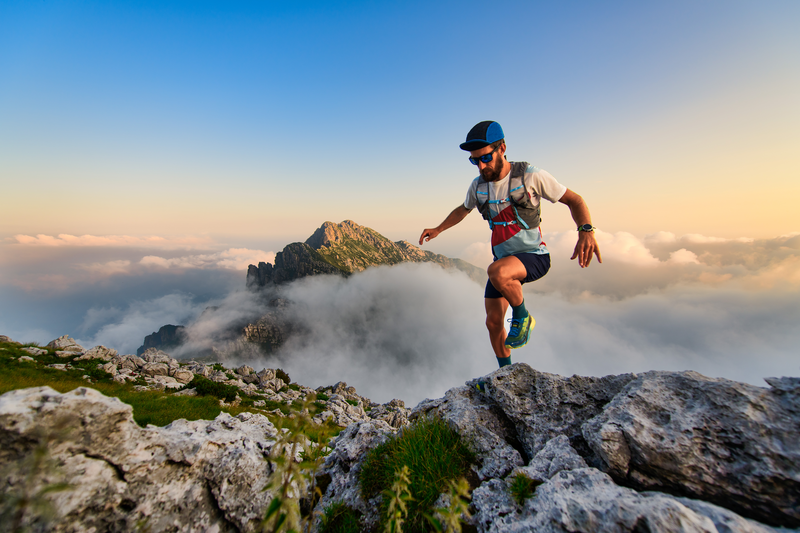
94% of researchers rate our articles as excellent or good
Learn more about the work of our research integrity team to safeguard the quality of each article we publish.
Find out more
MINI REVIEW article
Front. Genet. , 06 January 2023
Sec. Neurogenomics
Volume 13 - 2022 | https://doi.org/10.3389/fgene.2022.1114762
This article is part of the Research Topic Brain Cancer Pathogenesis and Data Integration View all 5 articles
Malignant primary brain tumors are the most common cancer in children aged 0–14 years, and are the most common cause of death among pediatric cancer patients. Compared to other cancers, pediatric brain tumors have been difficult to diagnose and study given the high risk of intracranial biopsy penetrating through vital midline structures, where the majority of pediatric brain tumors originate (Ostrom et al., 2015). Furthermore, the vast majority of these tumors recur. With limitations in the ability to monitor using clinical and radiographic methods alone, minimally invasive methods such as liquid biopsy will be crucial to our understanding and treatment. Liquid biopsy of blood, urine, and cerebrospinal fluid (CSF) can be used to sample cfDNA, ctDNA, RNA, extracellular vesicles, and tumor-associated proteins. In the past year, four seminal papers have made significant advances in the use of liquid biopsy in pediatric brain tumor patients (Liu et al., 2021; Cantor et al., 2022; Miller et al., 2022; Pagès et al., 2022). In this review, we integrate the results of these studies and others to discuss how the newest technologies in liquid biopsy are being developed for molecular diagnosis and treatment response in pediatric brain tumors.
The most common cause of death among pediatric cancer patients aged 0–14 years are malignant primary brain tumors (Ostrom et al., 2020). Unfortunately, diagnosis via clinical evaluation and MRI imaging is uncertain, with a differential diagnosis including abscesses and tumor variants requiring discordant treatments. Therefore, current clinical decision-making requires surgical biopsy or resection for histopathologic diagnosis. Even the most minimally invasive option–stereotactic needle biopsy–puts the patient at risk of side effects of general anesthesia, intracranial hemorrhage, neurologic deficit, and death (Nishihara et al., 2011; Hamisch et al., 2017; He et al., 2021). Furthermore, tissue biopsy samples a single point, failing to capture intratumoral heterogeneity for accurate diagnosis (Ng and Lim, 2008). It has previously been shown that neuropathologic diagnosis via stereotactic biopsy specimen versus tumor resection specimen of the same lesion differed in approximately 40% of cases (Jackson et al., 2001). The development of a safer, more accurate diagnostic tool would revolutionize the treatment of CNS tumors.
Similarly, the current paradigm of clinical and radiographic tumor monitoring during and following treatment is ineffective in guiding clinical decision-making due to radiographic changes with poor sensitivity and specificity for true tumor growth. So-called pseudoprogression–radiographic tumor growth and/or clinical deteriorationis a result of treatment-effect: Inflammatory changes which can be controlled with steroids (Thust et al., 2018). A reliable, non-invasive method for differentiation between progression and pseudoprogression would obviate the need for repeat biopsy and facilitate early treatment.
Histopathologic criteria once dominated brain tumor diagnosis, however, the newer WHO guidelines are progressively shifting toward molecular and genomic parameters (Louis et al., 2016; Kurokawa et al., 2022). A comprehensive method for DNA-methylation-based classification has been established and shown to correct diagnosis in up to 12% of prospective cases (Capper et al., 2018). While imaging fails to capture the genetic tumor profile, liquid biopsy of blood, urine, or cerebrospinal fluid (CSF) for isolation of genetic biomarkers including ctDNA, RNA, and tumor-associated proteins is an emerging method for diagnosis and monitoring of CNS malignancies (Wadden et al., 2022).
Given its ability to transcend the safety concerns and diagnostic limitations of stereotactic biopsy, the development and application of liquid biopsy in clinical CNS tumor management is critical. In this review, we will discuss the latest technologies in biofluid isolation and interpretation, as well as previously identified biomarkers for detection and tracking of various pediatric CNS tumors. Finally, we will discuss the applicability of these techniques and future integration into the current clinical system.
