- 1Evandro Chagas Institute (IEC), Ananindeua, Brazil
- 2Institute of Health Sciences (ICS), Federal University of Pará (UFPA), Belém, Brazil
- 3Department of Biomedicine, University of the Amazon (UNAMA), Ananindeua, Brazil
Toll-like Receptors (TLRs), such as the TLR4, are genes encoding transmembrane receptors of the same name, which induce a pro- or anti-inflammatory response according to their expression as the host’s first line of defense against pathogens, such as infectious ones. Single nucleotide polymorphisms (SNPs) are the most common type of mutation in the human genome and can generate functional modification in genes. The aim of this article is to review in which infectious diseases there is an association of susceptibility or protection by the TLR4 SNP rs4986790. A systematic review and meta-analysis of the literature was conducted in the Science Direct, PUBMED, MEDLINE, and SciELO databases between 2011 and 2021 based on the dominant genotypic model of this SNP for general and subgroup analysis of infectious agent type in random effect. Summary odds ratios (ORs) and corresponding 95% confidence intervals (CIs) were calculated for genotypic comparison. I2 statistics were calculated to assess the presence of heterogeneity between studies and funnel plots were inspected for indication of publication bias. A total of 27 articles were included, all in English. Among the results achieved, the categories of diseases that were most associated with the SNP studied were in decreasing order of number of articles: infections by bacteria (29.63%); caused by viruses (22.23%); urinary tract infection—UTI (7.4%), while 11 studies (40.74%) demonstrated a nonsignificant association. In this meta-analysis, a total of 5599 cases and 5871 controls were finalized. The present meta-analysis suggests that there is no significant association between TLR4-rs4986790 SNP and infections (OR = 1,11; 95% CI: 0,75–1,66; p = 0,59), but in the virus subgroup it was associated with a higher risk (OR = 2,16; 95% CI: 1,09–4,30; p = 0,03). The subgroups of bacteria and parasites did not show statistical significance (OR = 0,86; 95% CI: 0,56–1,30; p = 0,47, and no estimate of effects, respectively). Therefore, it has been shown that a diversity of infectious diseases is related to this polymorphism, either by susceptibility or even severity to them, and the receptor generated is also crucial for the generation of cell signaling pathways and immune response against pathogens.
Introduction
Infectious diseases are the second leading cause of mortality worldwide and the main factor in generating disability-adjusted life years (DALYs), which a DALY corresponds to the loss of 1 year of healthy life (World Health Organization, 2000). They are diseases of great weight for health surveillance due to being associated with poverty and inappropriate living conditions (de Souza et al., 2020). The social, demographic, economic, and health control transformations that occurred between 1950 and the 2000s were extremely important for reducing the magnitude of various infectious diseases in several countries around the world. However, from the end of the 20th century, there was a behavior of reappearance of emerging diseases, such as polio, measles, tetanus, diphtheria, pertussis, tuberculosis, malaria, and acquired immunodeficiency virus (HIV/AIDS) disease and new diseases, for example, coronavirus disease 2019 (COVID-19) that counterbalanced this situation and took global proportions (Waldman et al., 1999).
In a population endemic to a disease, some individuals are diagnosed with active infection, which can generate unfavorable clinical outcomes and even death, while others remain asymptomatic (Janeway and Medzhitov, 2002). This can be explained by the distinct immunological response developed by each individual, which is largely correlated with his genetic background. Immunogenetic studies involving genetic background factors have become of great value in the evaluation of susceptibility or infection protection roles (Johnson et al., 2006; Doetschman, 2009).
Single nucleotide polymorphisms (SNPs) are the most frequent type of mutation in the human genome. They can serve as important biological markers for defining therapeutic, diagnostic, and prophylactic strategies for diseases, including infectious ones (Caetano, 2009). Thus, a study of strategies for prevention and control of the occurrence of diseases in humans through health promotion improves the quality of life of the community and contributes to new public health perspectives (Souza and Grundy, 2004). Epidemiological studies seek, in general, to analyze this occurrence of diseases and investigate control measures (Ishikawa and Gomide, 2019).
Toll-like Receptors (TLRs) are a family of type 1 transmembrane receptors and are evolutionarily characterized and conserved proteins in vertebrates and invertebrates (Medzhitov et al., 1997). In this way, TLR4 functions as a specific cell receptor for viruses, bacterial and fungal components, can act both on the cell surface and endosomes for recognition of the linker and to initiate activation of cytokine production by the innate immune response and conduction of the adaptive immune response (Reis et al., 2016). It is an important mediator in the detection of lipopolysaccharide (LPS) and manuronic acid polymers, both found in Gram-negative bacteria. LPS is a key factor in triggering systemic inflammatory response that can lead to sepsis, organ failure, and septic shock (Jabłońska et al., 2020). TLR4 also recognizes lipoteichoic acid (LTA) from Gram-positive bacteria (Takeuchi et al., 1999), mannans from fungal pathogens (Campos et al., 2004) and mycobacteria such as Mycobacterium tuberculosis (Sánchez et al., 2010). The physico-chemical properties and location of LTA in Gram-positive bacterial cells are similar to those of LPS in Gram-negative bacteria (Takeuchi et al., 1999).
TLR4 can be activated by oxidized phospholipids, which also appear in viral lung infections, such as in the detection of respiratory syncytial virus fusion protein (Kurt-Jones et al., 2000) and severe acute respiratory coronavirus syndrome 2 (SARS-CoV-2) (Aboudounya and Heads, 2021). Therefore, it activates the innate immune response (Khanmohammadi and Rezaei, 2021). Furthermore, it can identify certain molecular patterns associated with damage (DAMPs) generated by dead or lysed cells after host tissue injury or viral infection (Aboudounya and Heads, 2021).
The human TLR4 gene is located on the human chromosome 9q33.1 and has three exons and two introns (Vaure and Liu, 2014). In this gene, SNP rs4986790, also known as 896A/G, is a non-synonymous mutation characterized by substitution within the 3rd exon of the TLR4 gene, from an adenine (A) to a guanin (G) at position 896 (896A>G) which leads to modification of the conserved residue of aspartic acid to a glycine in amino acid 299 of the protein sequence (Asp299Gly) on, in or at the domain of the extracellular structure of TLR4. It is responsible for causing change in the function of the encoded protein, diminished function, i.e. missense (Ohto et al., 2012). It has already been associated with the susceptibility and protection of various infectious and non-infectious diseases, as well as the severity of some of them (Arbour et al., 2000). The frequency of the G allele of this SNP ranges from 0 to 20% among populations (Agnese et al., 2002). Figure 1 demonstrates the location of SNP rs4986790 in the TLR4 gene and protein in a didactic model.
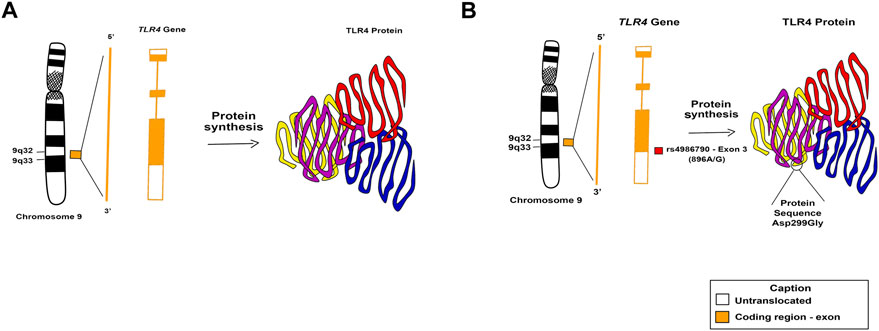
FIGURE 1. Location of the SNP rs4986790 on the TLR4 gene. (A) Normal structure of the TLR4 gene and protein. (B) SNP rs4986790 in the altered gene TLR4 and protein structure.
