- 1Department of Clinical and Molecular Pathology and Medical Genetics, Faculty of Medicine and University Hospital Ostrava, Ostrava, Czechia
- 2Department of Histology and Embryology, Medical Faculty, Masaryk University, Brno, Czechia
- 3Department of Hematooncology, University Hospital Ostrava, Ostrava, Czechia
- 4Department of Maxillofacial Surgery, University Hospital Ostrava, Ostrava, Czechia
- 5Laboratory of Molecular Morphogenesis, Institute of Animal Physiology and Genetics, Czech Academy of Sciences, Brno, Czechia
- 6Department of Clinical and Molecular Pathology, Faculty of Medicine and University Hospital Olomouc, Olomouc, Czechia
- 7Department of Otorhinolaryngology-Head and Neck Surgery, University of Maryland Medical Center, Baltimore, MD, United States
- 8Marlene and Stewart Greenebaum Comprehensive Cancer Center, University of Maryland Medical Center, Baltimore, MD, United States
- 9Institute for Genome Sciences, University of Maryland Medical Center, Baltimore, MD, United States
- 10Department of Oncology, Sidney Kimmel Comprehensive Cancer Center, Johns Hopkins University, Baltimore, MD, United States
- 11Department of Experimental Biology, Faculty of Science, Masaryk University, Brno, Czechia
Carcinomas of the oral cavity and oropharynx belong among the ten most common malignancies in the human population. The prognosis of head and neck squamous cell carcinoma (HNSCC) is determined by the degree of invasiveness of the primary tumor and by the extent of metastatic spread into regional and distant lymph nodes. Moreover, the level of the perineural invasion itself associates with tumor localization, invasion’s extent, and the presence of nodal metastases. Here, we summarize the current knowledge about different aspects of epigenetic changes, which can be associated with HNSCC while focusing on perineural invasion (PNI). We review epigenetic modifications of the genes involved in the PNI process in HNSCC from the omics perspective and specific epigenetic modifications in OSCC or other neurotropic cancers associated with perineural invasion. Moreover, we summarize DNA methylation status of tumor-suppressor genes, methylation and demethylation enzymes and histone post-translational modifications associated with PNI. The influence of other epigenetic factors on the HNSCC incidence and perineural invasion such as tobacco, alcohol and oral microbiome is overviewed and HPV infection is discussed as an epigenetic factor associated with OSCC and related perineural invasion. Understanding epigenetic regulations of axon growth that lead to tumorous spread or uncovering the molecular control of axon interaction with cancer tissue can help to discover new therapeutic targets for these tumors.
1 Introduction
Squamous cell carcinoma of the head and neck (HNSCC) originates from the mucosal lining of the upper aerodigestive tract, thus including cancers of the oral cavity, paranasal sinuses, nasal cavity, pharynx, and larynx. According to recent estimates, more than 650,000 new cases are diagnosed, and over 350,000 cancer deaths are reported every year worldwide (Torre et al., 2012). From these tumors, oral squamous cell carcinoma (OSCC) represents the most common oral malignancy, demonstrating up to 80–90% of all malignant neoplasms of the oral cavity (Johnson et al., 2000). Despite supporting health education and improving awareness among the general public, and primary care practitioners, many patients are diagnosed in advanced stages of disease (stages III and IV). In addition, the etiology of SCC (squamous cell carcinoma) is typically linked to tobacco and alcohol abuse (Mello et al., 2019). However, emerging evidence revealed an increasing proportion of oropharyngeal tumors caused by human papillomavirus (HPV) infection (Miller and Johnstone, 2001). Therefore, two biologically distinct types of HNSCC (HPV-positive and HPV-negative) can be distinguished. In the United States, HPV-positive oropharyngeal cancer is the fastest rising malignant disease in young white men (Gillison et al., 2019). This alarming trend is expected to expand soon into other economically developed countries (Kreimer et al., 2020).
Several biological and molecular criteria have been established to determine the histopathological staging of SCC, which play a key role in post-surgery treatment and estimation of prognosis of oral and oropharyngeal carcinomas (Woolgar, 2006). Staging and grading are based on the localization and volume of tumor, histopathological grading, histological type of squamous cell carcinoma, positivity of edges, the incidence of regional and distant metastasis, and the other signs of aggressive behavior (perineural invasion, endovascular invasion, etc.). Because the degree of primary tumor invasiveness and extent of metastatic spread into regional and distant lymph nodes closely correlate with the level of PNI, we will focus on PNI in HNSCC and epigenetic regulation of genes contributing to the cancer progression.
2 SCC and Perineural Invasion
Perineural tumor spreading is defined as the ability of tumor cells to penetrate into, around, or through the nerve tissue (Chatzistefanou et al., 2017) (Figure 1). Recently, this process has been described in colorectal carcinoma and salivary gland malignancies (Liebig et al., 2009a; Speight and Barett, 2009b). A possible cause of the perineural spread may lie in the chemotropism of tumor cells that can be stimulated by nerve tissue to grow further. Such interactions also act reciprocally, and cancer cells can induce the growth of the neural tissue. This process increases the invasion of the tumor into surrounding tissue and causes cancer to spread into areas located relatively distant from the primary tumor site.
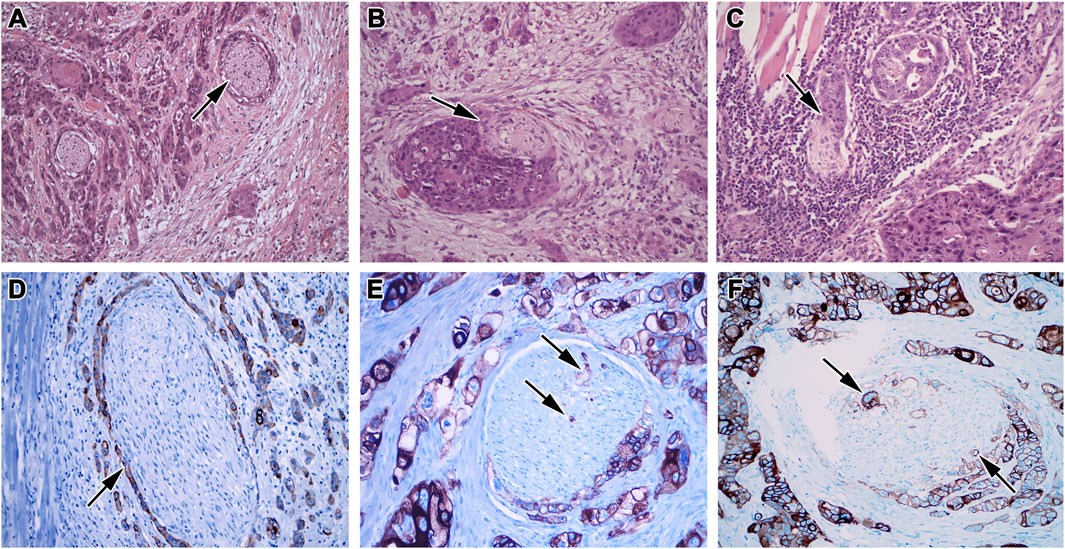
FIGURE 1. Morphology of perineural invasion. (A) Complete circular PNI in the invasive front of the tumor, (B) Partial PNI in more than 30% of the nerve, (C) Partial PNI in less than 30% of the nerve, HE, 200x. (D) Complete circular PNI without intraneural propagation, (E) Partial circular PNI in less than 50% of the nerve with focal intraneural propagation, (F) Partial circular PNI in more than 50% of the nerve with focal intraneural propagation, immunohistochemistry, cytokeratin AE1/A3 (DAB, brown), nuclei (HE, blue), 200x.
Primary cell types involved in PNI are neural Schwann cells (SCs) (Deborde and Wong, 2017), and cancer-associated fibroblasts (Zhang et al., 2019). OSCC is a neurotropic malignancy, and SCs are key mediators and promoters of PNI (Azam and Pecot, 2016; Deborde and Wong, 2017). During interaction with tumorous tissue, their activity recapitulates the normal Schwann cells’ response to peripheral nerve injury, i.e., dynamic transcriptional reprogramming of these cells to active dedifferentiated subtype. It is interesting to note that such reprogramming requires epigenomic regulation through modification of chromatin structure (Ma et al., 2016).
Previously, the invasion was thought to be caused by a mechanical incursion of the tumorous tissue through the relatively thin epineurium tissue (Bockman et al., 1994). However, this concept was rejected after the improved microscopic techniques demonstrated that the tumor cells did not grow passively around the nerves but instead penetrated the perineurium close to Schwann cells and axons up to endoneurium (Gil et al., 2009). Rarely, some less aggressive tumors also develop this nerve-tumor complex at relatively early stages, while others, more aggressive tumors, cause PNI at advanced stages (Chen et al., 2007). One possible explanation is the formation of specific microenvironments in the perineural space, which may contain cellular factors that act on neural tissue and certain types of tumorous tissues. cDNA microarray analysis of adenoid cystic carcinoma samples with and without perineural invasion, deregulation of genes controlling cell cycle, cytoskeleton, and extracellular matrix has been demonstrated. The extracellular matrix also contained increased amounts of neurotrophic factors and adhesive molecules, promoting tumor spreading based on chemotaxis (Liebig et al., 2009b). However, similar analyses have not been performed for HNSCC in relation to PNI yet.
Previously, it was proposed to define PNI as a tumor invasion into the proximity of the nerve comprising 1/3 of the nerve circuit and/or displaying the presence of cancer cells in any of three nerve layers (Fagan et al., 1998). Unfortunately, this classification cannot differentiate between perineural proliferation without infiltration of the nerve fascicle and intraneural propagation or penetration of the cells directly into the nerve, which may reflect a direct clinical impact and affect the patient’s prognosis. This fact also illustrates the need for a more comprehensive examination of regulatory processes, which can potentially lead to distinguishing between these two behaviors and serve as an essential indicator of the tumor aggressiveness, thus giving information about survival prediction and/or probability of the local tumor recurrence.
In clinical settings, the occurrence of perineural invasion among cancer patients usually ranges from 2–30% but can reach up to 82%, depending on the cancer type and the diagnostic methods (O’Brien et al., 1986; Soo et al., 1986; Kurtz et al., 2005). Perineural invasion of squamous cell carcinoma has been reported, but not fully investigated. In clinical trials investigating SCC in the orofacial area, this invasion can be a significant independent factor associated with increased local recurrence of the tumor, metastases, and median patient survival (Sutton et al., 2003; Binmadi and Basile, 2011; Jardim et al., 2015). In the orofacial area, perineural invasion can cause intracranial spreading of the lip carcinoma along the facial or trigeminal nerve branch, thus negatively affecting the patient survival rate (Caldemeyer et al., 1998; Sullivan and Smee, 2006). Previous findings are, however, not consistent as some studies have not found a statistically significant correlation between lymphogenic metastases and perineural invasion and carcinomas in the oral cavity (Wallwork et al., 2007), while other sources confirm this correlation as statistically significant (Woolgar and Scott, 1995; Rahima et al., 2004; Woolgar, 2006). Nevertheless, a relationship was found between the lymphogenic and perineural invasion of T1-T2 carcinomas in the anterior part of the tongue (Larsen et al., 2009). Also, tumor thickness, “non-cohesive invasion front,” neural and bone invasion were described as powerful factors affecting the lymphogenic spreading of the tumor (Ross et al., 2004). Therefore, perineural outgrowth may represent another issue important for clinical practice, particularly in younger patients whose tumors are generally more aggressive, although this has not been fully confirmed in the literature yet.
The interactions between tumor and neural cells are not limited to cell migration and tumor growth from the primary location. Such communication can also stimulate axonogenesis or extent of nerves together with an increase of axon numbers, which can lead to an increase in tissue density around the neural tissue. This process is important in embryonic development and wound healing, representing a physiological status (Aller et al., 2010). Unfortunately, in oncology, axonogenesis facilitates tumor progression, as demonstrated in adenoid cystic carcinoma, which is accompanied by the release of neural growth factors into surrounding tissues. These factors, in turn, stimulated the nervous tissue to neurogenesis, causing nerve elongation, which allowed the tumor to spread further into the tissue (Wang et al., 2009). A similar phenomenon has been demonstrated in the prostate tumor, where the nerve-tumor complex has created a microenvironment stimulating both the nerve and the tumor to grow together (Ayala et al., 2008). Whether the squamous cell carcinoma also similarly affects neurogenesis remains unclear (Binmadi and Basile, 2011).
Perineural invasion is involved in the progression of head and neck squamous cell carcinomas and significantly affects disease prognosis; that is why it is critical to clarify the underlying molecular mechanisms of PNI. However, only a minimal number of molecular studies have been focused on the roles of individual genes in the regulation of the PNI process (Binmadi et al., 2012; Yu et al., 2014; Scanlon et al., 2015; Zhang et al., 2019). To our best knowledge, no one has focused on the role of epigenetic mechanisms potentially involved in regulating genes in cells participating in perineural invasion of HNSCC.
3 Epigenetic Regulations and Their Association with Perineural Invasion
Epigenetics is the research field focused on heritable, reversible changes caused by modulation of gene regulation and expression that do not involve changes to the underlying DNA sequence itself. The word “epigenetic” literally means “above” or “on top of” genetics (Waddington, 1942). Epigenetic processes can lead to altered gene initiation and expression, affecting cell physiology, leading to cancer transformation and progression. Epigenetic changes are dynamic and more frequent than gene mutations and potentially play an important role in determining metastatic phenotypes of cancer cells (Guo et al., 2019). It is widely accepted that transcription and gene regulation is intimately coupled to covalent modifications of the underlying chromatin template. Recently, non-enzymatic covalent modifications (NECMs) by chemically reactive metabolites have been reported to manipulate chromatin architecture and gene transcription (Zheng et al., 2019a; Zheng et al., 2020a). In turn, miRNAs as short non-coding RNAs that regulate gene expression post-transcriptionally, generally bind to the 3′-terminal untranslated region (3′-UTR) of their target mRNAs and repress protein production by destabilizing the mRNA and translational silencing (Ramzan et al., 2021).
In this review, we focus on epigenetic modifications, including classical epigenetic changes affecting DNA methylation and histone modifications and small RNA-mediated processes, particularly those of miRNAs. The link between such epigenetic changes and metastatic dissemination of OSCC by perineural invasion has not been fully explored yet. However, the specific epigenetic alterations have been previously identified in a variety of other malignancies displaying perineural invasion (PNI), such as cutaneous squamous cell carcinoma (Hervás-Marín et al., 2019), pancreatic cancer (Zhang et al., 2020), colorectal cancer (Li et al., 2019), and bile duct cancer (Park et al., 2013).
Epigenetics in oral carcinogenesis can be frequently modulated by endogenous, as well as environmental factors. Among them, tobacco smoking, alcohol consumption, and human papillomavirus (HPV) persistent infection play a significant role (Lleras et al., 2013; Kumar et al., 2015; Degli Esposti et al., 2017; Russo et al., 2020). These factors have already been linked to perineural invasion occurrence in head and neck squamous cell carcinoma (Baumeister et al., 2018; Al Feghali et al., 2019).
Epigenetic changes, such as DNA hypermethylation of tumor-suppressor genes, are abundant for OSCC while there is a limited number of oncogenic cancer-driving genetic abnormalities in this disease. Moreover, PNI represents the major clinicopathological factor affecting the poor prognosis of oral cancers. These facts encourage us to summarize current knowledge on epigenetic abnormalities during PNI.
4 Epigenetic Modifications of the Genes Involved in the Perineural Invasion Process in Head and Neck Squamous Cell Carcinoma from the Omics Perspective
There are multiple cellular and molecular alterations known to occur along with the perineural invasion of tumorous tissues. These changes result in the molecular profiles associated with epithelial-mesenchymal transition, metastasis, and invasion as revealed by single-cell transcriptomics in HNSCC (Zhang et al., 2019). The global expression adjustments can be accompanied by altered activity of epigenetic factors that simultaneously control the expression of many gene sets. Targeted analysis on TGCA dataset using weighted gene co-expression network approach to identify a pool of most important genes highly associated with the presence of perineural invasion revealed the following 12 candidate genes: TIMP2, MIR198, LAMA4, FAM198B, MIR4649, COL5A1, COL1A2, OLFML2B, MMP2, FBN1, ADAM12, and PDGFRB (Zhang et al., 2019). Most of these genes were highly expressed in fibroblasts, with relatively high expression observed in macrophages and endothelial cells (Zhao et al., 2014; Zhang et al., 2019). The epigenetic regulation of those genes in the OSCC patients with perineural invasion is mainly unknown. However, the epigenetic status of many of those core genes was already described in other malignancies (Table 1).
