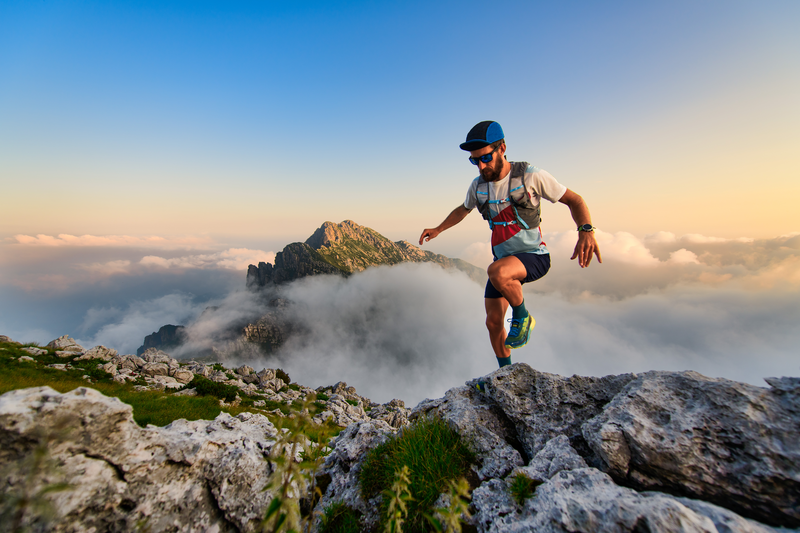
94% of researchers rate our articles as excellent or good
Learn more about the work of our research integrity team to safeguard the quality of each article we publish.
Find out more
ORIGINAL RESEARCH article
Front. Genet. , 30 March 2022
Sec. Livestock Genomics
Volume 13 - 2022 | https://doi.org/10.3389/fgene.2022.861763
This article is part of the Research Topic Sex Control and Sex Differentiation in Aquatic Animals View all 6 articles
Phosphatidylinositol transfer protein (pitp) plays an important role in phospholipid transfer in animals. A pitp variant (pitpβ_w) in Chinese tongue sole was identified by transcriptomic analysis for its female-biased expression. The coding sequence of pitpβ_w was 816 bp, encoding a 371-amino-acid protein. pitpβ_w showed female-biased expression and was relatively high in brain, muscle, and ovary tissues. In different developmental stages of the ovary, pitpβ_w could be detected from 40 days until 3 years post hatching, and the highest expression was observed at 90 days. In situ hybridization revealed that pitpβ_w was predominantly localized in early-stage oocytes (I–III stages). After siRNA-mediated knockdown of pitpβ_w in an ovarian cell line, the expression of sox9a was reduced, while that of figla_tv1 and sox9b was significantly increased. Our findings suggest that pitpβ_w might be involved in female differentiation and early oogenesis.
The gene-encoding phosphatidylinositol transfer protein (pitp) was discovered and named for its role in the transfer of phospholipids between membrane systems and the regulation of phospholipid signal transduction (Helmkamp et al., 1974). pitp is reported to function in various processes, such as neurodegeneration, intestinal absorption diseases, stress response, and developmental regulation (Wirtz, 1991; Cockcroft, 1999). As one of the three types of soluble pitp proteins in multicellular organisms (Hsuan and Cockcroft, 2001), the Pitp beta isoform (Pitpβ) is supposed to maintain the relative level between phosphatidylinositol (PI) and phosphatidylcholine (PC), while its functionality is poorly reported in comparison to the other two soluble members, Pitpα and RdgB (Cockcroft and Garner, 2013). In zebrafish, pitpβ is necessary for maintaining photoreceptor cell segments in the retina (Ile et al., 2010). In rats, pitpβ can be detected with high expression in the embryo and early postnatal stage, coincident with the formation of the nervous system and gray matter development (Utsunomiya et al., 1997). The content in the cells is approximately about 5–10 mmol/L accounting for 0.1% of the total protein in the brain cytoplasm (Hsuan and Cockcroft, 2001). These reports indicated the importance of pitpβ involved in neural development, but it remained unclear whether it was involved in other functions.
