., 2015; Gil et al., 2017; Iwarsson et al., 2017; Gil et al., 2019). However, there are false results that cannot be avoided owing to the limitations of the materials and methods. Several studies indicated the accuracy and positive predictive value (PPV) of NIPT were related to Z-score; and the higher the Z-score, the greater the likelihood of true positive (Tian et al., 2018; Wan et al., 2021). Another study showed that the optimal cut-off values for trisomy (T) 21 and T18 Z-scores were 5.79 and 6.05, respectively (Zhou et al., 2021), which PPV in the group of Z-score > optimal cutoff value was higher than that in the group of 3 ≤ Z-score < optimal cutoff value. However, increasing the cut-off value will produce more false negatives. Therefore, more research is necessary on the relationship between Z-score and PPV before adjusting the cut-off value.
Maternal copy number variations (CNVs) were ignored in either NIPT or NIPT-plus except identifying of fetal de novo CNVs. Pathogenic CNVs cause over 300 types of chromosomal microdeletion/microduplication syndromes (MMS), with a total incidence of 3% (Weise et al., 2012; Nevado et al., 2014; Levy et al., 2018). It is very common for heterogeneity of clinical feature due to the location and size of CNVs. A few of fetal structural abnormalities resulted from microdeletion/microduplication could be found by ultrasound screening, however, most of the MMS could not be identified during pregnancy (Grati et al., 2015). Recently, there were studies to shown that the PPV of fetal CNVs detected by NIPT-plus was 20–40% (Chen et al., 2019; Hu et al., 2019). However, no research pay attention to pathogenic CNVs inherited from parents. We found maternal CNV analysis through NIPT without additional cost was meaningful for prediction of birth defects and future treatment.
This prospective study explored a new NIPT model combining Z-score and maternal CNV analysis to identify high-risk fetuses, including aneuploidies and CNVs of each chromosome. In addition, we investigated whether the Z-score was correlated with PPV to assess the accuracy of NIPT-positive results from a single center within the past 5 years. This study aimed to provide a more accurate basis for clinical genetic counselling.
Materials and Methods
Subjects
Pregnant women selected for NIPT in Changzhi Maternal and Child Health Care Hospital were continuously included in this study from October 2016 to November 2021. Testing was successful in 61,525 pregnant women, of which 32,361 pregnancies from October 2016 to June 2019 were derived from (Li et al., in press). The study included pregnant women with aneuploidy to investigate the effects of maternal aneuploidy on the Z-score, and we also recommended that pregnant women participated simultaneously in invasive prenatal diagnosis. A total of 3,184 pregnant women were selected from August to November 2021 for NIPT combining Z-score and maternal CNV analysis (CNV > 0.5 Mb). All pregnant women voluntarily signed informed consent forms prior to the procedure. Unique identifiers were deleted before they were included in the study. All procedures were approved by the Medical Ethics Committee of Changzhi Maternal and Child Health Care Hospital (No. CZSFYLL2021017).
Noninvasive Prenatal Testing
Plasma was separated via a two-step centrifugation process within 72 h after collecting 5–8 ml maternal peripheral blood using a dedicated cell-free DNA collection tube. After cf-DNA extraction, library construction, and pooling, samples were sequenced on the Illumina NextSeq CN500 or NextSeq 550Dx platforms in collaboration with Findgene (Shanghai, China) or Biosan (Hangzhou, China). Sequences were aligned to the human genome-wide standard sequence (GRCh37) using BWA software, and Z-scores for each chromosome were obtained from bioinformatics analysis. Z-score were corrected by a series of bioinformatics methods such as normalization, GC correction and filtering out maternal CNVs. But it cannot correct for maternal aneuploidy interference. The control used a non-fixed reference set (96 experimental samples per batch) for internal comparison to eliminate batch differences. Qualified samples required the row sequencing reads greater than 3.5Mb and the fetal frequency greater than 4%. The thresholds of aneuploidy were ±3. Below the lower limit indicated a high risk of monosomy, above the upper limit indicated a high risk of trisomy. Between -3 and +3 represented a low risk of aneuploidy. Interpreting results from sex chromosome aneuploidies (SCAs) required combining the Z-scores of chromosome (Chr) X and ChrY. The Z-scores of ChrX and ChrY should be between −3 and 3 in normal female fetuses. The Z-scores of ChrX and ChrY in normal male fetuses should be < −3 and >3, respectively. All cases with positive results for the first time were verified in another plasma, and only the verified results were included in statistical analyses.
Analysis of Maternal Copy Number Variations
CNVs were detected using sliding window algorithm counting reads in each continuous bins (100kb size). To verify that the method for maternal CNVs is reliable, we performed genomic testing of maternal own lymphocytes by CNV-seq or SNP-array technology. CNVs were classified according to the technical standard for the interpretation and reporting of constitutional copy-number variants by the American College of Medical Genetics and Genomics (ACMG) and the Clinical Genome Resource (ClinGen) version 2020 (Riggs et al., 2020). Maternal phenotype and family history were assessed through genetic counselling. Prenatal diagnosis was recommended for pregnancies with maternal CNVs.
Prenatal Diagnosis
Prenatal diagnosis was recommended in pregnant women with NIPT-positive. Amniocentesis was performed under ultrasound guidance at 18–23 gestational weeks or umbilical blood was performed when over 23 gestational weeks, with the consent of pregnant women and family members. Prenatal diagnosis techniques were chosen by one or a combination of karyotyping, SNP-array or CNV-seq. Operations and analyses were performed in accordance with relevant international and national guidelines. The detailed precedures were applied as previously reported (Ma et al., 2021).