The expression of metalloproteinase tissue inhibitor 2 (TIMP2) was found to play a crucial role in the progression of malignant tumors, was downregulated in many cancers, including OSCC (Chen et al., 2019; Zhang et al., 2019). OSCC cell lines and tissue exhibited reduced expression of TIMP2, which was negatively correlated with CSN6 (COP9 Signalling Complex) (Gao et al., 2020). However, many studies identified high expression of TIMP2 to correlate with poor prognosis (Ruokolainen et al., 2006). This controversy of the results was already previously discussed (Shrestha et al., 2017). Nevertheless, silencing of TIMP2 by the activity of histone methyltransferase EZH2 was found to promote cell invasion and migration in metastatic ovarian cancer (Yi et al., 2017). Interestingly, another hub gene, COL5A1, as a target of EZH2, is upregulated by decreased activity of EZH2 in the breast carcinoma cell line (Kumari et al., 2019). As the expression of EZH2 was found to be increased in HNSCC, and the silencing of EZH2 led to the suppression of tumour invasion (Cao et al., 2012), this protein seems to be a promising target for therapies; however its association with PNI has to be evaluated yet. Moreover, COL1A2 is highly expressed in pancreatic cancer and represents a hallmark protein regulated by miR-25-3p (Wu et al., 2019). Hypomethylation of LAMA4 was identified as a marker of poor prognosis in pancreatic cancer (Zheng et al., 2020b). The relationship between LAMA4 expression and various clinicopathological features, including PNI, was analysed. Nevertheless, PNI was not significantly correlated with LAMA4 upregulation (p = 0.210). Epigenetic regulation of FAM198B is probably only indirect through its stabilization protein CELF2 (Guo et al., 2021). CELF2 is involved in mRNA splicing, and epigenetic loss of CELF2 was associated with a worse prognosis in breast cancer (Piqué et al., 2019). CELF2 was found to be differentially expressed in HNSCC, and its low expression was determined in normal head and neck tissues (Yang et al., 2021). Therefore, CELF2, similar to EZH2, was defined as a negative prognostic marker for HNSCC (Yang et al., 2021); however, the association of this cancer to PNI is still unknown and will need to be validated. For metalloproteinase 2 (MMP2), an epigenetic control by promoter hypomethylation was suggested to be associated with invasive cancer behaviour (Chernov et al., 2009). Similarly, hypomethylation of ADAM12 promoter was linked with worse outcomes in breast cancer (Mendaza et al., 2020).
Interestingly, the expression of the majority (75%) of core genes seemed to be the highest in fibroblasts (Zhang et al., 2019), in agreement with the known fact that cancer-associated fibroblasts are essential for disease progression and their potential role in directing and promoting perineural invasion will also be necessary to evaluate.
5 Specific Epigenetic Modifications in Head and Neck Squamous Cell Carcinoma and Other Neurotropic Cancers Associated with Perineural Invasion
Both DNA and histone modifications play a crucial role in regulating DNA transcriptional activity and chromatin structure. Those DNA and histone modifications are facilitated by epigenetic regulators (DNA methyltransferases, ten-eleven translocation 5-mC hydroxylases, histone acetyltransferases, histone methyltransferases, chromatin-remodeling enzymes, and many more), representing the executors of chromatin remodeling outcomes.
Potential links between DNA and histone modifications, or the expression of epigenetic regulators as well as short non-coding RNA, and the PNI extent have not been addressed in the HNSCC field so far. However, previously uncovered correlation of the altered expression level of epigenetic regulators and perineural invasion found in pancreatic ductal adenocarcinoma (PDA) provided the venue for developing potential therapeutic targeting these enzymes (Silverman and Shi, 2016). Those principles can also be adapted to target epigenetic regulators in PNI positive HNSCC patients.
Here, we focus on individual epigenetic changes in HNSCC and other neurotropic cancers and their possible association with PNI.
5.1 DNA and Histone Epigenetic Modifications Associated with Perineural Invasion
5.1.1 DNA Methylation
In mammals, DNA methylation refers to the covalent transfer of a methyl (-CH3) group to the C5 position of the cytosine ring of DNA by a family of DNA methyltransferases (DNMTs). DNMT family comprises a conserved set of DNA-modifying enzymes DNMT1, DNMT3A, and DNMT3B (Li and Zhang, 2014). Most of DNA methylations in somatic cells occur in the CpG dinucleotide context. The cluster of CpG dinucleotides, referred to as CpG islands, is commonly found near transcription start sites (TSS) of many genes. DNA methylation negatively correlates with gene expression, leading to the transcriptional silencing of genes controlling cancer progression.
As mentioned above, HNSCC is characterized by dysregulation of tumor-suppressor genes, primarily by epigenetic abnormalities (Faraji et al., 2018). CpG islands near TSSs of tumor suppressors are often hypermethylated in the oral squamous cell carcinoma (reviewed in Kim et al., 2019; Hema et al., 2017). DNA methylation status of these OSCC-specific tumor-suppressor genes was not studied specifically concerning PNI in oral cancers. Nevertheless, the differential expression of some of them, such as TFPI2, CDKN2A, CDH1, PTEN, and RUNX3, correlated with PNI in other neurotropic cancers (Supplementary Table S1).
TFPI2 (Tissue factor pathway inhibitor-2), Kunitz-type serine proteinase, and a presumed tumor suppressor gene was associated with PNI in pancreatic carcinoma tissue. TFPI2 expression was strongly negatively correlated (r = −0.460, p < 0.001) with PNI and proposed to be a predictor of a high risk of PNI in pancreatic cancer (Zhai et al., 2015). Moreover, aberrant methylation of the TFPI2 gene is significant in the decrease of TFPI2 expression in human cancers in general (Sierko et al., 2007). However, the association of the methylation status of these genes with PNI in OSCC has not been tested yet.
CDKN2A (cyclin-dependent kinase inhibitor 2A) is ubiquitously expressed in many tissues and cell types and codes for two tumor suppressor proteins. These are transcribed from alternative first exons and therefore translated from different reading frames, one of them being p16INK4a (p16), the other one p14ARF (p14) (Stott et al., 1998). In OSCC, CDKN2A loss of expression was associated with the disease recurrence (Deepak Roshan et al., 2019), and p16 as one of the well-accepted markers of HNSCC prognosis positively correlated with better disease outcomes (reviewed in Augustin et al., 2020). Association of OSCC recurrence and PNI was also tested but determined to be insignificant (Deepak Roshan et al., 2019). Remarkably, methylation of p16INK4a promoter was a more frequent event in prostatic tumors with PNI than without PNI (Verdoodt et al., 2011).
The adhesion molecule and tumor suppressor gene CDH1 (E-cadherin) was found to be frequently inactivated in salivary gland ACC and ACC cell lines through promoter methylation. Tumors with CDH1 promoter methylation exhibited a significantly more PNI than tumors, not demonstrating methylations (Zhang et al., 2007).
Loss of PTEN (phosphatase and tensin homolog), a commonly altered tumor suppressor gene in prostate cancer, was analyzed in prostatic adenocarcinoma specimens from patients who subsequently developed biochemical recurrence. PTEN loss demonstrated a significant correlation with perineural invasion (RR = 24.489, p < 0.001), as compared to wild-type PTEN (Kim et al., 2015). Interestingly, prostate tumors with PTEN loss harbored a distinct epigenome-wide methylation signature, which might mediate tumor progression when PTEN was deleted (Geybels et al., 2017).
The tumor regulatory role of RUNX3 (runt-related transcription factor 3), the Wnt pathway antagonist, in OSCC is still controversial. It was previously identified as a tumor suppressor regulating OSCC cells invasion (Zhou et al., 2017). Moreover, its promoter was found hypermethylated in OSCC (Gao et al., 2009; Supic et al., 2011). However, it may function either as an oncogene or a tumor suppressor gene, based on the data for other tumor types (Otálora-Otálora et al., 2019). Unfortunately, the reduction of this transcription factor has not been studied in relation to PNI in OSCC yet. Nevertheless, the correlation of RUNX3 methylation and PNI was reported in salivary gland adenoid cystic carcinoma (ACC) (Ge et al., 2011), indicating the necessity to analyze RUNX3 methylation in other types of neurotropic cancers.
In addition to tumor suppressor genes, methylation of the growth factor receptors such as TrkA, GFRA1, and TNFRSF10C were identified to play a role in perineural spreading and invasion in some tumors (Supplementary Table S1).
Neurotrophic tyrosine kinase receptor type 1 (NTRK1), also known as tropomyosin receptor kinase A (TrkA), is a high-affinity catalytic receptor for the nerve growth factor (NGF). NGF-TrkA signaling system is thought to be involved in the progression of various cancers (reviewed in Gao et al., 2018) and was suggested to play a role in perineural growth and invasion in HNSCC (Roh et al., 2015). An enhanced expression of the NGF and TrkA in pancreatic cancer was considered to be related to the perineural invasion of cancer cells (Zhu et al., 1999). Immunohistochemical analysis revealed strong TrkA expression in most stage IV pancreatic ductal cancer cells, especially with extensive perineural invasion. Intriguingly, TrkA expression was positively related to the methylation of non-CpG islands around the negatively regulating AP-1-like site in the 5′-untranslated region of the TrkA gene (Fujimoto et al., 2005).
GFRA1 (GDNF family receptor alpha-1) is a cell surface membrane receptor for glial cell-derived neurotrophic factor (GDNF). The GFRA1 gene is normally expressed in neural cells and is overexpressed in many cancer types. Methylation of CpG islands around the GFRA1 transcription start site epigenetically inactivates gene transcription, and demethylation of the locus is essential for gene reactivation. Interestingly, GFRA1 overexpression promotes perineural invasion in pancreatic (Gil et al., 2010) and bile duct cancer cells (Iwahashi et al., 2002).
Higher methylation levels at TNFRSF10C (tumor necrosis factor receptor superfamily member 10C) promoter region in peripheral blood and cancer tissue was positively associated with perineural tumor spread in pancreatic adenocarcinoma (Dauksa et al., 2012); however, it will be necessary to follow the gene expression regulation of this gene in HNSCC patients.
As of today, the only direct correlation of gene methylation with perineural invasion in HNSCC was reported for homeobox protein HOXA1 (Li et al., 2021). High HOXA1 expression was significantly related to DNA methylation decline and PNI (p = 0.0019) (Li et al., 2021). Therefore, HOXA1 was proposed to be a novel biomarker of HNSCC prognosis.
5.1.2 Methylation and Demethylation Enzymes
Modulation of the DNA methylation status may be reflected by the expression changes of the methylation and demethylation enzymes. Such changes in the level and activity of DNMTs and TET (ten-eleven translocation 5-mC hydroxylases) enzymes may contribute to OSCC initiation, progression, and clinical outcome. The gene expression data on DNMTs and TETs concerning PNI have been reported (Table 2).
The mRNA expression of all DNMT family members was upregulated in OSCC (Gaździcka et al., 2020). Thus, upregulation of the DNMT1 gene was confirmed to be an independent marker of relapse-free survival and poor clinical outcome of OSCC patients (Supic et al., 2017).
Overexpression of DNMT3A in OSCC was related to the downregulation of the anti-aging gene Klotho and may be one of the causes of carcinoma in the oral and maxillofacial region. Klotho may serve as a reliable marker for early detection of methylation changes in oral tissues or can be used as a potential target for therapeutic modification in OSCC (Adhikari et al., 2017).
Increased expression of DNMT3B leads to downregulation of E-cadherin, suggesting that changes in OSCC methylation status are involved in the induction of epithelial-mesenchymal transition (EMT) (Chen et al., 2016). Furthermore, in gene expression profile study of salivary ACC associated with PNI, DNMT3B was listed among substantially upregulated genes in the PNI ACC cell group relative to the non-PNI ACC cell group (Chen et al., 2007). Together, these findings agree with the detected E-cadherin promoter methylation in PNI-positive salivary gland ACC discussed in the previous chapter (Chapter 5.1.1 and Zhang et al., 2007).
Importantly, it was shown that targeting DNMTs using epigenetic inhibitors might potentially reverse methylated status and therefore enhance OSCC response to chemotherapy (Suzuki et al., 2009).
The process of active removal of methyl groups is opposite to DNA methylation. The TET enzymes, TET-1, TET-2, and TET-3, play a role as pivotal factors in DNA demethylation. The TET enzymes catalyze the hydroxylation of DNA 5-methylcytosine (5mC) to 5-hydroxymethylcytosine (5hmC) and can alter the regulation of transcription (Rasmussen and Helin, 2016) when molecular oxygen is served as a substrate to convert 5-mC to 5-hmC, and 5-hmC to 5-formylcytosine (5-fC) and 5-carboxycytosine (5-caC) (Ito et al., 2011; Ko et al., 2015). Furthermore, the discovery of 5-hmC, 5-fC, and 5-caC has raised the need to elucidate their function, and it is possible that such oxidized cytosine modifications constitute part of the pathways that lead to active demethylation (Booth et al., 2015).
TET proteins are involved in many important processes, which may influence the development and progression of tumorigenesis (Tan and Shi, 2012). In OSCC, high levels of 5-hmC were correlated with poor overall survival in patients, suggesting the level of demethylation as an important factor for disease prognosis (Wang et al., 2017) and were associated with perineural invasion in pancreatic ductal adenocarcinoma (Chen et al., 2018).
A downregulation of TET-1 expression may lead to enhanced O6-methylguanine-DNA methyltransferase (MGMT) promoter methylation, increasing the sensitivity of OSCC stem cells to chemotherapeutics (Wang et al., 2019). Furthermore, a downregulation of TET-2 correlated with a decreased level of 5-hmC in patients with OSCC (Jäwert et al., 2013). Thus, the reduced abundance of 5-hmC and the depletion of TET-2 expression in OSCC patients may contribute to OSCC development (Wang et al., 2017).
In addition, the multivariate analysis revealed the association of TET-3 gene methylation with poor survival of OSCC patients (Misawa et al., 2018); however, the direct effect of this epigenetic change on perineural invasion initiation in HNSCC patients has not been evaluated yet (Table 2).
5.1.3 Histone Modifications
Histone post-translational modifications, not limited to acetylation, methylation, and phosphorylation, represent distinct types of epigenetic regulation, which can be altered during cancer progression. A high level of H3K27me3 and a low level of H3K4ac were positively correlated with PNI and OSCC tumor stage (Chen et al., 2013). In OSCC tissue and cell lines, H3K27 acetylation promoted cell proliferation and invasion via the activation of the Wnt/βcatenin pathway (Chen et al., 2019). Furthermore, H3K14 was found to be highly acetylated in OSCC tumor patient samples compared to adjacent normal tissue (Arif et al., 2010).
Overexpression of chromatin assembly factor-1, CAF1/p60, which is implicated in incorporating H3K56-acetylated histones into chromatin in response to oxidative stress, predicts the metastasizing behavior of oral cancer (Mascolo et al., 2012).
A low level of H3K4me2 was associated with perineural invasion (p = 0.07) in Asian patients with pancreatic cancer (Watanabe et al., 2012). Potentially, a low level of H3K4me2 may influence PNI in HNSCC patients, but this possibility was not evaluated yet.
5.1.4 Histone Deacetylation Enzymes
Histone deacetylases (HDACs) are involved in various cellular functions, including cell survival and proliferation regulation. Therefore, aberrant expression of HDAC genes is often implicated in tumorigenesis (Hadley et al., 2019); specifically the overexpression of HDACs is usually associated with advanced OSCC (Chang et al., 2009).
The elevation of HDAC2 expression was frequently found in OSCC patients, and univariate analysis confirmed the association of HDAC2 overexpression with shorter overall survival of those patients. This data suggests that the expression level of HDAC2 can serve as a useful prognostic marker for patients with OSCC (Chang et al., 2009).
Higher mRNA and protein expression of HDAC6 were detected in oral cancers, and these changes were associated with a level of tumor aggressiveness (Sakuma et al., 2006).
HDAC8 was found to be overexpressed in OSCC tissues, and HDAC8 silencing significantly inhibited the proliferation of OSCC cells by the induction of apoptosis through caspases activation and pro-survival autophagy (Ahn and Yoon, 2017). In breast cancer tissue, there was a significant association between HDAC8 overexpression and perineural invasion (p < 0.05) (Menbari et al., 2020).
High expression of HDAC9 enhances the development of OSCC by alterations of the cell proliferation, cell cycle, and apoptosis (Rastogi et al., 2016).
Based on these data, chemical inhibition of histone deacetylase members might become a novel therapeutic strategy for OSCC. Indeed, the treatment of OSCC with histone deacetylase inhibitors revealed promising results (Sakuma et al., 2006; Tasoulas et al., 2015; Ahn and Yoon, 2017).
5.2 Short Non-Coding RNAs Associated with Perineural Invasion
MicroRNAs (miRNAs or miRs) are a widespread class of short non-coding RNAs, approximately 18–25 nucleotides in length, which are now recognized as one of the major regulators of gene families expression in eukaryotes (Yao et al., 2019). The roles of miRNAs in epigenetic regulation are complex. However, their primary function lies in the binding to complementary target sequences in mRNA, inducing mRNA decay and interfering with the translational process, thereby preventing or modifying the translation of the protein product. As epigenetic modulators, miRNAs affect the protein levels of the target mRNAs without altering the gene sequences. Moreover, miRNAs themselves can also be epigenetically regulated by DNA methylation and histone modifications of their promoters or by epigenetic modification of the miRNA itself. The reciprocal actions of miRNAs and epigenetic pathways appear to form a miRNA-epigenetic feedback loop (Yao et al., 2019). In miRNA-mediated regulatory networks, one miRNA can regulate many genes, and a single gene can be controlled by many miRNAs (Jonas and Izaurralde, 2015).
miRNA expression patterns have been studied in HNSCC patients and demonstrated to play an essential role in HNSCC pathogenesis. However, not much attention has been paid to the association of specific miRNA expression with PNI in HNSCC (Table 3; Figure 2), in contrast to other tumor types (Zhang et al., 2021). There are more studies focused on the differences in miRNAs expression between PNI positive and negative patients with prostate, pancreatic, colorectal, or gallbladder carcinoma (Prueitt et al., 2008; Liu et al., 2018; Fukada et al., 2020; Sim et al., 2020; Szabo et al., 2020). To the best of our knowledge, a small number of miRNAs have been studied in HNSCC in relation to PNI (Table 3).
