- 1Guangxi Key Laboratory of Environmental Exposomics and Entire Lifecycle Health, Guangxi Health Commission Key Laboratory of Entire Lifecycle Health and Care, Guilin Medical University, Guilin, China
- 2Department of Obstetrics and Gynecology, The Second Affiliated Hospital of Guilin Medical University, Guilin, China
Background: The involvement of oxidative stress in the pathological process of hypertensive disorders of pregnancy (HDP) gives rise to the interest in exploring the association of genetic variations in antioxidant metallothionein (MT) genes with HDP susceptibility.
Methods: Seventeen single-nucleotide polymorphisms(SNPs) in MT genes were selected to conduct genotyping based on a case-control study consisting of 371 HDP cases (pregnancy with chronic hypertension (66), gestational hypertension (172), and preeclampsia or preeclampsia superimposed on chronic hypertension (133)) and 479 controls. The association between SNPs in MTs and the risk of HDP was estimated with unconditional logistic regression analysis and further tested with the false-positive report probability (FPRP) procedure. The joint effects of SNPs on the HDP risk were assessed by haplotype analysis.
Results: After the adjustment for age and pre-pregnancy body mass index (pre-BMI) in the logistic regress analysis and followed by the FPRP test, the genetic variation rs10636 (OR = 0.46, 95% CI: 0.30–0.71 for GG vs. CC, p = 0.000 and OR = 0.48, 95% CI: 0.32–0.73 for GG vs. CG/CC, p = 0.001) in MT2A was associated with gestational hypertension. Other four SNPs, that is, rs11076161 (OR = 1.89, 95% CI: 1.35–2.63 for GG vs. GA/AA, p = 0.000) in MT1A; rs7191779 (OR = 1.54, 95% CI: 1.11–2.13 for CC vs. CG/GG, p = 0.010) in MT1B; rs8044719 (OR = 0.57, 95% CI: 0.40–0.80 for GT vs. GG, p = 0.001) in MT1DP; and rs8052334 (OR = 1.52, 95% CI: 1.10–2.11 for TT vs. TC/CC, p = 0.012) in MT1B were significantly associated with the susceptibility of HDP. The haplotype analysis among 11, 10, 10, and seven SNPs in MT (MT1A, MT2A, MT1M, MT1B, and MT1DP) genes showed that eight (A-C-G-T-C-G-A-G-C-G-C, OR = 4.559; A-C-T-C-C-C-A-G-C-G-C, OR = 5.777; A-C-T-T-C-G-A-G-C-G-C, OR = 4.590; G-A-T-C-C-G-C-G-G-C-C, OR = 4.065; G-A-T-C-G-C-C-G-G-C-C, OR = 4.652; G-A-T-T-C-C-C-G-G-C-C, OR = 0.404; G-C-T-C-C-C-A-G-G-C-C, OR = 1.901; G-C-T-T-C-C-A-G-G-C-C, and OR = 3.810), five (C-G-A-T-C-A-C-C-G-G, OR = 2.032; C-G-A-T-C-G-C-C-G-G, OR = 2.077; G-A-C-T-C-A-C-C-T-G, OR = 0.564; G-G-A-G-C-A-C-C-G-G, OR = 5.466; G-G-A-T-T-A-G-C-G-G, and OR = 0.284), five (A-C-G-T-C-G-A-G-C-C, OR = 2.399; A-C-T-C-C-C-C-T-G-G, OR = 0.259; G-A-T-C-C-C-C-G-G-C, OR = 1.572; G-A-T-C-G-C-C-G-G-C, OR = 0.001; G-C-T-C-G-C-A-G-G-C, and OR = 2.512), and five (A-C-T-C-C-C-G, OR = 0.634; G-A-G-C-C-C-G, OR = 4.047; G-A-T-T-G-C-G, OR = 0.499; G-C-G-T-C-A-G, and OR = 7.299; G-C-T-C-C-A-G, OR = 1.434) haplotypes were significantly associated with pregnancy with chronic hypertension, gestational hypertension, preeclampsia, or preeclampsia superimposed on chronic hypertension and HDP.
Conclusion: These variant MT alleles and their combination patterns may be used as genetic markers for predicting HDP susceptibility.
Introduction
Hypertensive disorders of pregnancy (HDP) are unique diseases in obstetrics characterized by a systolic pressure equal to or above 140 mmHg and/or diastolic pressure equal to or above 90 mmHg (Wilkerson and Ogunbodede, 2019), which include pregnancy with chronic hypertension, gestational hypertension, preeclampsia (PE)/eclampsia, and chronic hypertension with superimposed PE/eclampsia (Khedagi and Bello, 2021). The average estimated worldwide prevalence of HDP are 5.2–8.2%, leading to 10–15% of maternal deaths, particularly in developing countries (Cao et al., 2020; Khan et al., 2006; Shah and Gupta, 2019). In China, the morbidity and mortality rates of HDP are up to 9.4% and ultimately 10.77–13.83% of maternal deaths, respectively (Cao et al., 2020; Lu et al., 2018). Although a variety of susceptibility factors of HDP have been identified, such as advanced maternal age, nulliparity, obesity, multiple gestations, and diabetes mellitus (Cao et al., 2020; Shah and Gupta, 2019; Su et al., 2020), the underlying mechanism of HDP remains unknown. However, oxidative stress has been proposed to be one of the leading pathophysiological processes of HDP (Phoswa and Khaliq, 2021). This occurs when the production of reactive oxygen species (ROS) and/or reactive nitrogen species (RNS) overwhelms the antioxidant capacity in the vascular walls, which causes constriction of blood vessels, thus resulting in the development of hypertension (Phoswa and Khaliq, 2021; Rozas-Villanueva et al., 2020; Sorriento et al., 2018).
Although exposures to environmental factors such as cadmium, noise, or traffic-related air pollution may increase the risk of HDP (Padula et al., 2021; Stanhope et al., 2021; Tanaka et al., 2019), HDP displays a genetic predisposition and some HDP-related susceptibility regions and genes have been identified (Umesawa and Kobashi, 2017). For instance, the genetic variations in endoplasmic reticulum aminopeptidase 1 and 2 (ERAP1, 2) genes and deletion of the pregnancy-specific glycoprotein 11 (PSG11) gene are significantly associated with PE development (Ferreira et al., 2021; Seamon et al., 2020; Thakoordeen et al., 2018). A region in chromosome 2 predisposes Finland, Icelandic, Australian, and New Zealand populations to PE and, therefore, has been proposed as “PREG11” (preeclampsia, eclampsia gene 1) locus (Moses et al., 2000; Thakoordeen et al., 2018). A recent meta-analysis showed that rs3918242 in matrix metalloproteinase 9 (MMP9) genes was associated with the susceptibility of HDP, especially preeclampsia and gestational hypertension. The variant genotype and allele of rs3918242 C/T increased the risk of preeclampsia (OR = 1.48, 1.32; 95% CI: 1.18–1.86, 1.08–1.62) and gestational hypertension (OR = 2.23, 1.88; 95% CI: 1.52–3.28, 1.33–2.65) in the fixed-effect models (Zhou et al., 2021).
Metallothioneins (MTs) are a family of low–molecular weight (∼7,000 Da), highly conserved, and cysteine-rich metal-binding proteins (Shabb et al., 2017), which are involved in various physiological processes such as antioxidative stress, apoptosis, proliferation, angiogenesis, and detoxification of heavy metals (Bizon et al., 2017; Dutsch-Wicherek et al., 2008; Jin et al., 2002; Xue et al., 2012). In humans, MTs have four main subfamilies (MT1, MT2, MT3, and MT4) that are encoded by genes located at chromosome 16q13. MT genes comprise 12 functional isoforms (MT1A, MT1B, MT1E, MT1F, MT1G, MT1H, MT1L, MT1M, MT1X, MT2A, MT3, and MT4) and 7 nonfunctional alleles (MT-1C, MT-1D, MT-1I, MT-1J, MT-1K, MT-1L, and MT-2B) (McNeill et al., 2019; Raudenska et al., 2014). MTs can be highly induced by many different stimuli such as metals, hormones, cytokines, growth factors, oxidants, and irradiation (Alvarez-Barrios et al., 2021; Si and Lang, 2018) and thus have been proposed to be biomarkers for stress-related diseases/disorders including hypertension, tumors, diabetes mellitus, and metal poisoning (Sekovanic et al., 2020; Wang et al., 2018; Zhang et al., 2021; Zhang et al., 2020). In addition, MTs also function as powerful electrophilic scavengers and cytoprotective agents against oxidative stress and inflammatory injury by capturing ROS (Draganovic et al., 2016), and they keep protecting biological structures and the function of biomacromolecules from oxidative injuries (Jarosz et al., 2017).