Follow-Up
Pregnant women with NIPT-positive results who were not prenatally diagnosed at our institution were followed up to confirm the prenatal diagnosis at other institution or to perform postnatal diagnosis if they chose to continue their pregnancies.
Statistics
Logistic regression analysis was applied to associate Z-scores with the PPV of NIPT-positive results. The receiver operating characteristic (ROC) curve is a comprehensive index that reveals the relationship between sensitivity and specificity. The larger the area under the curve (AUC), the higher the accuracy. Analyses were in the R version 4.1.2. The trend of S-curves was drawn based on the Z-scores and PPV in MATLAB version R2021b with a single parameter logistic model, using the function
Results
Efficiency of the New Model Combining Z-Score and Maternal CNV
We combined Z-score and maternal CNV to analyze data from 3,184 pregnancies with 3,090 singletons and 94 twins, and 90 pregnancies using assisted reproductive technology. Participants were 30.24 ± 4.46 years old (range, 16–46 years). As shown in Table 1, a total of 22 pregnancies (all singletons) were positive, with outlier Z-scores (Z < -3 or Z > 3) suggesting fetal aneuploidy. Twelve were true positives (PPV = 54.5%), including seven with T21, one with T18, one with T13, two with SCAs, and one with T9. Maternal CNV analysis showed that 17 pregnant women had pathogenic or likely pathogenic CNVs, interpreted through websites such as DECIPHER (https://www.deciphergenomics.org) and ClinGen (https://dosage.clinicalgenome.org) in Table 2. They did not overlap with the 22 positives analyzed using Z-scores. The NIPT-positives increased from 22 (6.91‰) analyzed using Z-scores to 39 (12.25‰) analyzed using Z-scores and maternal CNVs. The consequences of validation by maternal own lymphocytes were consistent (Supplementary Table S1). Among 17 maternal CNVs group, 10 pregnant women chose prenatal diagnosis, of that seven fetuses carried the same CNVs as their mother, indicating that 70% (7/10) CNVs were passed to the next generation. The other seven pregnant women refused prenatal diagnosis. Of two male fetuses undiagnosed exhibited extremely low levels of unconjugated estriol (uE3) during pregnancy, a biochemical indicator of the X-linked ichthyosis (caused by the CNVs), and present skin symptoms after birth; thus we suspected them of having the same CNVs as their mothers. Through genetic counseling, we found that some pregnant women exhibited CNV-related phenotypes in themselves or relatives what they ignored before.
We compared 17 maternal CNVs with their Z-scores to explore the effect of CNV on Z-score. Z-scores with maternal CNVs were different between before and after correction. Z-scores before correction shown that 6 out of 13 autosomes and 2 out of 4 chromosome X had outlier Z-scores. Of maternal CNVs below 2 Mb size, 9 out of 11 had Z-scores before correction in the normal range. Of maternal CNVs above 2Mb, all of 6 were outliers with Z-scores before correction. It is noteworthy that Z-scores after correction were all negative. These CNVs would be missed if only concerned Z-scores.
General Analysis of NIPT-Positive Results Using Z-Scores
Z-score analysis without or maternal CNV analysis, was applied to the cohort of 61,525 pregnancies. Maternal and fetal characteristics, along with positive results, are shown in Table 3. We found outlier Z-scores in 402 pregnancies, and the positive rate was 6.53‰. Abnormalities of Chr21, Chr18, Chr13, SCAs, and other autosomes accounted for 61.03% (214/402), 16.92% (68/402), 7.21% (29/402), 14.18% (57/402), and 8.46% (34/402), respectively. Of the 402 pregnancies, 303 underwent prenatal or postnatal diagnoses, 44 pregnancies were miscarrige or induced labour due to structural abnormalities, and 55 pregnancies were lost to follow-up. PPV for twin pregnancies did not significantly differ from singletons, with 83.33% (5/6) of twin and 70.37% (209/297) of singleton. Overall PPV was 70.20%, of which Chr21, Chr18, sex chromosomes, Chr13, and other autosomes were lower successively with 86.21% (150/174), 66.67% (32/48), 50.00% (20/40), 33.33% (7/21), 25.00% (5/20), respectively.
We found outlier Z-score did not always indicate fetal aneuploidy. In this study, six pregnancies with outlier Z-scores were fetal CNVs verified by prenatal diagnosis, four were mosaic aneuploidies and three false positives were caused by maternal aneuploidies. An extreme outlier Z-scores for ChrY (Z = 362) were discovered in a pregnant woman with a history of bone marrow transplantation.
Accuracy Analysis of Z-Scores for Chr21, Chr18, and Chr13
The Chr21, Chr18, and Chr13 positive pregnancies were divided into six groups according to Z-scores: Z ≤ -3, 3 ≤ Z ≤ 4, 4 < Z ≤ 5, 5 < Z ≤ 6, 6 < Z ≤ 40, and Z > 40 (Table 4). At Z-scores of 3 ≤ Z ≤ 40, PPV increased with increasing Z-scores. At the same Z-score, the PPV of Chr21 was always the highest and that of Chr13, was lowest. Notably, two pregnancies with Z > 40 were both maternal Down syndrome. With Z-scores < -3, fetuses carried microdeletions instead of monosomy. As shown in Table 5, logistic regression analysis revealed that Z-scores were significantly associated with the PPV of T21 (OR = 3.752, p < 0.001) and T18 (OR = 1.532, p = 0.00817). In the ROC curves, AUCs of T21, T18, and T13 were 0.9624, 0.8043, and 0.7436, respectively. S-curves were simulated to predict the trend of PPV shown in Figure 1. We also revealed several special types of karyotype by diagnosis, including Robertsonian translocation, mosaic trisomy, and CNVs; these cases exhibited outlier Z-scores in NIPT.