Due to a lower total biofluid volume in pediatric patients, the ability to isolate and identify genetic and molecular components from small samples is crucial. Here we will discuss general principles of liquid biopsy in pediatric brain tumors and showcase the feasibility of tumor detection using biofluids (as seen in Table 1). Blood (plasma or serum), urine, and CSF have been identified as potential liquid biopsy candidates in pediatric patients with gliomas (primarily diffuse intrinsic pontine glioma) and embryonal neoplasms (medulloblastoma).
TABLE 1. A summary of current liquid biopsy detection methods using varied biofluids, tumor types, and targets.
PCR (polymerase chain reaction) amplifies DNA using a combination of primers, dNTP, and DNA polymerase. Specifically, quantitative PCR captures real-time amplification of targets using fluorescently assigned probes. ddPCR furthermore allows for unbiased amplification of a known target by isolating reactions in each of thousands of droplets (Martínez-Ricarte et al., 2018; Panditharatna et al., 2018). ddPCR is a sensitive and accurate method that can detect rare ctDNA mutations with a limit of detection of approximately .001% in CNS tumors (Hindson et al., 2011; Izquierdo et al., 2021; Li et al., 2021). To demonstrate the utility of this technique, one group used blood ddPCR to amplify ctDNA and found that it was possible to detect the H3.3K27M mutation in 85% of diffuse intrinsic pontine glioma (DIPG) patients at diagnosis and 100% of patients post-radiation or during therapy (Mueller et al., 2019).
ddPCR can only detect known targets via the addition of targeted primers and probes. NGS circumvents this issue with its capacity to seek innumerable unknown genetic alterations (Buermans and den Dunnen, 2014).
NGS (e.g, Illumina) sequences by synthesis, in which a 500-base-pair adaptor is ligated and fluorescent dNTPs are added to the strand to determine the sequence of the target strand (Voelkerding et al., 2009; Buermans and den Dunnen, 2014; Fox et al., 2014). Illumina can be used for whole-genome, whole-exome, or targeted generation sequencing. Results are obtained between 29 h and 4 days (Kanzi et al., 2020). A recent study utilized the NGS Memorial Sloan Kettering-Integrated Molecular Profiling of Actionable Cancer Targets (MSK-IMPACT) assay. Using CSF from pediatric patients with varied histologies and a sequencing depth of 2x to 1,368x, researchers screened patients with existing somatic alterations (Miller et al., 2022).
Nanopore sequencing utilizes miniature pores to sequence strands of DNA (Feng et al., 2015). As a strand of DNA passes through the pore, an electrical current is generated that base-calls nucleotides to sequence the strand. The MinION, a handheld nanopore device, is fast and more affordable than NGS with the ability to reuse parts. The nanopore device can provide same-day results and allows users to make genetic calls within the first few minutes of sequencing (Euskirchen et al., 2017).
Proteomic characterization of CNS tumors is possible via liquid chromatography, which separates compounds in a sample based on interactions between mobile and stationary phases. Mass spectrometry is then utilized to convert molecules to the ionized state allowing compound identification by measuring the speed of movement through a vacuum chamber (Pitt, 2009). With only 10 μL of plasma, 862 proteins were identified within 12 h using LC-MS (Xue et al., 2018). Using CSF LC-MS, six proteins were successfully identified that could discriminate the metastatic status of CNS tumors in children compared to controls (Spreafico et al., 2017).
LC-MS can also be used to analyze the metabolites in CSF created by the intake of anti-cancer drugs to assess penetrance beyond the blood-brain barrier (BBB), important for identifying new therapies for clinical trials. A study of seven oral anti-cancer drugs in pediatric CNS tumor patients successfully demonstrated the ability of LC-MS to identify medications with the highest BBB penetrance (Guntner et al., 2020).
The current state of the utility of DNA, RNA, and extracellular vesicles in pediatric liquid biopsy samples is reviewed in this section. Four recent seminal papers in the use of liquid biopsy in pediatric brain tumors are discussed in detail (Liu et al., 2021; Cantor et al., 2022; Miller et al., 2022; Pagès et al., 2022).
Cell-free DNA (cfDNA) are 50–200 base-pair DNA fragments that are released from cells via apoptosis, necrosis, and budding DNA release (Stroun et al., 2001). Circulating tumor DNA (ctDNA) are mutation-carrying fragments released by tumor cells. Both total cfDNA quantification and the mutations identified in ctDNA can be used as biomarkers for tumor diagnosis and progression. Due to its short half-life of 16 min to 2.5 h cfDNA could be a real-time marker of treatment effect and measurable residual disease (MRD) (Mayo et al., 2022).
Due to the high cell turnover rate in malignancies, elevated levels of overall cfDNA are often observed in CSF (Bagley et al., 2020; Nakamura et al., 2020). A study of brainstem gliomas including DIPG demonstrated the ability to reliably detect primary tumor alterations in CSF ctDNA (Pan et al., 2019). Our group demonstrated correlation between the concentration of characteristic mutant K27M copies in CSF ctDNA and contrast-enhancing tumor area on MRI, as well as with cell proliferation in an in vitro model (Stallard et al., 2018). We further applied this technique in a larger cohort as part of a prospective clinical trial, demonstrating that change in H3.3K27M variant allele fraction (VAF) in both CSF and plasma was associated with increased progression-free survival. VAF increase of >25% was found to precede tumor progression in many cases, and cases of radiographic progression without an increase in VAF were later found to reflect pseudo-progression. These data show that CSF or plasma ctDNA H3.3K27M VAF could be a reliable biomarker for prediction of progression and for differentiation from pseudoprogression (Cantor et al., 2022).