In this study, we have conducted a functional analysis of a pitpβ_w gene in Chinese tongue sole. As an economically important fish in Northeast Asia, Chinese tongue sole exhibits obvious sexual growth dimorphism where female individuals can grow 2–4 times faster than males; thus, understanding the sex differentiation mechanism would be pivotal for developing sex control technology and beneficial for the tongue sole industry (Chen et al., 2014). Many genes were reported to show female-biased expression, including figla_tv1, r-spondin 1, lhx9, and zglp1, and their possible role in ovarian development was investigated (Li et al., 2016; Li et al., 2017; Liu et al., 2018; Dong et al., 2019; Zhu et al., 2019). Recently, the study of female differentiation was greatly advanced by the progress of transcriptomic analysis. Lin et al. (2021) identified a number of genes involved in gonad differentiation in the tongue sole, such as the dmrt1, amh, and sox gene family. Combining lncRNA and mRNA transcriptome analyses, Dong et al. revealed several reproduction-related pathways and proposed that two lncRNAs were co-localized with cyp17a1 and cyp19a1 in the follicular cell layer (Dong et al., 2021). In our previous transcriptomic analysis, a set of genes was identified to show female-specific expression and to be involved in female differentiation (Xu et al., 2021). One of these genes, pitpβ_w, localized on the W chromosome, was chosen for further analysis including its expression pattern, cellular localization, and regulatory role in ovarian cell lines. The data would provide important information for understanding the molecular mechanism of sex differentiation in Chinese tongue sole.
The study was performed under the inspection of the committee at the Yellow Sea Fisheries Research Institute. MS-222 was used to anesthetize fish to minimize suffering during the experimental procedure.
All fish used in these experiments were purchased from Huanghai Aquaculture Ltd. (Haiyang, China). To determine the genetic sex of the fish, small samples of the caudal fins were collected and used for genetic sex screening as previously described. In brief, genomic DNA was extracted from the fin and used as a template for PCR reaction. The resultant PCR products were examined by agarose gel electrophoresis. One band was observed for the male sample, while two bands were observed for the female samples (Liu et al., 2014). The muscle, spleen, liver, head kidney, intestine, heart, brain, and ovary were sampled from 1.5 years post hatching (yph) fish (three male and three female individuals). The average body size (BS) and weight (BW) were 21 cm, 45 g for male and 28 cm, 126 g for female. The tissues were immediately frozen with liquid nitrogen and then stored in a −80°C refrigerator until RNA extraction.
At the same time, gonadal samples at different developmental stages, which are 40, 60, and 90 days post hatching (dph), 6 months post hatching (mph), and 1.5 and 3 yph, were extracted and fixed in 4% paraformaldehyde at 4°C for 24 h and then stored in 70% ethanol at −20°C for in situ hybridization. Gonads at 40 and 60 dph were not differentiated, so samples from these two stages were obtained at the latter part of the visceral mass, which were the mixture of gonad, muscle, and other tissues. After genetic sex screening, only gonads from genetic female individuals were used for further analysis. Three individuals were used for analysis, and the average BS and BW for 40, 60, 90 dph, 6 mph, and 1.5 and 3 yph were 3 cm, 0.35 g; 4.5 cm, 0.69 g; 6 cm, 1.45 g; 15.7 cm, 22,4 g; 28 cm, 126 g; and 54 cm, 900 g, respectively.
The coding sequence, molecular weight, and isoelectric point were calculated on http://web.expasy.org/. The protein domain was predicted by SMART (http://smart.embl-heidelberg.de/). A phylogenetic tree was constructed using MEGA 6.0 by employing the maximum likelihood method.