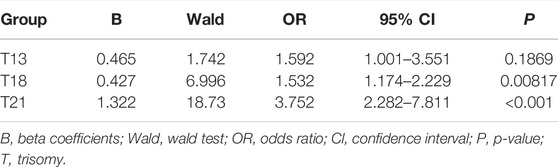
TABLE 5. Correlation between Z-score and Positive Predictive Value (PPV) by Logistic Regression Analysis.
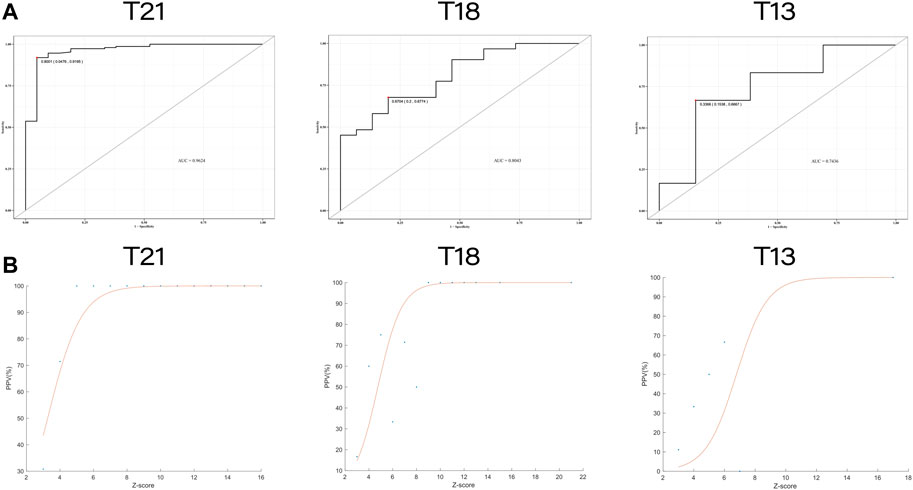
FIGURE 1. The receiver operating characteristic (ROC) curves and trend curves between Z-score and the positive predictive value. (A) The ROC curves of T21, T18, and T13. The area under the curve (AUC) were 0.9624, 0.8043 and 0.7436, respectively. (B) The trend curves of T21, T18 and T13 with S-curve model (
Accuracy Analysis of Z-Scores for Sex Chromosomes and Other Autosomes
In 40 pregnancies with abnormal Z-scores for sex chromosomes, the PPV was 50.00% (20/40) shown in Table 6. Among them, the PPV of sex chromosome trisomy was 64.00% (16/25), and that of monosomy was 26.67% (4/15), with a significant difference (p < 0.05). Five out of 20 for other autosomal abnormalities were true positive (PPV = 25%) diagnosed by SNP-array or CNV-seq technology, including aneuploidies (T16 and mosaic T16) and CNVs on Chr9 and Chr10.
Discussion
Because PPV indicates the possibility of true positive, it is usually used to evaluate the predictive ability of the test (Meck, et al., 2015; DiNonno, et al., 2019). PPV affected by factors such as population size and region, given that it is related to a population’s basic prevalence (Monaghan, et al., 2021).
Previous studies using the ion proton semiconductor platform and the BGISEQ-500 sequencing platform suggested that Z ≥ 9/10 had a higher PPV(Tian et al., 2018; Wan et al., 2021). However, there are potential differences in low Z-scores between different sequencing platforms. In this study, we performed Z-score grouping, logistic regression analysis, ROC curves and S-curve trends to determine correlations between Z-score and PPV. There was the significant correlations between Z-scores and PPV at T21 and T18, with the exception of T13. In addition, we found that the true positives in Z < −3 were all microdeletions instead of monosomy, which was not mentioned before. Because of the diversity in sex chromosomal and other autosomal abnormalities, more data are necessary to increase the accuracy of observed trends.
Several factors affect the accuracy of NIPT. Confined placental mosaicism (CPM), with an incidence of 1–2%, is a common reason (Malvestiti et al., 2015; Mardy et al., 2016). Another reason is maternal chromosomal abnormalities (Wang et al., 2014; Zhou et al., 2017). A study found that maternal CNVs could increase the false positive rate of NIPT by 10% (Snyder et al., 2015). Our study found three pregnancies with Z-scores > 40, were all false positive caused by maternal full aneuploidy. A common cause of false negatives is the low fetal frequency, where the cff-DNA increases as gestational weeks increase. Following previous research, we recollected blood samples in cases of inadequate fetal frequency (Wang et al., 2013). A false-negative case was found in our study due to low fetal frequency (FF=3.8%, Z < 3). Unexpectedly, the fetal frequency reached 9.6% re-collected after two weeks and Z-score of chromosome 21 was 6.66 which indicated that the fetus was T21 positive. The fetus was verified by prenatal diagnosis as 47,XN,+21. For this case, we also used CNV-seq on placental tissue to yield a results of 47,XY,+21, thus excluding CPM shown in Figure 2. Another factor that can interfere with NIPT results is history of transplantation (Zhu et al., 2021). In our study, a pregnant woman received a bone marrow transplant from a male donor 7 years ago. The Z-score of ChrY was 362, far exceeding Z-score from typical male fetuses (Z-score = 3–150). Prenatal diagnosis indicated a nomal fetal karyotype. We further explored cell sources of this pregnant woman’s peripheral blood, oral cavity, and hair follicle cells using STR markers in sex chromosomes, and found different proportions of cell sources. The hair follicle cells were all from the pregnant woman herself, the peripheral blood lymphocytes were all from the bone marrow donor, and the oral cells were the co-existence of the two sources.