When CSF was collected serially in children with medulloblastoma (MB), low-coverage WGS detected MRD in 54% and 85% of patients with localized and metastatic disease, respectively (Liu et al., 2021). Among 64 CSF samples from a diverse range of pediatric and young adult patients, cfDNA positivity correlated strongly with disseminated disease (Miller et al., 2022). While these data are promising, a large-scale attempt to standardize liquid biopsy for DNA biomarker isolation across multiple tumor histologies using ultra-low pass NGS was fairly unsuccessful with copy number alterations only detected in 20% of CSF samples and fewer in other biofluids (Pagès et al., 2022).
microRNAs (miRNAs) are non-coding molecules 18–24 nucleotides in length (Eibl and Schneemann, 2021). They are integral to the stability and translation of messenger RNA (mRNA) and can impact tumor angiogenesis, growth, and invasiveness (Floyd and Purow, 2014). miRNAs have been isolated from CSF, blood, and urine and are stable in biofluids. However, the standardization of control and tumor-specific miRNA panels is in its infancy.
Considering the difficulty in obtaining CSF specimens from children, liquid biopsy of serum and urine miRNA in pediatric brain tumor patients would be preferred, however, there is a relative paucity of information in this subject. Serum miRNA in pediatric astrocytomas has been found to demonstrate characteristic miRNA signatures; miRNA levels were used to successfully predict tumor size and therapeutic response (López-Aguilar et al., 2017; Bookland et al., 2018). Furthermore, reverse transcription of cell-free tumor RNA into complementary DNA has successfully detected EGFR(v)III amplification in pediatric high-grade gliomas (Figueroa et al., 2017; Manda et al., 2018).
In MB (most common cerebellar tumor in pediatric patients) miRNA expression changes have been identified that directly regulate tumorigenic MB pathways including SHH and WNT (Braoudaki and Lambrou, 2015; Wang et al., 2022). These biomarkers could provide MB genetic classification without surgical biopsy.
Extracellular vesicles (EV) are small, membrane-bound structures secreted from both normal and cancerous cells. EVs have been found to hold tumor-specific DNA, various RNAs, and proteins (Doyle and Wang, 2019). Because of their small size and tumor-specific cargo, EVs have been suggested as a biomarker for liquid biopsy in adult gliomas (Nikoobakht et al., 2022).
The diagnostic potential of EVs in pediatric brain tumors is not well studied. Proteomic signatures isolated from pediatric medulloblastoma cell lines and patient sera revealed both a brain tumor-specific profile as well as a MB-specific profile (Epple et al., 2012). Furthermore, spike-in media-derived exosomes were found to be cell attractants, increasing cell migration in vitro. Isolation of EVs from the cell media supernatant of 3 DIPG and 4 MB cell lines identified EV-derived miRNAs unique to DIPG and MB respectively (Magaña et al., 2022). Additionally, the study identified EV-specific miRNAs when compared to miRNAs isolated from parent tumor cells, suggesting targeted loading of miRNAs into EVs.
Further research may unlock important mechanistic discoveries and therapeutic targets. However, the lack of standard protocols for EV isolation and lack of liquid patient samples are barriers to further discovery.
Above we reviewed methods used to study transcriptomic and proteomic signatures of pediatric CNS tumors in biofluids. Next, we will discuss the utilization of liquid biopsy in practice, including applications in diagnosis and surveillance treatment as seen in Figure 1.
FIGURE 1. An overview of biofluid collection, extraction, detection, and diagnosis for improved treatment outcomes.
Genomic profiling of pediatric brain tumors has identified targetable mutations in more than half (Ramkissoon et al., 2017; Cole et al., 2018). In high-risk pediatric glial tumors, exome and transcriptome sequencing prompted a change in therapy in more than two-thirds (Koschmann et al., 2018). This has further been shown to be reproducible using liquid biopsy, with the ability to identify targetable mutations, monitor therapeutic response, and follow tumor evolution (Miller et al., 2022). Molecular profiling is now recommended along with IHC to guide care in pediatric glioma patients (Miklja et al., 2019). Spatial subclones with unique genotypes and phenotypes have been identified in pediatric GBM and DIPG (Vinci et al., 2018; Harpaz et al., 2022) limiting the accuracy of a single stereotaxic biopsy. Liquid biopsy includes elements secreted from all parts of the tumor, with better representation that can provide a more accurate diagnosis.
Liquid biopsy could also facilitate early minimally invasive tumor identification, avoiding treatment delays where appropriate. Of incidentally discovered pediatric brain tumors, most are monitored with imaging and 10% eventually require treatment (Soleman et al., 2020). In an adult diffuse glioma pilot study, 85% of tumors were successfully diagnosed via CSF ctDNA sequencing with a 7–10 days turnaround (Martínez-Ricarte et al., 2018). However, when specimens were prospectively collected in 564 children, copy number alterations were only detected in 20% of CSF, 1.3% of plasma, and 0% of urine samples (Pagès et al., 2022). In the setting of low ctDNA levels in children and low levels of clonal aberrations, extensive further research in the pediatric population is necessary to develop ctDNA as a biomarker and lumbar puncture as an early diagnostic option.
Several pediatric CNS tumors are difficult to diagnose even using tumor morphology, imaging, immunohistochemistry, sequencing, and FISH. Of these diagnostic enigmas, 71% were successfully diagnosed using DNA methylation profiling, a parameter easily accessible using liquid biopsy nucleic acids (Pages et al., 2021). Liquid biopsy miRNA markers for medulloblastoma have been identified for differentiation from controls with high specificity (Wang et al., 2022). In primary CNS lymphoma, the DNA yield, variant allele fraction, and ability to detect MYD88 (a characteristic mutation) were actually much better in CSF cfDNA than in stereotactic biopsy cellular DNA, the current gold standard for diagnosis (Yamagishi et al., 2021).