Total RNA was isolated using TRIzol reagent (Invitrogen, Carlsbad, CA) following the manufacturer’s instructions. The total RNA was reverse transcribed into cDNA using PrimeScript™ RT reagent kit (TaKaRa Bio Inc., Otsu, Japan), and gDNA Eraser was added to the resulting product to remove all DNA that might contaminate the genome. qPCR was used to determine the expression level of pitpβ_w in various tissues and at different developmental stages of the ovary. qPCR analysis was performed using a 7500 ABI real-time PCR instrument (Applied Biosystems, United States) with a TAKARA TB Green Premix Ex Taq II kit (TAKARA) following the manufacturer’s instructions. In brief, a 20 μL volume reaction system was prepared, containing 10.0 μL TB Green Premix Ex Tap, 0.4 μL ROX Dye II, 0.4 μL of each sense and anti-sense primer, 1.0 μL cDNA, and 7.8 μL ddH2O. The primers used in this study are shown in Table 1. The amplification procedure consisted of 30 s at 95°C and 40 cycles of 95°C for 5 s and 60°C for 34 s. Then, the default program of melting curve β-actin was used as an internal reference (Li et al., 2010). The transcriptional levels of genes were analyzed by the 2△△Ct method (Livak and Schmittgen, 2001). Using the t test, significant differences were defined when p < 0.05.
In situ hybridization (ISH) was performed with the previously reported method to understand the expression pattern (Kobayashi et al., 2000). In brief, primers were designed for pitpβ_w to amplify 248 bp fragments (Table 1). The T7 binding sequence was included in the primer to facilitate the direct transcription by T7 RNA polymerase and generate digoxin (DIG) labeled probes. Sections were subjected to deparaffination and incubated with probes at 55°C overnight and then blocked for 4 h at room temperature. Anti-DIG-antibodies (Roche) were added for overnight incubation, and the signal was developed using nitrobluetetrazolium/5-bromo-4-chloro-3-indolyl phosphate (Roche, Mannheim, Germany).
Specific ptpβ-siRNA targeting CAGTTGAGTTTAATGTTTCAATG and non-specific siRNA used as a negative control (NC) were synthesized by Sangon Co., Ltd. (Shanghai, China). siRNA-mediated interference was performed according to the reported procedure in the tongue sole ovarian cell line (Sun et al., 2015; Li et al., 2016). In brief, the ovary from adult fish was collected and cut into small pieces (∼1 mm3), which were washed with PBS and then digested with trypsin solution (0.25% trypsin and 0.2% EDTA in PBS) for 10 min. After centrifugation at 180 g for 10 min, the cell pellet was suspended and seeded into a six-well plate at 24°C for 3 days when the cells formed a confluent monolayer. The cells were then subjected to the abovementioned 0.25% trypsin–EDTA solution and cultured for 3 days to allow the formation of a confluent monolayer again. Till date, the ovary cell line has been cultured for over 70 passages. The siRNA was transfected into ovarian cell lines using Lipofectamine 3,000 reagent following the manufacturer’s instructions. The effects at different time periods (24, 48, and 72 h) after interference were compared, and the 48 h sample showed the most significant silencing effect (data not shown). After interference for 48 h, qPCR was conducted to analyze the expression levels of figla_tv1 (KT966740.1), sox9a (NM_001294243.1), sox9b (XM_008315177.3), cyp19a (NM_001294183.1), and insulin-like growth factor (igf1, NM_001294198.1) (primers listed in Table 1). All experiments were performed in triplicate.
As shown in Figure 1, the coding sequence of pitpβ_w was 816 bp (XM_008335237.3), encoding a 271-amino-acid (aa) protein with a predicted molecular weight of 31.52 kDa and an isoelectric point of 6.41. Based on SMART prediction, the protein composed a classic Pitp domain (aa 2-252). In the phylogenetic tree, vertebrate Pitpβ formed two clusters; fish Pitpβ including tongue sole Pitpβ_w formed one cluster, and another vertebrate Pitpβ formed the other cluster (Figure 2).
FIGURE 1. Coding sequence and deduced amino acid sequence of pitpβ_w. Amino acids in sky blue indicate the classic Pitp domain.