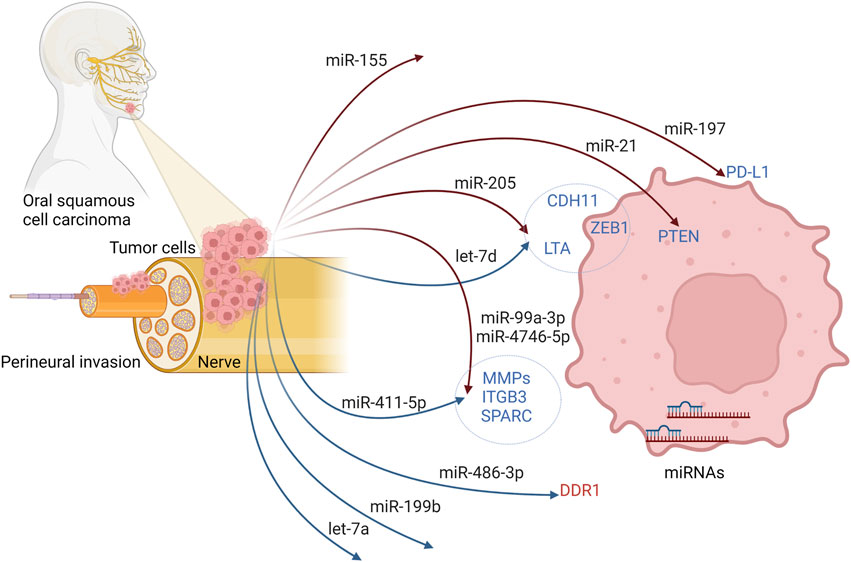
FIGURE 2. Possible regulatory mechanism of miRNAs and their targeted genes to initiate PNI in oral squamous cell carcinoma. Created with BioRender.com.
An extensive effort has been devoted to revealing direct (Yu et al., 2017) and indirect (Reis et al., 2010; Hedbäck et al., 2014; Mahmood et al., 2019) correlation of tumor progression with the miR-21 expression status in OSCC. miR-21 is one of the most studied oncogenic microRNAs in the field of carcinogenesis (Feng and Tsao, 2016), and it represents the most consistently dysregulated microRNA in OSCC (reviewed in Narasimhan and Narasimhan, 2018). Interestingly, high expression of miR-21, reversely correlated with PTEN expression, was related to perineural invasion and was suggested to promote cancer cells to invade the nerve bundle and spread out (Yu et al., 2017).
The effects of altered miR-197 expression on various clinicopathological features in the prognosis of OSCC were identified (Ahn et al., 2017). Increased level of miR-197 was found to be associated with gender, T stage, and PD-L1 (Programmed Death Ligand 1) expression changes. However, the association of miR-197 expression with PNI was not statistically significant (Ahn et al., 2017). In addition, the inverse correlation of miR-486-3p and DDR1 (Discoidin Domain Receptor Tyrosine Kinase 1) expression was reported in OSCC tissues, and clinical analysis uncovered the association of high expression of DDR1 with perineural invasion (Chou et al., 2019).
Increased expression of miR-205 was linked to less aggressive HNSCC tumors with a lower ability of perineural invasion (Kolenda et al., 2019). In that study, the expression of two tumor suppressors and regulators of epithelial-to-mesenchymal transition, let-7d and miR-205, were investigated together. Only high expression of miR-205 significantly inversely correlated with perineural invasion. Moreover, LTA, ZEB1, and CDH11 were found to be down-regulated in the group of patients with low let-7d and high miR-205 compared to patients with high let-7d and low miR-205 expression levels.
In another study with a relatively small group of HNSCC patients, a couple of miRNAs were analyzed in association with lymph node and perineural invasion (Sousa et al., 2016). In that study, the researchers found a significant association of reduced miR-199b levels with PNI (p = 0.040).
Specifically, in HPV16 + HNSCC subtype, miR-99a-3p high, miR-411-5p low, miR-4746-5p high expression phenotype correlated with increased overall survival and less frequent PNI (Zhang et al., 2020). This was probably caused by deregulation of EMT-related signaling and invasion-related genes in miR-99a-3p high, miR-411-5p low, miR-4746-5p high HNSCC patients.
In a broader category of HNSCC, downregulation of let-7a, miRNA involved in stem cell regulation, was significantly associated (p = 0.042) with perineural invasion (Brito et al., 2016).
Recently, a well-known oncogenic miR-155 was reported to be potentially valuable as a novel therapeutic target (Rajan et al., 2021). miR-155 was found to be overexpressed in OSCC patients exhibiting metastases into neck lymph nodes, and correlation of miR-155 expression with perineural invasion was statistically significant (Rajan et al., 2021). Earlier, a product of pre-miR-155 from the 5′ arm, miR-155-5p, was suggested to be a factor of poor disease-free survival rate and poor prognosis in OSCC (Baba et al., 2016). Moreover, in OSCC patients, enhanced overexpression of miR-155 inhibited the expression of the CDC73 gene, promoted cell proliferation, and inhibited apoptosis (Rather et al., 2013).
Several other miRNAs have been studied in oral squamous cell carcinoma cell lines and correlated with more aggressive characteristics and metastasizing behavior of OSCC in general (Table 4; Figure 3). The level of miR-21 was increased in OSCC patients with nodal metastasis (Reis et al., 2010). Its higher expression correlated with the downregulation of Programmed Cell Death 4 (PDCD4), the tumor suppressor genes, and the increased invasive potential of oral carcinoma cells.
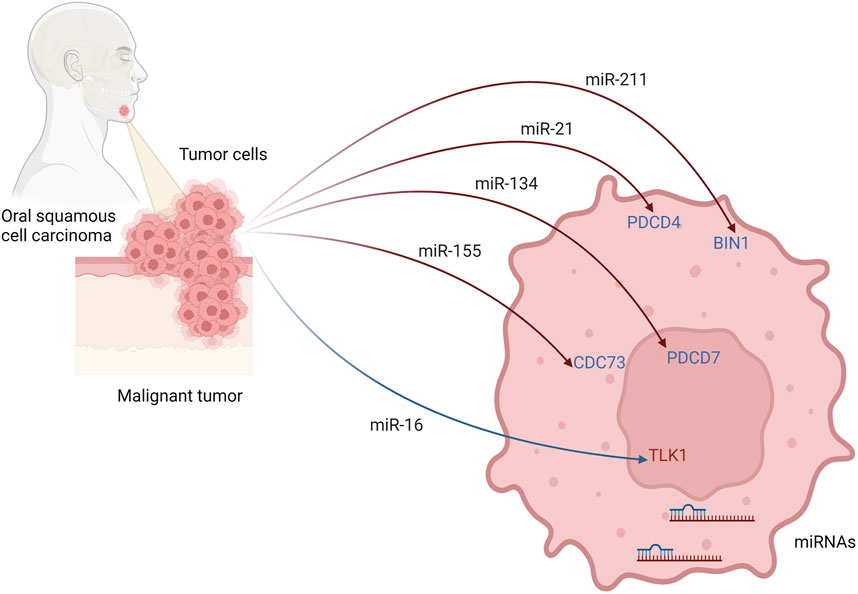
FIGURE 3. Possible regulatory mechanism of miRNAs and their targeted genes to initiate invasive behaviour of oral squamous cell carcinoma. Created with BioRender.com.
The increased expression of oncogenic miR-211 in OSCC correlated with decreased expression of another tumor suppressor gene, bridging integrator-1 (BIN1) (Zheng et al., 2019b). Indeed miR-211-binding was found on the 3′-untranslated region (3′-UTR) of BIN1 mRNA. Moreover, the ectopic overexpression of BIN1 protein in OSCC cell lines was associated with decreased proliferation, cell migration, and invasion (Zheng et al., 2019b).
Furthermore, upregulation of miR-134 was determined in OSCC cell lines and found to target and reduce expression of the PDCD7 gene, which led to OSCC progression (Peng et al., 2018).
Downregulation of miR-16 expression was observed in OSCC patients and cancer cell lines, and this was negatively correlated with enhanced expression of its target gene, Tousled-like kinase 1 (TLK1) (Hu et al., 2018). Moreover, oncogenes AKT3 and BCL2L2, which are responsible for promoting cell proliferation and inhibition of apoptosis in OSCC, have been determined as new target genes for miR-16 with a negative correlation between expression of miR-16 and expression of AKT3 and BCL2L2 (Wang and Li, 2018). Plus, a synergic effect of miR-15a, miR-16, and miR-132 overexpression caused suppression of proliferation, migration, and invasion in pituitary tumors (Renjie and Haiqian, 2015), Decreased expression of miR-638 in OSCC tissue and cells correlated with lymph node metastasis and TMN stages (Tang et al., 2019). Ectopic expression of miR-638 in OSCC cell lines inhibited migration, invasion, and proliferation, while its knockdown had an opposite outcome. miR-638 targets phospholipase D1 (PLD1) and thus may inhibit Wnt/β-catenin pathway (Tang et al., 2019).
Overall, the involvement of microRNAs in the PNI has gained increased attention recently (reviewed in Zhang et al., 2021). The recent data suggest that microRNAs could serve as diagnostic and prognostic markers, as well as a therapeutic target for HNSCC patients.
6 The Influence of Other Epigenetic Factors on the Head and Neck Squamous Cell Carcinoma Incidence and Perineural Invasion
Specific and general epigenetic factors represent risk components for head and neck squamous cell carcinoma, such as tobacco use and alcohol consumption. These addictive substances are independent risk factors but exert synergistic effects when combined. Indeed, there is a behavioral link between cigarette smoking and drinking alcohol; thus, addressing each habit as a separate risk factor in the patient is not always easy. Moreover, exposure to second-hand cigarette smoke is difficult to quantify, leading to patients’ background misclassification.
While HNSCC is traditionally considered a disease of smokers and drinkers, non-smoking and non-drinking patients also develop HNSCC. Another factor that can raise a person’s risk of head and neck cancer is HPV infection. HPV-positive HNSCC represents a distinct group of lesions that vary in their clinical presentation from those caused by classical risk factors (Aggarwal et al., 2020).
Nevertheless, all of the above-listed risk factors can influence or function through epigenetic mechanisms in head and neck squamous cell carcinoma (Colacino et al., 2013; Virani et al., 2015; Degli Esposti et al., 2017; Hema et al., 2017; Ghantous et al., 2018; Jiang et al., 2019; La Rosa et al., 2020). Therefore, it is critical to address whether these mechanisms also contribute to the occurrence of perineural invasion in HNSCC.
6.1 Tobacco as an Epigenetic Factor of Head and Neck Squamous Cell Carcinoma
Various epidemiological studies state that 60–95% of patients with oral squamous cell carcinoma have a personal history of using tobacco products (Llewelyn and Smoking, 1994; Jaber et al., 1999), making tobacco abuse one of the essential factors affecting etiopathogenesis. Tobacco risk factors include complex cigarette smoke, individual chemical components of cigarette smoke, process of cigarette combustion, and the use of non-cigarette tobacco products. It was demonstrated that tobacco in its chewable and smoking form contains a long list of potential carcinogens, cocarcinogens, and tumor promoters. The mechanisms of action of these compounds vary and can evoke several epigenetic changes, including enzymatic hypermethylation of promoter regions of genes ultimately leading to their silencing, altered methylation patterns in gene bodies and introns, alteration of miRNAs and long non-coding RNAs, and changes in histone modifications (reviewed in Centers for Disease Control and Prevention 2010; Ghantous et al., 2018; Choukrallah et al., 2019).
Carcinogens in cigarette smoke are regarded as one of the most potent environmental modifiers of DNA methylation (Breitling et al., 2011). They can give rise to DNA double-stranded breaks. Such damage is repaired by a coordinated action of DNA repair and checkpoint systems, including maintenance DNA methyltransferase 1 (DNMT1). It is recruited to the repair sites to methylate CpGs adjacent to the repaired nucleotides. Cigarette smoke modulates the expression and activity of DNMT1, thus influencing established DNA methylation patterns in cells. Further, nicotine binds to and activates the nicotinic acetylcholine receptors (nAchRs) (Brunzell et al., 2015). nAchRs are found abundantly in the central and peripheral nervous systems. Their activation ultimately leads to downstream activation of cAMP response element-binding protein (CREB), a key transcription factor for many genes (Lenz et al., 2010). Acting possibly via this mechanism, nicotine has been shown to downregulate DNMT1 mRNA and protein expression in neurons in the mouse brain (reviewed in Lee and Pausova, 2013).
Besides DNA methylation, cigarette smoke influences nucleosomal remodeling via histone acetylation, methylation, phosphorylation, and ubiquitination. Further, it can cause abnormal expression of unstable single-stranded miRNAs and lncRNA in mice and humans (Lee and Pausova, 2013). The role of epigenetic factors in tobacco-associated carcinogenesis is further supported by the reversibility of cancer risk after cigarette smoking cessation (Guida et al., 2015).
Only a handful of studies have evaluated the association of tobacco use and PNI in HNSCC patients, and the findings are somewhat inconsistent. Baumeister et al. concluded on the set of 178 HNSCC patients that current and former smokers demonstrated PNI significantly more often than tumors of never smokers (Baumeister et al., 2018). Al Feghali et al. uncovered on the set of 163 patients with oral cavity squamous cell carcinoma that smokers were more likely to have PNI than non-smokers (p < 0.01) (Al Feghali et al., 2019). On the other hand, smoking history was found to be not a risk factor for PNI in 178 patients with HNSCC examined (Zhang et al., 2019). Moreover, smoking history was not associated with perineural invasion in large cohort study of 136 HPV-positive OPSCC (Zebolsky et al., 2021).
Recently, genomic signature analyses and a DNA copy number variations (CNV) analysis of OSCC from patients who self-reported no smoking and drinking habits uncovered epidermal growth factor receptor (EGFR) oncogene amplification as the most common CNV in the examined individuals (Koo et al., 2021). Furthermore, EGFR amplification was significantly associated with PNI and extracapsular spread and non-smoking and non-drinking status as well. Increased EGFR gene copies in OSCC were also positively correlated with PNI in another study (Huang et al., 2012). Interestingly, a network of epigenetic factors that directly control EGFR DNA amplification was identified lately (Clarke et al., 2020). Collectively, these findings suggest that potentially epigenetically modified EGFR oncogene level in PNI positive OSCC might be independent of smoking and/or drinking alcohol.
6.2 Alcohol as an Epigenetic Factor of Oral Squamous Cell Carcinoma
The association between alcohol abuse and the development of OSCC has been previously described and discussed by many authors. The relative risk factor of daily drinking of 100 g of alcohol for SCC development is 6.0 for oral cavity, compared to 4.2 for esophageal and 3.2 for laryngeal cancer (Bagnardi et al., 2001; Morse et al., 2007; Li et al., 2014). Adverse effects of chronic exposure of the oral mucosa to alcohol lead to epithelial atrophy, reduction of the basal layer cell size, and stimulation of stem cells proliferation in the oral epithelium, which, together with chronic inflammation, helps to create an environment favorable for carcinogenesis (Li et al., 2014; Liu et al., 2015).
Alcohol is also transferred by blood into the saliva and subsequently microbially oxidized to acetaldehyde. However, its subsequent transformation into acetate is limited, and acetaldehyde remains in the saliva (Seitz and Stickel, 2007). Further, alcohol-associated acetaldehyde exposure may occur in the oral cavity independently from liver metabolism. Some alcoholic beverages naturally contain acetaldehyde produced by yeasts and acetic acid bacteria and acetaldehyde from coupled auto-oxidation of ethanol and phenolic compounds (Stornetta et al., 2018). In agreement, relatively high acetaldehyde concentration was found in the saliva of OSCC patients who were smokers, alcohol abusers, and displayed poor oral hygiene. Apart from its genotoxic effect, acetaldehyde was shown to cause epigenetic histone modifications in hepatocytes (Shukla et al., 2007) and specific epigenetic modifications in neuroblastoma cell lines (D'Addario et al., 2011). However, a direct effect of the presence of acetaldehyde or ethanol on the development of the oral squamous cell carcinoma or perineural invasion has not been sufficiently proven yet (Salaspuro, 2003; Seitz et al., 2004).
Nevertheless, ethanol-associated modifications of epigenetics have been investigated in OSCC. For example, exposure to ethanol increased acetylation in the H3K9/14 and H3K27 and methylation in H3K27 and H3K9 related to an inferior survival prognosis, increased occurrence of metastases, and OSCC recurrence (Urvalek et al., 2015). In addition, in the patients with higher alcohol consumption, a higher rate of hypermethylation of the promotor region of five tumor-suppressor genes (P16, DAPK, APC, CDH1, and MGMT) was found in association with OSCC (Supic et al., 2009).