Intraoperatively, the MinION nanopore device identifies genetic data within the first few minutes of sequencing using fresh frozen tumor tissue (Euskirchen et al., 2017), which could dictate extent-of-resection decision-making. Though Nanopore has previously been limited by a higher error rate as compared to NGS, our group demonstrated 85% sensitivity and 100% specificity for ctDNA in CSF samples from pediatric high-grade glioma patients (Slatko et al., 2018; Bruzek et al., 2020).
During and following treatment of brain tumors, patients are monitored with serial MRI imaging which often fails to differentiate true progression from pseudo-progression. Serum biomarkers have been used to monitor progression in other cancers (i.e. PSA in prostate cancer, CEA in colorectal cancer, CA125 in ovarian cancer, AFP in hepatocellular carcinoma, and beta-hCG in gestational trophoblastic disease). A correlate in pediatric brain cancers could play an important role in post-treatment monitoring and early treatment of recurrence. Serial liquid biopsy in addition to serial MRI may reveal new genetic targets for precision chemotherapy (Kim et al., 2015; Salloum et al., 2017).
Our group demonstrated that change in CSF cfDNA H3K27M variant allele fraction predicted treatment response and tumor progression following ONC201 treatment in diffuse midline glioma (Cantor et al., 2022). The same biomarker was also found to be correlated with treatment response to radiotherapy by another group (Panditharatna et al., 2018). In medulloblastoma, CSF cfDNA was used as a means to detect MRD, demonstrating that patients with persistent copy number alteration detection were at higher risk of progression. Furthermore, marker detection preceded radiographic progression in half of relapsing patients (Liu et al., 2021). DNA methylation status in CSF ctDNA has also been identified as an epigenetic prognostic marker to predict outcomes in MB (Li J et al., 2020).
Brain biopsy, the gold standard of pediatric brain tumor diagnosis, is a risky procedure with unreliable diagnostic value. Liquid biopsy could be a minimally invasive, definitive method to diagnose, monitor, and treat these lethal cancers. CSF procurement via lumbar puncture in children is complicated. Furthermore, it has been shown that CSF sampling more proximal to an intracranial tumor may have increased sensitivity for CSF ctDNA detection (Stallard et al., 2018). Studies have also found that placement of a fourth ventricular catheter for even greater proximity to a posterior fossa tumor is safe (Sandberg and Kerr, 2016).
LP is the safest, least invasive method to obtain CSF during initial brain lesion workup. However, in patients who undergo surgical resection, an alternative CSF procurement method could involve placement of an Ommaya reservoir and peritumoral or intraventricular catheter. Lateral and third ventricular catheters are routinely placed by neurosurgeons for CSF diversion or intrathecal chemotherapy. Still, reservoir placement for tumor monitoring and CAR-T therapy in pediatric patients has only recently become standard of care (Vitanza et al., 2021).
Given that most pediatric tumors present in the posterior fossa, hydrocephalus is a common issue. More than 60% of children with DIPG require surgical intervention for relief, many of whom require shunt placement. In a review of several studies of shunt catheters in DIPG, the overall infection rate was <1% (Li D et al., 2020). In children requiring ventricular Ommaya reservoir for CSF chemotherapy delivery, only 1% developed an infection requiring explantation (Peyrl et al., 2014). Other rare complications include hemorrhage during placement, clogging, and superficial local CSF effusion. Given safety of placement, the potential benefits for longitudinal disease monitoring and treatment guidance far outweigh the benefits.
Blood sampling is innocuous when compared with CSF extraction. The sensitivities of sequencing technologies used to study biofluids, specifically low-coverage WGS and targeted Nanopore NGS, are increasingly improving. As the ability to detect nucleic acid and proteomic biomarkers from liquid biopsies improve, the emphasis will transition from CSF to serum studies. We have already begun to demonstrate that plasma can be used to direct clinical decision-making without awaiting identifiable radiographic progression (Cantor et al., 2022).
Further research is required in the characterization and standardization of biomarkers found in CSF and other biofluids, which could represent the next paradigm shift in clinical management of pediatric brain tumor patients.
AT and VJ designed the original paper and contributed equally to this manuscript. JW, SK, and SS were responsible for initial manuscript generation and editing. AT and VJ were responsible for final manuscript preparation. CK provided funding and editing. All authors contributed to the article and approved the submitted version.
CK is supported by NIH/NINDS Grant R01-NS124607 and R01-NS119231 and Department of Defense Grant CA201129P1, the University of Michigan Chad Carr Pediatric Brain Tumor Center, the ChadTough Defeat DIPG Foundation, Catching Up With JW, The Pediatric Brain Tumor Foundation, The Yuvaan Tiwari Memorial Foundation, The Morgan Behen Golf Classic, and the Michael Miller Memorial Foundation. JW is supported by the National Institutes of Health under award number T32HL749, the University of Michigan Chad Carr Pediatric Brain Tumor Center, Catching Up With JW, and the Pediatric Brain Tumor Foundation.
We would like to thank the editors and reviewers for their work. Figure 1 was created using BioRender.com.
The authors declare that the research was conducted in the absence of any commercial or financial relationships that could be construed as a potential conflict of interest.
All claims expressed in this article are solely those of the authors and do not necessarily represent those of their affiliated organizations, or those of the publisher, the editors and the reviewers. Any product that may be evaluated in this article, or claim that may be made by its manufacturer, is not guaranteed or endorsed by the publisher.