HDP is directly associated with oxidative stress based on the fact that unsaturated lipids and thiol proteins in the membrane of endothelial cells in the vessel system are susceptible to be attacked by free radicals (Bizerea et al., 2018), and oxidative stress induces excessive production of ROS and RNS (Wisdom et al., 1991). This prompts us to systematically study the linkage of MT variations with the susceptibility of HDP. We conducted a case-control study to explore the association between genetic variations of MT genes (MT1A, MT1B, MT1DP, MT1M, MT2A, and MT3) and the risks of HDP by using the unconditional logistic regression, false-positive report probability (FPRP), and haplotype analysis in the study.
Materials and Methods
Study Population
A total of 371 HDP cases, including 66 of pregnancy with chronic hypertension (PCH), 172 of gestational hypertension (GH), and 133 of preeclampsia or preeclampsia superimposed on chronic hypertension (PE/PESCH), and 479 controls were recruited in the Affiliated Hospital of Guilin Medical University from September 2014 to June 2016 in this study. HDP was diagnosed by medical professionals in the hospital according to the recommendations from the International Society for the Study of Hypertension in Pregnancy (ISSHP) (Brown et al., 2018). The controls were matched to the cases by normal singleton pregnant women of ±3 years in the same period. The subjects with other basic diseases related to the liver, kidney, endocrine, nervous and respiratory systems, etc., were excluded. Also, all participants were of Guangxi nationality who had lived in Guilin city for more than 10 years with no communication barriers. This study was approved by the Ethics Committee Review Boards of Guilin Medical University. Each participant signed the written informed consent form.
Questionnaire Survey
A uniform epidemiological questionnaire was used to collect the demographic characteristics of the study populations, including age, height, weight, systolic blood pressure (SBP), diastolic blood pressure (DBP), personal history, and family history of hypertension and other diseases. The body mass index before gestation (pre-pregnancy BMI) was calculated as body weight (kg) divided by the square of height (m2). The biochemical data including fasting blood glucose (FBG), triglyceride (TG), total cholesterol (TC), and high-density lipoprotein cholesterol (HDL-c), which were determined during first trimester, were collected from medical records in hospitals.
Blood Sample Collection and DNA Extraction
The peripheral blood sample (5 ml) was obtained from each participant, and genomic DNA was extracted by using a DNA extraction kit (Aidlab Biotechnologies Co., Ltd, China) and stored at −20°C for later analysis.
SNP Selection
Seventeen functional SNPs of MT genes were chosen to conduct genotyping in the study according to the NIEHS SNPinfo Web Server (http://snpinfo.niehs.nih.gov/), NCBI dbSNP database (http://www.ncbi.nlm.nih.gov/projects/SNP), HapMap database (https://www.genome.gov/10001688), 1,000 Genomes (https://www.ncbi.nlm.nih.gov/variation/tools/1000genomes/), and the literature with the following criteria: 1) the SNPs are located at the regulatory region of MT genes, that is, the 5′ near gene, 5′ untranslated regions (UTR), 3′UTR, 3′near gene, splicing site (ESE or ESS), or miRNA binding site; 2) the minor allele frequency (MAF) of SNPs in Han Chinese in Beijing (CHB) or Asian/East Asian reported in HapMap or 1,000 Genomes is greater than 0.05; 3) the SNPs might affect transcription factor binding site (TFBS) activity in the putative promoter region or the posttranscriptional splicing process; 4) the linkage disequilibrium (LD) coefficient r2 between candidate SNPs should be less than 0.8 (Rahimmadar et al., 2021; Xu et al., 2007) or one of them will be selected as tagSNP; and 5) the SNPs that have been identified to be related to MT functions by published studies even though they are located outside the functional regions will also be preferentially chosen.
A total of 191 SNPs were picked up in SNP Function Prediction by the gene name, in which 76 SNPs located out of functional regions and 70 SNPs with MAF<0.05 were excluded. The remaining 45 SNPs were maintained for LD analysis. Among them, six SNPs (rs11640851 (MT1A), rs8052394 (MT1A), rs8052334 (MT1B), rs1610216 (MT2A), rs28366003 (MT2A), and rs45570941 (MT3)) were included as tagSNPs (LD coefficient r2 < 0.8); nine SNPs (rs1599823 (MT1DP), rs8044719 (MT1DP), rs9936741 (MT1M), rs1827208 (MT1M), rs10636 (MT2A), rs11644094 (MT3), rs8049883 (MT1A), rs11076161 (MT1A), and rs7196890 (MT1A)) were selected (LD coefficient r2 > 0.8); and two SNPs (rs1580833 (MT2A) and rs7191779 (MT1B)) were taken from literatures (Supplementary Table S1).
Genotyping
SNP genotyping was performed by using the Agena MassARRAY genotyping system (Agena; San Diego, CA) according to the manufacturer’s recommendation. The sequence of the SNP was obtained from the NCBI website, and the primers were designed and synthesized by Bio Miao Biological (Beijing, China). The main steps included the following: 1) polymerase chain reaction (PCR): each PCR master mix (5 μl) comprised 1 μl DNA template (10 ng/μl), 1.850 μl water, 0.625 μl of 1.25×PCR buffer (with 15 mmol/L MgCl2), 0.325 μl of 25 mmol/L MgCl2, 0.1 μl of 25 mmol/L dNTP mix, 1 μl of 0.5 μmol/L primer mix, and 0.1 μl of 5 U/μl Hot Star Taq polymerase. PCR was carried out at 94°C for 5 min; followed by running through 45 cycles consisting of 94°C for 20 s, 56°C for 30 s, and 72°C for 1 min; and finally incubated at 72°C for 3 min; 2) shrimp alkali enzyme purification (SAP) reaction: the volume contained 0.17 μl of 10 × SAP buffer, 0.3 μl of 1 U/μl SAP enzyme, and 1.53 μl water; 3) single-base extension reaction included 0.2 μl of iPLEX Buffer Plus, 0.2 μl of iPLEX termination mix, 0.94 μl of primer mix, 0.041 μl of iPLEX enzyme, 0.619 μl of water, and 7 μl of SAP + PCR reaction; and 4) resin purification. The data of the genotyping were analyzed with MassARRAY Typer software version 4.0.
Statistical Analysis
The data were analyzed by using IBM SPSS Statistics 25. Student’s t-test was performed to analyze the quantitative data. The chi-square (χ2) test was applied to examine the accordance of genotypes of designated SNPs between HDP cases and controls. Hardy–Weinberg equilibrium (HWE) in the controls was tested by using a chi-square goodness-of-fit test. Unconditional logistic regression analysis was used to estimate the associations between the genotypes of SNPs and HDP susceptibility by computing the odds ratios (ORs) and their 95% confidence intervals (CIs). The statistical power of each SNP was estimated by using post hoc power analysis, in which the allele frequency and the effect (OR) of the SNP on the disease in studied populations were introduced into the logistic regression model. All statistical tests were two-tailed, and p < 0.05 was the criterion of statistical significance. In addition, FPRP analysis was used to further assess the robustness of the statistically significant associations. The threshold of the analysis was set to 0.2, and the prior probability was set to 0.1 to detect the efficacy for OR of 1.5 (risk) or 0.67 (protective), with an alpha level equal to the observed p-value (Wacholder et al., 2004; Zhu et al., 2013). LD and haplotype analysis were performed with the program SHEsis (http://27.223.74.230:8099/myAnalysis.php). The lowest frequency threshold was set as 0.01, and the haplotype with frequency less than 0.01 would be excluded from the analysis. The significance level (0.05/17 = 0.003) of Bonferroni’s correction was applied to account for multiple testing according to the number of designated SNPs in the study (Iskander et al., 2020; Yekutieli and Benjamini, 1999).
Results
The Characteristics of the Study Populations
The demographic characteristics of the study populations are listed in Table 1. There were no significant differences in age between HDP patients and controls (p > 0.05). However, the pre-pregnancy BMI (pre-BMI), systolic blood pressure (SBP), diastolic blood pressure (DBP), fasting blood glucose (FBG), triglyceride (TG), total cholesterol (TC), and high-density lipoprotein cholesterol (HDL-c) of HDP cases were higher than those of controls (p < 0.001).