Due to their importance in generating an immune response in the fight against pathogens, polymorphic variants of TLR4, such as rs4986790, have great value in identifying the risk and defense of infectious diseases. In this sense, the following problem arises: “In which Infectious Diseases is the SNP rs4986790 proven to be related to susceptibility or protection?".
Material and methods
Identification and eligibility of relevant studies
This work consists of a systematic review, which uses the PICOS strategy for the elaboration of the guiding question in order to offer greater engendering of results and resolution of the problem, its anagram being characterized by: population; intervention; comparison; outcome; and study design (Santos et al., 2007). Thus, the following question was asked: “In which Infectious Diseases is the SNP rs4986790 proven to be related to susceptibility or protection?” Following the ensuing questions: Patient: patients with Infectious Diseases/Intervention: evaluate the TLR4 SNP for each population studied and infectious diseases/Comparison: TLR4 SNP and Infectious Diseases/Outcome: identification of which Infectious Diseases are associated with susceptibility or protection through the SNP studied/Study designs: cross-sectional studies, clinical trials, case-control and cohort studies.
The following keywords (MeSH) were selected as search strategy: “TLR4” and “Polymorphisms” and “Infectious Diseases”, together with the Boolean operator “AND”. The research was carried out in the following databases: National Library of Medicine National Institutes of Health of the United States (PubMed), Cochrane Collaboration and Medical Literature Analysis and Retrieval System Online (MEDLINE), Scientific Electronic Library Online (SciELO) and Science Direct.
Available, complete open access of the original categories in Portuguese, English, or Spanish, of the types of cross-sectional studies, clinical trials, case-control, and cohort studies were defined as inclusion criteria between 2011 and 2021. This period was used to condense updates on the subject over the last decade. Exclusion criteria were articles published prior to 2011, articles that were duplicated, available only abstract, letters to the editor, inaccessibility to important information in the article and articles with topics not pertinent to the research question. Thus, the final sample was reached, characterizing all stages, inclusion, and exclusion.
Quality assessment and data extraction
Excel software was used for organization and screening of titles and abstracts and grade pro GDT software for classification of articles in the system. To classify the studies, the Grading of Recommendations Assessment, Development and Evaluation (GRADE system) was used, which is a system created to identify the degree of evidence and the strength of health recommendations (Aguayo-Albasini et al., 2014). For the inclusion of the articles, only those with high or moderate methodological quality were used.
Synthesis of data and risk of bias
For the synthesis of data, the studies were presented according to the following categories: author, year, type of infectious agent, methodology, study population, genotyping method, allele-genotype frequencies in cases and controls, results of the Hardy-Weinberg test (HWE), country, Ethnic Group and results. Degrees of significance (p < 0.05) were considered statistically significant correlations between the SNP and each infectious disease studied. The Preferred Reporting Items for Systematic Reviews and Meta-Analyzes (PRISMA) flowchart, based on the PRISMA protocol, was used to present the steps followed for the present study (Liberati et al., 2009).
The data selection step for the visualization of the search was performed by two investigators (MJAS and DSS) independently, thus ensuring their reliability. Evidence is graded by research design, providing standards that establish the appropriate grade for medical decision making. The types of studies included in this article were composed according to the degree of evidence in decreasing order of clinical trials, cohort, case-control, and cross-sectional studies (Duncan and Schmidt, 1999).
Statistical analysis
The meta-analysis was carried out using Review Manager 5.4.1 (Nordic Cochrane Center, Cochrane Collaboration, Copenhagen, Sweden). Only genotypic comparison was performed employing a dominant genetic model (AG + GG vs AA) was performed due to the rarity of the GG genotype (1% in some populations, such as East Asian) (Kim and Jeong, 2020). Summary odds ratios (ORs) and corresponding 95% confidence intervals (CIs) were determined using a random effect analysis method. A division into subgroups was prepared for evaluation by the types of infectious agents found here for this TLR4 SNP. Heterogeneity between studies for comparisons was assessed using I2 statistics; A score of 25% indicates the absence of substantial heterogeneity, a result of 5% or more shows substantial heterogeneity, and a result of 75% or more indicates considerable heterogeneity. One of the most popular methods to determine the importance of heterogeneity is the chi-square test, with a conventional level of more conservative significance of p < 0.10, instead of the usual p < 0.05.
Results
A total of 172 articles were found for reading from the databases. After excluding 10 duplicate studies, in addition to 20 letters to the editor, 20 studies available only the abstract, removing 94 studies irrelevant to the theme based on title, abstract, and body of the text. The final sample consisted of 27 articles, most of them belonging to the case-control type (Figure 2). These included studies are presented in Table 1. The presence of the mutant allele of the SNP was associated with susceptibility to several infectious diseases: COVID-19 (Taha et al., 2021); Escherichia coli infection (Sampath et al., 2013); Orientia tsutsugamushi infection (Janardhanan et al., 2013); S. pyogenes and H. influenzae (Liadaki et al., 2011); mononucleosis (Jabłońska et al., 2020); UTI in Indian diabetic patients (Gond et al., 2018); dengue infection (Sharma et al., 2016); influenza H1N1 in the Italian population (Esposito et al., 2012a); HBV/HCV (Sghaier et al., 2019); HIV (Vidyant et al., 2019).
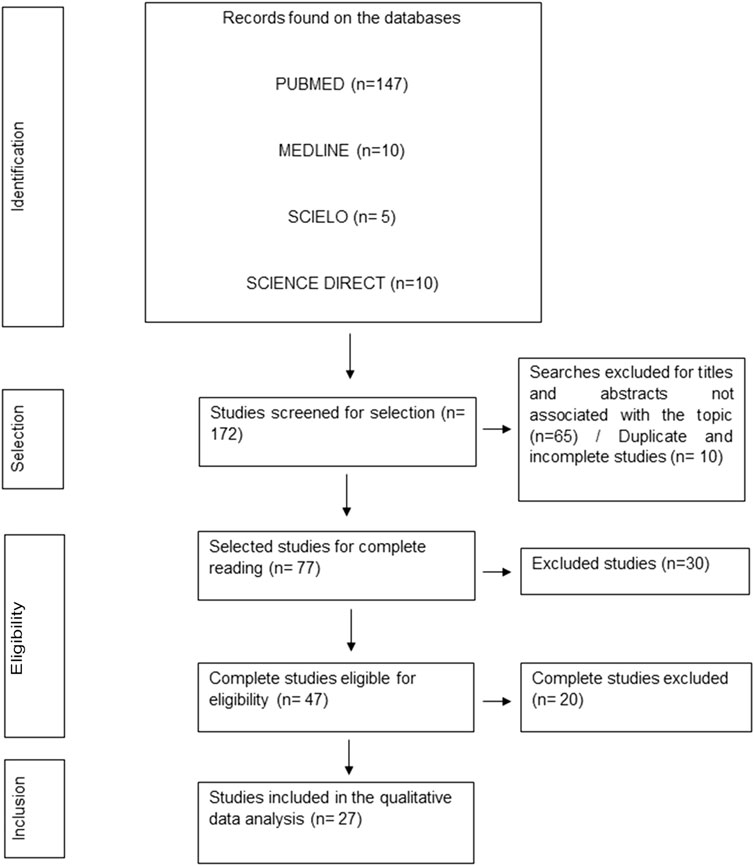
FIGURE 2. Flowchart representative of the stages of selection, eligibility, and inclusion of studies for analysis.