FIGURE 2. Phylogenetic analysis of Pitpβ from C. semilaevis and other vertebrates. Numbers at nodes represent NJ bootstrap values. The GenBank accession numbers were as follows: Cynoglossus semilaevis Pitp_w, XP_008333459.1; Cynoglossus semilaevis Pitp_z, XP_008334298.1; Cynoglossus semilaevis Pitpnb, XP_008323858.1; Morone saxatilis, XP_035514806.1; Betta splendens, XP_029019228.1; Toxotes jaculatrix, XP_040898022.1; Siniperca chuatsi, XP_044053196.1; Echeneis naucrates, XP_029366369.1; Mastacembelus armatus, XP_026177048.1; Xenopus tropicalis, NP_989122.1; Gallus gallus, NP_001383302.1; Mus musculus, AAA87593.1; and Homo sapiens, AAH18704.1.
To analyze the tissue distribution of pitpβ_w, qPCR was performed using eight different tissues from 1.5 yph tongue sole. No expression was detected in tissues from males (data not shown). In females, pitpβ_w was detected in all eight examined tissues, with the highest expression in the brain and muscle, followed by the ovary and other tissues (Figure 3A). The female-biased expression led us to analyze its profile at different developmental stages of the ovary. As shown in Figure 3B, pitpβ_w is expressed from 40 dph to 3 yph. The highest expression occurred at 90 dph.
FIGURE 3. Relative expression of pitpβ_w in different tissues from female (A) and different developmental stages of the ovary (B). Expression levels with different letters indicate a significant difference (p < 0.05).
ISH was performed to investigate the cellular localization of pitpβ_w in the ovary. As shown in Figures 4A–C,E–G, the signals could be observed in the oocytes both at 6 mph and 1 yph. However, the signals seemed stronger in early-stage oocytes (I–III stages) than in stage IV (Figure 4G). Sense probes used as a negative control showed no significant signals (Figures 4D,H).
FIGURE 4. Cellular localization of pitpβ_w in the ovaries. (A–C) ISH in the 6 mph ovary with antisense probes corresponding to 40 ×, 100 ×, and 200 × magnification; enlarged areas are highlighted by a red frame; (D) ISH in the 6 mph ovary with sense probes; (E–G) ISH in the 1 yph ovary with antisense probes corresponding to 40ⅹ, 100ⅹ, and 200ⅹ magnification; enlarged areas are highlighted by a red frame; and (H) ISH in the 1 yph ovary with sense probes. Oocytes at different developmental stages are marked by I, II, III, and IV. Scale bars: 100 μm.
An ovarian cell line was employed to assess the pitpβ_w knockdown effect. After siRNA transfection for 48 h, pitpβ_w expression was reduced to approximately 13% (Figure 5A), suggesting an efficient knockdown effect. The sex-related genes were then evaluated, revealing that sox9a was reduced, while figla_tv1 and sox9b were significantly increased (Figure 5B).
FIGURE 5. Expression levels of pitpβ_w and sex-related genes in the ovarian cell line after RNAi for 48 h (A) Expression of pitpβ_w after siRNA. (B) Expression of figla_tv1, igf1, sox9a, sox9b, and cyp19a after RNAi. NC, negative control group. siRNA, rchy1-siRNA-treated group. Asterisks indicate significant differences (*p < 0.05; **p < 0.01).
From the structural perspective, the pitp family can be divided into two classes. Class I contains single-domain proteins (Pitpα, Pitpα, and RdgBβ, all soluble), and class II consists of multiple-domain proteins (RdgBαI and RdgBαII). In Chinese tongue sole, there were five class I members, but no class II members were identified after genomic screening. This observation is similar to that in zebrafish, which has four class I members and one class II member (but lacks the pitp domain) (Elagin et al., 2000; Xie et al., 2005; Ile et al., 2010; Ashlin et al., 2018), suggesting that class I members in fish might be sufficient for phospholipid transfer. In Chinese tongue sole, the five class I members include one pitpα, one rdgBβ, and three pitpβ homologs, namely, pitpβ_w on chromosome W, pitpβ_z (XM_008336076.3) on chromosome Z, and pitpnb (XM_008325636.3) localized on chromosome 14. Although pitpβ_w transcription was specific to females and pitpβ_z and pitpnb transcription could be detected in both males and females (data not shown), their transcripts and amino acids showed high similarity (Supplementary Figure S1). Especially for pitpβ_z and pitpβ_w, there were only 14 amino acid differences at the protein level (271 aa). In tongue sole, we have observed a set of genes with the expression patterns similar to those of pitpβ_w and pitpβ_z. These genes are localized on the Z and W chromosomes, respectively (Xu et al., 2021), producing similar transcripts and proteins but with distinguishable differences, and the transcripts from W chromosome-localized genes are usually female specific. Therefore, it would be interesting to test whether their functionality was redundant or divergent in the future.