Associations of SNPs in MT Genes With the Susceptibility of HDP Subtypes
Genotype distributions of the 17 selected SNPs in HDP and their subtypes, PCH, GH, and PE/PESCH, and controls are listed in Supplementary Table S2. All the genotype frequencies of the 17 SNPs agreed with the Hardy–Weinberg equilibrium in the study populations. In the logistic regression analysis, two, seven, and six SNPs were found to be significantly associated with PCH, GH, and PE/PESCH, respectively. After adjusting for age and pre-BMI, an increased risk of PCH was significantly associated with rs11076161 G variants in MT1A, and a decreased risk of PCH was associated with rs8044719 T variants in MT1DP. For GH, an increased risk was associated with rs11076161 G variants in MT1A, rs28366003 G variants in MT2A, and both of rs7191779 C and rs8052334 T variants in MT1B. A decreased risk of GH was significantly associated with rs10636 G variants in MT2A. For PE/PESCH, an increased risk was significantly associated with rs11076161 G variants in MT1A and rs11644094 G variants in MT3. Compared with individuals carrying the genotype CC of rs10636 in MT2A, those carrying the GC variants had a decreased risk, whereas the carriers with the GG variants of rs10636 had an increased risk of PE/PESCH compared with those carrying CG/CC alleles (Table 2).
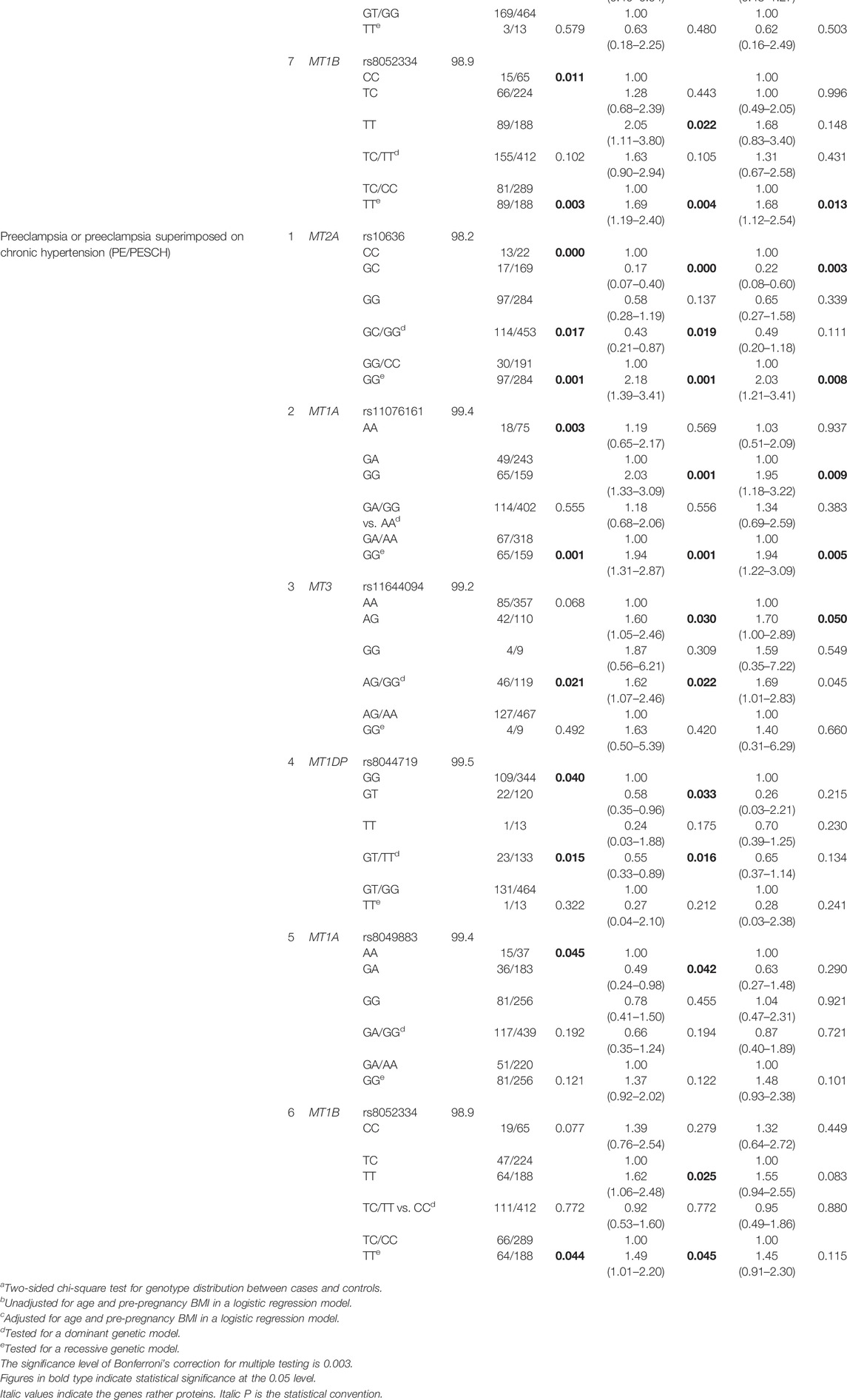
TABLE 2. Association between assayed SNPs in MT genes and the susceptibility of PCH, GH, and PE/PESCH.
To further test the associations estimated from the logistic regression analysis, FPRP analyses were conducted. Under a prior probability of 0.1, the OR for a specific genotype was estimated to be 0.67/1.5 (protection/susceptibility), and p < 0.2 was the criterion of statistical significance (Zhu et al., 2013). It turned out that the association of rs11076161 and rs8044719 with PCH and the association of rs10636 with GH remained (p < 0.2). However, the statistical powers of rs11076161 (GG vs. GA/AA) and rs8044719 (GT/TT vs. GG) related to PCH were only 0.180 and 0.052, respectively. Moreover, the association between SNPs in MTs and PE/PESCH disappeared (Table 4).
Associations of SNPs in MT Genes With HDP Susceptibility
Since oxidative stress and hypertension are the common pathophysiological processes of HDP (Phoswa and Khaliq, 2021), we merged HDP subtypes to estimate the association of disease risks with SNPs in MTs. In the single-locus analyses, seven SNPs were found to be related to HDP. After adjustment for age and pre-BMI, four SNPs including rs11076161 (OR = 2.02, 95% CI: 1.22–3.36 for GG vs. AA and OR = 1.89, 95% CI: 1.35–2.63 for GG vs. GA/AA) in MT1A; rs7191779 (OR = 1.52, 95% CI: 1.08–2.15 for CC vs. CG and OR = 1.54, 95% CI: 1.11–2.13 for CC vs. CG/GG) in MT1B; rs8044719 (OR = 0.65, 95% CI: 0.44–0.95 for GT/TT vs. GG) in MT1DP; and rs8052334 (OR = 1.53, 95% CI: 1.08–2.16 for TT vs. TC and OR = 1.52, 95% CI: 1.10–2.11 for TT vs. TC/CC) in MT1B remained to be associated with HDP. Furthermore, the association of decreased HDP risk with rs8052394 (OR = 0.69, 95% CI: 0.49–0.98 for GA vs. AA, OR = 0.69, 95% CI: 0.50–0.96 for GA/GG vs. AA) in MT1A and an increased HDP risk with rs8049883 (OR = 1.50, 95% CI: 1.08–2.09 for GA/GG vs. GG) in MT1A emerged (Table 3).
After the FPRP analyses, the four SNPs, that is, rs11076161, rs7191779, rs8044719, and rs8052334 had robust associations with HDP risks. In the heterozygous genotype comparison of rs8044719 (GT vs. GG), recessive models of rs11076161 (GG vs. GA/AA), rs7191779 (CC vs. CG/GG), and rs8052334 (TT vs. TC/CC), with their observed p values were 0.052, 0.013, 0.167, and 0.189, respectively (Table 4). Furthermore, the associations of HDP risks with rs8044719 and rs11076161 remained statistically significant following Bonferroni’s correction (Table 3).