The presence of mutant allele of this SNP was associated with the protection of: Escherichia coli infection (Lee et al., 2011); severe respiratory syncytial virus disease (Kresfelder et al., 2011); urinary tract-UTI infection (Akil et al., 2012); prosthetic joint infection in the Czech population (Mrazek et al., 2013); renal parenchyma infection (Hussein et al., 2018); chronic periodontitis and chronic gengivitis (Garlet et al., 2012); P. gingivalis infection (Sellers et al., 2016) and with the reduction in severity of periodontitis (Sellers et al., 2016).
The forest plot of the meta-analysis was represented by Figure 3. The meta-analysis was performed for random effect due to the high heterogeneity found (I2) in the analysis of both subgroups and in general.
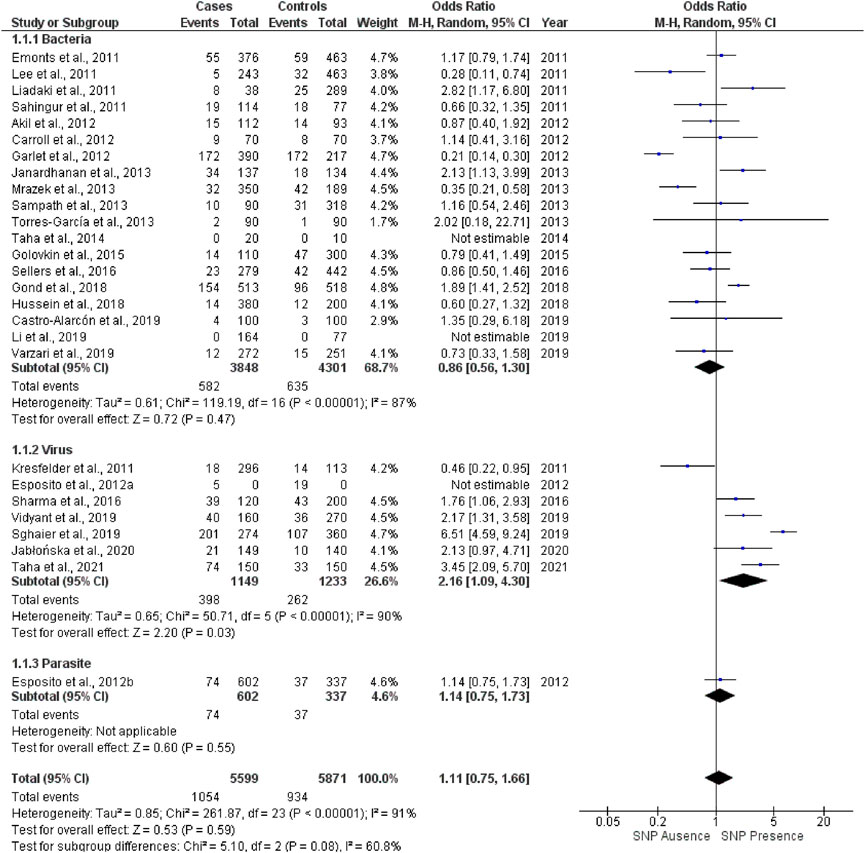
FIGURE 3. Forest plot of the association between the SNP rs4986790 of the TLR4 gene and susceptibility to infectious diseases in a dominant genotypic model.
In a generalized analysis, the SNP TLR4 rs4986790 did not show a significant association with susceptibility to infections (OR = 1,11; 95% CI: 0,75–1,66; p = 0,59). Regarding the subgroups, for bacteria, although this SNP was not statistically significant, it showed a greater strength of association for the protection of infections related to them (OR = 0,86; 95% CI: 0,56–1,30; p = 0,47). It should be taken into account that, in the meta-analysis, the etiological agent of UTI considered was bacteria. Regarding viruses, a significantly positive relationship was found between the presence of this mutant SNP allele and the risk of contracting viral infections (OR = 2,16; 95% CI: 1,09–4,30; p = 0,03). For parasites, only one study was included, and therefore it was not possible to observe significant associations in this subgroup (no effect estimate).
A total of 5599 cases and 5871 controls were included. The numerical configuration of researches was composed of 19 studies (70.37%) for the bacteria subgroup, with 3848 cases and 4301 controls, while six researches (22.22%) were in virus subgroup (constituted of 1149 cases and 1233 controls) and only one for parasites (3.7%), forming a subgroup with 74 patients and 37 controls. A symmetrical funnel plot presented the reduced risk of bias in Figure 4.
Schematic presentation of the data found for TLR4 Signaling Transduction Pathways was performed in Figure 5 and these pathways in the presence of the investigated SNP of TLR4 in Figure 6.
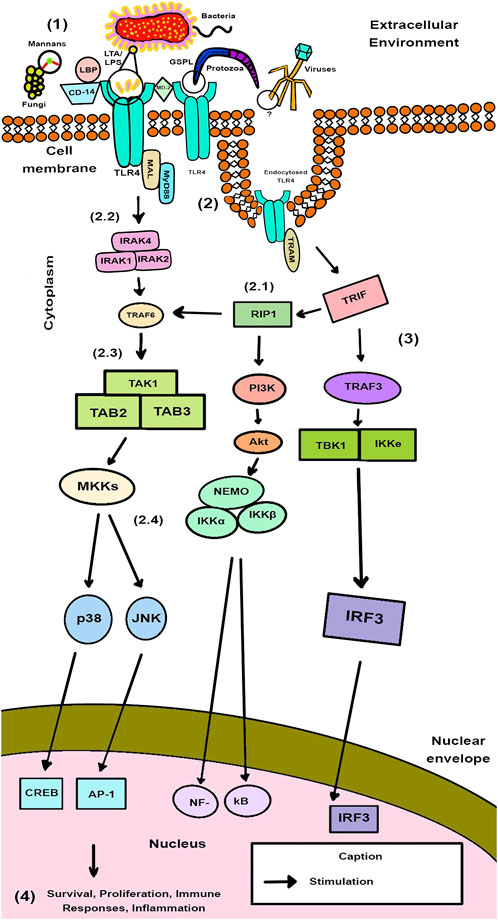
FIGURE 5. Schematic Model of TLR4 Signaling Transduction Pathways in Pathogen Recognition. Black circles from the top section of the figure indicate the PAMPs that are recognized by TLR4 for each type of infectious agent.
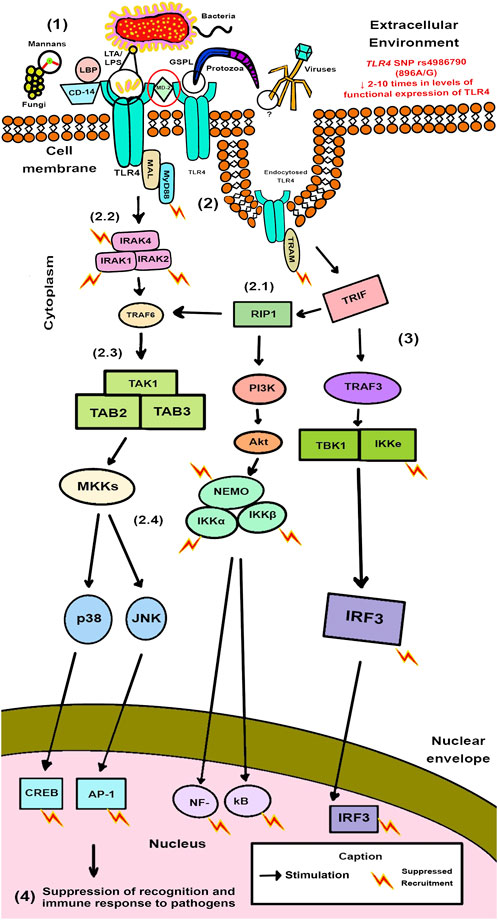
FIGURE 6. Schematic Model of TLR4 Signaling Transduction Pathways in Pathogen Recognition in the SNP rs4986790 presence. Black circles from the top section of the figure indicate the PAMPs that are recognized by TLR4 for each type of infectious agent. The red circle highlights the increase in receptor dysfunction in its immune functions in the absence of MD-2. The red lightning symbols indicate the structural components of the TLR4 signaling pathway in which the mutation was associated with increased infection sensitivity identified in humans.