Bagley, S. J., Nabavizadeh, S. A., Mays, J. J., Till, J. E., Ware, J. B., Levy, S., et al. (2020). Clinical utility of plasma cell-free DNA in adult patients with newly diagnosed glioblastoma: A pilot prospective study. Clin. Cancer Res. 26, 397–407. doi:10.1158/1078-0432.CCR-19-2533
Bookland, M., Tang-Schomer, M., Gillan, E., and Kolmakova, A. (2018). Circulating serum oncologic miRNA in pediatric juvenile pilocytic astrocytoma patients predicts mural nodule volume. Acta Neurochir. (Wien) 160, 1571–1581. doi:10.1007/s00701-018-3589-6
Braoudaki, M., and Lambrou, G. I. (2015). MicroRNAs in pediatric central nervous system embryonal neoplasms: The known unknown. J. Hematol. Oncol.J Hematol. Oncol. 8, 6. doi:10.1186/s13045-014-0101-5
Bruzek, A. K., Ravi, K., Muruganand, A., Wadden, J., Babila, C. M., Cantor, E., et al. (2020). Electronic DNA analysis of CSF cell-free tumor DNA to quantify multi-gene molecular response in pediatric high-grade glioma. Clin. Cancer Res. 26, 6266–6276. doi:10.1158/1078-0432.CCR-20-2066
Buermans, H. P. J., and den Dunnen, J. T. (2014). Next generation sequencing technology: Advances and applications. Biochim. Biophys. Acta BBA - Mol. Basis Dis., genome Funct. 1842, 1932–1941. doi:10.1016/j.bbadis.2014.06.015
Cantor, E., Wierzbicki, K., Tarapore, R. S., Ravi, K., Thomas, C., Cartaxo, R., et al. (2022). Serial H3K27M cell-free tumor DNA (cf-tDNA) tracking predicts ONC201 treatment response and progression in diffuse midline glioma. Neuro-Oncol. 24, 1366–1374. doi:10.1093/neuonc/noac030
Capper, D., Jones, D. T. W., Sill, M., Hovestadt, V., Schrimpf, D., Sturm, D., et al. (2018). DNA methylation-based classification of central nervous system tumours. Nature 555, 469–474. doi:10.1038/nature26000
Cole, B. L., Lockwood, C. M., Stasi, S., Stevens, J., Lee, A., Ojemann, J. G., et al. (2018). Year 1 in the molecular era of pediatric brain tumor diagnosis: Application of universal clinical targeted sequencing in an unselected cohort of children. JCO Precis. Oncol. 2, 1–13. doi:10.1200/PO.17.00151
Doyle, L. M., and Wang, M. Z. (2019). Overview of extracellular vesicles, their origin, composition, purpose, and methods for exosome isolation and analysis. Cells 8, 727. doi:10.3390/cells8070727
Eibl, R. H., and Schneemann, M. (2021). Liquid biopsy and primary brain tumors. Cancers 13, 5429. doi:10.3390/cancers13215429
Epple, L. M., Griffiths, S. G., Dechkovskaia, A. M., Dusto, N. L., White, J., Ouellette, R. J., et al. (2012). Medulloblastoma exosome proteomics yield functional roles for extracellular vesicles. PLoS ONE 7, e42064. doi:10.1371/journal.pone.0042064
Euskirchen, P., Bielle, F., Labreche, K., Kloosterman, W. P., Rosenberg, S., Daniau, M., et al. (2017). Same-day genomic and epigenomic diagnosis of brain tumors using real-time nanopore sequencing. Acta Neuropathol. (Berl.) 134, 691–703. doi:10.1007/s00401-017-1743-5
Feng, Y., Zhang, Y., Ying, C., Wang, D., and Du, C. (2015). Nanopore-based fourth-generation DNA sequencing technology. Genomics Proteomics Bioinforma. 13, 4–16. doi:10.1016/j.gpb.2015.01.009
Figueroa, J. M., Skog, J., Akers, J., Li, H., Komotar, R., Jensen, R., et al. (2017). Detection of wild-type EGFR amplification and EGFRvIII mutation in CSF-derived extracellular vesicles of glioblastoma patients. Neuro-Oncol. 19, 1494–1502. doi:10.1093/neuonc/nox085
Floyd, D., and Purow, B. (2014). Micro-masters of glioblastoma biology and therapy: Increasingly recognized roles for microRNAs. Neuro-Oncol 16, 622–627. doi:10.1093/neuonc/nou049
Fox, E. J., Reid-Bayliss, K. S., Emond, M. J., and Loeb, L. A. (2014). Accuracy of next generation sequencing platforms. Gener. Seq. Appl. 1, 1000106. doi:10.4172/jngsa.1000106
Guntner, A. S., Peyrl, A., Mayr, L., Englinger, B., Berger, W., Slavc, I., et al. (2020). Cerebrospinal fluid penetration of targeted therapeutics in pediatric brain tumor patients. Acta Neuropathol. Commun. 8, 78. doi:10.1186/s40478-020-00953-2
Hamisch, C., Kickingereder, P., Fischer, M., Simon, T., and Ruge, M. I. (2017). Update on the diagnostic value and safety of stereotactic biopsy for pediatric brainstem tumors: A systematic review and meta-analysis of 735 cases. J. Neurosurg. Pediatr. 20, 261–268. doi:10.3171/2017.2.PEDS1665
Harpaz, N., Mittelman, T., Beresh, O., Griess, O., Furth, N., Salame, T.-M., et al. (2022). Single-cell epigenetic analysis reveals principles of chromatin states in H3.3-K27M gliomas. Mol. Cell 82, 2696–2713.e9. e9. doi:10.1016/j.molcel.2022.05.023
He, L., He, D., Qi, Y., Zhou, J., Yuan, C., Chang, H., et al. (2021). Stereotactic biopsy for brainstem lesions: A meta-analysis with noncomparative binary data. Cancer Control J. Moffitt Cancer Cent. 28, 10732748211059858. doi:10.1177/10732748211059858
Hindson, B. J., Ness, K. D., Masquelier, D. A., Belgrader, P., Heredia, N. J., Makarewicz, A. J., et al. (2011). High-throughput droplet digital PCR system for absolute quantitation of DNA copy number. Anal. Chem. 83, 8604–8610. doi:10.1021/ac202028g
Izquierdo, E., Proszek, P., Pericoli, G., Temelso, S., Clarke, M., Carvalho, D. M., et al. (2021). Droplet digital PCR-based detection of circulating tumor DNA from pediatric high grade and diffuse midline glioma patients. Neuro-Oncol. Adv. 3, vdab013. vdab013. doi:10.1093/noajnl/vdab013