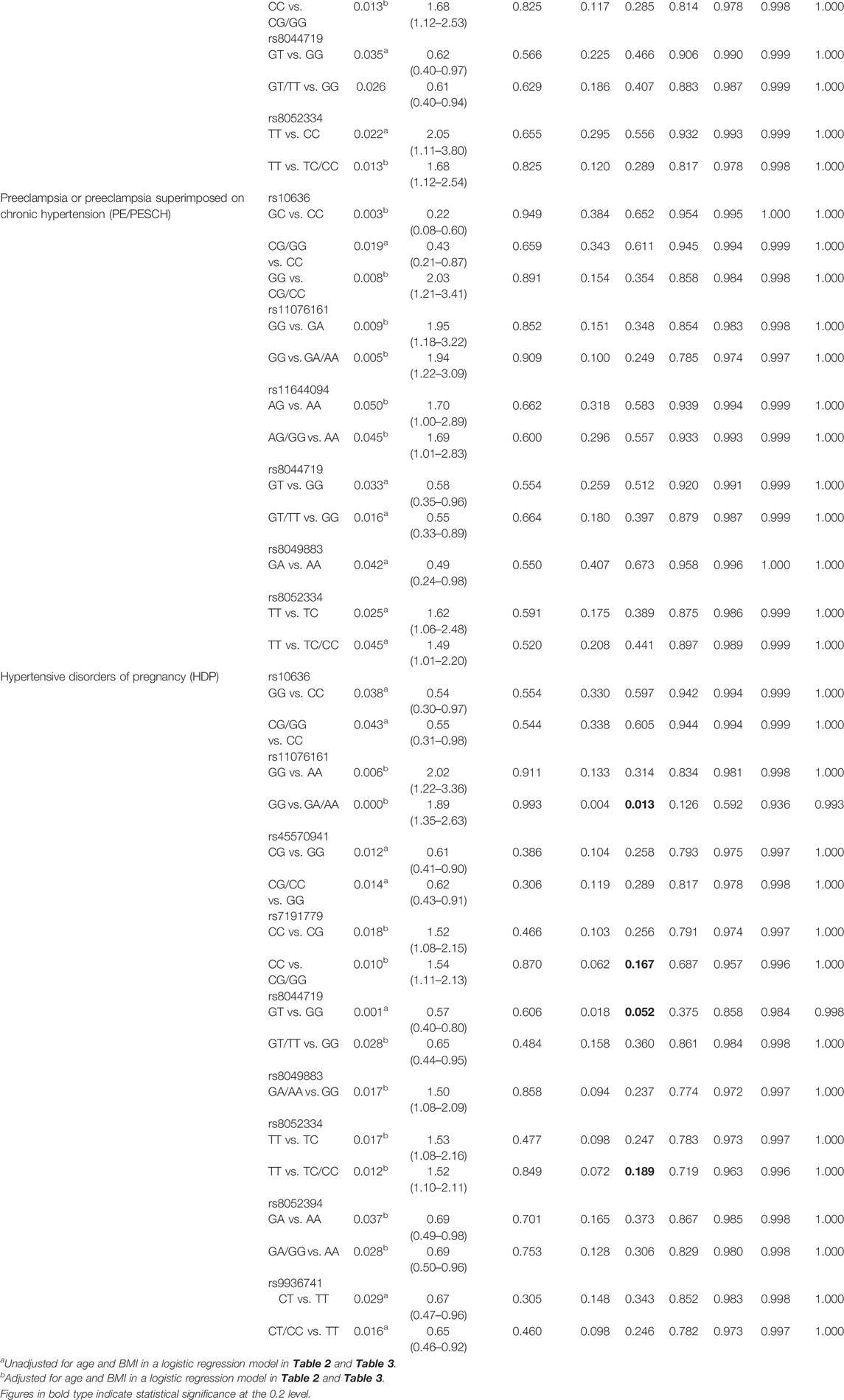
TABLE 4. False-positive report probability (FPRP) analysis for the association between SNPs in MT genes and the susceptibility of PCH, GH, PE/PESCH, and HDP.
The Linkage Disequilibrium and the Haplotype Analysis
The LD is assessed with the coefficient of linkage disequilibrium (|D’|) and the squared Pearson’s coefficient of correlation (r2) (Aumer et al., 2019). The SNPs with |D’| > 0.7 and r2 > 1/3 were chosen as tagSNP to conduct haplotype analysis. If r2 > 0.8, the two SNPs should be randomly picked up as one tagSNP(El et al., 2021; He et al., 2021; Yoshida et al., 2019). The LD analysis among the 17 designated SNPs in MT genes in PCH, GH, PE/PESCH, and HDP and their corresponding controls are shown in Supplementary Figures 1–4, respectively. There were 11 (rs11076161 G > A, rs11640851 C > A, rs1580833 T > G, rs1610216 C > T, rs1827208 C > T, rs7191779 C > G, rs7196890 C > A, rs8044719 G > T, rs8049883 G > A, rs8052394 C > T, and rs9936741 T > C), 10 (rs10636 C > G, rs11076161 G > A, rs11640851 C > A, rs1580833 T > G, rs1610216 C > T, rs28366003 A > G, rs7191779 C > G, rs7196890 C > A, rs8044719 G > T, and rs8049883 G > A), 10 (rs11076161 G > A, rs11640851 C > A, rs1580833 T > G, rs1610216 C > T, rs1827208 C > T, rs7191779 C > G, rs7196890 C > A, rs8044719 G > T, rs8049883 G > A, and rs9936741 T > C), and seven (rs11076161 G > A, rs11640851 C > A, rs1580833 T > G, rs1610216 C > T, rs7191779 C > G, rs7196890 C > A, and rs8049883 G > A) independent SNPs that were identified for haplotype analysis for PCH, GH, PE/PESCH, and HDP, respectively. The global p-value of the analysis for each group was lower than 0.0001. It turned out that eight (A-C-G-T-C-G-A-G-C-G-C, OR = 4.559; A-C-T-C-C-C-A-G-C-G-C, OR = 5.777; A-C-T-T-C-G-A-G-C-G-C, OR = 4.590; G-A-T-C-C-G-C-G-G-C-C, OR = 4.065; G-A-T-C-G-C-C-G-G-C-C, OR = 4.652; G-A-T-T-C-C-C-G-G-C-C, OR = 0.404; G-C-T-C-C-C-A-G-G-C-C, OR = 1.901; G-C-T-T-C-C-A-G-G-C-C, OR = 3.810), five (C-G-A-T-C-A-C-C-G-G, OR = 2.032; C-G-A-T-C-G-C-C-G-G, OR = 2.077; G-A-C-T-C-A-C-C-T-G, OR = 0.564; G-G-A-G-C-A-C-C-G-G, OR = 5.466; G-G-A-T-T-A-G-C-G-G, OR = 0.284), five (A-C-G-T-C-G-A-G-C-C, OR = 2.399; A-C-T-C-C-C-C-T-G-G, OR = 0.259; G-A-T-C-C-C-C-G-G-C, OR = 1.572; G-A-T-C-G-C-C-G-G-C, OR = 0.001; G-C-T-C-G-C-A-G-G-C, OR = 2.512), and five (A-C-T-C-C-C-G, OR = 0.634; G-A-G-C-C-C-G, OR = 4.047; G-A-T-T-G-C-G, OR = 0.499; G-C-G-T-C-A-G, OR = 7.299; G-C-T-C-C-A-G, OR = 1.434) haplotypes were significantly associated with PCH, GH, PE/PESCH, and HDP, respectively (Table 5).
Discussion
In the present study, rs10636 (MT2A) was identified to be significantly associated with GH risk. Other four SNPs, that is, rs11076161 (MT1A), rs7191779 (MT1B), rs8044719 (MT1DP), and rs8052334 (MT1B) in MT genes were identified to be significantly associated with HDP susceptibility. A joint effect of 11, 10, 10, and 7 SNPs in MT genes including MT1A, MT2A, MT1M, MT1B, and MT1DP on HDP susceptibility was identified in the haplotype analysis, showing that eight, five, five, and five haplotypes of MT alleles were significantly associated with the risks of PCH, GH, PESCH, and HDP, respectively.
Of the SNPs in MT genes associated with HDP susceptibility, both rs11076161 in MT1A and rs8052334 in MT1B are intron-located SNPs, which may modulate gene expression in a cumulative manner (Goebels et al., 2013). In addition, the SNP Function Prediction (FuncPred) showed that rs11076161 was related to the transcription factor binding site (TFBS). The genetic variations at the site may influence gene expression by altering the interaction between transcription factors and TFBS (Lai et al., 2019). This might affect the function of MT1A as an antioxidant and ultimately disturb the cellular redox homeostasis, and that may be one of the potential mechanisms by which the genetic variations in MT genes modify the individual susceptibility to HDP. Our study revealed that the HDP risk of rs11076161 GG variants was 1.89 times as high as that of the GA/AA alleles, while the HDP risk of rs8052334 TT variants was 1.52 times as high as that of TC/CC variants. It has been shown that rs11076161 is significantly associated with the oxidation-related diabetic neuropathy in patients with type 2 diabetes and the body’s load of cadmium (Lei et al., 2012) and that rs8052334 is associated to a decreased utilization of fatty acid (hyperlipidemia) in type 2 diabetes (Yang et al., 2008). The MT-1 A-G-T haplotypes (MT1A rs8052394 A allele, MT1B rs964372 G allele, and MT1B rs8052334 T allele) are related to an increased susceptibility of hepatocellular carcinoma (HCC), especially in those who smoke, presenting a notable combined effect of cigarette smoking and MT-1 haplotypes on HCC development (Wong et al., 2013).