Figure 5 represented its stages chronologically through numbers in ascending order. It starts at phase (1) Recognition of the microorganism: TLR4 is activated on the cell membrane by PAMPs of pathogens, such as lipoteichoic acid (LTA) from Gram-positive bacteria, LPS from Gram-negative bacteria, elements not fully unraveled for viruses, fungal mannans, and glycosphingolipid (GSPL) from parasites, while CD-14, liposaccharide binding protein (LBP) and MD-2 act as accessory proteins in a conformational complex. From this binding, in phase (2), the left and right routes for TLR4 signaling are shown to be MyD88-dependent and independent, respectively, in which there is the generation of pathways: TLR4 dimerizes and recruits downstream adapter proteins such as MyD88/MAL and TRIF/TRAM to mount an inflammatory response in the cytoplasm. (2.1) RIP1 is recruited to the receptor by binding to TRIF and is then phosphorylated and polyubiquitinated, inducing Akt phosphorylation. (2.2) Concurrently, the MyD88 molecule-dependent activations are facilitated with IRAK4, which contributes to the phosphorylation of IRAK1. This reaction allows the binding of the TRAF6 molecule to the complex. (2.3) Furthermore, binding of TRAF6 activates TAK1, which forms an aggregation with TAB2 and TAB3. After its formation, TAK1 induces the IKK complex through NEMOylation. (2.4) Then, both pathways converge in the generation of NF-kB. In addition to activating NF-κB, TAK1 also phosphorylates MKKs, which subsequently activate JUN N-terminal kinase (JNK) and p38 to further enhance the inflammatory response. In stage (3) the TRIF/TRAM pathway not only activates NF-κB (translocated to the nucleus), but also triggers IRF3 to mount an antiviral response by interferons (IFNs) actions. In step 4) TLR4 levels cause the expression of pro-inflammatory cytokines and the maturation of APCs of the innate immune system that will define the course of the infection. This stimulation of TLR4 is crucial for inducing powerful responses to protect against host damage. Innate immunity develops because of the coordinated effects of activating several TLRs, together with the activation of numerous additional receptors involved in host defense and is linked to the formation of adaptive immunity. Specific defense is made possible by cytokine induction; for example, IRF-3 facilitates antiviral responses via IFN-β, while interleukin (IL) - 12 is crucial for defense against intracellular infections. TLR-mediated up-regulation of inducible NO synthase contributes to macrophages’ ability to destroy phagocytosed bacteria.
Figure 6 represents at phase (1) Recognition of the microorganism: TLR4 is activated on the cell membrane by PAMPs of pathogens, such as lipoteichoic acid (LTA) from Gram-positive bacteria, LPS from Gram-negative bacteria, elements not fully unraveled for viruses, fungal mannans, and glycosphingolipid (GSPL) from parasites, while CD-14, liposaccharide binding protein (LBP) and MD-2 act as accessory proteins in a conformational complex. TLR4 mutation change the structure of TLR4 and limit its ability to interact with LPS, CD-14, and/or MD-2. The TLR4 SNP rs4986790 acts by decreasing the expression of TLR4 by 2 times. In this sense, the absence of myeloid differentiation factor 2 (MD-2), which forms a complex with TLR4 and LPS, this decrease is elevated in 10 times. From this binding, in phase (2), both MyD88-dependent and independent routes are affected by the presence of this mutation and there is the generation of TLR4 signaling pathways: the aforementioned vias (2.1), (2.2), (2.3), and (2.4). This mutation leads to suppressed recruitment of downstream adapter molecules and kinases involved in the TLR4 pathways, such as IRAK4, NEMO, IKKe and IRF3, which can thus eliminate signaling activity. In stage (3), there is suppression of all these vias, including, the NF-κB transactivation and translocation in human monocytes with the 896A/G TLR4 expression, which could lead to the development of adaptive immunity. In step (4), this dysfunctional stimulation of TLR4 is characterized by suppression of recognition and immune response to pathogens, generating an uncoordinated immune response and, consequently, in most cases, a poor prognosis to infectious diseases.
Discussion
The analysis of host genetic factors such as SNPs can become a useful strategy to identify people at increased risk of specific infections, patients at higher risk of unfavorable disease outcomes, and contribute to more effective therapeutic interventions (Mukherjee et al., 2019). The pro-inflammatory response induced by TLRs is considered the first form of body defense against pathogens and marks substantial variations in antimicrobial defense of populations.
In this sense, the manifestation of infectious diseases may be related to mutations in the TLR4 gene due to the absence/presence of the mutant allele of SNPs, in addition to genetic, epigenetic, and environmental factors (Medzhitov and Janeway, 2000). SNP rs4986790 has been shown to cause hyporesponsiveness to LPS. This SNP causes local structural changes around the mutation area that can affect the effectiveness of folding, cell surface expression, protein stability, and interaction with downstream messenger proteins (Ohto et al., 2012). At the molecular level, this SNP has been reported to interfere with the interaction of TLR4 with downstream messengers, such as MyD88 and other downstream messengers (Figueroa et al., 2012). Additionally, SNP appears to affect the levels of functional expression of TLR4, causing a decrease of 2 times (Prohinar et al., 2010). This reduction is further amplified to 10 times in the absence of myeloid differentiation factor 2 (MD-2) that forms a complex with TLR4 and LPS (Park et al., 2009). This decline in functionality is further amplified before TLR4 and LPS form a complex (Prohinar et al., 2010).
In the present systematic review, most of the studies were observed to come from populations in the United States (5 studies - 18.51%) and the number of studies associated with susceptibility to infectious diseases was higher for the African continent (with 10 studies in all continents −37.03%). Despite the numerical supply of articles and the number of positive associations relating bacterial infections to the present SNP of TLR4, the strength of association of the meta-analysis did not generate a significant degree of due evidence from the analyzed researches, while for viruses there was a significant association. A total of 16 of the 27 studies (59.25%) did not report the ethnic group belonging to the investigated population, most of them coming from the Asian and African continent (5 researches each, with 31.25%) and, with respect to the country, mostly from the United States (with four researches, 25%). Therefore, there is a great bias in reporting these ethnic terms in these studies, especially from the United States.
In our analysis, the most studied diseases for SNP were those caused by bacterial infections (with 17 studies—62.96%), followed by viral infections, with seven studies, equivalent to 25.93% and urinary tract infection (UTI) with two studies (7.4%). The excess percentage was delimited by studies involving parasitic agents (1 study, 3.7%).
The most commonly associated infectious diseases in different countries for TLR4 SNP and susceptibility were those caused by viruses (6 researches, 85.71% in virus subgroup). The infectious diseases that for this SNP were most associated with protection in different countries had several studies in decreasing order among those caused by bacteria infections (n = 9, 33.33%), viruses (n = 1, 3.7%), and UTI (n = 1, 3.7%).