Jackson, R. J., Fuller, G. N., Abi-Said, D., Lang, F. F., Gokaslan, Z. L., Shi, W. M., et al. (2001). Limitations of stereotactic biopsy in the initial management of gliomas. Neuro-Oncol 3, 193–200. doi:10.1093/neuonc/3.3.193
Kanzi, A. M., San, J. E., Chimukangara, B., Wilkinson, E., Fish, M., Ramsuran, V., et al. (2020). Next generation sequencing and bioinformatics analysis of family genetic inheritance. Front. Genet. 11, 544162. doi:10.3389/fgene.2020.544162
Kim, H., Zheng, S., Amini, S. S., Virk, S. M., Mikkelsen, T., Brat, D. J., et al. (2015). Whole-genome and multisector exome sequencing of primary and post-treatment glioblastoma reveals patterns of tumor evolution. Genome Res. 25, 316–327. doi:10.1101/gr.180612.114
Koschmann, C., Wu, Y.-M., Kumar-Sinha, C., Lonigro, R., Vats, P., Kasaian, K., et al. (2018). Clinically integrated sequencing alters therapy in children and young adults with high-risk glial brain tumors. JCO Precis. Oncol. 2, 1–34. doi:10.1200/po.17.00133
Kurokawa, R., Kurokawa, M., Baba, A., Ota, Y., Pinarbasi, E., Camelo-Piragua, S., et al. (2022). Major changes in 2021 world Health organization classification of central nervous system tumors. Radiogr. Rev. Publ. Radiol. Soc. N. Am. Inc. 42, 1474–1493. doi:10.1148/rg.210236
Li, D., Romanski, K., Kilgallon, M., Speck, S., Bowman, R., DiPatri, A., et al. (2021). Safety of ventricular reservoir sampling in pediatric posthemorrhagic hydrocephalus patients: Institutional experience and review of the literature. J. Neurosci. Nurs. J. Am. Assoc. Neurosci. Nurses 53, 11–17. doi:10.1097/JNN.0000000000000566
Li, D., Stellpflug, W., Romanski, K., Kilgallon, M., Speck, S., and Saratsis, A. M. (2020). Ventricular cerebrospinal fluid sampling in pediatric diffuse midline glioma patients: Institutional experience and review of the literature. Front. Pediatr. 8, 556802. doi:10.3389/fped.2020.556802
Li, J., Zhao, S., Lee, M., Yin, Y., Li, J., Zhou, Y., et al. (2020). Reliable tumor detection by whole-genome methylation sequencing of cell-free DNA in cerebrospinal fluid of pediatric medulloblastoma. Sci. Adv. 6, eabb5427. doi:10.1126/sciadv.abb5427
Liu, A. P. Y., Smith, K. S., Kumar, R., Paul, L., Bihannic, L., Lin, T., et al. (2021). Serial assessment of measurable residual disease in medulloblastoma liquid biopsies. Cancer Cell 39, 1519–1530.e4. e4. doi:10.1016/j.ccell.2021.09.012
López-Aguilar, J. E., Velázquez-Flores, M. A., Simón-Martínez, L. A., Ávila-Miranda, R., Rodríguez-Florido, M. A., and Ruiz-Esparza Garrido, R. (2017). Circulating microRNAs as biomarkers for pediatric astrocytomas. Arch. Med. Res. 48, 323–332. doi:10.1016/j.arcmed.2017.07.002
Louis, D. N., Perry, A., Reifenberger, G., von Deimling, A., Figarella-Branger, D., Cavenee, W. K., et al. (2016). The 2016 world Health organization classification of tumors of the central nervous system: A summary. Acta Neuropathol. (Berl.) 131, 803–820. doi:10.1007/s00401-016-1545-1
Magaña, S. M., Peterson, T. E., Evans, J. E., Decker, P. A., Simon, V., Eckel-Passow, J. E., et al. (2022). Pediatric brain tumor cell lines exhibit miRNA-depleted, Y RNA-enriched extracellular vesicles. J. Neurooncol. 156, 269–279. doi:10.1007/s11060-021-03914-4
Manda, S. V., Kataria, Y., Tatireddy, B. R., Ramakrishnan, B., Ratnam, B. G., Lath, R., et al. (2018). Exosomes as a biomarker platform for detecting epidermal growth factor receptor-positive high-grade gliomas. J. Neurosurg. 128, 1091–1101. doi:10.3171/2016.11.JNS161187
Martínez-Ricarte, F., Mayor, R., Martínez-Sáez, E., Rubio-Pérez, C., Pineda, E., Cordero, E., et al. (2018). Molecular diagnosis of diffuse gliomas through sequencing of cell-free circulating tumor DNA from cerebrospinal fluid. Clin. Cancer Res. 24, 2812–2819. doi:10.1158/1078-0432.CCR-17-3800
Mayo, S., Gómez-Manjón, I., Fernández-Martínez, F. J., Camacho, A., Martínez, F., and Benito-León, J. (2022). CfDNA measurement as a diagnostic tool for the detection of brain somatic mutations in refractory epilepsy. Int. J. Mol. Sci. 23, 4879. doi:10.3390/ijms23094879
Miklja, Z., Pasternak, A., Stallard, S., Nicolaides, T., Kline-Nunnally, C., Cole, B., et al. (2019). Molecular profiling and targeted therapy in pediatric gliomas: Review and consensus recommendations. Neuro-Oncol. 21, 968–980. doi:10.1093/neuonc/noz022
Miller, A. M., Szalontay, L., Bouvier, N., Hill, K., Ahmad, H., Rafailov, J., et al. (2022). Next-generation sequencing of cerebrospinal fluid for clinical molecular diagnostics in pediatric, adolescent and young adult brain tumor patients. Neuro-Oncol. 24, 1763–1772. doi:10.1093/neuonc/noac035
Mueller, S., Jain, P., Liang, W. S., Kilburn, L., Kline, C., Gupta, N., et al. (2019). A pilot precision medicine trial for children with diffuse intrinsic pontine glioma—pnoc003: A report from the pacific pediatric neuro-oncology consortium. Int. J. Cancer 145, 1889–1901. doi:10.1002/ijc.32258
Nakamura, A., Abe, M., Saeki, Y., Kono, F., Ono, Y., and Abe, H. (2020). Circulating tumor cells (CTC) and Cell-free DNA (cfDNA): Liquid biopsy for cancer diagnostics. San Francisco: Academia.