In HNSCC patients, miR-30a, miR-934, miR-3164, and miR-3178 were upregulated in oral keratinocytes exposed to ethanol and acetaldehyde. The consequence of miR-30a and miR-934 dysregulation was studied in normal and HNSCC cell lines. Induction of cell proliferation and anti-apoptotic Bcl2 gene expression were detected (Saad et al., 2015). Alcohol-associated changes in lncRNAs were also reported in OSCC. Namely, dysregulation of lnc-PSD4-1 and lnc-NETO1-1 (Yu et al., 2016).
However, a causal link between alcohol and PNI has not been studied yet. To our knowledge, the data taking into account alcohol consumption in PNI positive patients are still limited and controversial. Lee et al. tested prognostic implications for OSCC patients with two distinct forms of perineural invasion, intratumoral (IPNI) and extratumoral (EPNI). In this study, patients with EPNI, compared to patients with IPNI, had a higher prevalence of preoperative alcohol consumption (Lee et al., 2019). However, other studies demonstrated no association of alcohol history with perineural invasion in HNSCC (Zhang et al., 2019) and HPV-positive oropharynx squamous cell carcinoma (Zebolsky et al., 2021).
6.3 Relationship of Alteration in the Oral Microbiome as an Epigenetic Factor with OSCC and Perineural Invasion
Another local factor possibly affecting the carcinogenesis in the head and neck area is the oral microbiome, which is usually associated with pH alteration in the oral cavity. Alcohol and tobacco abuse are two of the most common causes of such microbiome changes (Chattopadhyay et al., 2019). Bacteria Porphyromonas gingivalis and Fusobacterium nucleatum produce inflammatory cytokines, induce cell proliferation, inhibit apoptosis and affect cell migration. These changes in cell behavior can further support processes leading to carcinogenesis in the oral cavity. Bacteria species (e.g., Lactobacillus gasseri and Lactobacillus vaginalis, Fusobacterium nucleatum) can even be used for general oral health screening, diagnosis of early changes in the oral epithelium, and prediction of chemoprevention in OSCC patients (Chattopadhyay et al., 2019).
Information on oral microbial dysbiosis in PNI-positive carcinomas is minimal. However, the association of gut microbiota composition with perineural invasion was discussed in colorectal carcinoma (Kinross et al., 2017; Scott et al., 2019), and a systematic review of metagenomic studies on the oral microbiome in oral cancer indicate the need to test the correlation of perineural invasion with oral microbiome (Su Mun et al., 2021).
6.4 HPV Infection as an Epigenetic Factor Associated With OSCC and Related Perineural Invasion
HPV infection has also been found to induce malignant tumors in the orofacial region (Hübbers and Akgül, 2015). Especially HPV16 expressing viral E6 and E7 oncoproteins inactivate p53 and RB tumor suppressor proteins and thus support the tumorous cell proliferation. Recently, HPV16-positivity has been routinely tested during diagnosis, and patients with HPV16 detected in the tumorous tissue are predicted to respond well to radiotherapy (de Abreu et al., 2018). Moreover, HPV-positive head and neck squamous cell carcinoma cases are less likely to develop PNI than HPV-negative cases (Zhang et al., 2019).
The association of HPV infection with other epigenetic factors has been determined in OSCC (Klussmann et al., 2009; Sartor et al., 2011; Jithesh et al., 2013). Downregulation of methylation in CCNA1 promotor was found in HPV-positive patients with OSCC (Sartor et al., 2011). On the contrary, hypermethylation was proven in CCNA1, DCC, TIMP3, EYA4, and WT1 genes in HPV-positive OSCC (Viet and Schmidt, 2008; Arantes et al., 2015). The methylation status of the HOXA9 gene, encoding a homeobox protein, could even serve as a biomarker for early detection of usually HPV-negative OSCC (Guerrero-Preston et al., 2011). We also found other robustly methylated regions, specific for HPV + OPSCC, such as KCNA3, EMBP1, CCDC181, DPP4, ITGA4, BEND4, ELMO1, SFMBT2, C1QL3, MIR129-2, NID2, HOXB4, ZNF439, ZNF93, VSTM2B, ZNF137P, and ZNF773 (Ren et al., 2018). We have demonstrated the link between the abundant DNA methylation, chromatin modifications, and gene expression for this disease (Guo et al., 2017; Ando et al., 2019; Guo et al., 2020). Moreover, our recent work demonstrated that HPV causes epigenetic deformation at the site of viral integration into the host genome (Kelley et al., 2017). Based on these observations, it was proposed that viral-related tumors have different epigenetic patterns than chemical-related tumors and healthy patients (Faraji et al., 2018).
7 Possible Therapeutic Targets and Recent Clinical Trials
Perineural invasion is widely regarded as an indicator of poor prognosis in oral cancer patients. It significantly correlates with aggressiveness of tumor, disease recurrence, and increased morbidity and mortality. Radiation is usually indicated for patients with head and neck SCC with PNI after resection as adjuvant therapy (Bakst et al., 2019; Bur et al., 2016). Unfortunately, dose selection, risk of catastrophic failure, and possible toxicity to nearby physiological tissues make radiation not always beneficial for patients. Therefore, focusing on the molecular basis of perineural invasion and perineural spread and epigenetic mechanisms playing a role in PNI could enable us to target nerve invasion independently from cancer itself. Such therapy would broaden treatment options for neurotropic cancers. To date, there are no clinical trials focused on PNI; therefore, we summarize epigenetic approaches used in OSCC therapy in general.
As histones are found to be highly hyperacetylated in OSCC patient samples, this implicates a HAT inhibitor as a potential therapeutic molecule. Inhibition of HAT by small molecule inhibitor Hydrazinocurcumin (CTK7A) was proven to substantially reduce the xenografted oral tumor growth in mice (Arif et al., 2010). Also, Zebularine is an inhibitor of DNA methyltransferase, which seems to be effective in OSCC therapy (Jha et al., 2015). In combination with cisplatin, Zebularine promotes cell death via an apoptotic pathway. On the other hand, the combination of Zebularine with 5-fluorouracil minimized the efficiency of Zebularine (Suzuki et al., 2009). Another DNA methyltransferase inhibiting analog, Azacitidine, in combination with Cisplatin, has been used in clinical trials for patients with advanced lung or Head and Neck Cancer and recurrent or metastatic Squamous Cell Carcinoma of the Head and Neck. Unfortunately, both clinical studies have been terminated prematurely due to study enrollment issues (ClinicalTrials.gov Identifier: NCT00901537 and NCT00443261).
Also, histone deacetylase inhibition constitutes an attractive target for the therapy of OSCC. The main benefits of HDAC inhibition consist of promoting the tumor suppressor genes activity and preserving the loose structure of chromatin. One of the most promising results has been shown in a combination of HDAC inhibitor MS-275 with chemotherapeutic agent cisplatin, which leads to the inhibition of malignant tumor behavior (Sato et al., 2006). Furthermore, the treatment of OSCC cell lines by HDAC inhibitor Entinostat resulted in reduced proliferation followed by cell cycle arrest at the G0/G1 phase and substantial apoptosis induction (Marques et al., 2020). The recent study examined the antitumor activity of Apicidin in OSCC in murine models. Apicidin inhibited cell growth through HDAC8 inhibition in vitro and in vivo, indicating that Apicidin may be a new effective therapeutic agent for OSCC (Ahn, 2018).
miRNAs therapeutic approach also has an increased potential in oral cancer administration of the epigenetic profile (Wang and Wu, 2009). The traditional perspective involves introducing exogenous tumor suppressor sequences for their enhanced expression (Saito et al., 2006). Another approach could be to restore miRNAs expression that inhibits the aberrant activity of enzymes under normal conditions, e.g., DNA methyltransferase. The constant issue of this therapeutical approach consists of the non-specificity of these epigenetic modifier drugs because healthy cells could be affected, or previously suppressed oncogenic genes in tumor cells might be activated (Irimie et al., 2018).
8 Conclusion
Here, we summarized the association of epigenetic regulation of genes related to squamous cell carcinoma with special attention on perineural invasion from different points of view, including alterations of DNA and histones modification up to epigenetic factors such as alcohol, smoke, or HPV-positivity and their possible effect on these tumorous cells.
While previously, attention was mainly paid to epigenetic changes in HNSCC, their relation to the perineural invasion was at the edge of the attention. As there is a close relationship between perineural invasion and tumor invasiveness, inhibition of perineural invasion through regulation of epigenetic changes could open new avenues for effective cancer treatment in these patients.
Author Contributions
Study design: ZC, MB, and EH. Original draft writing: PH, ZC, TS, JS, KST, DAG, MB, and EH. Final manuscript inspection: DAG, MB, and EH. All authors contributed to the article and approved the submitted version.
Funding
This research was supported by the Ministry of Health (NV19-08-00383) and by RVO FNO 2018. DG was supported by a Research Scholarship Grant, RSG-21-020-01-MPC from the American Cancer Society and by R01DE027809 from the National Institute of Health.
Conflict of Interest
The authors declare that the research was conducted in the absence of any commercial or financial relationships that could be construed as a potential conflict of interest.
Publisher’s Note
All claims expressed in this article are solely those of the authors and do not necessarily represent those of their affiliated organizations, or those of the publisher, the editors and the reviewers. Any product that may be evaluated in this article, or claim that may be made by its manufacturer, is not guaranteed or endorsed by the publisher.
Supplementary Material
The Supplementary Material for this article can be found online at: https://www.frontiersin.org/articles/10.3389/fgene.2022.848557/full#supplementary-material
References
Adhikari, B. R., Uehara, O., Matsuoka, H., Takai, R., Harada, F., Utsunomiya, M., et al. (2017). Immunohistochemical Evaluation of Klotho and DNA Methyltransferase 3a in Oral Squamous Cell Carcinomas. Med. Mol. Morphol. 50 (3), 155–160. doi:10.1007/s00795-017-0156-9
Aggarwal, N., Yadav, J., Thakur, K., Bibban, R., Chhokar, A., Tripathi, T., et al. (2020). Human Papillomavirus Infection in Head and Neck Squamous Cell Carcinomas: Transcriptional Triggers and Changed Disease Patterns. Front. Cel. Infect. Microbiol. 10, 537650. doi:10.3389/fcimb.2020.537650
Ahn, H., Yang, J. M., Kim, H., Chung, J. H., Ahn, S. H., Jeong, W. J., et al. (2017). Clinicopathologic Implications of the miR-197/PD-L1 axis in Oral Squamous Cell Carcinoma. Oncotarget 8 (39), 66178–66194. doi:10.18632/oncotarget.19842
Ahn, M.-Y., and Yoon, J.-H. (2017). Histone Deacetylase 8 as a Novel Therapeutic Target in Oral Squamous Cell Carcinoma. Oncol. Rep. 37 (1), 540–546. doi:10.3892/or.2016.5280
Ahn, M. Y. (2018). HDAC Inhibitor Apicidin Suppresses Murine Oral Squamous Cell Carcinoma Cell Growth In Vitro and In Vivo via Inhibiting HDAC8 Expression. Oncol. Lett. 16 (5), 6552–6560. doi:10.3892/ol.2018.9468
Al Feghali, K. A., Ghanem, A. I., Burmeister, C., Chang, S. S., Ghanem, T., Keller, C., et al. (2019). Impact of Smoking on Pathological Features in Oral Cavity Squamous Cell Carcinoma. J. Cancer Res. Ther. 15 (3), 582–588. doi:10.4103/jcrt.JCRT_641_16
Aller, M.-A., Arias, J.-I., and Arias, J. (2010). Pathological Axes of Wound Repair: Gastrulation Revisited. Theor. Biol. Med. Model. 7, 37. doi:10.1186/1742-4682-7-37
Ando, M., Saito, Y., Xu, G., Bui, N. Q., Medetgul-Ernar, K., Pu, M., et al. (2019). Chromatin Dysregulation and DNA Methylation at Transcription Start Sites Associated with Transcriptional Repression in Cancers. Nat. Commun. 10 (1), 2188. doi:10.1038/s41467-019-09937-w
Arantes, L. M. R. B., de Carvalho, A. C., Melendez, M. E., Centrone, C. C., Góis-Filho, J. F., Toporcov, T. N., et al. (2015). Validation of Methylation Markers for Diagnosis of Oral Cavity Cancer. Eur. J. Cancer 51 (5), 632–641. doi:10.1016/j.ejca.2015.01.060
Arif, M., Vedamurthy, B. M., Choudhari, R., Ostwal, Y. B., Mantelingu, K., Kodaganur, G. S., et al. (2010). Nitric Oxide-Mediated Histone Hyperacetylation in Oral Cancer: Target for a Water-Soluble HAT Inhibitor, CTK7A. Chem. Biol. 17 (8), 903–913. doi:10.1016/j.chembiol.2010.06.014
Augustin, J. G., Lepine, C., Morini, A., Brunet, A., Veyer, D., Brochard, C., et al. (2020). HPV Detection in Head and Neck Squamous Cell Carcinomas: What Is the Issue? Front. Oncol. 10, 1751. doi:10.3389/fonc.2020.01751
Ayala, G. E., Dai, H., Powell, M., Li, R., Ding, Y., Wheeler, T. M., et al. (2008). Cancer-related Axonogenesis and Neurogenesis in Prostate Cancer. Clin. Cancer Res. 14 (23), 7593–7603. doi:10.1158/1078-0432.ccr-08-1164
Azam, S. H., and Pecot, C. V. (2016). Cancer's Got Nerve: Schwann Cells Drive Perineural Invasion. J. Clin. Invest. 126 (4), 1242–1244. doi:10.1172/jci86801
Baba, O., Hasegawa, S., Nagai, H., Uchida, F., Yamatoji, M., Kanno, N. I., et al. (2016). MicroRNA-155-5p Is Associated with Oral Squamous Cell Carcinoma Metastasis and Poor Prognosis. J. Oral Pathol. Med. 45 (4), 248–255. doi:10.1111/jop.12351
Bagnardi, V., Blangiardo, M., La Vecchia, C., and Corrao, G. (2001). Alcohol Consumption and the Risk of Cancer: a Meta-Analysis. Alcohol. Res. Health 25 (4), 263–270.
Bakst, R. L., Glastonbury, C. M., Parvathaneni, U., Katabi, N., Hu, K. S., and Yom, S. S. (2019). Perineural Invasion and Perineural Tumor Spread in Head and Neck Cancer. Int. J. Radiat. Oncology*Biology*Physics 103 (5), 1109–1124. doi:10.1016/j.ijrobp.2018.12.009
Baumeister, P., Welz, C., Jacobi, C., and Reiter, M. (2018). Is Perineural Invasion of Head and Neck Squamous Cell Carcinomas Linked to Tobacco Consumption? Otolaryngol. Head Neck Surg. 158 (5), 878–881. doi:10.1177/0194599817750354
Binmadi, N. O., and Basile, J. R. (2011). Perineural Invasion in Oral Squamous Cell Carcinoma: a Discussion of Significance and Review of the Literature. Oral Oncol. 47 (11), 1005–1010. doi:10.1016/j.oraloncology.2011.08.002
Binmadi, N. O., Yang, Y.-H., Zhou, H., Proia, P., Lin, Y.-L., Batista De Paula, A. M., et al. (2012). RETRACTED: Plexin-B1 and Semaphorin 4D Cooperate to Promote Perineural Invasion in a RhoA/ROK-dependent Manner. Am. J. Pathol. 180 (3), 1232–1242. doi:10.1016/j.ajpath.2011.12.009
Bockman, D. E., Büchler, M., and Beger, H. G. (1994). Interaction of Pancreatic Ductal Carcinoma with Nerves Leads to Nerve Damage. Gastroenterology 107 (1), 219–230. doi:10.1016/0016-5085(94)90080-9
Booth, M. J., Raiber, E.-A., and Balasubramanian, S. (2015). Chemical Methods for Decoding Cytosine Modifications in DNA. Chem. Rev., 115, 2240PMC4378238–2254. doi:10.1021/cr5002904
Breitling, L. P., Yang, R., Korn, B., Burwinkel, B., and Brenner, H. (2011). Tobacco-smoking-related Differential DNA Methylation: 27K Discovery and Replication. Am. J. Hum. Genet. 88 (4), 450–457. doi:10.1016/j.ajhg.2011.03.003
Brito, B. d. L., Lourenço, S. V., Damascena, A. S., Kowalski, L. P., Soares, F. A., and Coutinho-Camillo, C. M. (2016). Expression of Stem Cell-Regulating miRNAs in Oral Cavity and Oropharynx Squamous Cell Carcinoma. J. Oral Pathol. Med. 45 (9), 647–654. doi:10.1111/jop.12424
Brunzell, D. H., Stafford, A. M., and Dixon, C. I. (2015). Nicotinic Receptor Contributions to Smoking: Insights from Human Studies and Animal Models. Curr. Addict. Rep. 2 (1), 33–46. doi:10.1007/s40429-015-0042-2
Bur, A. M., Lin, A., and Weinstein, G. S. (2016). Adjuvant Radiotherapy for Early Head and Neck Squamous Cell Carcinoma with Perineural Invasion: A Systematic Review. Head Neck 38 Suppl 1 (Suppl. 1), E2350–E2357. doi:10.1002/hed.24295
Caldemeyer, K. S., Mathews, V. P., Righi, P. D., and Smith, R. R. (1998). Imaging Features and Clinical Significance of Perineural Spread or Extension of Head and Neck Tumors. Radiographics 18 (1), 97–110. doi:10.1148/radiographics.18.1.9460111
Cao, W., Feng, Z., Cui, Z., Zhang, C., Sun, Z., Mao, L., et al. (2012). Up-regulation of Enhancer of Zeste Homolog 2 Is Associated Positively with Cyclin D1 Overexpression and Poor Clinical Outcome in Head and Neck Squamous Cell Carcinoma. Cancer 118 (11), 2858–2871. doi:10.1002/cncr.26575
Celentano, A., Yap, T., Paolini, R., Yiannis, C., Mirams, M., Koo, K., et al. (2021). Inhibition of Matrix Metalloproteinase‐2 Modulates Malignant Behaviour of Oral Squamous Cell Carcinoma Cells. J. Oral Pathol. Med. 50 (3), 323–332. doi:10.1111/jop.12992
Centers for Disease Control and Prevention (2010). How Tobacco Smoke Causes Disease: The Biology and Behavioral Basis for Smoking-Attributable Disease: A Report of the Surgeon General. Atlanta (GA): Centers for Disease Control and Prevention.