Among the classic phosphatidylinositol transfer proteins, pitpβ function has been less studied than pitpα and rdgBβ, and its role in phospholipid transfer seemed dispensable. In this study, pitpβ_w showed female-biased expression, high expression at 90 dph (key stage for gonadal differentiation), and was mainly localized in early-stage oocytes (stages I, II, and III). In Chinese tongue sole, sex determination was proposed at approximately 50 dph, and gonadal differentiation was initiated at 56–62 dph. However, the cellular differentiation is lagging, and cellular differentiation usually occurs in the ovary at 90–120 dph, accompanied by the appearance of the ovarian cavity. Subsequently, the oocyte continues to differentiate and reaches the sexually mature stage at approximately 2 yph (Chen et al., 2014). Thus, high pitpβ_w expression at 90 dph was consistent with cellular differentiation in the ovary. Since there have been few reports regarding the function of pitpβ in sex differentiation, we conducted in vitro experiments to obtain some clues. Knockdown of pitpβ_w in an ovarian cell line resulted in the upregulation of figla_tv1 and sox9b and downregulation of sox9a. As is known, figla_tv1 in fish has been previously suggested to have a conserved function in oogenesis (folliculogenesis) (Kanamori et al., 2008; Yuan et al., 2014; Rouault et al., 2015; Li et al., 2016). In contrast, sox9a in fish is commonly accepted as a key modulator in male differentiation and testicular development (Berbejillo et al., 2012; Adolfi et al., 2015). Thus, we postulate that pitpβ_w might play an important role in female differentiation and early oogenesis by acting as a negative regulator. sox9b was recently identified as a sox9a homologue, and it showed high expression in the gonad of early-stage tongue sole compared to other tissues, so we proposed it was related to gonadal differentiation and, thus, examined its expression pattern after RNAi. cyp19a was not changed after RNAi which was unexpected. The unchanged expression might be due to the following reasons: 1) the rather short-term RNAi could not trigger the cyp19a response, or 2) in vitro experiment could not totally mimic the in vivo condition, so long-term RNAi or in vivo trials should be considered in the future. However, it showed unbiased expression between the testis and ovary, and the function of sox9b needs to be determined. Notably, the expression of pitpβ_w is high in brain and muscle tissues in addition to the ovary. It is possible that pitpβ_w could also function in phosphatidylinositol transfer and neural development (not the research focus in this study), and its regulatory role in ovarian differentiation may be coordinated by participating in the hypothalamic–pituitary–gonadal axis. In the future, we will perform RNAi using a brain cell line to provide a more complete picture. This approach will be ideal for conducting in vivo trials, such as gene editing, to address the remaining unresolved issues (Dong et al., 2021).
The original contributions presented in the study are included in the article/Supplementary Material, further inquiries can be directed to the corresponding author.
The animal study was reviewed and approved by the committee at the Yellow Sea Fisheries Research Institute.
NW and WX conceptualized the data; NW and WX acquired fund; YS, MZ, PC, and ZG conducted investigation; XL and WX administered the project; XX and WX wrote the original draft; and MW performed review and editing.
This work was supported by the National Nature Science Foundation (31730099), the National Key R&D Program of China (2018YFD0900202), the Key Research and Development Project of Shandong Province (2021LZGC028), and the Taishan Scholar Climbing Project Fund of Shandong, China.