Ng, W. H., and Lim, T. (2008). Targeting regions with highest lipid content on MR spectroscopy may improve diagnostic yield in stereotactic biopsy. J. Clin. Neurosci. 15, 502–506. doi:10.1016/j.jocn.2007.04.005
Nikoobakht, M., Shamshiripour, P., Shahin, M., Bouzari, B., Razavi-Hashemi, M., Ahmadvand, D., et al. (2022). A systematic update to circulating extracellular vesicles proteome; transcriptome and small RNA-ome as glioma diagnostic, prognostic and treatment-response biomarkers. Cancer Treat. Res. Commun. 30, 100490. doi:10.1016/j.ctarc.2021.100490
Nishihara, M., Sasayama, T., Kudo, H., and Kohmura, E. (2011). Morbidity of stereotactic biopsy for intracranial lesions. Kobe J. Med. Sci. 56, E148–E153.
Ostrom, Q. T., de Blank, P. M., Kruchko, C., Petersen, C. M., Liao, P., Finlay, J. L., et al. (2015). Alex’s lemonade stand foundation infant and childhood primary brain and central nervous system tumors diagnosed in the United States in 2007–2011. Neuro-Oncol 16, x1–x36. doi:10.1093/neuonc/nou327
Ostrom, Q. T., Patil, N., Cioffi, G., Waite, K., Kruchko, C., and Barnholtz-Sloan, J. S. (2020). CBTRUS statistical report: Primary brain and other central nervous system tumors diagnosed in the United States in 2013-2017. Neuro-Oncol 22, iv1–iv96. iv1–iv96. doi:10.1093/neuonc/noaa200
Pagès, M., Rotem, D., Gydush, G., Reed, S., Rhoades, J., Ha, G., et al. (2022). Liquid biopsy detection of genomic alterations in pediatric brain tumors from cell-free DNA in peripheral blood, CSF, and urine. Neuro-Oncol. 24, 1352–1363. doi:10.1093/neuonc/noab299
Pages, M., Uro-Coste, E., Colin, C., Meyronet, D., Gauchotte, G., Maurage, C.-A., et al. (2021). The implementation of DNA methylation profiling into a multistep diagnostic process in pediatric neuropathology: A 2-year real-world experience by the French neuropathology network. Cancers 13, 1377. doi:10.3390/cancers13061377
Pan, C., Diplas, B. H., Chen, X., Wu, Y., Xiao, X., Jiang, L., et al. (2019). Molecular profiling of tumors of the brainstem by sequencing of CSF-derived circulating tumor DNA. Acta Neuropathol. (Berl.) 137, 297–306. doi:10.1007/s00401-018-1936-6
Panditharatna, E., Kilburn, L. B., Aboian, M. S., Kambhampati, M., Gordish-Dressman, H., Magge, S. N., et al. (2018). Clinically relevant and minimally invasive tumor surveillance of pediatric diffuse midline gliomas using patient-derived liquid biopsy. Clin. Cancer Res. Off. J. Am. Assoc. Cancer Res. 24, 5850–5859. doi:10.1158/1078-0432.CCR-18-1345
Peyrl, A., Chocholous, M., Azizi, A. A., Czech, T., Dorfer, C., Mitteregger, D., et al. (2014). Safety of Ommaya reservoirs in children with brain tumors: A 20-year experience with 5472 intraventricular drug administrations in 98 patients. J. Neurooncol. 120, 139–145. doi:10.1007/s11060-014-1531-1
Pitt, J. J. (2009). Principles and applications of liquid chromatography-mass spectrometry in clinical biochemistry. Clin. Biochem. Rev. 30, 19–34.