Chang, H.-H., Chiang, C.-P., Hung, H.-C., Lin, C.-Y., Deng, Y.-T., and Kuo, M. Y.-P. (2009). Histone Deacetylase 2 Expression Predicts Poorer Prognosis in Oral Cancer Patients. Oral Oncol. 45 (7), 610–614. doi:10.1016/j.oraloncology.2008.08.011
Chattopadhyay, I., Verma, M., and Panda, M. (2019). Role of Oral Microbiome Signatures in Diagnosis and Prognosis of Oral Cancer. Technol. Cancer Res. Treat. 18 (1), 1533033819867354–19. doi:10.1177/1533033819867354
Chatzistefanou, I., Lubek, J., Markou, K., and Ord, R. A. (2017). The Role of Perineural Invasion in Treatment Decisions for Oral Cancer Patients: A Review of the Literature. J. Craniomaxillofac. Surg. 45 (6), 821–825. doi:10.1016/j.jcms.2017.02.022
Chen, F., Qi, S., Zhang, X., Wu, J., Yang, X., and Wang, R. (2019). lncRNA PLAC2 Activated by H3K27 Acetylation Promotes Cell Proliferation and Invasion via the Activation of Wnt/β-catenin P-athway in O-ral S-quamous C-ell C-arcinoma. Int. J. Oncol. 54 (4), 1183–1194. doi:10.3892/ijo.2019.4707
Chen, L.-H., Hsu, W.-L., Tseng, Y.-J., Liu, D.-W., and Weng, C.-F. (2016). Involvement of DNMT 3B Promotes Epithelial-Mesenchymal Transition and Gene Expression Profile of Invasive Head and Neck Squamous Cell Carcinomas Cell Lines. BMC Cancer 16, 431. doi:10.1186/s12885-016-2468-x
Chen, W., Zhang, H.-L., Shao, X.-J., Jiang, Y.-G., Zhao, X.-G., Gao, X., et al. (2007). Gene Expression Profile of Salivary Adenoid Cystic Carcinoma Associated with Perineural Invasion. Tohoku J. Exp. Med. 212 (3), 319–334. doi:10.1620/tjem.212.319
Chen, Y.-W., Kao, S.-Y., Wang, H.-J., and Yang, M.-H. (2013). Histone Modification Patterns Correlate with Patient Outcome in Oral Squamous Cell Carcinoma. Cancer 119 (24), 4259–4267. doi:10.1002/cncr.28356
Chen, Y. L., Hu, C. M., Hsu, J. T., Chang, C. C., Huang, T. Y., Chiang, P. H., et al. (2018). Cellular 5-hydroxylmethylcytosine Content Determines Tumorigenic Potential and Prognosis of Pancreatic Ductal Adenocarcinoma. Am. J. Cancer Res. 8 (12), 2548–2563.
Chernov, A. V., Sounni, N. E., Remacle, A. G., and Strongin, A. Y. (2009). Epigenetic Control of the Invasion-Promoting MT1-MMP/MMP-2/TIMP-2 axis in Cancer Cells. J. Biol. Chem. 284 (19), 12727–12734. doi:10.1074/jbc.m900273200
Chou, S.-T., Peng, H.-Y., Mo, K.-C., Hsu, Y.-M., Wu, G.-H., Hsiao, J.-R., et al. (2019). MicroRNA-486-3p Functions as a Tumor Suppressor in Oral Cancer by Targeting DDR1. J. Exp. Clin. Cancer Res. 38 (1), 281. doi:10.1186/s13046-019-1283-z
Choukrallah, M.-A., Sierro, N., Martin, F., Baumer, K., Thomas, J., Ouadi, S., et al. (2019). Tobacco Heating System 2.2 Has a Limited Impact on DNA Methylation of Candidate Enhancers in Mouse Lung Compared with Cigarette Smoke. Food Chem. Toxicol. 123, 501–510. doi:10.1016/j.fct.2018.11.020
Clarke, T. L., Tang, R., Chakraborty, D., Van Rechem, C., Ji, F., Mishra, S., et al. (2020). Histone Lysine Methylation Dynamics ControlEGFRDNA Copy-Number Amplification. Cancer Discov. 10 (2), 306–325. doi:10.1158/2159-8290.cd-19-0463
Colacino, J. A., Dolinoy, D. C., Duffy, S. A., Sartor, M. A., Chepeha, D. B., Bradford, C. R., et al. (2013). Comprehensive Analysis of DNA Methylation in Head and Neck Squamous Cell Carcinoma Indicates Differences by Survival and Clinicopathologic Characteristics. PLoS One 8 (1), e54742. doi:10.1371/journal.pone.0054742
Coppedè, F., Lopomo, A., Spisni, R., and Migliore, L. (2014). Genetic and Epigenetic Biomarkers for Diagnosis, Prognosis and Treatment of Colorectal Cancer. Wjg 20 (4), 943–56. doi:10.3748/wjg.v20.i4.943
D'Addario, C., Johansson, S., Candeletti, S., Romualdi, P., Ögren, S. O., Terenius, L., et al. (2011). Ethanol and Acetaldehyde Exposure Induces Specific Epigenetic Modifications in the Prodynorphin Gene Promoter in a Human Neuroblastoma Cell Line. FASEB j. 25 (3), 1069–1075. doi:10.1096/fj.10-168534
Dauksa, A., Gulbinas, A., Barauskas, G., Pundzius, J., Oldenburg, J., and El-Maarri, O. (2012). Whole Blood DNA Aberrant Methylation in Pancreatic Adenocarcinoma Shows Association with the Course of the Disease: a Pilot Study. PLoS One 7 (5), e37509. doi:10.1371/journal.pone.0037509
de Abreu, P. M., Có, A. C. G., Azevedo, P. L., do Valle, I. B., de Oliveira, K. G., Gouvea, S. A., et al. (2018). Frequency of HPV in Oral Cavity Squamous Cell Carcinoma. BMC Cancer 18 (1), 324. doi:10.1186/s12885-018-4247-3
Deborde, S., and Wong, R. J. (2017). How Schwann Cells Facilitate Cancer Progression in Nerves. Cell. Mol. Life Sci. 74 (24), 4405–4420. doi:10.1007/s00018-017-2578-x
Deepak Roshan, V. G., Sinto, M. S., Vargees, B. T., and Kannan, S. (2019). Loss of CDKN2A and CDKN2B Expression Is Associated with Disease Recurrence in Oral Cancer. J. Oral Maxillofac. Pathol. 23 (1), 82–89. doi:10.4103/jomfp.JOMFP_184_18
Degli Esposti, D., Sklias, A., Lima, S. C., Beghelli-de la Forest Divonne, S., Cahais, V., Fernandez-Jimenez, N., et al. (2017). Unique DNA Methylation Signature in HPV-Positive Head and Neck Squamous Cell Carcinomas. Genome Med. 9 (1), 33. doi:10.1186/s13073-017-0419-z
Fagan, J. J., Collins, B., Barnes, L., D'Amico, F., Myers, E. N., and Johnson, J. T. (1998). Perineural Invasion in Squamous Cell Carcinoma of the Head and Neck. Arch. Otolaryngol. Head Neck Surg. 124, 637–40. doi:10.1001/archotol.124.6.637
Faraji, F., Schubert, A. D., Kagohara, L. T., Tan, M., Xu, Y., Zaidi, M., et al. (2018). “The Genome-wide Molecular Landscape of HPV-Driven and HPV-Negative Head and Neck Squamous Cell Carcinoma,” in Molecular Determinants of Head and Neck Cancer. 2018. Current Cancer Research. Editors B. Burtness, and E. Golemis (Cham: Humana Press).
Feng, B., Wang, K., Herpel, E., Plath, M., Weichert, W., Freier, K., et al. (2021). Prognostic Gene Signature for Squamous Cell Carcinoma with a Higher Risk for Treatment Failure and Accelerated MEK-ERK Pathway Activity. Cancers 13 (20), 5182. doi:10.3390/cancers13205182
Feng, Y.-H., and Tsao, C.-J. (2016). Emerging Role of microRNA-21 in Cancer. Biomed. Rep. 5 (4), 395–402. doi:10.3892/br.2016.747
Franz, M., Wolheim, A., Richter, P., Umbreit, C., Dahse, R., Driemel, O., et al. (2010). Stromal Laminin Chain Distribution in normal, Hyperplastic and Malignant Oral Mucosa: Relation to Myofibroblast Occurrence and Vessel Formation. J. Oral Pathol. Med. 39 (4), 290–8. doi:10.1111/j.1600-0714.2009.00840.x
Fujimoto, M., Kitazawa, R., Maeda, S., and Kitazawa, S. (2005). Methylation Adjacent to Negatively Regulating AP-1 Site Reactivates TrkA Gene Expression during Cancer Progression. Oncogene 24 (32), 5108–5118. doi:10.1038/sj.onc.1208697
Fukada, M., Matsuhashi, N., Takahashi, T., Sugito, N., Heishima, K., Akao, Y., et al. (2020). Tumor Tissue MIR92a and Plasma MIRs21 and 29a as Predictive Biomarkers Associated with Clinicopathological Features and Surgical Resection in a Prospective Study on Colorectal Cancer Patients. Jcm 9 (8), 2509. doi:10.3390/jcm9082509
Gao, F., Griffin, N., Faulkner, S., Rowe, C. W., Williams, L., Roselli, S., et al. (2018). The Neurotrophic Tyrosine Kinase Receptor TrkA and its Ligand NGF Are Increased in Squamous Cell Carcinomas of the Lung. Sci. Rep. 8 (1), 8135. doi:10.1038/s41598-018-26408-2
Gao, F., Huang, C., Lin, M., Wang, Z., Shen, J., Zhang, H., et al. (2009). Frequent Inactivation of RUNX3 by Promoter Hypermethylation and Protein Mislocalization in Oral Squamous Cell Carcinomas. J. Cancer Res. Clin. Oncol. 135 (5), 739–747. doi:10.1007/s00432-008-0508-x
Gao, W. Y., Yang, G., Wang, J., He, J. M., and Wang, P. (2020). CSN6 Promotes Malignant Progression of Oral Squamous Cell Carcinoma by Down-Regulating TIMP-2. Eur. Rev. Med. Pharmacol. Sci. 24 (10), 5419–5428. doi:10.26355/eurrev_202005_21326
Gaździcka, J., Gołąbek, K., Strzelczyk, J. K., and Ostrowska, Z. (2020). Epigenetic Modifications in Head and Neck Cancer. Biochem. Genet. 58 (2), 213–244. doi:10.1007/s10528-019-09941-1
Ge, M. H., Chen, C., Xu, J. J., and Ling, Z. Q. (2011). Unfavorable Clinical Implications for Hypermethylation of RUNX3 in Patients with Salivary Gland Adenoid Cystic Carcinoma. Oncol. Rep. 26 (2), 349–57. doi:10.3892/or.2011.1282
Gentilini, F., Capitani, O., Tinto, D., Rigillo, A., Sabattini, S., Bettini, G., et al. (2020). Assessment of PDGFRβ Promoter Methylation in Canine Osteosarcoma Using Methylation‐sensitive High‐resolution Melting Analysis. Vet. Comp. Oncol. 18 (4), 484–493. doi:10.1111/vco.12567
Geybels, M. S., Fang, M., Wright, J. L., Qu, X., Bibikova, M., Klotzle, B., et al. (2017). PTEN Loss Is Associated with Prostate Cancer Recurrence and Alterations in Tumor DNA Methylation Profiles. Oncotarget 8 (48), 84338–84348. doi:10.18632/oncotarget.20940
Ghantous, Y., Schussel, J. L., and Brait, M. (2018). Tobacco and Alcohol-Induced Epigenetic Changes in Oral Carcinoma. Curr. Opin. Oncol. 30 (3), 152–158. doi:10.1097/cco.0000000000000444
Gil, Z., Carlson, D. L., Gupta, A., Lee, N., Hoppe, B., Shah, J. P., et al. (2009). Patterns and Incidence of Neural Invasion in Patients with Cancers of the Paranasal Sinuses. Arch. Otolaryngol. Head Neck Surg. 135 (2), 173–9. doi:10.1001/archoto.2008.525
Gil, Z., Cavel, O., Kelly, K., Brader, P., Rein, A., Gao, S. P., et al. (2010). Paracrine Regulation of Pancreatic Cancer Cell Invasion by Peripheral Nerves. J. Natl. Cancer Inst. 102 (2), 107–118. doi:10.1093/jnci/djp456
Gillison, M. L., Akagi, K., Xiao, W., Jiang, B., Pickard, R. K. L., Li, J., et al. (2019). Human Papillomavirus and the Landscape of Secondary Genetic Alterations in Oral Cancers. Genome Res. 29 (1), 1–17. doi:10.1101/gr.241141.118
Guerrero-Preston, R., Soudry, E., Acero, J., Orera, M., Moreno-López, L., Macía-Colón, G., et al. (2011). NID2 and HOXA9 Promoter Hypermethylation as Biomarkers for Prevention and Early Detection in Oral Cavity Squamous Cell Carcinoma Tissues and Saliva. Cancer Prev. Res. 4 (7), 1061–1072. doi:10.1158/1940-6207.capr-11-0006
Guida, F., Sandanger, T. M., Castagné, R., Campanella, G., Polidoro, S., Palli, D., et al. (2015). Dynamics of Smoking-Induced Genome-wide Methylation Changes with Time since Smoking Cessation. Hum. Mol. Genet. 24 (8), 2349–2359. doi:10.1093/hmg/ddu751
Guo, M., Peng, Y., Gao, A., Du, C., and Herman, J. G. (2019). Epigenetic Heterogeneity in Cancer. Biomark Res. 7, 23. doi:10.1186/s40364-019-0174-y
Guo, Q., Song, Y., Zhang, H., Wu, X., Xia, P., and Dang, C. (2013). Detection of Hypermethylated Fibrillin-1 in the Stool Samples of Colorectal Cancer Patients. Med. Oncol. 30 (4), 695. doi:10.1007/s12032-013-0695-4
Guo, Q., Wu, Y., Guo, X., Cao, L., Xu, F., Zhao, H., et al. (2021). The RNA-Binding Protein CELF2 Inhibits Ovarian Cancer Progression by Stabilizing FAM198B. Mol. Ther. - Nucleic Acids 23, 169–184. doi:10.1016/j.omtn.2020.10.011
Guo, T., Sakai, A., Afsari, B., Considine, M., Danilova, L., Favorov, A. V., et al. (2017). A Novel Functional Splice Variant of AKT3 Defined by Analysis of Alternative Splice Expression in HPV-Positive Oropharyngeal Cancers. Cancer Res. 77 (19), 5248–5258. doi:10.1158/0008-5472.can-16-3106
Guo, T., Zambo, K. D. A., Zamuner, F. T., Ou, T., Hopkins, C., Kelley, D. Z., et al. (2020). Chromatin Structure Regulates Cancer-specific Alternative Splicing Events in Primary HPV-Related Oropharyngeal Squamous Cell Carcinoma. Epigenetics 15 (9), 959–971. doi:10.1080/15592294.2020.1741757
Hadley, M., Noonepalle, S., Banik, D., and Villagra, A. (2019). “Functional Analysis of HDACs in Tumorigenesis,” in Protein Acetylatio Methods in Molecular Biology. Editor R. BroshJr. (New York, NY: Humana), 1983. doi:10.1007/978-1-4939-9434-2_17
Hedbäck, N., Jensen, D. H., Specht, L., Fiehn, A.-M. K., Therkildsen, M. H., Friis-Hansen, L., et al. (2014). MiR-21 Expression in the Tumor Stroma of Oral Squamous Cell Carcinoma: an Independent Biomarker of Disease Free Survival. PLoS One 9 (4), e95193. doi:10.1371/journal.pone.0095193
Hema, K., Smitha, T., Sheethal, H., and Mirnalini, S. (2017). Epigenetics in Oral Squamous Cell Carcinoma. J. Oral Maxillofac. Pathol. 21 (2), 252–259. doi:10.4103/jomfp.jomfp_150_17
Hervás-Marín, D., Higgins, F., Sanmartín, O., López-Guerrero, J. A., Bañó, M. C., Igual, J. C., et al. (2019). Genome Wide DNA Methylation Profiling Identifies Specific Epigenetic Features in High-Risk Cutaneous Squamous Cell Carcinoma. PLoS One 14 (12), e0223341. doi:10.1371/journal.pone.0223341
Hu, S., Wang, H., and Yan, D. (2018a) Loss of miR-16 Contributes to Tumor Progression by Activation of Tousled-like Kinase 1 in Oral Squamous Cell Carcinoma. Cell Cycle 17:2284–2295.doi:10.1080/15384101.2018.1526601
Huang, S. F., Cheng, S. D., Chien, H. T., Liao, C. T., Chen, I. H., Wang, H. M., et al. (2012). Relationship between Epidermal Growth Factor Receptor Gene Copy Number and Protein Expression in Oral Cavity Squamous Cell Carcinoma. Oral Oncol. 48 (1), 67–72. doi:10.1016/j.oraloncology.2011.06.511
Huang, W., Li, G., Wang, Z., Zhou, L., Yin, X., Yang, T., et al. (2021). A Ten-N6-Methyladenosine (m6A)-Modified Gene Signature Based on a Risk Score System Predicts Patient Prognosis in Rectum Adenocarcinoma. Front. Oncol. 10, 567931. doi:10.3389/fonc.2020.567931
Hübbers, C. U., and Akgül, B. (2015). HPV and Cancer of the Oral Cavity. Virulence 6 (3), 244–8. doi:10.1080/21505594.2014.999570
Irimie, A. I., Ciocan, C., Gulei, D., Mehterov, N., Atanasov, A. G., Dudea, D., et al. (2018). Current Insights into Oral Cancer Epigenetics. Int. J. Mol. Sci. 19 (3), 670. doi:10.3390/ijms19030670
Ito, S., Shen, L., Dai, Q., Wu, S. C., Collins, L. B., Swenberg, J. A., et al. (2011). Tet Proteins Can Convert 5-methylcytosine to 5-formylcytosine and 5-carboxylcytosine. Science 333 (6047), 1300–3. doi:10.1126/science.1210597
Iwahashi, N., Nagasaka, T., Tezel, G., Iwashita, T., Asai, N., Murakumo, Y., et al. (2002). Expression of Glial Cell Line-Derived Neurotrophic Factor Correlates with Perineural Invasion of Bile Duct Carcinoma. Cancer 94 (1), 167–74. doi:10.1002/cncr.10169
Jaber, M. A., Porter, S. R., Gilthorpe, M. S., Bedi, R., and Scully, C. (1999). Risk Factors for Oral Epithelial Dysplasia-Tthe Role of Smoking and Alcohol. Oral Oncol. 35 (2), 151–6. doi:10.1016/s1368-8375(98)00106-7
Jardim, J. F., Francisco, A. L., Gondak, R., Damascena, A., and Kowalski, L. P. (2015). Prognostic Impact of Perineural Invasion and Lymphovascular Invasion in Advanced Stage Oral Squamous Cell Carcinoma. Int. J. Oral Maxillofac. Surg. 44 (1), 23–8. doi:10.1016/j.ijom.2014.10.006
Jäwert, F., Hasséus, B., Kjeller, G., Magnusson, B., Sand, L., and Larsson, L. (2013). Loss of 5-hydroxymethylcytosine and TET2 in Oral Squamous Cell Carcinoma. Anticancer Res. 33 (10), 4325–8.