XX was employed by the company Vland Biotech Co., Ltd.
The remaining authors declare that the research was conducted in the absence of any commercial or financial relationships that could be construed as a potential conflict of interest.
All claims expressed in this article are solely those of the authors and do not necessarily represent those of their affiliated organizations, or those of the publisher, the editors, and the reviewers. Any product that may be evaluated in this article, or claim that may be made by its manufacturer, is not guaranteed or endorsed by the publisher.
The Supplementary Material for this article can be found online at: https://www.frontiersin.org/articles/10.3389/fgene.2022.861763/full#supplementary-material
Supplementary Figure S1 | Alignment of the three Pitpβ in Chinese tongue sole. Depending on different extents of conservation, the sites are labelled in black, grey, and white.
Adolfi, M. C., Carreira, A. C., Jesus, L. W., Bogerd, J., Funes, R. M., Schartl, M., et al. (2015). Molecular Cloning and Expression Analysis of Dmrt1 and Sox9 during Gonad Development and Male Reproductive Cycle in the Lambari Fish, Astyanax Altiparanae. Reprod. Biol. Endocrinol. 13 (1), 2. doi:10.1186/1477-7827-13-2
Ashlin, T. G., Blunsom, N. J., Ghosh, M., Cockcroft, S., and Rihel, J. (2018). Pitpnc1a Regulates Zebrafish Sleep and Wake Behavior through Modulation of Insulin-like Growth Factor Signaling. Cel Rep. 24 (6), 1389–1396. doi:10.1016/j.celrep.2018.07.012
Berbejillo, J., Martinez-Bengochea, A., Bedó, G., and Vizziano-Cantonnet, D. (2012). Expression of Dmrt1 and Sox9 during Gonadal Development in the Siberian sturgeon (Acipenser baerii). Fish. Physiol. Biochem. 39 (1), 91–94. doi:10.1007/s10695-012-9666-5
Chen, S., Zhang, G., Shao, C., Huang, Q., Liu, G., Zhang, P., et al. (2014). Whole-genome Sequence of a Flatfish Provides Insights into ZW Sex Chromosome Evolution and Adaptation to a Benthic Lifestyle. Nat. Genet. 46 (3), 253–260. doi:10.1038/ng.2890
Cockcroft, S. (1999). Mammalian Phosphatidylinositol Transfer Proteins: Emerging Roles in Signal Transduction and Vesicular Traffic. Chem. Phys. Lipids 98 (1-2), 23–33. doi:10.1016/s0009-3084(99)00015-8
Cockcroft, S., and Garner, K. (2013). Potential Role for Phosphatidylinositol Transfer Protein (PITP) Family in Lipid Transfer during Phospholipase C Signalling. Adv. Biol. Regul. 53 (3), 280–291. doi:10.1016/j.jbior.2013.07.007
Dong, Y., Lyu, L., Zhang, D., Li, J., Wen, H., and Shi, B. (2021). Integrated lncRNA and mRNA Transcriptome Analyses in the Ovary of Cynoglossus Semilaevis Reveal Genes and Pathways Potentially Involved in Reproduction. Front. Genet. 12, 671729. doi:10.3389/fgene.2021.671729
Dong, Z., Zhang, N., Liu, Y., Xu, W., Cui, Z., Shao, C., et al. (2019). Expression Analysis and Characterization of Zglp1 in the Chinese Tongue Sole (Cynoglossus Semilaevis). Gene 683, 72–79. doi:10.1016/j.gene.2018.10.003
Elagin, V. A., Elagina, R. B., Doro, C. J., Vihtelic, T. S., and Hyde, D. R. (2000). Cloning and Tissue Localization of a Novel Zebrafish RdgB Homolog that Lacks a Phospholipid Transfer Domain. Vis. Neurosci. 17 (2), 303–311. doi:10.1017/s095252380017213x