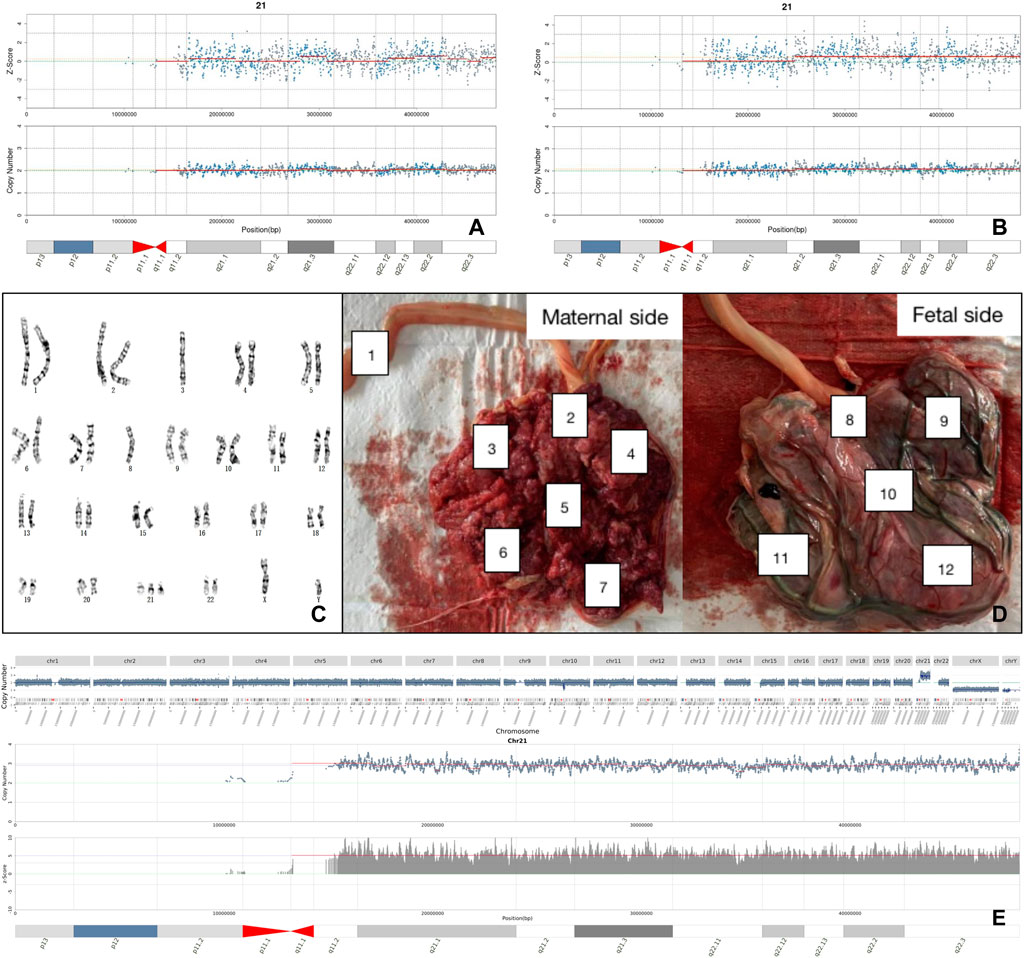
FIGURE 2. Analysis of a false negative case. (A) The negative result of NIPT with first blood sampling. (B) The positive result of NIPT with re-sampling. (C) The karyotype of fetal amniotic fluid cell. (D) Sampling locations of placental tissue after labor induction. (E) Placental CNV-seq results suggested full T21.
Maternal CNVs were seldom researched in NIPT or NIPT-plus technology. NIPT-plus can identify fetal de novo MMS with 0.174% positive rate (Liang et al., 2019). Considering the incidence of pathological CNVs (1.7%) in all pregnancies with normal fetal structures (Wapner et al., 2012), the inherited CNVs were underestimated. By maternal CNV analysis in this study, we found that maternally-inherited CNVs with clinically significant reached 0.28% (9/3184) comprising 7 fetuses with prenatal diagnosis and 2 with specific phenotypes after birth. It was valuable of increasing detection rate of NIPT without increasing testing costs.