Contradictory findings were found for susceptibility related to the presence of the mutant allele of this SNP and infectious diseases were found in populations around the world and this may be directly related to environmental or even epigenetic factors, associated with the individual genetic background. Conflicting data were found in different populations for the following diseases: reduced risk of periodontitis in Brazilian population (Garlet et al., 2012), and a protective effect was conferred for periodontitis in a United States population (Sellers et al., 2016), while no association between this SNP and periodontitis was found in populations in China and United States (Sahingur et al., 2011; Li et al., 2019); what concerns gram-negative bacteria infection, otitis media has no association with this SNP in the American population (Carroll et al., 2012), a no significant association was observed for susceptibility to E. coli infection in Mexico population (Castro-Alarcón et al., 2019); a protective effect conferred by this SNP in the United States population (Lee et al., 2011), a higher risk of these infections in Greece, India, and United States populations (Liadaki et al., 2011; Janardhanan et al., 2013; Sampath et al., 2013); regarding to UTI, the study conducted by Akil et al., 2012 associated the presence of the mutant allele of this SNP with reduced risk of this disease in Turkey (Akil et al., 2012), while Gond et al., 2018 revealed a higher risk of this infection in India (Gond et al., 2018).
No significant statistical associations were observed for the diseases: periodontitis (in the American and Chinese population) (Sahingur et al., 2011; Li et al., 2019); malaria in the Burundi population (Esposito et al., 2012b); tuberculosis (in Moldavia and Mexico populations) (Torres-García et al., 2013; Varzari et al., 2019); endocarditis in the Russian population (Golovkin et al., 2015); rheumatoid arthritis (in Egyptian population, Netherlands) (Emonts et al., 2011; Taha et al., 2014); otitis media in the American population (Carroll et al., 2012); E. coli infection in Mexico population (Castro-Alarcón et al., 2019).
SNP rs4986790 in the TLR4 gene in bacterial infections
The main association found in this review in a numerical offer for susceptibility to SNP and infectious diseases is correlated with the main TLR4 ligand (LPS) and reveals the effect of the G allele in various functional studies for Gram-negative bacteria (Jabłońska et al., 2020).
The functional expression of TLR4 activation and its main ligand (LPS) emphasizes its activity in detecting the molecular patterns associated with pathogens (PAMPs) of Gram-negative bacteria (Jabłońska et al., 2020). The activation of TLR4 by LPS is a multistage process. The first step highlights the LPS binding protein (LBP), which extracts LPS from bacterial membranes and vesicles containing LPS and transfers it to the grouping of TLR4 differentiation 14 coreceptors (CD-14). CD-14 exists in two forms, soluble and membrane-bound. Both forms can interact with LPS-loaded LBP. CD-14 disassembles LPS aggregates and transfers monomer LPS to a hydrophobic pouch in myeloid differentiation factor 2 (MD-2), which is part of the MD-2/TLR4 complex. Activation of TLR4 leads to the recruitment of intracellular adaptor proteins, primary myeloid differentiation response 88 (MyD88) and/or toll/interleukin-1 receptor (TIR) containing interferon-beta (TRIF), leading to the expression and secretion of pro-inflammatory mediators (Kuzmich et al., 2017).
SNP rs4986790 in the TLR4 gene in viral infections
Viral infections have in common membrane-associated TLR4 activating ligands, which bind to the TLR4 complex. In this context, this structure is activated by non-LPS DAMPs and PAMPs, which vary widely in their structure—some without structural similarities with LPS, the determination of the role of TLR4 activation remains uncertain for viruses (Olejnik et al., 2018). Further studies can improve the understanding of the association of the role of this TLR4 SNP in its receptor and how this activation can interfere with the recognition of viruses in human organisms, since a strong association was found between viruses and this SNP.
SNP rs4986790 in the TLR4 gene in urinary tract infection
UTI is an infectious disease that can be caused by bacteria, viruses, parasites, and fungi. Microorganisms enter through two pathways, the lower urinary tract or the bloodstream (into the kidneys). TLR4 has been reported to control the immune response to infection of epithelial cells that line the mucosa of the urinary tract and the signaling response of this TLR regulates cell balance and death by releasing some urothelium cytokines (Ruch and Engel, 2017). Finally, the UTIs were also considered in this study a disease of infection caused by Gram-negative bacteria due to their etiological diversity, even though in most studies of the polymorphism in question there was a predominance of infection by these bacteria. TLR4 signaling is activated by binding the P fimbriae to the glycosphingolipid (GSPL) receptor of the cell surface, and ceramide acts as a signaling intermediate to activate TLR4. In a type of human inoculation therapy, p-fimbriae have been shown to promote bacteriuria and mucosal inflammation. In addition, the TLR4 SNP demonstrates importance in the innate immune regulation of UTI. In this scenario, more than 85% of UTIs are caused by bacteria and about 80% of them are caused by Escherichia coli. In addition to being caused by other Gram-negative bacteria and to a lesser extent by Gram-positive bacteria (Ragnarsdóttir et al., 2010).
In a review and meta-analysis by Reza Mortazavi-Tabatabaei et al., 2019, with epidemiological studies involving patterns of antibacterial resistance to UTI, among the most common pathogens that cause UTIs were E. coli (with about 80%), followed by Klebsiella, Staphylococcus, and Streptococcus with a frequency of 62%, 13%, 12%, and 11%, respectively (Reza Mortazavi-Tabatabaei et al., 2019).
Based on a review and meta-analysis by Huang; Xu (2019), in Asian populations, the mutation in rs4986790 may make them more susceptible to urinary tract infections (Huang et al., 2020). This information on the association of the susceptibility to UTI for Asian populations was corroborated in the present review.
In the murine UTI model, TLR4 controls the innate immune response to Escherichia coli and TLR4 mice −/− develop asymptomatic bacteriuria instead of severe infection. Low levels of TLR4 expression have also been observed in children with asymptomatic bacteriuria. In addition to being related to susceptibility and protection agaisnt UTI, the SNP can distinguish phenotypes from symptomatic and asymptomatic patients, it determines the severity of acute infection, as well as the long-term effects on the integrity of the renal tissue. On the other hand, susceptibility to UTI can also be discussed in terms of social and environmental factors or dysfunctional urination (Ragnarsdóttir et al., 2007).
SNP rs4986790 in the TLR4 gene in parasitic infections
As far as parasites are concerned, the highlighted SNP was only analyzed for malaria (in one study), with no significant association. At the molecular level, this receptor can function as an upstream sensor for GSPL and induce the intracellular inflammatory signaling necessary to kill parasites (Aguirre-García et al., 2019).
SNP rs4986790 in the TLR4 gene in fungal infections
With regard to fungi, no study had significant associations for the SNP analyzed. On the other hand, TLR4 has a scientific framework directly related to fungal infections, such as candidiasis and paracoccidioidomycosis, caused, respectively, by the fungi Candida albicans and Paracoccidioides brasiliensis. In this sense, it directly protects the oral mucosa from fungal infection by Candida through a process mediated by polymorphonuclear leukocytes (PMNs) (Qin et al., 2016). What concerns P. brasiliensis, its most virulent strain (Pb 18 strain) can use TLR4 to gain access to neutrophils that pathogenically produce interleukin (IL)—eight and IL-10, increasing the inflammatory process and thus patient morbidity (Acorci-Valério et al., 2010; Freitas et al., 2016).
TLR4 signaling pathways in the immune response to pathogens and final considerations
SNPs can be associated or involved, or they can even determine the differential risks of infection. This is due to the fact that in some infections the immune response leads to a protective inflammatory response, while in others this response may be essential in the pathogenesis of the infectious process (Silva et al., 2021).
At this conjuncture, TLR4 signaling is mediated by four adapting molecules: myeloid differentiation factor 88 (MyD88); adapter-type protein MyD88 (Mal), also known as the TIR domain adaptor protein (TIRAP); TIR-containing ligant-induced interferon-beta (TRIF), also known as TLR-containing ligant molecule 1 (TICAM-1); TRIF-related ligant molecule (TRAM), also known as IRR-containing protein (TIRP) or ligating molecule 2 (TICAM-2). TLR4 requires all four ligands to mediate a comprehensive immune response (Lizundia et al., 2008).