Jha, M., Aggarwal, R., Jha, A. K., and Shrivastava, A. (2015). Natural Compounds: DNA Methyltransferase Inhibitors in Oral Squamous Cell Carcinoma. Appl. Biochem. Biotechnol. 177 (3), 577–94. doi:10.1007/s12010-015-1768-y
Jiang, C., Liu, F., Xiao, S., He, L., Wu, W., and Zhao, Q. (2021). miR-29a-3p Enhances the Radiosensitivity of Oral Squamous Cell Carcinoma Cells by Inhibiting ADAM12. Eur. J. Histochem. 65 (3), 3295. doi:10.4081/ejh.2021.3295
Jiang, X., Wu, J., Wang, J., and Huang, R. (2019). Tobacco and Oral Squamous Cell Carcinoma: A Review of Carcinogenic Pathways. Tob. Induc Dis. 17, 29. doi:10.18332/tid/105844
Jithesh, P. V., Risk, J. M., Schache, A. G., Dhanda, J., Lane, B., Liloglou, T., et al. (2013). The Epigenetic Landscape of Oral Squamous Cell Carcinoma. Br. J. Cancer 108, 370–379. doi:10.1038/bjc.2012.568
Johnson, N. W., Jayasekara, P., and Amarasinghe, A. A. (2011). Squamous Cell Carcinoma and Precursor Lesions of the Oral Cavity: Epidemiology and Aetiology. Periodontol 57 (1), 19–37. doi:10.1111/j.1600-0757.2011.00401.x
Jonas, S., and Izaurralde, E. (2015). Towards a Molecular Understanding of microRNA-Mediated Gene Silencing. Nat. Rev. Genet. 16 (7), 421–33. doi:10.1038/nrg3965
Kartha, V. K., Stawski, L., Han, R., Haines, P., Gallagher, G., Noonan, V., et al. (2016). PDGFRβ Is a Novel Marker of Stromal Activation in Oral Squamous Cell Carcinomas. PLoS One 11 (4), e0154645. doi:10.1371/journal.pone.0154645
Kelley, D. Z., Flam, E. L., Izumchenko, E., Danilova, L. V., Wulf, H. A., Guo, T., et al. (2017). Integrated Analysis of Whole-Genome ChIP-Seq and RNA-Seq Data of Primary Head and Neck Tumor Samples Associates HPV Integration Sites with Open Chromatin Marks. Cancer Res. 77 (23), 6538–6550. doi:10.1158/0008-5472.can-17-0833
Kim, S. H., Kim, S. H., Joung, J. Y., Lee, G. K., Hong, E. K., Kang, K. M., et al. (2015). Overexpression of ERG and Wild-type PTEN Are Associated with Favorable Clinical Prognosis and Low Biochemical Recurrence in Prostate Cancer. PLoS One 10 (4), e0122498. doi:10.1371/journal.pone.0122498
Kim, S. Y., Han, Y. K., Song, J. M., Lee, C. H., Kang, K., Yi, J. M., et al. (2019). Aberrantly Hypermethylated Tumor Suppressor Genes Were Identified in Oral Squamous Cell Carcinoma (OSCC). Clin. Epigenetics 11 (1), 116. doi:10.1186/s13148-019-0715-0
Kinross, J., Mirnezami, R., Alexander, J., Brown, R., Scott, A., Galea, D., et al. (2017). A Prospective Analysis of Mucosal Microbiome-Metabonome Interactions in Colorectal Cancer Using a Combined MAS 1HNMR and Metataxonomic Strategy. Sci. Rep. 7 (1), 8979. doi:10.1038/s41598-017-08150-3
Klussmann, J. P., Mooren, J. J., Lehnen, M., Claessen, S. M., Stenner, M., Huebbers, C. U., et al. (2009). Genetic Signatures of HPV-Related and Unrelated Oropharyngeal Carcinoma and Their Prognostic Implications. Clin. Cancer Res. 15 (5), 1779–86. doi:10.1158/1078-0432.ccr-08-1463
Ko, M., An, J., Pastor, W. A., Koralov, S. B., Rajewsky, K., and Rao, A. (2015). TET Proteins and 5-methylcytosine Oxidation in Hematological Cancers. Immunol. Rev. 263 (1), 6–21. doi:10.1111/imr.12239
Kolenda, T., Guglas, K., Teresiak, A., Bliźniak, R., and Lamperska, K. (2019). Low Let-7d and High miR-205 Expression Levels Positively Influence HNSCC Patient Outcome. J. Biomed. Sci. 26 (1), 17. doi:10.1186/s12929-019-0511-3
Koo, K., Mouradov, D., Angel, C. M., Iseli, T. A., Wiesenfeld, D., McCullough, M. J., et al. (2021). Genomic Signature of Oral Squamous Cell Carcinomas from Non-smoking Non-drinking Patients. Cancers (Basel). 13 (5), 1029. doi:10.3390/cancers13051029
Kreimer, A. R., Chaturvedi, A. K., Alemany, L., Anantharaman, D., Bray, F., Carrington, M., et al. (2020). Summary from an International Cancer Seminar Focused on Human Papillomavirus (HPV)-positive Oropharynx Cancer, Convened by Scientists at IARC and NCI. Oral Oncol. 108, 104736. doi:10.1016/j.oraloncology.2020.104736
Kumar, R., Rai, A. K., Das, D., Das, R., Kumar, R. S., Sarma, A., et al. (2015). Alcohol and Tobacco Increases Risk of High Risk HPV Infection in Head and Neck Cancer Patients: Study from North-East Region of India. PLoS One 10 (10), e0140700. doi:10.1371/journal.pone.0140700
Kumari, K., Das, B., Adhya, A. K., Rath, A. K., and Mishra, S. K. (2019). Genome-wide Expression Analysis Reveals Six Contravened Targets of EZH2 Associated with Breast Cancer Patient Survival. Scientific Rep. 9, 1974. doi:10.1038/s41598-019-39122-4
Kurtz, K. A., Hoffman, H. T., Zimmerman, M. B., and Robinson, R. A. (2005). Perineural and Vascular Invasion in Oral Cavity Squamous Carcinoma: Increased Incidence on Re-review of Slides and by Using Immunohistochemical Enhancement. Arch. Pathol. Lab. Med. 129 (3), 354–9. doi:10.5858/2005-129-354-paviio
La Rosa, G. R. M., Gattuso, G., Pedullà, E., Rapisarda, E., Nicolosi, D., and Salmeri, M. (2020). Association of Oral Dysbiosis with Oral Cancer Development. Oncol. Lett. 19 (4), 3045–3058. doi:10.3892/ol.2020.11441
Larsen, S. R., Johansen, J., Sørensen, J. A., and Krogdahl, A. (2009). The Prognostic Significance of Histological Features in Oral Squamous Cell Carcinoma. J. Oral Pathol. Med. 38 (8), 657–62. doi:10.1111/j.1600-0714.2009.00797.x
Lee, K. W., and Pausova, Z. (2013). Cigarette Smoking and DNA Methylation. Front. Genet. 4, 132. doi:10.3389/fgene.2013.00132
Lee, L. Y., De Paz, D., Lin, C. Y., Fan, K. H., Wang, H. M., Hsieh, C. H., et al. (2019). Prognostic Impact of Extratumoral Perineural Invasion in Patients with Oral Cavity Squamous Cell Carcinoma. Cancer Med. 8 (14), 6185–6194. doi:10.1002/cam4.2392
Lenz, B., Klafki, H. W., Hillemacher, T., Killisch, N., Schaller, G., Frieling, H., et al. (2010). Smoking Behaviour Is Associated with Expression and Phosphorylation of CREB in Human Buffy Coat. Int. J. Neuropsychopharmacol. 13 (2), 207–15. doi:10.1017/S1461145709991052
Li, D., Bai, Y., Feng, Z., Li, W., Yang, C., Guo, Y., et al. (2019). Study of Promoter Methylation Patterns of HOXA2, HOXA5, and HOXA6 and its Clinicopathological Characteristics in Colorectal Cancer. Front. Oncol. 9, 394. doi:10.3389/fonc.2019.00394
Li, E., and Zhang, Y. (2014). DNA Methylation in Mammals. Cold Spring Harb Perspect. Biol. 6 (5), a019133. doi:10.1101/cshperspect.a019133
Li, G., Li, X., Yang, M., Xu, L., Deng, S., and Ran, L. (2017). Prediction of Biomarkers of Oral Squamous Cell Carcinoma Using Microarray Technology. Sci. Rep. 7, 42105. doi:10.1038/srep42105
Li, H., Wang, X., Zhang, M., Wang, M., Zhang, J., and Ma, S. (2021). Identification of HOXA1 as a Novel Biomarker in Prognosis of Head and Neck Squamous Cell Carcinoma. Front. Mol. Biosci. 7, 602068. doi:10.3389/fmolb.2020.602068
Li, Y., Mao, Y., Zhang, Y., Cai, S., Chen, G., Ding, Y., et al. (2014). Alcohol Drinking and Upper Aerodigestive Tract Cancer Mortality: A Systematic Review and Meta-Analysis. Oral Oncol. doi:10.1016/j.oraloncology.2013.12.015
Liebig, C., Ayala, G., Wilks, J., Verstovsek, G., Liu, H., Agarwal, N., et al. (2009a). Perineural Invasion Is an Independent Predictor of Outcome in Colorectal Cancer. J. Clin. Oncol. 27 (31), 5131–7. doi:10.1200/jco.2009.22.4949
Liebig, C., Ayala, G., Wilks, J. A., Berger, D. H., and Albo, D. (2009b). Perineural Invasion in Cancer: a Review of the Literature. Cancer 115 (15), 3379–91. doi:10.1002/cncr.24396
Lin, L. H., Lin, J. S., Yang, C. C., Cheng, H. W., Chang, K. W., and Liu, C. J. (2020a). Overexpression of Platelet-Derived Growth Factor and its Receptor Are Correlated with Oral Tumorigenesis and Poor Prognosis in Oral Squamous Cell Carcinoma. Int. J. Mol. Sci. 21 (7), 2360. doi:10.3390/ijms21072360
Lin, .P, Tian, P., Pang, J., Lai, L., He, G., Song, Y., et al. (2020b). Clinical Significance of COL1A1 and COL1A2 Expression Levels in Hypopharyngeal Squamous Cell Carcinoma. Oncol Lett 20 (1), 803–809. doi:10.3892/ol.2020.11594
Liu, D., Song, L., Dai, Z., Guan, H., Kang, H., Zhang, Y., et al. (2018). MiR-429 Suppresses Neurotrophin-3 to Alleviate Perineural Invasion of Pancreatic Cancer. Biochem. Biophys. Res. Commun. 505 (4), 1077–1083. doi:10.1016/j.bbrc.2018.09.147
Liu, S., de Medeiros, M. C., Fernandez, E. M., Zarins, K. R., Cavalcante, R. G., Qin, T., et al. (2020). 5-Hydroxymethylation Highlights the Heterogeneity in Keratinization and Cell Junctions in Head and Neck Cancers. Clin. Epigenetics 12 (1), 175. doi:10.1186/s13148-020-00965-8
Liu, Y., Chen, H., Sun, Z., and Chen, X. (2015). Molecular Mechanisms of Ethanol-Associated Oro-Esophageal Squamous Cell Carcinoma. Cancer Lett. 361 (2), 164–73. doi:10.1016/j.canlet.2015.03.006
Lleras, R. A., Smith, R. V., Adrien, L. R., Schlecht, N. F., Burk, R. D., Harris, T. M., et al. (2013). Unique DNA Methylation Loci Distinguish Anatomic Site and HPV Status in Head and Neck Squamous Cell Carcinoma. Clin. Cancer Res. 19 (19), 5444–55. doi:10.1158/1078-0432.ccr-12-3280
Llewelyn, J., and Smoking, Mitchell. R. (1994). Alcohol and Oral Cancer in South East Scotland: a 10-year Experience. Br. J. Oral Maxillofac. Surg. 32 (3), 146–152. doi:10.1016/0266-4356(94)90098-1
Ma, K. H., Hung, H. A., and Svaren, J. (2016). Epigenomic Regulation of Schwann Cell Reprogramming in Peripheral Nerve Injury. J. Neurosci. 36 (35), 9135–47. doi:10.1523/jneurosci.1370-16.2016
Mahmood, N., Hanif, M., Ahmed, A., Jamal, Q., Mushtaq, S., Khan, A., et al. (2019). Circulating miR-21 as a Prognostic and Predictive Biomarker in Oral Squamous Cell Carcinoma. Pak J. Med. Sci. 35 (5), 1408–1412. doi:10.12669/pjms.35.5.331
Marques, A. E. M., do Nascimento Filho, C. H. V., Marinho Bezerra, T. M., Guerra, E. N. S., Castilho, R. M., and Squarize, C. H. (2020). Entinostat Is a Novel Therapeutic Agent to Treat Oral Squamous Cell Carcinoma. J. Oral Pathol. Med. 49 (8), 771–779. doi:10.1111/jop.13039
Mascolo, M., Siano, M., Ilardi, G., Russo, D., Merolla, F., De Rosa, G., et al. (2012). Epigenetic Disregulation in Oral Cancer. Int. J. Mol. Sci. 13 (2), 2331–53. doi:10.3390/ijms13022331
Mello, F. W., Melo, G., Pasetto, J. J., Silva, C. A. B., Warnakulasuriya, S., and Rivero, E. R. C. (2019). The Synergistic Effect of Tobacco and Alcohol Consumption on Oral Squamous Cell Carcinoma: a Systematic Review and Meta-Analysis. Clin. Oral Investig. 23 (7), 2849–2859. doi:10.1007/s00784-019-02958-1
Menbari, M. N., Rahimi, K., Ahmadi, A., Mohammadi-Yegane, S., Elyasi, A., Darvishi, N., et al. (2020). Association of HDAC8 Expression with Pathological Findings in Triple Negative and Non-triple Negative Breast Cancer: Implications for Diagnosis. Iran Biomed. J. 24 (5), 288–94. doi:10.29252/ibj.24.5.283
Mendaza, S., Ulazia-Garmendia, A., Monreal-Santesteban, I., Córdoba, A., Azúa, Y. R., Aguiar, B., et al. (2020). ADAM12 Is A Potential Therapeutic Target Regulated by Hypomethylation in Triple-Negative Breast Cancer. Int. J. Mol. Sci. 21 (3), 903. doi:10.3390/ijms21030903
Miller, C. S., and Johnstone, B. M. (2001). Human Papillomavirus as a Risk Factor for Oral Squamous Cell Carcinoma: a Meta-Analysis, 1982-1997. Oral Surg. Oral Med. Oral Pathol. Oral Radiol. Endod. 91 (6), 622–35. doi:10.1067/moe.2001.115392
Misawa, K., Imai, A., Mochizuki, D., Mima, M., Endo, S., Misawa, Y., et al. (2018). Association of TET3 Epigenetic Inactivation with Head and Neck Cancer. Oncotarget 9 (36), 24480–24493. doi:10.18632/oncotarget.25333
Misawa, K., Kanazawa, T., Misawa, Y., Imai, A., Endo, S., Hakamada, K., et al. (2011-2012). Hypermethylation of Collagen α2 (I) Gene (COL1A2) Is an Independent Predictor of Survival in Head and Neck Cancer. Cancer Biomark 10 (3-4), 135–44. doi:10.3233/CBM-2012-0242