The SNP rs10636 is located at the 3′ UTR region in the MT2A gene. Importantly, the 3′ UTR regions are often highly conserved and play an important role in modulating protein abundance through the regulation of translation or stability of mRNAs (Mayr, 2019). FuncPred analysis showed that rs10636 is related to TFBS as functioning with splicing and miRNA regulations. In our study, rs10636 G variants were identified to be associated with a decreased risk of GH. This result is consistent with the work of Liu et al. (2017) in which the genotype GG of rs10636 has a protective role in GH (Liu et al., 2017). The genetic combination of rs10636 GC-rs28366003 AA in MT2A presented less susceptibility to lead (Pb)-induced lipid oxidation than other genotypes (Yang et al., 2017).
MT expression is mainly regulated at the transcriptional level (Raudenska et al., 2014). In addition to the basal regulatory elements such as the TATA-box, GC-box, and basal level enhancer (BLE) sequences in the upstream of MT genes, there exist several inducible elements including metal response elements (MREs), antioxidant responsive elements (AREs), and glucocorticoid responsive elements (GRE) for regulating MT expression (Dalton et al., 1994; Raudenska et al., 2014). The SNP rs7191779 is located at 2 kb upstream of the MT1B gene and is related to the TFBS in FuncPred analyses. Our data showed that people with the CC genotype of rs7191779 demonstrated a 1.43-fold risk of those with GG/CG to develop HDP, suggesting that genetic variations may modify the susceptibility of HDP probably by regulating MT expression during pregnancy, which is more easily subjected to oxidative stress. It has been shown that some other oxidation-related conditions are also associated with the genetic variations. For example, the rs7191779 CC genotypes are presented as the risk factors of age-related macular degeneration (Garcia et al., 2017) and type 2 diabetes mellitus (Yang et al., 2008), whereas the rs7191779 GC genotypes experience significant protection against oral squamous cell carcinoma development (Zavras et al., 2011).
One of the most intriguing characteristics of MTs is the existence of 7 nonfunctional alleles in addition to 12 functional isoforms (Raudenska et al., 2014; McNeill et al., 2019). The MT1D has been predicted to be a non-active form (Gai et al., 2020). However, there exists an MT1D pseudogene (MT1DP) that is identified as a long noncoding RNA (lncRNA), which functions to aggravate oxidative stress by repressing antioxidation (Gai et al., 2020) and is known as an oncogene (Liao et al., 2021). MT1DP is proposed to be a result of gene duplicates to preserve the parental function and acts as backup compensation copies to buffer against the loss of a functionally related gene (Moleirinho et al., 2011). Liao et al. (2021)found that the combination of lncRNA MT1DP and miR-365 damaged the cell mitochondrial membrane to reduce mitochondrial function and the ability of ROS elimination and increased cell apoptosis. In our study, the SNP rs8044719 that is located at 500 bp downstream of MT1DP was associated with a decreased HDP risk. The carriers of the GT genotypes of rs8044719 were less likely to develop HDP than those carrying GG (OR = 0.57 for GT vs. GG). It is hence speculated that the GT genotypes of rs8044719 may attenuate the effect of MT1DP on repressing antioxidation to reduce the risk of HDP development.
The association of variations in MT genes with HDP was assessed by different approaches in this work. In the logistic regression model, two, seven, six, and seven SNPs were presented to be associated with the susceptibility of PCH, GH, PE/PESCH, and HDP, respectively. After tested by FPRP analysis, rs11076161 in MT1A and rs8044719 in MT1DP were related to PCH, whereas only rs10636 in MT2A was associated with GH. The association of MT variations with PE/PESCH disappeared. Four SNPs, that is, rs11076161 (MT1A), rs7191779 (MT1B), rs8044719 (MT1DP), and rs8052334 (MT1B) were associated with HDP. FPRP confirmed the noteworthy findings in the current study. Even in Bonferroni’s, test that is a very conservative correction procedure (White et al., 2019; Zill et al., 2004), rs8044719 and rs11076161 were still recognized to be associated with HDP. The statistical power was generally set as 80% for determining the sample size that may yield the acceptable probability estimates (Hong and Park, 2012). In this study, the post hoc power analysis showed that the power of rs10636 (GG vs. GC; GG vs. CG/CC), rs11076161 (GG vs. GA/AA), rs7191779 (CC vs. CG/GG), or rs8052334 (TT vs. TC/CC) was greater than 0.8. Although the power of rs8044719 (GT vs. GG) was 0.612, the association of the SNP with HDP risk was further tested with FPRP and maintained statistically significance. On the other hand, the powers of rs11076161 (GG vs. GA/AA) and rs8044719 (GT/TT vs. GG) related to PCH were low (0.180 and 0.052, respectively) as the probability of detecting the association might not be acceptable.
Haplotype analysis was used to identify the joint effects among studied SNPs. We found that the frequencies of seven haplotypes of MT alleles were higher and one haplotype was lower in PCH and the frequencies of three haplotypes were higher and two haplotypes were lower in GH, PESCH, and HDP than that in their corresponding controls. This evidence reveals not only the single-locus effect but a joint effect of SNPs in different MT genes on HDP susceptibility, yet the mechanisms remain to be further elucidated. It has been shown that the differential expression levels of MT isoforms present in different diseases and tumor types (Aquime et al., 2020; Hung et al., 2019; Saiegh et al., 2019; Sampaio et al., 2019), suggesting that MT isoforms may play distinct roles in pathophysiological processes under extreme conditions. MTs are highly evolutionarily conserved but non-essential; the antioxidative action of MTs is far stronger than that of superoxide dismutase (SOD) and GSH (Ma'Rifah et al., 2019; Thornalley and Vasak, 1985), and the latter is required for the recycling of oxidative and reduced forms of MTs (Kang, 2006). It, thus, appeared that MTs act as a backup of antioxidative systems, providing an amplification of the antioxidant activity in working with other antioxidants such as GSH and Nrf2 (Gu et al., 2017). The variation rs8044719 located at an MT pseudogene (MT1DP) was also considered to be a significant genetic factor affecting HDP susceptibility. This finding is reminiscent of the implication that the coexistence of the original and pseudogene genes may be related to the reconstruction and evolutionary history of the MT gene family (Moleirinho et al., 2011).
HDP has a common feature that is elevated blood pressure. Furthermore, PE has proteinuria (Phoswa, 2019). This implies that there exist different pathogenic contexts of PE from PCH or GH. Our data supported the notion by presenting that seven independent SNPs (rs11076161 G > A, rs11640851 C > A, rs1580833 T > G, rs1610216 C > T, rs7191779 C > G, rs7196890 C > A, and rs8049883 G > A) were related to all three subtypes of HDP in haplotype analysis, but no single SNP in MT genes is simultaneously associated with all subtypes. PE has been proposed to reduce uteroplacental perfusion as a result of abnormal cytotrophoblast invasion of spiral arterioles (Phoswa and Khaliq, 2021) and is closely related to oxidative stress in the placenta (Vitoratos et al., 2012), indicating more complicated etiological processes than that in other subtypes of HDP. In a study on the effect of SNPs in the renin–angiotensin–aldosterone system (RAAS) genes on HDP risks, it was shown that rs3789678 in the angiotensinogen gene was significantly associated with GH, whereas rs275645 in the angiotensin II receptor type 1 gene was significantly associated with PE (Li et al., 2016).
There are some advantages in our study. The associations between MT polymorphisms and HDP susceptibility were estimated with diverse methods, including logistic regression analysis and haplotype analysis, for accessing the possible effect of a single SNP or joint effects of studied multiple genetic variations on HDP risks. Moreover, the associations were further confirmed by FPRP analyses. However, this study also has several limitations. First, this study was a hospital-based case-control study design, which may be affected by selection bias. Second, the selected SNPs do not include all functional SNPs in MT genes, which results in missing some meaningful information for functional MTs. Third, the study lacked the ability to assess gene–environment interactions. A nested case-control study based on the prospective cohort with a larger sample size should be conducted to further elucidate the issues.
Conclusion
The genetic variation rs10636 G in MT2A was related to a decreased risk of gestational hypertension. Other four SNPs (rs11076161 in MT1A, rs7191779 in MT1B, rs8044719 in MT1DP, and rs8052334 in MT1B) in MT genes are individually associated with HDP susceptibility. The frequencies of seven haplotypes of MT alleles were higher and those of one haplotype were lower in PCH, and the frequencies of three haplotypes were higher and those of two haplotypes were lower in GH, PESCH, and HDP than those in their corresponding controls. These variations and their haplotypes may be used as early warning genetic markers to predict HDP for women of childbearing age at high risk.
Data Availability Statement
The original contributions presented in the study are publicly available. This data can be found on Dryad with the doi:10.5061/dryad.1c59zw3xz
Ethics Statement
The studies involving human participants were reviewed and approved by Medical Ethics Committee of Guilin Medical University. The patients/participants provided their written informed consent to participate in this study.