MyD88 propagates through the factor associated with tumor necrosis factor receptor—TNF (TRAF)—6, which induces the production of an inflammation regulator, the nuclear factor - kappa beta (NF-κB). This regulator triggers the genes encoding the immune activators, which include tumor necrosis factor alpha (TNF-α) and interleukins (IL) -1, IL-8, IL-12, and IL-6. However, uncontrolled defense processes of organisms against infectious agents can lead to a number of organic dysfunctions, including coagulation dysfunction, fever, vasodilation, and decreased blood pressure, leading to necrosis of tissues and organs caused by the accumulation of cytokines (Costalonga and Zell, 2007).
As in a review and meta-analysis by Ziakas et al. (2013), this analysis highlights that this SNP of TLR4 was associated with susceptibility to a diverse spectrum of infections and has a complex effect of the TLR4 variant on susceptibility to infectious diseases. However, a greater association with parasitic diseases is recorded in a study by Ziakas et al. (2013), while in this present study it is associated with viral infections. This is due to the fact that the aforementioned study sought to achieve two TLR4 SNPs, with a different time frame, different types of epidemiological studies and only open access studies (Ziakas et al., 2013).
The presence of significant effects may depend on the magnitude of TLR4 functional expression. Protection or risk can be reduced by the levels of functional expression of TLR4, which are regulated by its polymorphisms and the presence of MD-2. Therefore, it is necessary to explore whether MD-2 is important in response to some infections, but not others, or if TLR4 levels differ in one infection compared to another (Prohinar et al., 2010).
The limitations of the study are, in fact, the difficulty of its reproducibility due to the design, sample size, and environmental and genetic heterogeneity of the different populations around the world due to the diversity in genetic background. The environmental heterogeneity of populations is related to the various outcomes of diseases due to individual risk factors for each of them. In addition, different populations generate different immune responses that may affect conclusive information. Although this was true, the papers supported substantial contributions to answer the leading issue and featured current validation and standardization, enabling a crucial and effective qualitative synthesis of the data. Therefore, these elements do not suggest a considerable amount of methodological variability.
Conclusion
The data engendered in this study indicate the importance of the main TLR4 (LPS) linker for SNP rs4986790 and infectious agents, since the most associated and studied infectious diseases were infections by Gram-negative bacteria, mediated by LPS, even with nonsignificant association here. This meta-analysis also indicates a new association between infections and this TLR4 SNP with respect to viruses, with 2 times greater risk in this subgroup in a relationship of increased susceptibility to the presence of the mutant allele in individuals from different countries that needs to be further investigated.
In the same way that there are controversial results on susceptibility to infectious diseases, we report the need for immunogenetic studies that are performed in different populations. Furthermore, the study reveals the characterization of pathophysiology in clinical conditions of diseases associated with this SNP. From this perspective, new omic studies can help close the gaps in understanding the extent to which immunogenetics interferes with the outcome of infectious diseases. Therefore, this study provides an overview for the creation of strategies in the field of medicine with respect to the treatment of infectious diseases.
Data availability statement
The original contributions presented in the study are included in the article/Supplementary Material, further inquiries can be directed to the corresponding author.
Author contributions
MS, DS, and LL designed the study. MS and DS searched databases and performed the selection of studies. MS and DS analysed the data and wrote the manuscript. LD and EM performed the resources and software. LL critically evaluated the review and commented on it. All authors read and approved the final manuscript.
Conflict of interest
The authors declare that the research was conducted in the absence of any commercial or financial relationships that could be construed as a potential conflict of interest.
Publisher’s note
All claims expressed in this article are solely those of the authors and do not necessarily represent those of their affiliated organizations, or those of the publisher, the editors and the reviewers. Any product that may be evaluated in this article, or claim that may be made by its manufacturer, is not guaranteed or endorsed by the publisher.
References
Aboudounya, M. M., and Heads, R. J. (2021). COVID-19 and toll-like receptor 4 (TLR4): SARS-CoV-2 may bind and activate TLR4 to increase ACE2 expression, facilitating entry and causing hyperinflammation. Mediat. Inflamm. 2021, 8874339. doi:10.1155/2021/8874339
Acorci-Valério, M. J., Bordon-Graciani, A. P., Dias-Melicio, L. A., De Assis Golim, M., Nakaira-Takahagi, E., and De Campos Soares, Â. M. V. (2010). Role of TLR2 and TLR4 in human neutrophil functions against Paracoccidioides brasiliensis. Scand. J. Immunol. 71, 99–108. doi:10.1111/j.1365-3083.2009.02351.x
Agnese, D. M., Calvano, J. E., Hahm, S. J., Coyle, S. M., Corbett, S. A., Calvano, S. E., et al. (2002). Human toll-like receptor 4 mutations but not CD14 polymorphisms are associated with an increased risk of gram-negative infections. J. Infect. Dis. 186, 1522–1525. doi:10.1086/344893
Aguayo-Albasini, J. L., Flores-Pastor, B., and Soria-Aledo, V. (2014). GRADE system: Classification of quality of evidence and strength of recommendation. Cir. Esp. 92, 82–88. doi:10.1016/j.ciresp.2013.08.002
Aguirre-García, M. M., Rojas-Bernabé, A., Gómez-García, A. P., and Escalona-Montaño, A. R. (2019). TLR-mediated host immune response to parasitic infectious diseases. IntechOpen. doi:10.5772/intechopen.84679
Akil, I., Ozkinay, F., Onay, H., Canda, E., Gumuser, F., and Kavukçu, S. (2012). Assessment of Toll-like receptor-4 gene polymorphism on pyelonephritis and renal scar. Int. J. Immunogenet. 39, 303–307. doi:10.1111/j.1744-313X.2012.01090.x
Arbour, N. C., Lorenz, E., Schutte, B. C., Zabner, J., Kline, J. N., Jones, M., et al. (2000). TLR4 mutations are associated with endotoxin hyporesponsiveness in humans. Nat. Genet. 25, 187–191. doi:10.1038/76048
Caetano, A. R. (2009). Marcadores SNP: Conceitos básicos, aplicações no manejo e no melhoramento animal e perspectivas para o futuro. R. Bras. Zootec. 38, 64–71. doi:10.1590/S1516-35982009001300008