Although some pregnant women did not show obvious symptoms due to incomplete penetrance of CNVs, further genetic counseling may reveal mild phenotypes or severe family histories. In our study, the pregnant woman of No. 21J101249 (Figure 3A, I-2) had slight facial asymmetry and communication impairment. Her daughter (II-1) suffered from congenital incomplete cleft palate, as well as mental and physical retardation with a low developmental quotient (DQ = 62). The fetus was induced due to severe cleft lip and palate (Figure 3B). Among I-2, II-1, and II-2, we found an approximately 2.9 Mb heterozygous deletion in 22q11.21-q11.23 (Figure 3C). SMARCB1 gene and 22q11.2 recurrent region (distal type I,D-E/F) in the fragment have sufficient evidence for haploinsufficiency, which are associated with clinical phenotypes such as global developmental delay, intellectual disability, cleft lip, and behavioral problems (Mikhail et al., 2014; Holsten et al., 2018). The pregnant woman of No. 21J108971 (Figure 3D, II-2) had mild schizophrenia; her brother (II-3) exhibited obvious mental retardation, but her mother (I-2) had no obvious abnormality. SNP-array technology revealed that I-2, II-2, and II-3 harbored an approximately 4.4 Mb heterozygous duplication in 15q11.2-q13.1 (Figure 3E), and analysis of fetal amniotic fluid cells (III-1) indicated that the fetus carried the same CNV 15q11.2q13 recurrent (PWS/AS) region (Class 1, BP1-BP3) and 15q11.2q13 recurrent (PWS/AS) region (Class 2,BP2-BP3) in the fragment have sufficient evidence for triplosensitivity, associated with autism, intellectual disability, seizures, and psychiatric disorders (Christian et al., 2008; Ingason et al., 2011). Evidence suggests a parent-of-origin effect, with maternally-derived duplications being more frequently associated with abnormal phenotypes. While the pregnant woman of No. 21J100568 (Figure 3H, I-2) had no obvious abnormality, prenatal diagnosis indicated that the fetus (II-2) carried the same CNV on 16p13.11 (Figure 3G). Additionally her first son suffered from retarded intellectual and language development that had not been detected (II-1) 16p13.11 recurrent region (BP2-BP3) (includes MYH11) in the fragment has sufficient evidence for haploinsufficiency, associated with intellectual disability and/or multiple congenital anomalies (de Kovel et al., 2010; Jähn et al., 2013). It showed sex-limited effect on the penetrance with a significant enrichment among male cases (Tropeano et al., 2013). The fetus had been born for one month without abnormality. The pregnant woman of No. 21J108961 with microdeletion on 17q12 had a renal cyst, congenital abnormal splenic structure, and gallstones but continued her pregnancy without a prenatal diagnosis. The pregnant woman of No. 21J101817 with microdeletion on 4q34.3-q35.2 are less than 150 cm tall despite no obvious developmental delay. The pregnant women of No. 21J101676 and No. 21J104969 had deletions on ChrX (CNVs >10 Mb) which can cause male lethality but no effect on female carriers. The pregnant woman of No.21J105324 and No.21J107686 had microdeletions on Xp22.31 and were pregnant with male fetuses. Inheritance of these CNVs by the male fetuses could result in X-linked ichthyosis caused by haploinsufficiency of STS gene. Additionally, we found that their uE3 was far below normal. studies have shown that a characteristic of ichthyosis is significantly reduced uE3 during the embryonic period (Kashork et al., 2002).
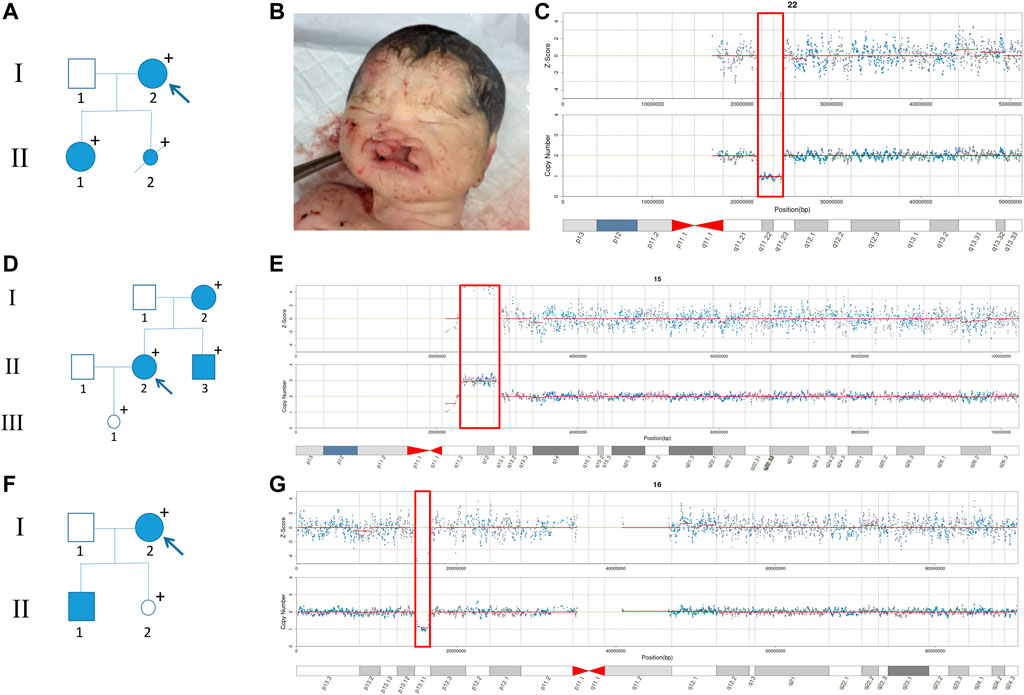
FIGURE 3. Family pedigrees with maternal CNVs. (A) Family pedigrees of No. 21J101249. (B) The facial phenotype of post-induction fetus (No. 21J101249), including cleft lip and palate. (C) Maternal CNV of No. 21J101249 suggested by NIPT. (D) Family pedigrees of No. 21J108971. (E) Maternal CNV of No. 21J108971 suggested by NIPT. (F) Family pedigrees of No. 21J100568. (G) Maternal CNV of No. 21J100568 suggested by NIPT.