Misawa, K., Mochizuki, D., Imai, A., Endo, S., Mima, M., Misawa, Y., et al. (2016). Prognostic Value of Aberrant Promoter Hypermethylation of Tumor-Related Genes in Early-Stage Head and Neck Cancer. Oncotarget 7 (18), 26087–98. doi:10.18632/oncotarget.8317
Morse, D. E., Psoter, W. J., Cleveland, D., Cohen, D., Mohit-Tabatabai, M., Kosis, D. L., et al. (2007). Smoking and Drinking in Relation to Oral Cancer and Oral Epithelial Dysplasia. Cancer Causes Control 18, 919–929. doi:10.1007/s10552-007-9026-4
Narasimhan, N. S., and Narasimhan, N. M. (2018). The Emerging Role of MicroRNA21 in Oral Cancer. Biomed. Pharmacol. J. 11 (4). doi:10.13005/bpj/1569
O'Brien, C. J., Lahr, C. J., Soong, S. J., Gandour, M. J., Jones, J. M., Urist, M. M., et al. (1986). Surgical Treatment of Early-Stage Carcinoma of the Oral Tongue-Wwound Adjuvant Treatment Be Beneficial? Head Neck Surg. 8 (6), 401–8. doi:10.1002/hed.2890080603
Ondruschka, C., Buhtz, P., Motsch, C., Freigang, B., Schneider-Stock, R., Roessner, A., et al. (2002). Prognostic Value of MMP-2, -9 and TIMP-1,-2 Immunoreactive Protein at the Invasive Front in Advanced Head and Neck Squamous Cell Carcinomas. Pathol. Res. Pract. 198 (8), 509–15. doi:10.1078/s0344-0338(04)70292-7
Otálora-Otálora, B. A., Henríquez, B., López-Kleine, L., and Rojas, A. (2019). RUNX Family: Oncogenes or Tumor Suppressors (Review). Oncol. Rep. 42 (1), 3–19. doi:10.3892/or.2019.7149
Park, J. S., Park, Y. N., Lee, K. Y., Kim, J. K., and Yoon, D. S. (2013). P16 Hypermethylation Predicts Surgical Outcome Following Curative Resection of Mid/distal Bile Duct Cancer. Ann. Surg. Oncol. 20 (8), 2511–7. doi:10.1245/s10434-013-2908-7
Peng, S. Y., Tu, H. F., Yang, C. C., Wu, C. H., Liu, C. J., Chang, K. W., et al. (2018). miR-134 Targets PDCD7 to Reduce E-Cadherin Expression and Enhance Oral Cancer Progression. Int. J. Cancer 143 (11), 2892–2904. doi:10.1002/ijc.31638
Piqué, L., Martinez de Paz, A., Piñeyro, D., Martínez-Cardús, A., Castro de Moura, M., Llinàs-Arias, P., et al. (2019). Epigenetic Inactivation of the Splicing RNA-Binding Protein CELF2 in Human Breast Cancer. Oncogene 38 (45), 7106–7112. doi:10.1038/s41388-019-0936-x
Prueitt, R. L., Yi, M., Hudson, R. S., Wallace, T. A., Howe, T. M., Yfantis, H. G., et al. (2008). Expression of microRNAs and Protein-Coding Genes Associated with Perineural Invasion in Prostate Cancer. Prostate 68 (11), 1152–64. doi:10.1002/pros.20786
Pulukuri, S. M., Patibandla, S., Patel, J., Estes, N., and Rao, J. S. (2007). Epigenetic Inactivation of the Tissue Inhibitor of Metalloproteinase-2 (TIMP-2) Gene in Human Prostate Tumors. Oncogene 26 (36), 5229–37. doi:10.1038/sj.onc.1210329
Puram, S. V., Tirosh, I., Parikh, A. S., Patel, A. P., Yizhak, K., Gillespie, S., et al. (2017). Single-Cell Transcriptomic Analysis of Primary and Metastatic Tumor Ecosystems in Head and Neck Cancer. Cell 171 (7), 1611–1624.e24. doi:10.1016/j.cell.2017.10.044
Rahima, B., Shingaki, S., Nagata, M., and Saito, C. (2004). Prognostic Significance of Perineural Invasion in Oral and Oropharyngeal Carcinoma. Oral Surg. Oral Med. Oral Pathol. Oral Radiol. Endod. 97 (4), 423–31. doi:10.1016/j.tripleo.2003.10.014
Rajan, C., Roshan, V. G. D., Khan, I., Manasa, V. G., Himal, I., Kattoor, J., et al. (2021). MiRNA Expression Profiling and Emergence of New Prognostic Signature for Oral Squamous Cell Carcinoma. Sci. Rep. 11 (1), 7298. doi:10.1038/s41598-021-86316-w
Ramzan, F., Vickers, M. H., and Mithen, R. F. (2021). Epigenetics, microRNA and Metabolic Syndrome: A Comprehensive Review. Int. J. Mol. Sci. 22 (9), 5047. doi:10.3390/ijms22095047
Rasmussen, K. D., and Helin, K. (2016). Role of TET Enzymes in DNA Methylation, Development, and Cancer. Genes Dev. 30 (7), 733–750. doi:10.1101/gad.276568.115
Rastogi, B., Raut, S. K., Panda, N. K., Rattan, V., Radotra, B. D., and Khullar, M. (2016). Overexpression of HDAC9 Promotes Oral Squamous Cell Carcinoma Growth, Regulates Cell Cycle Progression, and Inhibits Apoptosis. Mol. Cell Biochem 415 (1-2), 183–96. doi:10.1007/s11010-016-2690-5
Rather, M. I., Nagashri, M. N., Swamy, S. S., Gopinath, K. S., and Kumar, A. (2013). Oncogenic microRNA-155 Down-Regulates Tumor Suppressor CDC73 and Promotes Oral Squamous Cell Carcinoma Cell Proliferation: Implications for Cancer Therapeutics. J. Biol. Chem. 288 (1), 608–18. doi:10.1074/jbc.m112.425736
Reis, P. P., Tomenson, M., Cervigne, N. K., Machado, J., Jurisica, I., Pintilie, M., et al. (2010). Programmed Cell Death 4 Loss Increases Tumor Cell Invasion and Is Regulated by miR-21 in Oral Squamous Cell Carcinoma. Mol. Cancer 9, 238. doi:10.1186/1476-4598-9-238
Ren, S., Gaykalova, D., Wang, J., Guo, T., Danilova, L., Favorov, A., et al. (2018). Discovery and Development of Differentially Methylated Regions in Human Papillomavirus-Related Oropharyngeal Squamous Cell Carcinoma. Int. J. Cancer 143 (10), 2425–2436. doi:10.1002/ijc.31778
Renjie, W., and Haiqian, L. (2015). MiR-132, miR-15a and miR-16 Synergistically Inhibit Pituitary Tumor Cell Proliferation, Invasion and Migration by Targeting Sox5. Cancer Lett. 356, 568–78. doi:10.1016/j.canlet.2014.10.003
Roh, J., Muelleman, T., Tawfik, O., and Thomas, S. M. (2015). Perineural Growth in Head and Neck Squamous Cell Carcinoma: a Review. Oral Oncol. 51 (1), 16–23. doi:10.1016/j.oraloncology.2014.10.004
Ross, G. L., Soutar, D. S., MacDonald, D. G., Shoaib, T., Camilleri, I. G., and Robertson, A. G. (2004). Improved Staging of Cervical Metastases in Clinically Node-Negative Patients with Head and Neck Squamous Cell Carcinoma. Ann. Surg. Oncol. 11 (2), 213–8. doi:10.1245/aso.2004.03.057
Ruokolainen, H., Pääkkö, P., and Turpeenniemi-Hujanen, T. (2006). Tissue and Circulating Immunoreactive Protein for MMP-2 and TIMP-2 in Head and Neck Squamous Cell Carcinoma-Ttissue Immunoreactivity Predicts Aggressive Clinical Course. Mod. Pathol. 19 (2), 208–17. doi:10.1038/modpathol.3800506
Russo, D., Merolla, F., Varricchio, S., Salzano, G., Zarrilli, G., Mascolo, M., et al. (2020). Erratum: Epigenetics of Oral and Oropharyngeal Cancers (Review). Biomed. Rep. 12 (5), 290. doi:10.3892/br.2020.1290
Saad, M. A., Kuo, S. Z., Rahimy, E., Zou, A. E., Korrapati, A., Rahimy, M., et al. (2015). Alcohol-dysregulated miR-30a and miR-934 in Head and Neck Squamous Cell Carcinoma. Mol. Cancer 14, 181. doi:10.1186/s12943-015-0452-8
Saito, Y., Liang, G., Egger, G., Friedman, J. M., Chuang, J. C., Coetzee, G. A., et al. (2006). Specific Activation of Microrna-127 with Downregulation of the Proto-Oncogene Bcl6 by Chromatin-Modifying Drugs in Human Cancer Cells. Cancer Cell 9, 435–443. doi:10.1016/j.ccr.2006.04.020
Sakuma, T., Uzawa, K., Onda, T., Shiiba, M., Yokoe, H., Shibahara, T., et al. (2006). Aberrant Expression of Histone Deacetylase 6 in Oral Squamous Cell Carcinoma. Int. J. Oncol. 29 (1), 117–24. doi:10.3892/ijo.29.1.117
Salaspuro, M. P. (2003). Acetaldehyde, Microbes, and Cancer of the Digestive Tract. Crit. Rev. Clin. Lab. Sci. 40, 183–208. doi:10.1080/713609333
Sartor, M. A., Dolinoy, D. C., Jones, T. R., Colacino, J. A., Prince, M. E., Carey, T. E., et al. (2011). Genome-wide Methylation and Expression Differences in HPV(+) and HPV(-) Squamous Cell Carcinoma Cell Lines Are Consistent with Divergent Mechanisms of Carcinogenesis. Epigenetics 6 (6), 777–87. doi:10.4161/epi.6.6.16216
Sato, T., Suzuki, M., Sato, Y., Echigo, S., and Rikiishi, H. (2006). Sequence-dependent Interaction between Cisplatin and Histone Deacetylase Inhibitors in Human Oral Squamous Cell Carcinoma Cells. Int. J. Oncol. 28, 1233–1241. doi:10.3892/ijo.28.5.1233
Scanlon, C. S., Banerjee, R., Inglehart, R. C., Liu, M., Russo, N., Hariharan, A., et al. (2015). Galanin Modulates the Neural Niche to Favour Perineural Invasion in Head and Neck Cancer. Nat. Commun. 6, 6885. doi:10.1038/ncomms7885
Scott, A. J., Alexander, J. L., Merrifield, C. A., Cunningham, D., Jobin, C., Brown, R., et al. (2019). International Cancer Microbiome Consortium Consensus Statement on the Role of the Human Microbiome in Carcinogenesis. Gut 68 (9), 1624–1632. doi:10.1136/gutjnl-2019-318556
Seitz, H. K., Stickel, F., and Homann, N. (2004). Pathogenetic Mechanisms of Upper Aerodigestive Tract Cancer in Alcoholics. Int. J. Cancer 108 (4), 483–7. doi:10.1002/ijc.11600
Seitz, H. K., and Stickel, F. (2007). Molecular Mechanisms of Alcohol-Mediated Carcinogenesis. Nat. Rev. Cancer 7, 599–612. doi:10.1038/nrc2191
Shrestha, B., Bajracharya, D., Byatnal, A. A., Kamath, A., and Radhakrishnan, R. (2017). May High MMP-2 and TIMP-2 Expressions Increase or Decrease the Aggressivity of Oral Cancer? Pathol. Oncol. Res. 23 (1), 197–206. doi:10.1007/s12253-016-0149-3
Shukla, S. D., Lee, Y. J., Park, P. H., and Aroor, A. R. (2007). Acetaldehyde Alters MAP Kinase Signalling and Epigenetic Histone Modifications in Hepatocytes. Novartis Found. Symp. 285, 217–24. doi:10.1002/9780470511848.ch16
Sierko, E., Wojtukiewicz, M. Z., and Kisiel, W. (2007). The Role of Tissue Factor Pathway Inhibitor-2 in Cancer Biology. Semin. Thromb. Hemost. 33 (7), 653–9. doi:10.1055/s-2007-991532
Silverman, B. R., and Shi, J. (2016). Alterations of Epigenetic Regulators in Pancreatic Cancer and Their Clinical Implications. Int. J. Mol. Sci. 17 (12), 2138. doi:10.3390/ijms17122138
Sim, J., Kim, Y., Kim, H., Bang, S., Jee, S., Park, S., et al. (2020). Loss of MTUS1 Expression Is Associated with Poor Prognosis in Patients with Gallbladder Carcinoma. Vivo 34 (1), 125–132. doi:10.21873/invivo.11753
Soo, K. C., Carter, R. L., O'Brien, C. J., Barr, L., Bliss, J. M., and Shaw, H. J. (1986). Prognostic Implications of Perineural Spread in Squamous Carcinomas of the Head and Neck. Laryngoscope 96 (10), 1145–8. doi:10.1288/00005537-198610000-00015
Sousa, L. O., Sobral, L. M., Matsumoto, C. S., Saggioro, F. P., López, R. V., Panepucci, R. A., et al. (2016). Lymph Node or Perineural Invasion Is Associated with Low miR-15a, miR-34c and miR-199b Levels in Head and Neck Squamous Cell Carcinoma. BBA Clin. 6, 159–164. doi:10.1016/j.bbacli.2016.11.001
Speight, P. M., and Barrett, A. W. (2009). Prognostic Factors in Malignant Tumours of the Salivary Glands. Br. J. Oral Maxillofac. Surg. 47 (8), 587–93. doi:10.1016/j.bjoms.2009.03.017
Staibano, S., Mignogna, C., Lo Muzio, L., Mascolo, M., Salvatore, G., Di Benedetto, M., et al. (2007). Chromatin Assembly Factor-1 (CAF-1)-Mediated Regulation of Cell Proliferation and DNA Repair: a Link with the Biological Behaviour of Squamous Cell Carcinoma of the Tongue? Histopathology 50 (7), 911–9. doi:10.1111/j.1365-2559.2007.02698.x
Stornetta, A., Guidolin, V., and Balbo, S. (2018). Alcohol-Derived Acetaldehyde Exposure in the Oral Cavity. Cancers (Basel) 10 (1), 20. doi:10.3390/cancers10010020
Stott, F. J., Bates, S., James, M. C., McConnell, B. B., Starborg, M., Brookes, S., et al. (1998). The Alternative Product from the Human CDKN2A Locus, p14(ARF), Participates in a Regulatory Feedback Loop with P53 and MDM2. EMBO J. 17 (17), 5001–14. doi:10.1093/emboj/17.17.5001
Su Mun, L., Wye Lum, S., Kong Yuiin Sze, G., Hock Yoong, C., Ching Yung, K., Kah Lok, L., et al. (2021). Association of Microbiome with Oral Squamous Cell Carcinoma: A Systematic Review of the Metagenomic Studies. Int. J. Environ. Res. Public Health 18 (14), 7224. doi:10.3390/ijerph18147224
Sullivan, L. M., and Smee, R. (2006). Leptomeningeal Carcinomatosis from Perineural Invasion of a Lip Squamous Cell Carcinoma. Australas. Radiol. 50 (3), 262–6. doi:10.1111/j.1440-1673.2006.01577.x
Supic, G., Kozomara, R., Brankovic-Magic, M., Jovic, N., and Magic, Z. (2009). Gene Hypermethylation in Tumor Tissue of Advanced Oral Squamous Cell Carcinoma Patients. Oral Oncol. 45 (12), 1051–7.