Campos, M. A., Rosinha, G. M. S., Almeida, I. C., Salgueiro, X. S., Jarvis, B. W., Splitter, G. A., et al. (2004). Role of Toll-like receptor 4 in induction of cell-mediated immunity and resistance to Brucella abortus infection in mice. Infect. Immun. 72, 176–186. doi:10.1128/IAI.72.1.176-186.2004
Carroll, S. R., Zald, P. B., Soler, Z. M., Milczuk, H. A., Trune, D. R., and MacArthur, C. J. (2012). Innate immunity gene single nucleotide polymorphisms and otitis media. Int. J. Pediatr. Otorhinolaryngol. 76, 976–979. doi:10.1016/j.ijporl.2012.03.011
Castro-Alarcón, N., Rodríguez-García, R., Ruíz-Rosas, M., Muñoz-Valle, J. F., Guzmán-Guzmán, I. P., Parra-Rojas, I., et al. (2019). Association between TLR4 polymorphisms (896 A>G, 1196 C>T, - 2570 A>G, - 2081 G>A) and virulence factors in uropathogenic Escherichia coli. Clin. Exp. Med. 19, 105–113. doi:10.1007/s10238-018-0527-0
Costalonga, M., and Zell, T. (2007). Lipopolysaccharide enhances in vivo interleukin-2 production and proliferation by naive antigen-specific CD4 T cells via a Toll-like receptor 4-dependent mechanism. Immunology 122, 124–130. doi:10.1111/j.1365-2567.2007.02620.x
de Souza, H. P., de Oliveira, W. T. G. H., dos Santos, J. P. C., Toledo, J. P., Ferreira, I. P. S., de Sousa Esashika, S. N. G., et al. (2020). Infectious and parasitic diseases in Brazil, 2010 to 2017: Considerations for surveillanceEnfermedades infecciosas y parasitarias en brasil de 2010 a 2017: Aspectos para la vigilancia sanitaria. Rev. Panam. Salud Publica 44, e10. doi:10.26633/rpsp.2020.10
Doetschman, T. (2009). Influence of genetic background on genetically engineered mouse phenotypes. Methods Mol. Biol. 530, 423–433. doi:10.1007/978-1-59745-471-1_23
Duncan, B. B., and Schmidt, M. I. (1999). Evidence-based medicine. Rev. Assoc. Med. Bras. 45, 247–254. doi:10.1590/S0104-42301999000300010
Emonts, M., Hazes, M. J. M. W., Houwing-Duistermaat, J. J., van der Gaast-de Jongh, C. E., de Vogel, L., Han, H. K. H., et al. (2011). Polymorphisms in genes controlling inflammation and tissue repair in rheumatoid arthritis: A case control study. BMC Med. Genet. 12, 36. doi:10.1186/1471-2350-12-36
Esposito, S., Molteni, C. G., Giliani, S., Mazza, C., Scala, A., Tagliaferri, L., et al. (2012a). Toll-like receptor 3 gene polymorphisms and severity of pandemic A/H1N1/2009 influenza in otherwise healthy children. Virol. J. 9, 270. doi:10.1186/1743-422X-9-270
Esposito, S., Molteni, C. G., Zampiero, A., Baggi, E., Lavizzari, A., Semino, M., et al. (2012b). Role of polymorphisms of toll-like receptor (TLR) 4, TLR9, toll-interleukin 1 receptor domain containing adaptor protein (TIRAP) and FCGR2A genes in malaria susceptibility and severity in Burundian children. Malar. J. 11, 196. doi:10.1186/1475-2875-11-196
Figueroa, L., Xiong, Y., Song, C., Piao, W., Vogel, S. N., and Medvedev, A. E. (2012). The Asp299Gly polymorphism alters TLR4 signaling by interfering with recruitment of MyD88 and TRIF. J. Immunol. 188, 4506–4515. doi:10.4049/jimmunol.1200202
Freitas, M. S., Oliveira, A. F., Da Silva, T. A., Fernandes, F. F., Gonçales, R. A., Almeida, F., et al. (2016). Paracoccin induces M1 polarization of macrophages via interaction with TLR4. Front. Microbiol. 7, 1003. doi:10.3389/fmicb.2016.01003
Garlet, G. P., Trombone, A. P. F., Menezes, R., Letra, A., Repeke, C. E., Vieira, A. E., et al. (2012). The use of chronic gingivitis as reference status increases the power and odds of periodontitis genetic studies: A proposal based in the exposure concept and clearer resistance and susceptibility phenotypes definition. J. Clin. Periodontol. 39, 323–332. doi:10.1111/j.1600-051X.2012.01859.x
Golovkin, A. S., Ponasenko, A. V., Yuzhalin, A. E., Salakhov, R. R., Khutornaya, M. V., Kutikhin, A. G., et al. (2015). An association between single nucleotide polymorphisms within TLR and TREM-1 genes and infective endocarditis. Cytokine 71, 16–21. doi:10.1016/j.cyto.2014.08.001
Gond, D. P., Singh, S., and Agrawal, N. K. (2018). Testing an association between TLR4 and CXCR1 gene polymorphisms with susceptibility to urinary tract infection in type 2 diabetes in north Indian population. Gene 641, 196–202. doi:10.1016/j.gene.2017.10.060
Huang, W.-L., Xu, Y., and Wan, S.-P. (2020). Association of toll-like 4 receptor gene polymorphism (rs4986790, rs4986791) with the risk of urinary tract infection: A systematic review and meta-analysis. Kaohsiung J. Med. Sci. 36, 206–211. doi:10.1002/kjm2.12158
Hussein, A., Saad, K., Askar, E., Zahran, A. M., Farghaly, H., Metwalley, K., et al. (2018). Functional variants in intercellular adhesion molecule-1 and toll-like receptor-4 genes are more frequent in children with febrile urinary tract infection with renal parenchymal involvement. Acta Paediatr. 107, 339–346. doi:10.1111/apa.14118
Ishikawa, É. K. S., and Gomide, L. M. M. (2019). Doenças emergentes E reemergentes: Um problema do passado que persiste No presente. Rev. InterSaúde 1, 59–72.
Jabłońska, A., Studzińska, M., Szenborn, L., Wiśniewska-Ligier, M., Karlikowska-Skwarnik, M., Gęsicki, T., et al. (2020). TLR4 896A/G and TLR9 1174G/A polymorphisms are associated with the risk of infectious mononucleosis. Sci. Rep. 10, 13154. doi:10.1038/s41598-020-70129-4
Janardhanan, J., Joseph Martin, S., Astrup, E., Veeramanikandan, R., Aukrust, P., Abraham, O. C., et al. (2013). Single-nucleotide polymorphisms in Toll-like receptor (TLR)-2, TLR4 and heat shock protein 70 genes and susceptibility to scrub typhus. J. Hum. Genet. 58, 707–710. doi:10.1038/jhg.2013.89
Janeway, C. A., and Medzhitov, R. (2002). Innate immune recognition. Annu. Rev. Immunol. 20, 197–216. doi:10.1146/annurev.immunol.20.083001.084359
Johnson, K. R., Zheng, Q. Y., and Noben-Trauth, K. (2006). Strain background effects and genetic modifiers of hearing in mice. Brain Res. 1091, 79–88. doi:10.1016/j.brainres.2006.02.021
Khanmohammadi, S., and Rezaei, N. (2021). Role of Toll-like receptors in the pathogenesis of COVID-19. J. Med. Virol. 93, 2735–2739. doi:10.1002/jmv.26826
Kim, Y.-C., and Jeong, B.-H. (2020). Strong association of the rs4986790 single nucleotide polymorphism (SNP) of the toll-like receptor 4 (TLR4) gene with human immunodeficiency virus (HIV) infection: A meta-analysis. Genes (Basel) 12, 36. doi:10.3390/genes12010036
Kresfelder, T. L., Janssen, R., Bont, L., Pretorius, M., and Venter, M. (2011). Confirmation of an association between single nucleotide polymorphisms in the VDR gene with respiratory syncytial virus related disease in South African children. J. Med. Virol. 83, 1834–1840. doi:10.1002/jmv.22179
Kurt-Jones, E. A., Popova, L., Kwinn, L., Haynes, L. M., Jones, L. P., Tripp, R. A., et al. (2000). Pattern recognition receptors TLR4 and CD14 mediate response to respiratory syncytial virus. Nat. Immunol. 1, 398–401. doi:10.1038/80833