Author Contributions
BH, SW, and XY contributed to the conception and design of the study. XY and QL organized the database. SW and XW performed the statistical analysis. SW wrote the first draft of the manuscript. BH, XY, MZ, and PZ wrote sections of the manuscript. All authors contributed to manuscript revision, read, and approved the submitted version.
Funding
This study was supported by the Key Research and Development Program of Guangxi (Grant Number 2018AB62004), the National Natural Science Foundation of China (Grant Number 81760614), and the Basic Ability Improvement Project of Young and Middle-aged Teachers in College of Guangxi Education Department (Grant Number 2020KY 2028).
Conflict of Interest
The authors declare that the research was conducted in the absence of any commercial or financial relationships that could be construed as a potential conflict of interest.
Publisher’s Note
All claims expressed in this article are solely those of the authors and do not necessarily represent those of their affiliated organizations, or those of the publisher, the editors, and the reviewers. Any product that may be evaluated in this article, or claim that may be made by its manufacturer, is not guaranteed or endorsed by the publisher.
Acknowledgments
The authors thank the Affiliated Hospital of Guilin Medical University for their support.
Supplementary Material
The Supplementary Material for this article can be found online at: https://www.frontiersin.org/articles/10.3389/fgene.2022.830446/full#supplementary-material
References
Álvarez-Barrios, A., Álvarez, L., García, M., Artime, E., Pereiro, R., and González-Iglesias, H. (2021). Antioxidant Defenses in the Human Eye: A Focus on Metallothioneins. Antioxidants 10 (1), 89. doi:10.3390/antiox10010089
Aquime, J. R. H. S., Zampieri, L. C. D. A. P., Kataoka, M. S. d. S., Ribeiro, N. A. B., Jaeger, R. G., da Silva, A. L., et al. (2020). Metallothionein Expression and its Influence on the In Vitro Biological Behavior of Mucoepidermoid Carcinoma. Cells 9 (1), 157. doi:10.3390/cells9010157
Aumer, D., Stolle, E., Allsopp, M., Mumoki, F., Pirk, C. W. W., and Moritz, R. F. A. (2019). A Single SNP Turns a Social Honey Bee (Apis mellifera) Worker into a Selfish Parasite. Mol. Biol. Evol. 36 (3), 516–526. doi:10.1093/molbev/msy232
Bizerea, T., Dezsi, S., Marginean, O., Stroescu, R., Rogobete, A., Bizerea-Spiridon, O., et al. (2018). The Link between Selenium, Oxidative Stress and Pregnancy Induced Hypertensive Disorders. Clin. Lab. 64 (10), 1593–1610. doi:10.7754/Clin.Lab.2018.180307
Bizoń, A., Jędryczko, K., and Milnerowicz, H. (2017). The Role of Metallothionein in Oncogenesis and Cancer Treatment. Postepy Hig. Med. Dosw 71 (0), 98–109. doi:10.5604/01.3001.0010.3794
Brown, M. A., Magee, L. A., Kenny, L. C., Karumanchi, S. A., McCarthy, F. P., Saito, S., et al. (2018). Hypertensive Disorders of Pregnancy. Hypertension 72 (1), 24–43. doi:10.1161/HYPERTENSIONAHA.117.10803
Cao, W., Dong, M., Sun, X., Liu, X., Xiao, J., Feng, B., et al. (2020). Associations of Maternal Ozone Exposures during Pregnancy with Maternal Blood Pressure and Risk of Hypertensive Disorders of Pregnancy: A Birth Cohort Study in Guangzhou, China. Environ. Res. 183, 109207. doi:10.1016/j.envres.2020.109207
Dalton, T., Palmiter, R. D., and Andrews, G. K. (1994). Transcriptional Induction of the Mouse Metallothionein-I Gene in Hydrogen Peroxide-Treated Hepa Cells Involves a Composite Major Late Transcription Factor/antioxidant Response Element and Metal Response Promoter Elements. Nucl. Acids Res. 22 (23), 5016–5023. doi:10.1093/nar/22.23.5016
Draganovic, D., Lucic, N., and Jojic, D. (2016). Oxidative Stress Marker and Pregnancy Induced Hypertension. Med. Arh. 70 (6), 437–440. doi:10.5455/medarh.2016.70.437-440
Dutsch-Wicherek, M., Sikora, J., and Tomaszewska, R. (2008). The Possible Biological Role of Metallothionein in Apoptosis. Front. Biosci. 13, 4029–4038. doi:10.2741/2991
El Hou, A., Rocha, D., Venot, E., Blanquet, V., and Philippe, R. (2021). Long-range Linkage Disequilibrium in French Beef Cattle Breeds. Genet. Sel. Evol. 53 (1), 63. doi:10.1186/s12711-021-00657-8
Ferreira, L. C., Gomes, C. E. M., Duggal, P., De Paula Holanda, I., de Lima, A. S., do Nascimento, P. R. P., et al. (2021). Genetic Association of ERAP1 and ERAP2 with Eclampsia and Preeclampsia in Northeastern Brazilian Women. Sci. Rep. 11 (1), 6764. doi:10.1038/s41598-021-86240-z
Gai, C., Liu, C., Wu, X., Yu, M., Zheng, J., Zhang, W., et al. (2020). MT1DP Loaded by Folate-Modified Liposomes Sensitizes Erastin-Induced Ferroptosis via Regulating miR-365a-3p/NRF2 axis in Non-small Cell Lung Cancer Cells. Cell Death Dis. 11 (9), 751. doi:10.1038/s41419-020-02939-3
García, M., Álvarez, L., Fernández, Á., González-Iglesias, H., Escribano, J., Fernández-Vega, B., et al. (2017). Metallothionein Polymorphisms in a Northern Spanish Population with Neovascular and Dry Forms of Age-Related Macular Degeneration. Ophthalmic Genet. 38 (5), 451–458. doi:10.1080/13816810.2017.1288825
Goebels, C., Thonn, A., Gonzalez-Hilarion, S., Rolland, O., Moyrand, F., Beilharz, T. H., et al. (2013). Introns Regulate Gene Expression in Cryptococcus Neoformans in a Pab2p Dependent Pathway. PLoS Genet. 9 (8), e1003686. doi:10.1371/journal.pgen.1003686
Gu, J., Cheng, Y., Wu, H., Kong, L., Wang, S., Xu, Z., et al. (2017). Metallothionein Is Downstream of Nrf2 and Partially Mediates Sulforaphane Prevention of Diabetic Cardiomyopathy. Diabetes 66 (2), 529–542. doi:10.2337/db15-1274
He, T., Liu, M., Tao, D., Leng, X., Wang, Z., Xie, S., et al. (2021). Is BRD7 Associated with Spermatogenesis Impairment and Male Infertility in Humans? A Case-Control Study in a Han Chinese Population. Basic Clin. Androl. 31 (1), 19. doi:10.1186/s12610-021-00139-3
Hong, E. P., and Park, J. W. (2012). Sample Size and Statistical Power Calculation in Genetic Association Studies. Genomics Inf. 10 (2), 117–122. doi:10.5808/GI.2012.10.2.117
Hung, K.-C., Huang, T.-C., Cheng, C.-H., Cheng, Y.-W., Lin, D.-Y., Fan, J.-J., et al. (2019). The Expression Profile and Prognostic Significance of Metallothionein Genes in Colorectal Cancer. Ijms 20 (16), 3849. doi:10.3390/ijms20163849
Iskander, D. R., Consejo, A., Solarski, J., Karnowski, K., and Wojtkowski, M. (2020). Corneal Properties of Glaucoma Based on Scheimpflug Light Intensity Distribution. ARVO 61 (7), 4716. doi:10.1167/iovs.19-26963
Jarosz, M., Olbert, M., Wyszogrodzka, G., Młyniec, K., and Librowski, T. (2017). Antioxidant and Anti-inflammatory Effects of Zinc. Zinc-dependent NF-Κb Signaling. Inflammopharmacol 25 (1), 11–24. doi:10.1007/s10787-017-0309-4
Jin, R., Chow, V. T., Tan, P. H., Dheen, S. T., Duan, W., and Bay, B. H. (2002). Metallothionein 2A Expression Is Associated with Cell Proliferation in Breast Cancer. Carcinogenesis 23 (1), 81–86. doi:10.1093/carcin/23.1.81