Helmkamp, G. M., Harvey, M. S., Wirtz, K. W. A., and van Deenen, L. L. M. (1974). Phospholipid Exchange between Membranes. J. Biol. Chem. 249 (20), 6382–6389. doi:10.1016/s0021-9258(19)42169-8
Hsuan, J., and Cockcroft, S. (2001). The PITP Family of Phosphatidylinositol Transfer Proteins. Genome Biol. 2 (9), reviews3011. doi:10.1186/gb-2001-2-9-reviews3011
Ile, K. E., Kassen, S., Cao, C., Vihtehlic, T., Shah, S. D., Mousley, C. J., et al. (2010). Zebrafish Class 1 Phosphatidylinositol Transfer Proteins: PITPβ and Double Cone Cell Outer Segment Integrity in Retina. Traffic 11 (9), 1151–1167. doi:10.1111/j.1600-0854.2010.01085.x
Kanamori, A., Toyama, K., Kitagawa, S., Kamehara, A., Higuchi, T., Kamachi, Y., et al. (2008). Comparative Genomics Approach to the Expression of Figα, One of the Earliest Marker Genes of Oocyte Differentiation in Medaka (Oryzias latipes). Gene 423 (2), 180–187. doi:10.1016/j.gene.2008.07.007
Kobayashi, T., Kajiura-Kobayashi, H., and Nagahama, Y. (2000). Differential Expression of Vasa Homologue Gene in the Germ Cells during Oogenesis and Spermatogenesis in a Teleost Fish, tilapia, Oreochromis niloticus. Mech. Dev. 99, 139–142. doi:10.1016/s0925-4773(00)00464-0
Li, Z., Yang, L., Wang, J., Shi, W., Pawar, R. A., Liu, Y., et al. (2010). β-Actin Is a Useful Internal Control for Tissue-specific Gene Expression Studies Using Quantitative Real-Time PCR in the Half-Smooth Tongue Sole Cynoglossus Semilaevis Challenged with LPS or Vibrio Anguillarum. Fish Shellfish Immunol. 29 (1), 89–93. doi:10.1016/j.fsi.2010.02.021
Li, H., Xu, W., Zhang, N., Shao, C., Zhu, Y., Dong, Z., et al. (2016). Two Figla Homologues Have Disparate Functions during Sex Differentiation in Half-Smooth Tongue Sole (Cynoglossus Semilaevis). Sci. Rep. 6 (1), 28219. doi:10.1038/srep28219
Li, H., Xu, W., Zhu, Y., Zhang, N., Ma, J., Sun, A., et al. (2017). Characterization and Expression Pattern of R-Spondin1 in Cynoglossus Semilaevis. J. Exp. Zool. (Mol. Dev. Evol. 328 (8), 772–780. doi:10.1002/jez.b.22774
Lin, G., Gao, D., Lu, J., and Sun, X. (2021). Transcriptome Profiling Reveals the Sexual Dimorphism of Gene Expression Patterns during Gonad Differentiation in the Half-Smooth Tongue Sole (Cynoglossus Semilaevis). Mar. Biotechnol. 23 (1), 18–30. doi:10.1007/s10126-020-09996-x
Liu, Y., Chen, S., Gao, F., Meng, L., Hu, Q., Song, W., et al. (2014). SCAR Transformation of Sex-specific SSR Marker and its Application in Half-Smooth Tongue Sole (Cynoglossus Semiliaevis). J. Agric. Biotechnol. 22, 787–792. doi:10.3969/j.issn.1674-7968.2014.06.015
Liu, J., Liu, T., Niu, J., Wu, X., Zhai, J., Zhang, Q., et al. (2018). Expression Pattern and Functional Analysis of R-Spondin1 in Tongue Sole Cynoglossus Semilaevis. Gene 642, 453–460. doi:10.1016/j.gene.2017.11.047
Livak, K. J., and Schmittgen, T. D. (2001). Analysis of Relative Gene Expression Data Using Real-Time Quantitative PCR and the 2−ΔΔCT Method. Methods 25, 402–408. doi:10.1006/meth.2001.1262
Qiu, Y., Sun, S., Charkraborty, T., Wu, L., Sun, L., Wei, J., et al. (2015). Figla Favors Ovarian Differentiation by Antagonizing Spermatogenesis in a Teleosts, Nile Tilapia (Oreochromis niloticus). Plos One 10 (4), e0123900. doi:10.1371/journal.pone.0123900