Supic, G., Kozomara, R., Jovic, N., Zeljic, K., and Magic, Z. (2011). Hypermethylation of RUNX3 but Not WIF1 Gene and its Association with Stage and Nodal Status of Tongue Cancers. Oral Dis. 17 (8), 794–800. doi:10.1111/j.1601-0825.2011.01838.x
Supic, G., Kozomara, R., Zeljic, K., Jovic, N., and Magic, Z. (2017). Prognostic Value of the DNMTs mRNA Expression and Genetic Polymorphisms on the Clinical Outcome in Oral Cancer Patients. Clin. Oral Investig. 21 (1), 173–182. doi:10.1007/s00784-016-1772-9
Sutton, D. N., Brown, J. S., Rogers, S. N., Vaughan, E. D., and Woolgar, J. A. (2003). The Prognostic Implications of the Surgical Margin in Oral Squamous Cell Carcinoma. Int. J. Oral Maxillofac. Surg. 32 (1), 30–4. doi:10.1054/ijom.2002.0313
Suzuki, M., Shinohara, F., Endo, M., Sugazaki, M., Echigo, S., and Rikiishi, H. (2009). Zebularine Suppresses the Apoptotic Potential of 5-fluorouracil via cAMP/PKA/CREB Pathway against Human Oral Squamous Cell Carcinoma Cells. Cancer Chemother. Pharmacol. 64 (2), 223–32. doi:10.1007/s00280-008-0833-4
Szabo, A., Gurlich, R., Liberko, M., Soumarova, R., Vernerova, Z., Mandys, V., et al. (2020). Expression of Selected microRNAs in Pancreatic Ductal Adenocarcinoma: Is There a Relation to Tumor Morphology, Progression and Patient's Outcome? Neoplasma 67 (5), 1170–1181. doi:10.4149/neo_2020_200123N87
Tan, L., and Shi, Y. G. (2012). Tet Family Proteins and 5-hydroxymethylcytosine in Development and Disease. Development 139 (11), 1895–902. doi:10.1242/dev.070771
Tang, K. L., Tang, H. Y., Du, Y., Tian, T., and Xiong, S. J. (2019). MiR-638 Suppresses the Progression of Oral Squamous Cell Carcinoma through Wnt/β-Catenin Pathway by Targeting Phospholipase D1. Artif. Cell Nanomed Biotechnol 47 (1), 3278–3285. doi:10.1080/21691401.2019.1647222
Tasoulas, J., Giaginis, C., Patsouris, E., Manolis, E., and Theocharis, S. (2015). Histone Deacetylase Inhibitors in Oral Squamous Cell Carcinoma Treatment. Expert Opin. Investig. Drugs 24 (1), 69–78. doi:10.1517/13543784.2014.952368
Thienpont, B., Steinbacher, J., Zhao, H., D'Anna, F., Kuchnio, A., Ploumakis, A., et al. (2016). Tumour Hypoxia Causes DNA Hypermethylation by Reducing TET Activity. Nature 537 (7618), 63–68. doi:10.1038/nature19081
Torre, L. A., Bray, F., Siegel, R. L., Ferlay, J., Lortet-Tieulent, J., and Jemal, A. (2015). Global Cancer Statistics. CA Cancer J. Clin. 65 (2), 87–108. doi:10.3322/caac.21262
Uehara, E., Shiiba, M., Shinozuka, K., Saito, K., Kouzu, Y., Koike, H., et al. (2012). Upregulated Expression of ADAM12 Is Associated with Progression of Oral Squamous Cell Carcinoma. Int. J. Oncol. 40 (5), 1414–22. doi:10.3892/ijo.2012.1339
Urvalek, A. M., Osei-Sarfo, K., Tang, X. H., Zhang, T., Scognamiglio, T., and Gudas, L. J. (2015). Identification of Ethanol and 4-Nitroquinoline-1-Oxide Induced Epigenetic and Oxidative Stress Markers during Oral Cavity Carcinogenesis. Alcohol. Clin. Exp. Res. 39 (8), 1360–72. doi:10.1111/acer.12772
Verdoodt, B., Sommerer, F., Palisaar, R. J., Noldus, J., Vogt, M., Nambiar, S., et al. (2011). Inverse Association of P16 INK4a and P14 ARF Methylation of the CDKN2a Locus in Different Gleason Scores of Prostate Cancer. Prostate Cancer Prostatic Dis. 14 (4), 295–301. doi:10.1038/pcan.2011.45
Viet, C. T., and Schmidt, B. L. (2008). Methylation Array Analysis of Preoperative and Postoperative Saliva DNA in Oral Cancer Patients. Cancer Epidemiol. Biomarkers Prev. 17 (12), 3603–11. doi:10.1158/1055-9965.epi-08-0507
Virani, S., Bellile, E., Bradford, C. R., Carey, T. E., Chepeha, D. B., Colacino, J. A., et al. (2015). NDN and CD1A Are Novel Prognostic Methylation Markers in Patients with Head and Neck Squamous Carcinomas. BMC Cancer 15, 825. doi:10.1186/s12885-015-1806-8
Wallwork, B. D., Anderson, S. R., and Coman, W. B. (2007). Squamous Cell Carcinoma of the Floor of the Mouth: Tumour Thickness and the Rate of Cervical Metastasis. ANZ J. Surg. 77 (9), 761–4. doi:10.1111/j.1445-2197.2007.04219.x
Wang, V., and Wu, W. (2009). MicroRNA-based Therapeutics for Cancer. BioDrugs 23 (1), 15–23. doi:10.2165/00063030-200923010-00002
Wang, W., Gao, J., Man, X. H., Li, Z. S., and Gong, Y. F. (2009). Significance of DNA Methyltransferase-1 and Histone Deacetylase-1 in Pancreatic Cancer. Oncol. Rep. 21 (6), 1439–47. doi:10.3892/or_00000372
Wang, X., and Li, G. H. (2018). MicroRNA-16 Functions as a Tumor-Suppressor Gene in Oral Squamous Cell Carcinoma by Targeting AKT3 and BCL2L2. J. Cell Physiol 233 (12), 9447–9457. doi:10.1002/jcp.26833
Wang, X., Li, H., and Shi, J. (2019). LncRNA HOXA11-AS Promotes Proliferation and Cisplatin Resistance of Oral Squamous Cell Carcinoma by Suppression of miR-214-3p Expression. Biomed. Res. Int. 2019, 8645153. doi:10.1155/2019/8645153
Wang, Y., Hu, H., Wang, Q., Li, Z., Zhu, Y., Zhang, W., et al. (2017). The Level and Clinical Significance of 5-hydroxymethylcytosine in Oral Squamous Cell Carcinoma: An Immunohistochemical Study in 95 Patients. Pathol. Res. Pract. 213 (8), 969–974. doi:10.1016/j.prp.2017.04.016
Watanabe, T., Morinaga, S., Akaike, M., Numata, M., Tamagawa, H., Yamamoto, N., et al. (2012). The Cellular Level of Histone H3 Lysine 4 Dimethylation Correlates with Response to Adjuvant Gemcitabine in Japanese Pancreatic Cancer Patients Treated with Surgery. Eur. J. Surg. Oncol. 38 (11), 1051–7. doi:10.1016/j.ejso.2012.08.008
Woolgar, J. A. (2006). Histopathological Prognosticators in Oral and Oropharyngeal Squamous Cell Carcinoma. Oral Oncol. 42 (3), 229–39. doi:10.1016/j.oraloncology.2005.05.008
Woolgar, J. A., and Scott, J. (1995). Prediction of Cervical Lymph Node Metastasis in Squamous Cell Carcinoma of the Tongue/floor of Mouth. Head Neck 17 (6), 463–72. doi:10.1002/hed.2880170603
Wu, J., Liu, J., Wei, X., Yu, Q., Niu, X., Tang, S., et al. (2019). A Feature-Based Analysis Identifies COL1A2 as a Regulator in Pancreatic Cancer. J. Enzyme Inhib. Med. Chem. 34 (1), 420–428. doi:10.1080/14756366.2018.1484734
Yamamoto, T., Hirosue, A., Nakamoto, M., Yoshida, R., Sakata, J., Matsuoka, Y., et al. (2020). BRD4 Promotes Metastatic Potential in Oral Squamous Cell Carcinoma through the Epigenetic Regulation of the MMP2 Gene. Br. J. Cancer 123 (4), 580–590. doi:10.1038/s41416-020-0907-6
Yang, X., Han, B., Zhang, R., Su, Y., Hosseini, D. K., Wu, H., et al. (2021). Development and Validation of a RNA Binding Protein-Associated Prognostic Model for Head and Neck Squamous Cell Carcinoma. Aging (Albany NY) 13 (6), 7975–7997. doi:10.18632/aging.202848
Yang, Y., Deng, X., Chen, X., Chen, S., Song, L., Meng, M., et al. (2020). Landscape of Active Enhancers Developed De Novo in Cirrhosis and Conserved in Hepatocellular Carcinoma. Am. J. Cancer Res. 10 (10), 3157–3178.
Yao, Q., Chen, Y., and Zhou, X. (2019). The Roles of microRNAs in Epigenetic Regulation. Curr. Opin. Chem. Biol. 51, 11–17. doi:10.1016/j.cbpa.2019.01.024
Yi, X., Guo, J., Guo, J., Sun, S., Yang, P., Wang, J., et al. (2017). EZH2-mediated Epigenetic Silencing of TIMP2 Promotes Ovarian Cancer Migration and Invasion. Scientific Rep. 7, 3568. doi:10.1038/s41598-017-03362-z
Yorioka, C. W., Coletta, R. D., Alves, F., Nishimoto, I. N., Kowalski, L. P., and Graner, E. (2002). Matrix Metalloproteinase-2 and -9 Activities Correlate with the Disease-free Survival of Oral Squamous Cell Carcinoma Patients. Int. J. Oncol. 20 (1), 189–94. doi:10.3892/ijo.20.1.189
Yoshizaki, T., Maruyama, Y., Sato, H., and Furukawa, M. (2001). Expression of Tissue Inhibitor of Matrix Metalloproteinase-2 Correlates with Activation of Matrix Metalloproteinase-2 and Predicts Poor Prognosis in Tongue Squamous Cell Carcinoma. Int. J. Cancer 95 (1), 44–50. doi:10.1002/1097-0215(20010120)95:1<44::aid-ijc1008>3.0.co;2-m
Yu, E. H., Tu, H. F., Wu, C. H., Yang, C. C., and Chang, K. W. (2017). MicroRNA-21 Promotes Perineural Invasion and Impacts Survival in Patients with Oral Carcinoma. J. Chin. Med. Assoc. 80 (6), 383–388. doi:10.1016/j.jcma.2017.01.003
Yu, S. Y., Wang, Y. P., Chang, J. Y., Shen, W. R., Chen, H. M., and Chiang, C. P. (2014). Increased Expression of MCM5 Is Significantly Associated with Aggressive Progression and Poor Prognosis of Oral Squamous Cell Carcinoma. J. Oral Pathol. Med. 43 (5), 344–9. doi:10.1111/jop.12134
Yu, V., Singh, P., Rahimy, E., Zheng, H., Kuo, S. Z., Kim, E., et al. (2016). RNA-seq Analysis Identifies Key Long Non-coding RNAs Connected to the Pathogenesis of Alcohol-Associated Head and Neck Squamous Cell Carcinoma. Oncol. Lett. 12 (4), 2846–2853. doi:10.3892/ol.2016.4972
Zebolsky, A. L., George, E., Gulati, A., Wai, K. C., Carpenter, P., Van Zante, A., et al. (2021). Risk of Pathologic Extranodal Extension and Other Adverse Features after Transoral Robotic Surgery in Patients with HPV-Positive Oropharynx Cancer. JAMA Otolaryngol. Head Neck Surg., e212777. doi:10.1001/jamaoto.2021.2777
Zhai, L. L., Wu, Y., Cai, C. Y., and Tang, Z. G. (2015). Upregulated Matrix Metalloproteinase-2 and Downregulated Tissue Factor Pathway Inhibitor-2 Are Risk Factors for Lymph Node Metastasis and Perineural Invasion in Pancreatic Carcinoma. Onco Targets Ther. 8, 2827–34. doi:10.2147/ott.s90599
Zhang, C. Y., Mao, L., Li, L., Tian, Z., Zhou, X. J., Zhang, Z. Y., et al. (2007). Promoter Methylation as a Common Mechanism for Inactivating E-Cadherin in Human Salivary Gland Adenoid Cystic Carcinoma. Cancer 110 (1), 87–95. doi:10.1002/cncr.22758
Zhang, J., Fu, X., Liu, D., Yang, M., Yang, J., Huo, Y., et al. (2020). Molecular Markers Associated with Perineural Invasion in Pancreatic Ductal Adenocarcinoma. Oncol. Lett. 20 (4), 5. doi:10.3892/ol.2020.11866
Zhang, M., Xian, H. C., Dai, L., Tang, Y. L., and Liang, X. H. (2021). MicroRNAs: Emerging Driver of Cancer Perineural Invasion. Cell Biosci 11 (1), 117. doi:10.1186/s13578-021-00630-4
Zhang, R., Qi, F., Zhao, F., Li, G., Shao, S., Zhang, X., et al. (2019). Cancer-associated Fibroblasts Enhance Tumor-Associated Macrophages Enrichment and Suppress NK Cells Function in Colorectal Cancer. Cell Death Dis 10 (4), 273. doi:10.1038/s41419-019-1435-2
Zhao, L., Yu, Y., Wu, J., Bai, J., Zhao, Y., Li, C., et al. (2014). Role of EZH2 in Oral Squamous Cell Carcinoma Carcinogenesis. Gene 537 (2), 197–202. doi:10.1016/j.gene.2014.01.006
Zheng, B., Qu, J., Ohuchida, K., Feng, H., Chong, S. J. F., Yan, Z., et al. (2020b). LAMA4 Upregulation Is Associated with High Liver Metastasis Potential and Poor Survival Outcome of Pancreatic Cancer. Theranostics 10, 10274–10289. doi:10.7150/thno.47001
Zheng, J., Wang, J., Jia, Y., Liu, T., Duan, Y., Liang, X., et al. (2019b). microRNA-211 Promotes Proliferation, Migration, and Invasion Ability of Oral Squamous Cell Carcinoma Cells via Targeting the Bridging Integrator 1 Protein. J. Cell Biochem 120 (3), 4644–4653. doi:10.1002/jcb.27753
Zheng, Q., Maksimovic, I., Upad, A., and David, Y. (2020a). Non-enzymatic Covalent Modifications: a New Link between Metabolism and Epigenetics. Protein Cell 11 (6), 401–416. doi:10.1007/s13238-020-00722-w
Zheng, Q., Prescott, N. A., Maksimovic, I., and David, Y. (2019a). (De) Toxifying the Epigenetic Code. Chem. Res. Toxicol. 32 (5), 796–807. doi:10.1021/acs.chemrestox.9b00013
Zhou, W. N., Du, Y. F., Bai, J., Song, X. M., Zheng, Y., Yuan, H., et al. (2017). RUNX3 Plays a Tumor Suppressor Role by Inhibiting Cell Migration, Invasion and Angiogenesis in Oral Squamous Cell Carcinoma. Oncol. Rep. 38 (4), 2378–2386. doi:10.3892/or.2017.5857
Keywords: HNSCC, perineural invasion, epigenetics, oral cancer, miRNAs
Citation: Hurník P, Chyra Z, Ševčíková T, Štembírek J, Trtková KS, Gaykalova DA, Buchtová M and Hrubá E (2022) Epigenetic Regulations of Perineural Invasion in Head and Neck Squamous Cell Carcinoma. Front. Genet. 13:848557. doi: 10.3389/fgene.2022.848557
Received: 04 January 2022; Accepted: 09 March 2022;
Published: 27 April 2022.
Edited by:
Ángeles Carlos Reyes, Instituto Nacional de Enfermedades Respiratorias-México (INER), MexicoReviewed by:
Jozsef Dudas, Innsbruck Medical University, AustriaYarely M. Salinas-Vera, Universidad Autónoma de la Ciudad de México, Mexico
Copyright © 2022 Hurník, Chyra, Ševčíková, Štembírek, Trtková, Gaykalova, Buchtová and Hrubá. This is an open-access article distributed under the terms of the Creative Commons Attribution License (CC BY). The use, distribution or reproduction in other forums is permitted, provided the original author(s) and the copyright owner(s) are credited and that the original publication in this journal is cited, in accordance with accepted academic practice. No use, distribution or reproduction is permitted which does not comply with these terms.
*Correspondence: Eva Hrubá, uzievama@gmail.com
†These authors have contributed equally to this work and share first authorship