The screening act as an early warning for parents regarding pathogenic CNVs that may be passed down to their offspring. Despite the small number of samples, our combined analysis increased NIPT positive rates from, 6.91‰ to 12.25‰ and found 9 clinically significant fetal CNVs which inherited from mothers without increasing detection costs and placing more economic pressure on pregnant women. Therefore, we propose a new NIPT screening model that integrates Z-scores and maternal CNVs (Figure 4).
Data Availability Statement
The datasets presented in this study can be found in online repositories. The names of the repository/repositories and accession number(s) can be found below: NCBI SRA BioProject, accession No:PRJNA837410.
Ethics Statement
The studies involving human participants were reviewed and approved by Medical Ethics Committee of Changzhi Maternal and Child Health Care Hospital. The patients/participants provided their written informed consent to participate in this study. Written informed consent was obtained from the individual(s) for the publication of any potentially identifiable images or data included in this article.
Author Contributions
XL, YA, and LC conceived the study, carried out the assays and participated in the study design. LC, LW, ZH, YT, and WS carried out the laboratory tests, statistical analysis, and followed-up.All authors contributed to the article and approved the submitted version.
Conflict of Interest
The authors declare that the research was conducted in the absence of any commercial or financial relationships that could be construed as a potential conflict of interest.
Publisher’s Note
All claims expressed in this article are solely those of the authors and do not necessarily represent those of their affiliated organizations, or those of the publisher, the editors and the reviewers. Any product that may be evaluated in this article, or claim that may be made by its manufacturer, is not guaranteed or endorsed by the publisher.
Acknowledgments
We thank all the participants for their contribution to this study.
Supplementary Material
The Supplementary Material for this article can be found online at: https://www.frontiersin.org/articles/10.3389/fgene.2022.887176/full#supplementary-material
References
Chen, Y., Yu, Q., Mao, X., Lei, W., He, M., and Lu, W. (2019). Noninvasive Prenatal Testing for Chromosome Aneuploidies and Subchromosomal Microdeletions/microduplications in a Cohort of 42,910 Single Pregnancies with Different Clinical Features. Hum. Genomics. 13, 60. doi:10.1186/s40246-019-0250-2
Chiu, R. W. K., Chan, K. C. A., Gao, Y., Lau, V. Y. M., Zheng, W., Leung, T. Y., et al. (2008). Noninvasive Prenatal Diagnosis of Fetal Chromosomal Aneuploidy by Massively Parallel Genomic Sequencing of DNA in Maternal Plasma. Proc. Natl. Acad. Sci. U.S.A. 105, 20458–20463. doi:10.1073/pnas.0810641105
Christian, S. L., Brune, C. W., Sudi, J., Kumar, R. A., Liu, S., Karamohamed, S., et al. (2008). Novel Submicroscopic Chromosomal Abnormalities Detected in Autism Spectrum Disorder. Biol. Psychiatry. 63, 1111–1117. doi:10.1016/j.biopsych.2008.01.009
de Kovel, C. G., Trucks, H., Helbig, I., Mefford, H. C., Baker, C., Leu, C., et al. (2010). Recurrent Microdeletions at 15q11.2 and 16p13.11 Predispose to Idiopathic Generalized Epilepsies. Brain 133, 23–32. doi:10.1093/brain/awp262
DiNonno, W., Demko, Z., Martin, K., Billings, P., Egbert, M., Zneimer, S., et al. (2019). Quality Assurance of Non-invasive Prenatal Screening (NIPS) for Fetal Aneuploidy Using Positive Predictive Values as Outcome Measures. Jcm 8, 1311. doi:10.3390/jcm8091311
Gil, M. M., Accurti, V., Santacruz, B., Plana, M. N., and Nicolaides, K. H. (2017). Analysis of Cell-free DNA in Maternal Blood in Screening for Aneuploidies: Updated Meta-Analysis. Ultrasound. Obstet. Gynecol. 50, 302–314. doi:10.1002/uog.17484
Gil, M. M., Galeva, S., Jani, J., Konstantinidou, L., Akolekar, R., Plana, M. N., et al. (2019). Screening for Trisomies by cfDNA Testing of Maternal Blood in Twin Pregnancy: Update of the Fetal Medicine Foundation Results and Meta‐analysis. Ultrasound. Obstet. Gynecol. 53, 734–742. doi:10.1002/uog.20284
Grati, F. R., Molina Gomes, D., Ferreira, J. C. P. B., Dupont, C., Alesi, V., Gouas, L., et al. (2015). Prevalence of Recurrent Pathogenic Microdeletions and Microduplications in over 9500 Pregnancies. Prenat. Diagn. 35, 801–809. doi:10.1002/pd.4613