Sun, A., Wang, T. Z., Wang, N., Liu, X. F., Sha, Z. X., and Chen, S. L. (2015). Establishment and Characterization of an Ovarian Cell Line from Half-Smooth Tongue Sole Cynoglossus Semilaevis. J. Fish. Biol. 86 (1), 46–59. doi:10.1111/jfb.12535
Utsunomiya, A., Owada, Y., Yoshimoto, T., and Kondo, H. (1997). Localization of Gene Expression for Phosphatidylinositol Transfer Protein in the Brain of Developing and Mature Rats. Mol. Brain Res. 45 (2), 349–352. doi:10.1016/s0169-328x(97)00030-2
Wirtz, K. W. A. (1991). Phospholipid Transfer Proteins. Annu. Rev. Biochem. 60 (1), 73–99. doi:10.1146/annurev.bi.60.070191.000445
Xie, Y., Ding, Y.-Q., Hong, Y., Feng, Z., Navarre, S., Xi, C.-X., et al. (2005). Phosphatidylinositol Transfer Protein-α in Netrin-1-Induced PLC Signalling and Neurite Outgrowth. Nat. Cel Biol 7 (11), 1124–1132. doi:10.1038/ncb1321
Xu, W., Cui, Z., Wang, N., Zhang, M., Wang, J., Xu, X., et al. (2021). Transcriptomic Analysis Revealed Gene Expression Profiles during the Sex Differentiation of Chinese Tongue Sole (Cynoglossus Semilaevis). Comp. Biochem. Physiol. D: Genomics Proteomics 40, 100919. doi:10.1016/j.cbd.2021.100919
Yuan, C., Wu, T., Zhang, Y., Gao, J., Yang, Y., Qin, F., et al. (2014). Responsiveness of Four Gender-specific Genes, Figla, Foxl2, Scp3 and Sox9a to 17α-Ethinylestradiol in Adult Rare Minnow Gobiocypris Rarus. Gen. Comp. Endocrinol. 200, 44–53. doi:10.1016/j.ygcen.2014.02.018
Keywords: Cynoglossus semilaevis, pitpβ_w, female differentiation, oogenesis, RNA interference
Citation: Sun Y, Zhang M, Cheng P, Gong Z, Li X, Wang N, Wei M, Xu X and Xu W (2022) pitpβ_w Encoding Phosphatidylinositol Transfer Protein Is Involved in Female Differentiation of Chinese Tongue Sole, Cynoglossus semilaevis. Front. Genet. 13:861763. doi: 10.3389/fgene.2022.861763
Received: 25 January 2022; Accepted: 24 February 2022;
Published: 30 March 2022.
Edited by:
Qiaomu Hu, Chinese Academy of Fishery Sciences (CAFS), ChinaReviewed by:
Hongyu Ma, Shantou University, ChinaCopyright © 2022 Sun, Zhang, Cheng, Gong, Li, Wang, Wei, Xu and Xu. This is an open-access article distributed under the terms of the Creative Commons Attribution License (CC BY). The use, distribution or reproduction in other forums is permitted, provided the original author(s) and the copyright owner(s) are credited and that the original publication in this journal is cited, in accordance with accepted academic practice. No use, distribution or reproduction is permitted which does not comply with these terms.
*Correspondence: Wenteng Xu, eHV3dEB5c2ZyaS5hYy5jbg==
†These authors have contributed equally to this work
Disclaimer: All claims expressed in this article are solely those of the authors and do not necessarily represent those of their affiliated organizations, or those of the publisher, the editors and the reviewers. Any product that may be evaluated in this article or claim that may be made by its manufacturer is not guaranteed or endorsed by the publisher.
Research integrity at Frontiers
Learn more about the work of our research integrity team to safeguard the quality of each article we publish.