Kang, Y. J. (2006). Metallothionein Redox Cycle and Function. Exp. Biol. Med. (Maywood) 231 (9), 1459–1467. doi:10.1177/153537020623100903
Khan, K. S., Wojdyla, D., Say, L., Gülmezoglu, A. M., and Van Look, P. F. (2006). WHO Analysis of Causes of Maternal Death: A Systematic Review. Lancet 367 (9516), 1066–1074. doi:10.1016/S0140-6736(06)68397-9
Khedagi, A. M., and Bello, N. A. (2021). Hypertensive Disorders of Pregnancy. Cardiol. Clin. 39 (1), 77–90. doi:10.1016/j.ccl.2020.09.005
Lai, X., Stigliani, A., Vachon, G., Carles, C., Smaczniak, C., Zubieta, C., et al. (2019). Building Transcription Factor Binding Site Models to Understand Gene Regulation in Plants. Mol. Plant 12 (6), 743–763. doi:10.1016/j.molp.2018.10.010
Lei, L., Chang, X., Rentschler, G., Tian, L., Zhu, G., Chen, X., et al. (2012). A Polymorphism in Metallothionein 1A (MT1A) Is Associated with Cadmium-Related Excretion of Urinary Beta 2‐microglobulin. Toxicol. Appl. Pharmacol. 265 (3), 373–379. doi:10.1016/j.taap.2012.09.006
Li, X., Tan, H., Zhou, S., Hu, S., Zhang, T., Li, Y., et al. (2016). Renin-angiotensin-aldosterone System Gene Polymorphisms in Gestational Hypertension and Preeclampsia: A Case-Control Gene-Association Study. Sci. Rep. 6, 38030. doi:10.1038/srep38030
Liao, Z.-W., Fan, Z.-W., Huang, Y., Liang, C.-Y., Liu, C., Huang, S., et al. (2021). Long Non-coding RNA MT1DP Interacts with miR-365 and Induces Apoptosis of Nucleus Pulposus Cells by Repressing NRF-2-Induced Anti-oxidation in Lumbar Disc Herniation. Ann. Transl. Med. 9 (2), 151. doi:10.21037/atm-20-8123
Liu, D., Wang, M., Tian, T., Wang, X.-J., Kang, H.-F., Jin, T.-B., et al. (2017). Genetic Polymorphisms (Rs10636 and Rs28366003) in Metallothionein 2A Increase Breast Cancer Risk in Chinese Han Population. Aging 9 (2), 547–555. doi:10.18632/aging.101177
Lu, C.-Q., Lin, J., Yuan, L., Zhou, J.-G., Liang, K., Zhong, Q.-H., et al. (2018). Pregnancy Induced Hypertension and Outcomes in Early and Moderate Preterm Infants. Pregnancy Hypertens. 14, 68–71. doi:10.1016/j.preghy.2018.06.008
Ma’rifah, F., Saputri, M. R., Soegianto, A., Irawan, B., and Putranto, T. W. C. (2019). The Change of Metallothionein and Oxidative Response in Gills of the Oreochromis niloticus after Exposure to Copper. Animals 9 (6), 353. doi:10.3390/ani9060353
Mayr, C. (2019). What Are 3′ UTRs Doing? Cold Spring Harb. Perspect. Biol. 11 (10), a034728. doi:10.1101/cshperspect.a034728
McNeill, R., Mason, A., Hodson, M., Catto, J., and Southgate, J. (2019). Specificity of the Metallothionein-1 Response by Cadmium-Exposed Normal Human Urothelial Cells. Ijms 20 (6), 1344. doi:10.3390/ijms20061344
Moleirinho, A., Carneiro, J., Matthiesen, R., Silva, R. M., Amorim, A., and Azevedo, L. (2011). Gains, Losses and Changes of Function after Gene Duplication: Study of the Metallothionein Family. PLoS One 6 (4), e18487. doi:10.1371/journal.pone.0018487
Moses, E. K., Lade, J. A., Guo, G., Wilton, A. N., Grehan, M., Freed, K., et al. (2000). A Genome Scan in Families from Australia and New Zealand Confirms the Presence of a Maternal Susceptibility Locus for Pre-eclampsia, on Chromosome 2. Am. J. Hum. Genet. 67 (6), 1581–1585. doi:10.1086/316888
Padula, A. M., Ma, C., Huang, H., Morello-Frosch, R., Woodruff, T. J., and Carmichael, S. L. (2021). Drinking Water Contaminants in California and Hypertensive Disorders in Pregnancy. Environ. Epidemiol. 5 (2), e149. doi:10.1097/EE9.0000000000000149
Phoswa, W. N. (2019). Dopamine in the Pathophysiology of Preeclampsia and Gestational Hypertension: Monoamine Oxidase (MAO) and Catechol-O-Methyl Transferase (COMT) as Possible Mechanisms. Oxidative Med. Cell. Longev. 2019, 3546294. doi:10.1155/2019/3546294
Phoswa, W. N., and Khaliq, O. P. (2021). The Role of Oxidative Stress in Hypertensive Disorders of Pregnancy (Preeclampsia, Gestational Hypertension) and Metabolic Disorder of Pregnancy (Gestational Diabetes Mellitus). Oxidative Med. Cell. Longev. 2021, 1–10. doi:10.1155/2021/5581570
Rahimmadar, S., Ghaffari, M., Mokhber, M., and Williams, J. L. (2021). Linkage Disequilibrium and Effective Population Size of Buffalo Populations of iran, turkey, pakistan, and egypt Using a Medium Density SNP Array. Front. Genet. 12, 608186. doi:10.3389/fgene.2021.608186
Raudenska, M., Gumulec, J., Podlaha, O., Sztalmachova, M., Babula, P., Eckschlager, T., et al. (2014). Metallothionein Polymorphisms in Pathological Processes. Metallomics 6 (1), 55–68. doi:10.1039/c3mt00132f
Rozas-Villanueva, M. F., Casanello, P., and Retamal, M. A. (2020). Role of ROS/RNS in Preeclampsia: Are Connexins the Missing Piece? Ijms 21 (13), 4698. doi:10.3390/ijms21134698
Saiegh, L., Sheikh-Ahmad, M., Shechner, C., Reut, M., Darawsha, Y., Zolotov, S., et al. (2019). Metallothionein Protein and Minichromosome Maintenance Protein-2 Expression in Adrenocortical Tumors. Ann. d'Endocrinologie 80 (5-6), 324–328. doi:10.1016/j.ando.2019.09.003
Sampaio, F. A., Martins, L. M., Dourado, C. S. d. M. E., Revoredo, C. M. S., Costa-Silva, D. R., Oliveira, V. A. d., et al. (2019). A Case-Control Study of Metallothionein-1 Expression in Breast Cancer and Breast Fibroadenoma. Sci. Rep. 9 (1), 7407. doi:10.1038/s41598-019-43565-0
Seamon, K., Kurlak, L. O., Warthan, M., Stratikos, E., Strauss, J. F., Mistry, H. D., et al. (2020). The Differential Expression of ERAP1/ERAP2 and Immune Cell Activation in Pre-eclampsia. Front. Immunol. 11, 396. doi:10.3389/fimmu.2020.00396
Sekovanić, A., Jurasović, J., and Piasek, M. (2020). Metallothionein 2A Gene Polymorphisms in Relation to Diseases and Trace Element Levels in Humans. Arh. Hig. Rada Toksikol. 71 (1), 27–47. doi:10.2478/aiht-2020-71-3349
Shabb, J. B., Muhonen, W. W., and Mehus, A. A. (2017). Quantitation of Human Metallothionein Isoforms in Cells, Tissues, and Cerebrospinal Fluid by Mass Spectrometry. Methods Enzymol. 586, 413–431. doi:10.1016/bs.mie.2016.11.004
Shah, S., and Gupta, A. (2019). Hypertensive Disorders of Pregnancy. Cardiol. Clin. 37 (3), 345–354. doi:10.1016/j.ccl.2019.04.008
Si, M., and Lang, J. (2018). The Roles of Metallothioneins in Carcinogenesis. J. Hematol. Oncol. 11 (1), 107. doi:10.1186/s13045-018-0645-x
Sorriento, D., De Luca, N., Trimarco, B., and Iaccarino, G. (2018). The Antioxidant Therapy: New Insights in the Treatment of Hypertension. Front. Physiol. 9, 258. doi:10.3389/fphys.2018.00258
Stanhope, K. K., Adeyemi, D. I., Li, T., Johnson, T., and Boulet, S. L. (2021). The Relationship between the Neighborhood Built and Social Environment and Hypertensive Disorders of Pregnancy: A Scoping Review. Ann. Epidemiol. 64, 67–75. doi:10.1016/j.annepidem.2021.09.005
Su, X., Zhao, Y., Yang, Y., and Hua, J. (2020). Correlation between Exposure to Fine Particulate Matter and Hypertensive Disorders of Pregnancy in Shanghai, China. Environ. Health 19 (1), 101. doi:10.1186/s12940-020-00655-1