Holsten, T., Bens, S., Oyen, F., Nemes, K., Hasselblatt, M., Kordes, U., et al. (2018). Germline Variants in SMARCB1 and Other Members of the BAF Chromatin-Remodeling Complex Across Human Disease Entities: A Meta-Analysis. Eur. J. Hum. Genet. 26, 1083–1093. doi:10.1038/s41431-018-0143-1
Hu, H., Wang, L., Wu, J., Zhou, P., Fu, J., Sun, J., et al. (2019). Noninvasive Prenatal Testing for Chromosome Aneuploidies and Subchromosomal Microdeletions/microduplications in a Cohort of 8141 Single Pregnancies. Hum. Genomics. 13, 14. doi:10.1186/s40246-019-0198-2
Ingason, A., Kirov, G., Giegling, I., Hansen, T., Isles, A. R., Jakobsen, K. D., et al. (2011). Maternally Derived Microduplications at 15q11-Q13: Implication Of Imprinted Genes in Psychotic Illness. Am. J. Psychiatry 168, 408–417. doi:10.1176/appi.ajp.2010.09111660
Iwarsson, E., Jacobsson, B., Dagerhamn, J., Davidson, T., Bernabé, E., and Heibert Arnlind, M. (2017). Analysis of Cell-free Fetal DNA in Maternal Blood for Detection of Trisomy 21, 18 and 13 in a General Pregnant Population and in a High Risk Population - a Systematic Review and Meta-Analysis. Acta. Obstet. Gynecol. Scand. 96, 7–18. doi:10.1111/aogs.13047
Jähn, J. A., von Spiczak, S., Muhle, H., Obermeier, T., Franke, A., Mefford, H. C., et al. (2014). Iterative Phenotyping of 15q11.2, 15q13.3 and 16p13.11 Microdeletion Carriers in Pediatric Epilepsies. Epilepsy. Res. 108, 109–116. doi:10.1016/j.eplepsyres.2013.10.001
Junhui, W., Ru, L., Qiuxia, Y., Dan, W., Xiuhong, S., Yongling, Z., et al. (2021). Evaluation of the Z‐score Accuracy of Noninvasive Prenatal Testing for Fetal Trisomies 13, 18 and 21 at a Single Center. Prenat. Diagn. 41, 690–696. doi:10.1002/pd.5908
Kashork, C. D., Sutton, V. R., Fonda Allen, J. S., Schmidt, D. E., Likhite, M. L., Potocki, L., et al. (2002). Low or Absent Unconjugated Estriol in Pregnancy: an Indicator for Steroid Sulfatase Deficiency Detectable by Fluorescence In Situ Hybridization and Biochemical Analysis. Prenat. Diagn. 22, 1028–1032. doi:10.1002/pd.466
Levy, B., and Wapner, R. (2018). Prenatal Diagnosis by Chromosomal Microarray Analysis. Fertil. Steril. 109, 201–212. doi:10.1016/j.fertnstert.2018.01.005
Li, X., Wang, L., Yao, Z., Ruan, F., Hu, Z., and Song, W. Clinical Evaluation of Non-invasive Prenatal Screening in 32,394 Pregnancies from Changzhi Maternal and Child Health Care Hospital of Shanxi China. J. Med. Biochem. (in press), 41, 1-6. doi:10.5937/jomb0-33513
Liang, D., Cram, D. S., Tan, H., Linpeng, S., Liu, Y., Sun, H., et al. (2019). Clinical Utility of Noninvasive Prenatal Screening for Expanded Chromosome Disease Syndromes. Genet. Med. 21, 1998–2006. doi:10.1038/s41436-019-0467-4
Lo, Y. M. D., Corbetta, N., Chamberlain, P. F., Rai, V., Sargent, I. L., Redman, C. W., et al. (1997). Presence of Fetal DNA in Maternal Plasma and Serum. Lancet 350, 485–487. doi:10.1016/s0140-6736(97)02174-0
Ma, N., Xi, H., Chen, J., Peng, Y., Jia, Z., Yang, S., et al. (2021). Integrated CNV-Seq, Karyotyping and SNP-Array Analyses for Effective Prenatal Diagnosis of Chromosomal Mosaicism. BMC Med. Genomics 14, 56. doi:10.1186/s12920-021-00899-x
Malvestiti, F., Agrati, C., Grimi, B., Pompilii, E., Izzi, C., Martinoni, L., et al. (2015). Interpreting Mosaicism in Chorionic Villi: Results of a Monocentric Series of 1001 Mosaics in Chorionic Villi with Follow-Up Amniocentesis. Prenat. Diagn. 35, 1117–1127. doi:10.1002/pd.4656
Mardy, A., and Wapner, R. J. (2016). Confined Placental Mosaicism and its Impact on Confirmation of NIPT Results. Am. J. Med. Genet. 172, 118–122. doi:10.1002/ajmg.c.31505
Meck, J. M., Kramer Dugan, E., Matyakhina, L., Aviram, A., Trunca, C., Pineda-Alvarez, D., et al. (2015). Noninvasive Prenatal Screening for Aneuploidy: Positive Predictive Values Based on Cytogenetic Findings. Am. J. Obstetrics Gynecol. 213, e1–214. doi:10.1016/j.ajog.2015.04.001
Mikhail, F. M., Burnside, R. D., Rush, B., Ibrahim, J., Godshalk, R., Rutledge, S. L., et al. (2014). The Recurrent Distal 22q11.2 Microdeletions are Often De Novo and do not Represent a Single Clinical Entity: A Proposed Categorization System. Genet. Med. 16, 92–100. doi:10.1038/gim.2013.79
Monaghan, T. F., Rahman, S. N., Agudelo, C. W., Wein, A. J., Lazar, J. M., Everaert, K., et al. (2021). Foundational Statistical Principles in Medical Research: Sensitivity, Specificity, Positive Predictive Value, and Negative Predictive Value. Medicina 57, 503. doi:10.3390/medicina57050503