Ramkissoon, S. H., Bandopadhayay, P., Hwang, J., Ramkissoon, L. A., Greenwald, N. F., Schumacher, S. E., et al. (2017). Clinical targeted exome-based sequencing in combination with genome-wide copy number profiling: Precision medicine analysis of 203 pediatric brain tumors. Neuro-Oncol. 19, 986–996. doi:10.1093/neuonc/now294
Salloum, R., McConechy, M. K., Mikael, L. G., Fuller, C., Drissi, R., DeWire, M., et al. (2017). Characterizing temporal genomic heterogeneity in pediatric high-grade gliomas. Acta Neuropathol. Commun. 5, 78. doi:10.1186/s40478-017-0479-8
Sandberg, D. I., and Kerr, M. L. (2016). Ventricular access device placement in the fourth ventricle to treat malignant fourth ventricle brain tumors: Technical note. Childs Nerv. Syst. ChNS Off. J. Int. Soc. Pediatr. Neurosurg. 32, 703–707. doi:10.1007/s00381-015-2969-y
Slatko, B. E., Gardner, A. F., and Ausubel, F. M. (2018). Overview of next generation sequencing technologies. Curr. Protoc. Mol. Biol. 122, e59. doi:10.1002/cpmb.59
Sobol-Milejska, G., Mizia-Malarz, A., Musiol, K., Chudek, J., Bożentowicz-Wikarek, M., Wos, H., et al. (2017). Serum levels of vascular endothelial growth factor and basic fibroblast growth factor in children with brain tumors. Adv. Clin. Exp. Med. 26, 571–575. doi:10.17219/acem/62320
Soleman, J., Kozyrev, D. A., Ben-Sira, L., Constantini, S., and Roth, J. (2020). Management of incidental brain tumors in children: A systematic review. Childs Nerv. Syst. ChNS Off. J. Int. Soc. Pediatr. Neurosurg. 36, 1607–1619. doi:10.1007/s00381-020-04658-8
Spreafico, F., Bongarzone, I., Pizzamiglio, S., Magni, R., Taverna, E., De Bortoli, M., et al. (2017). Proteomic analysis of cerebrospinal fluid from children with central nervous system tumors identifies candidate proteins relating to tumor metastatic spread. Oncotarget 8, 46177–46190. doi:10.18632/oncotarget.17579
Stallard, S., Savelieff, M. G., Wierzbicki, K., Mullan, B., Miklja, Z., Bruzek, A., et al. (2018). CSF H3F3A K27M circulating tumor DNA copy number quantifies tumor growth and in vitro treatment response. Acta Neuropathol. Commun. 6, 80. doi:10.1186/s40478-018-0580-7
Stroun, M., Lyautey, J., Lederrey, C., Olson-Sand, A., and Anker, P. (2001). About the possible origin and mechanism of circulating DNA apoptosis and active DNA release. Clin. Chim. Acta Int. J. Clin. Chem. 313, 139–142. doi:10.1016/s0009-8981(01)00665-9
Thust, S. C., van den Bent, M. J., and Smits, M. (2018). Pseudoprogression of brain tumors. J. Magn. Reson. Imaging 48, 571–589. doi:10.1002/jmri.26171
Vinci, M., Burford, A., Molinari, V., Kessler, K., Popov, S., Clarke, M., et al. (2018). Functional diversity and cooperativity between subclonal populations of pediatric glioblastoma and diffuse intrinsic pontine glioma cells. Nat. Med. 24, 1204–1215. doi:10.1038/s41591-018-0086-7
Vitanza, N. A., Johnson, A. J., Wilson, A. L., Brown, C., Yokoyama, J. K., Künkele, A., et al. (2021). Locoregional infusion of HER2-specific CAR T cells in children and young adults with recurrent or refractory CNS tumors: An interim analysis. Nat. Med. 27, 1544–1552. doi:10.1038/s41591-021-01404-8
Voelkerding, K. V., Dames, S. A., and Durtschi, J. D. (2009). Next-generation sequencing: From basic research to diagnostics. Clin. Chem. 55, 641–658. doi:10.1373/clinchem.2008.112789
Wadden, J., Ravi, K., John, V., Babila, C. M., and Koschmann, C. (2022). Cell-free tumor DNA (cf-tDNA) liquid biopsy: Current methods and use in brain tumor immunotherapy. Front. Immunol. 13, 882452. doi:10.3389/fimmu.2022.882452
Wang, C., Liu, Y., Chen, R., Wang, X., Wang, Y., Wei, J., et al. (2022). Electrochemical biosensing of circulating microRNA-21 in cerebrospinal fluid of medulloblastoma patients through target-induced redox signal amplification. Mikrochim. Acta 189, 105. doi:10.1007/s00604-022-05210-y
Xue, L., Lin, L., Zhou, W., Chen, W., Tang, J., Sun, X., et al. (2018). Mixed-mode ion exchange-based integrated proteomics technology for fast and deep plasma proteome profiling. J. Chromatogr. A 1564, 76–84. doi:10.1016/j.chroma.2018.06.020
Keywords: liquid biopsy, pediatric, brain tumor, cell-free tumor DNA (cf-tDNA), cerebrospinal fluid (CSF), plasma, targeted treatment
Citation: Tripathy A, John V, Wadden J, Kong S, Sharba S and Koschmann C (2023) Liquid biopsy in pediatric brain tumors. Front. Genet. 13:1114762. doi: 10.3389/fgene.2022.1114762
Received: 02 December 2022; Accepted: 23 December 2022;
Published: 06 January 2023.
Edited by:
Xinzhong Li, Teesside University, United KingdomReviewed by:
David Meredith, Brigham and Women’s Hospital and Harvard Medical School, United StatesCopyright © 2023 Tripathy, John, Wadden, Kong, Sharba and Koschmann. This is an open-access article distributed under the terms of the Creative Commons Attribution License (CC BY). The use, distribution or reproduction in other forums is permitted, provided the original author(s) and the copyright owner(s) are credited and that the original publication in this journal is cited, in accordance with accepted academic practice. No use, distribution or reproduction is permitted which does not comply with these terms.
*Correspondence: Carl Koschmann, Y2tvc2NobWFAbWVkLnVtaWNoLmVkdQ==
†These authors have contributed equally to this work
Disclaimer: All claims expressed in this article are solely those of the authors and do not necessarily represent those of their affiliated organizations, or those of the publisher, the editors and the reviewers. Any product that may be evaluated in this article or claim that may be made by its manufacturer is not guaranteed or endorsed by the publisher.
Research integrity at Frontiers
Learn more about the work of our research integrity team to safeguard the quality of each article we publish.