Tanaka, K., Nishigori, H., Nishigori, H., Watanabe, Z., Iwama, N., Satoh, M., et al. (2019). Higher Prevalence of Hypertensive Disorders of Pregnancy in Women Who Smoke: The Japan Environment and Children's Study. Hypertens. Res. 42 (4), 558–566. doi:10.1038/s41440-019-0206-x
Thakoordeen, S., Moodley, J., and Naicker, T. (2018). Candidate Gene, Genome-wide Association and Bioinformatic Studies in Pre-eclampsia: A Review. Curr. Hypertens. Rep. 20 (10), 91. doi:10.1007/s11906-018-0891-x
Thornalley, P. J., and Vašák, M. (1985). Possible Role for Metallothionein in Protection against Radiation-Induced Oxidative Stress. Kinetics and Mechanism of its Reaction with Superoxide and Hydroxyl Radicals. Biochimica Biophysica Acta (BBA) - Protein Struct. Mol. Enzym. 827 (1), 36–44. doi:10.1016/0167-4838(85)90098-6
Umesawa, M., and Kobashi, G. (2017). Epidemiology of Hypertensive Disorders in Pregnancy: Prevalence, Risk Factors, Predictors and Prognosis. Hypertens. Res. 40 (3), 213–220. doi:10.1038/hr.2016.126
Vitoratos, N., Vrachnis, N., Iavazzo, C., and Kyrgiou, M. (2012). Preeclampsia: Molecular Mechanisms, Predisposition, and Treatment. J. Pregnancy 2012, 145487. doi:10.1155/2012/145487
Wacholder, S., Chanock, S., Garcia-Closas, M., El ghormli, L., and Rothman, N. (2004). Assessing the Probability that a Positive Report Is False: An Approach for Molecular Epidemiology Studies. J. Natl. Cancer Inst. 96 (6), 434–442. doi:10.1093/jnci/djh075
Wang, L., Xin, F., Lin, N., Wang, Y., Liu, X., and Liu, J. (2018). Metallothioneins May Be a Potential Prognostic Biomarker for Tumors. Med. Baltim. 97 (52), e13786. doi:10.1097/MD.0000000000013786
White, T., van der Ende, J., and Nichols, T. E. (2019). Beyond Bonferroni Revisited: Concerns over Inflated False Positive Research Findings in the Fields of Conservation Genetics, Biology, and Medicine. Conserv. Genet. 20 (4), 927–937. doi:10.1007/s10592-019-01178-0
Wilkerson, R. G., and Ogunbodede, A. C. (2019). Hypertensive Disorders of Pregnancy. Emerg. Med. Clin. N. Am. 37 (2), 301–316. doi:10.1016/j.emc.2019.01.008
Wisdom, S. J., Wilson, R., McKillop, J. H., and Walker, J. J. (1991). Antioxidant Systems in Normal Pregnancy and in Pregnancy-Induced Hypertension. Am. J. Obstet. Gynecol. 165 (6 Pt 1), 1701–1704. doi:10.1016/0002-9378(91)90018-m
Wong, R.-H., Huang, C.-H., Yeh, C.-B., Lee, H.-S., Chien, M.-H., and Yang, S.-F. (2013). Effects of Metallothionein-1 Genetic Polymorphism and Cigarette Smoking on the Development of Hepatocellular Carcinoma. Ann. Surg. Oncol. 20 (6), 2088–2095. doi:10.1245/s10434-012-2456-6
Xu, Z., Kaplan, N. L., and Taylor, J. A. (2007). TAGster: Efficient Selection of LD Tag SNPs in Single or Multiple Populations. Bioinformatics 23 (23), 3254–3255. doi:10.1093/bioinformatics/btm426
Xue, W., Liu, Y., Zhao, J., Cai, L., Li, X., and Feng, W. (2012). Activation of HIF-1 by Metallothionein Contributes to Cardiac Protection in the Diabetic Heart. Am. J. Physiology-Heart Circulatory Physiology 302 (12), H2528–H2535. doi:10.1152/ajpheart.00850.2011
Yang, C.-C., Chuang, C.-S., Lin, C.-I., Wang, C.-L., Huang, Y.-C., and Chuang, H.-Y. (2017). The Association of the Blood Lead Level and Serum Lipid Concentrations May Be Modified by the Genetic Combination of the Metallothionein 2A Polymorphisms Rs10636 GC and Rs28366003 AA. J. Clin. Lipidol. 11 (1), 234–241. doi:10.1016/j.jacl.2016.12.010
Yang, L., Li, H., Yu, T., Zhao, H., Cherian, M. G., Cai, L., et al. (2008). Polymorphisms in Metallothionein-1 and -2 Genes Associated with the Risk of Type 2 Diabetes Mellitus and its Complications. Am. J. Physiology-Endocrinology Metabolism 294 (5), E987–E992. doi:10.1152/ajpendo.90234.2008
Yekutieli, D., and Benjamini, Y. (1999). Resampling-based False Discovery Rate Controlling Multiple Test Procedures for Correlated Test Statistics. J. Stat. Plan. Infer. 82 (1), 171–196. doi:10.1016/S0378-3758(99)00041-5
Yoshida, G. M., Barria, A., Correa, K., Cáceres, G., Jedlicki, A., Cadiz, M. I., et al. (2019). Genome-Wide Patterns of Population Structure and Linkage Disequilibrium in Farmed Nile tilapia (Oreochromis niloticus). Front. Genet. 10, 745. doi:10.3389/fgene.2019.00745
Zavras, A. I., Yoon, A. J., Chen, M. K., Lin, C. W., and Yang, S. F. (2011). Metallothionein-1 Genotypes in the Risk of Oral Squamous Cell Carcinoma. Ann. Surg. Oncol. 18 (5), 1478–1483. doi:10.1245/s10434-010-1431-3
Zhang, F., Guo, S., Zhong, W., Huang, K., and Liu, Y. (2021). Integrative Analysis of Metallothioneins Identifies MT1H as Candidate Prognostic Biomarker in Hepatocellular Carcinoma. Front. Mol. Biosci. 8, 672416. doi:10.3389/fmolb.2021.672416
Zhang, Y., Hao, J., Xu, X., Chen, X., and Wang, J. (2020). Protein Corona-Triggered Catalytic Inhibition of Insufficient POSS Polymer-Caged Gold Nanoparticles for Sensitive Colorimetric Detection of Metallothioneins. Anal. Chem. 92 (2), 2080–2087. doi:10.1021/acs.analchem.9b04593
Zhou, Y., Wang, R., Chen, S. X., Wang, R., Chen, S. X., Wu, L. S., et al. (2021). Matrix Metalloproteinase 9 Gene Promoter Region -1562 C/T Single Nucleotide Polymorphism Increases the Susceptibility to Hypertensive Disorders of Pregnancy: A Meta-Analysis. Clin. Exp. Obstet. Gyn. 48 (2), 245–252. doi:10.31083/j.ceog.2021.02.2265
Zhu, M.-L., Yu, H., Shi, T.-Y., He, J., Wang, M.-Y., Li, Q.-X., et al. (2013). Polymorphisms in mTORC1 Genes Modulate Risk of Esophageal Squamous Cell Carcinoma in Eastern Chinese Populations. J. Thorac. Oncol. 8 (6), 788–795. doi:10.1097/JTO.0b013e31828916c6
Keywords: hypertensive disorders of pregnancy, metallothionein, single-nucleotide polymorphism, case-control study, genetic susceptibility
Citation: Wei S, Yu X, Wen X, Zhang M, Lang Q, Zhong P and Huang B (2022) Genetic Variations in Metallothionein Genes and Susceptibility to Hypertensive Disorders of Pregnancy: A Case-Control Study. Front. Genet. 13:830446. doi: 10.3389/fgene.2022.830446
Received: 05 January 2022; Accepted: 19 April 2022;
Published: 06 June 2022.
Edited by:
Jared C. Roach, Institute for Systems Biology (ISB), United StatesReviewed by:
Jaclyn Goodrich, University of Michigan, United StatesPatricia Canto, Universidad Nacional Autónoma de México, Mexico
Copyright © 2022 Wei, Yu, Wen, Zhang, Lang, Zhong and Huang. This is an open-access article distributed under the terms of the Creative Commons Attribution License (CC BY). The use, distribution or reproduction in other forums is permitted, provided the original author(s) and the copyright owner(s) are credited and that the original publication in this journal is cited, in accordance with accepted academic practice. No use, distribution or reproduction is permitted which does not comply with these terms.
*Correspondence: Bo Huang, Qm8uSHVhbmdAZ2xtYy5lZHUuY24=
†These authors have contributed equally to this work and share first authorship