Nevado, J., Mergener, R., Palomares-Bralo, M., Souza, K. R., Vallespín, E., Mena, R., et al. (2014). New Microdeletion and Microduplication Syndromes: A Comprehensive Review. Genet. Mol. Biol. 37, 210–219. doi:10.1590/s1415-47572014000200007
Riggs, E. R., Andersen, E. F., Cherry, A. M., Kantarci, S., Kearney, H., Patel, A., et al. (2020). Technical Standards for the Interpretation and Reporting of Constitutional Copy-Number Variants: a Joint Consensus Recommendation of the American College of Medical Genetics and Genomics (ACMG) and the Clinical Genome Resource (ClinGen). Genet. Med. 22, 245–257. doi:10.1038/s41436-019-0686-8
Snyder, M. W., Simmons, L. E., Kitzman, J. O., Coe, B. P., Henson, J. M., Daza, R. M., et al. (2015). Copy-number Variation and False Positive Prenatal Aneuploidy Screening Results. N. Engl. J. Med. 372, 1639–1645. doi:10.1056/NEJMoa1408408
Tian, Y., Zhang, L., Tian, W., Gao, J., Jia, L., and Cui, S. (2018). Analysis of the Accuracy of Z-Scores of Non-invasive Prenatal Testing for Fetal Trisomies 13, 18, and 21 that Employs the Ion Proton Semiconductor Sequencing Platform. Mol. Cytogenet. 11, 49. doi:10.1186/s13039-018-0397-x
Tropeano, M., Ahn, J. W., Dobson, R. J., Breen, G., Rucker, J., Dixit, A., et al. (2013). Male-Biased Autosomal Effect of 16p13.11 Copy Number Variation in Neurodevelopmental Disorders. PLoS One 8, e61365. doi:10.1371/journal.pone.0061365
Wang, E., Batey, A., Struble, C., Musci, T., Song, K., and Oliphant, A. (2013). Gestational Age and Maternal Weight Effects on Fetal Cell-free DNA in Maternal Plasma. Prenat. Diagn. 33, 662–666. doi:10.1002/pd.4119
Wang, Y., Chen, Y., Tian, F., Zhang, J., Song, Z., Wu, Y., et al. (2014). Maternal Mosaicism Is a Significant Contributor to Discordant Sex Chromosomal Aneuploidies Associated with Noninvasive Prenatal Testing. Clin. Chem. 60, 251–259. doi:10.1373/clinchem.2013.215145
Wapner, R. J., Martin, C. L., Levy, B., Ballif, B. C., Eng, C. M., Zachary, J. M., et al. (2012). Chromosomal Microarray versus Karyotyping for Prenatal Diagnosis. N. Engl. J. Med. 367, 2175–2184. doi:10.1056/NEJMoa1203382
Weise, A., Mrasek, K., Klein, E., Mulatinho, M., Llerena, J. C., Hardekopf, D., et al. (2012). Microdeletion and Microduplication Syndromes. J. Histochem Cytochem. 60, 346–358. doi:10.1369/0022155412440001
Zhang, H., Gao, Y., Jiang, F., Fu, M., Yuan, Y., Guo, Y., et al. (2015). Non-invasive Prenatal Testing for Trisomies 21, 18 and 13: Clinical Experience from 146 958 Pregnancies. Ultrasound. Obstet. Gynecol. 45, 530–538. doi:10.1002/uog.14792
Zhou, L., Zhang, B., Liu, J., Shi, Y., Wang, J., and Yu, B. (2021). The Optimal Cutoff Value of Z-Scores Enhances the Judgment Accuracy of Noninvasive Prenatal Screening. Front. Genet. 12, 690063. doi:10.3389/fgene.2021.690063
Zhou, X., Sui, L., Xu, Y., Song, Y., Qi, Q., Zhang, J., et al. (2017). Contribution of Maternal Copy Number Variations to False-Positive Fetal Trisomies Detected by Noninvasive Prenatal Testing. Prenat. Diagn. 37, 318–322. doi:10.1002/pd.5014
Keywords: non-invasive prenatal testing (NIPT), copy number variations (CNVs), aneuploidies, prenatal diagnosis, birth defects, positive predictive value (PPV), z-scores
Citation: Chen L, Wang L, Hu Z, Tao Y, Song W, An Y and Li X (2022) Combining Z-Score and Maternal Copy Number Variation Analysis Increases the Positive Rate and Accuracy in Non-Invasive Prenatal Testing. Front. Genet. 13:887176. doi: 10.3389/fgene.2022.887176
Received: 01 March 2022; Accepted: 02 May 2022;
Published: 02 June 2022.
Edited by:
Cynthia Casson Morton, Brigham and Women’s Hospital, United StatesReviewed by:
Ye Cao, The Chinese University of Hong Kong, ChinaZirui Dong, The Chinese University of Hong Kong, China
Copyright © 2022 Chen, Wang, Hu, Tao, Song, An and Li. This is an open-access article distributed under the terms of the Creative Commons Attribution License (CC BY). The use, distribution or reproduction in other forums is permitted, provided the original author(s) and the copyright owner(s) are credited and that the original publication in this journal is cited, in accordance with accepted academic practice. No use, distribution or reproduction is permitted which does not comply with these terms.
*Correspondence: Yu An, QW55dUBmdWRhbi5lZHUuY24=; Xiaoze Li, bGl4aWFvemU1MjBAMTI2LmNvbQ==