Kuzmich, N. N., Sivak, K. V., Chubarev, V. N., Porozov, Y. B., Savateeva-Lyubimova, T. N., and Peri, F. (2017). TLR4 signaling pathway modulators as potential therapeutics in inflammation and sepsis. Vaccines 5, 34. doi:10.3390/vaccines5040034
Lee, S.-O., Brown, R. A., Kang, S. H., Abdel Massih, R. C., and Razonable, R. R. (2011). Toll-like receptor 4 polymorphisms and the risk of gram-negative bacterial infections after liver transplantation. Transplantation 92, 690–696. doi:10.1097/TP.0b013e31822b589f
Li, W., Cao, X., He, L., Meng, H., Yang, B., and Liao, Y. (2019). TLR4 polymorphisms may increase susceptibility to periodontitis in Pg-positive individuals. PeerJ 7, e7828e7828. doi:10.7717/peerj.7828
Liadaki, K., Petinaki, E., Skoulakis, C., Tsirevelou, P., Klapsa, D., Germenis, A. E., et al. (2011). Toll-like receptor 4 gene (TLR4), but not TLR2, polymorphisms modify the risk of tonsillar disease due to Streptococcus pyogenes and Haemophilus influenzae. Clin. Vaccine Immunol. 18, 217–222. doi:10.1128/CVI.00460-10
Liberati, A., Altman, D. G., Tetzlaff, J., Mulrow, C., Gøtzsche, P. C., Ioannidis, J. P. A., et al. (2009). The PRISMA statement for reporting systematic reviews and meta-analyses of studies that evaluate healthcare interventions: Explanation and elaboration. BMJ 339, b2700. doi:10.1136/bmj.b2700
Lizundia, R., Sauter, K.-S., Taylor, G., and Werling, D. (2008). Host species-specific usage of the TLR4-LPS receptor complex. Innate Immun. 14, 223–231. doi:10.1177/1753425908095957
Medzhitov, R., and Janeway, C. J. (2000). Innate immune recognition: Mechanisms and pathways. Immunol. Rev. 173, 89–97. doi:10.1034/j.1600-065x.2000.917309.x
Medzhitov, R., Preston-Hurlburt, P., and Janeway, C. A. J. (1997). A human homologue of the Drosophila Toll protein signals activation of adaptive immunity. Nature 388, 394–397. doi:10.1038/41131
Mrazek, F., Gallo, J., Stahelova, A., and Petrek, M. (2013). Coding variants of TLR2 and TLR4 genes do not substantially contribute to prosthetic joint infection. Inflamm. Res. 62, 483–487. doi:10.1007/s00011-013-0601-8
Mukherjee, S., Huda, S., and Sinha Babu, S. P. (2019). Toll-like receptor polymorphism in host immune response to infectious diseases: A review. Scand. J. Immunol. 90, e12771. doi:10.1111/sji.12771
Ohto, U., Yamakawa, N., Akashi-Takamura, S., Miyake, K., and Shimizu, T. (2012). Structural analyses of human Toll-like receptor 4 polymorphisms D299G and T399I. J. Biol. Chem. 287, 40611–40617. doi:10.1074/jbc.M112.404608
Olejnik, J., Hume, A. J., and Mühlberger, E. (2018). Toll-like receptor 4 in acute viral infection: Too much of a good thing. PLoS Pathog. 14, e1007390. doi:10.1371/journal.ppat.1007390
Park, B. S., Song, D. H., Kim, H. M., Choi, B.-S., Lee, H., and Lee, J.-O. (2009). The structural basis of lipopolysaccharide recognition by the TLR4-MD-2 complex. Nature 458, 1191–1195. doi:10.1038/nature07830
Prohinar, P., Rallabhandi, P., Weiss, J. P., and Gioannini, T. L. (2010). Expression of functional D299G.T399I polymorphic variant of TLR4 depends more on coexpression of MD-2 than does wild-type TLR4. J. Immunol. 184, 4362–4367. doi:10.4049/jimmunol.0903142
Qin, Y., Zhang, L., Xu, Z., Zhang, J., Jiang, Y., Cao, Y., et al. (2016). Innate immune cell response upon Candida albicans infection. Virulence 7, 512–526. doi:10.1080/21505594.2016.1138201
Ragnarsdóttir, B., Jönsson, K., Urbano, A., Grönberg-Hernandez, J., Lutay, N., Tammi, M., et al. (2010). Toll-like receptor 4 promoter polymorphisms: Common TLR4 variants may protect against severe urinary tract infection. PLOS ONE 5, e10734. doi:10.1371/journal.pone.0010734
Ragnarsdóttir, B., Samuelsson, M., Gustafsson, M. C., Leijonhufvud, I., Karpman, D., and Svanborg, C. (2007). Reduced toll-like receptor 4 expression in children with asymptomatic bacteriuria. J. Infect. Dis. 196, 475–484. doi:10.1086/518893
Reis, L. M., Rodrigues, F. W., Silva, R. E. da, Taleb, A. C., Ávila, M. P. de, and Avila, M. P. d. (2016). Análise da relação da ocorrência de polimorfismo de nucleotídeo único do gene DOCK9 em ceratocone. Rev. Bras. Oftalmol. 75, 223–227. doi:10.5935/0034-7280.20160046
Reza Mortazavi-Tabatabaei, S., Ghaderkhani, J., Nazari, A., Sayehmiri, K., Sayehmiri, F., and Pakzad, I. (2019). Pattern of antibacterial resistance in urinary tract infections: A systematic review and meta-analysis. Int. J. Prev. Med. 10, 169. Available at: https://www.ijpvmjournal.net/article.asp?issn=2008-7802;year=2019;volume=10;issue=1;spage=169;epage=169;aulast=Reza.
Ruch, T. R., and Engel, J. N. (2017). Targeting the mucosal barrier: How pathogens modulate the cellular polarity network. Cold Spring Harb. Perspect. Biol. 9, a027953. doi:10.1101/cshperspect.a027953
Sahingur, S. E., Xia, X.-J., Gunsolley, J., Schenkein, H. A., Genco, R. J., and De Nardin, E. (2011). Single nucleotide polymorphisms of pattern recognition receptors and chronic periodontitis. J. Periodontal Res. 46, 184–192. doi:10.1111/j.1600-0765.2010.01327.x
Sampath, V., Mulrooney, N. P., Garland, J. S., He, J., Patel, A. L., Cohen, J. D., et al. (2013). Toll-like receptor genetic variants are associated with Gram-negative infections in VLBW infants. J. Perinatol. 33, 772–777. doi:10.1038/jp.2013.80
Sánchez, D., Rojas, M., Hernández, I., Radzioch, D., García, L. F., and Barrera, L. F. (2010). Role of TLR2- and TLR4-mediated signaling in Mycobacterium tuberculosis-induced macrophage death. Cell. Immunol. 260, 128–136. doi:10.1016/j.cellimm.2009.10.007
Santos, C. M. da C., Pimenta, C. A. de M., and Nobre, M. R. C. (2007). The PICO strategy for the research question construction and evidence search. Rev. Lat. Am. Enferm. 15, 508–511. doi:10.1590/S0104-11692007000300023
Sellers, R. M., Payne, J. B., Yu, F., LeVan, T. D., Walker, C., and Mikuls, T. R. (2016). TLR4 Asp299Gly polymorphism may be protective against chronic periodontitis. J. Periodontal Res. 51, 203–211. doi:10.1111/jre.12299
Sghaier, I., Zidi, S., Mouelhi, L., Ghazoueni, E., Brochot, E., Almawi, W. Y., et al. (2019). TLR3 and TLR4 SNP variants in the liver disease resulting from Hepatitis B virus and hepatitis C virus infection. Br. J. Biomed. Sci. 76, 35–41. doi:10.1080/09674845.2018.1547179
Sharma, S., Singh, S. K., Kakkar, K., Nyari, N., Dhole, T. N., Kashyap, R., et al. (2016). Analysis of TLR4 (Asp299Gly and Thr399Ile) gene polymorphisms and mRNA level in patients with dengue infection: A case-control study. Infect. Genet. Evol. 43, 412–417. doi:10.1016/j.meegid.2016.06.027
Silva, M. J. A., Lima, M. B. M., Lima, K. V. B., and Lima, L. N. G. C. (2021). The relationship of TLR2 polymorphisms with infectious diseases. Annu. Res. Rev. Biol., 57–73. doi:10.9734/arrb/2021/v36i1130451
Souza, E. M. de, and Grundy, E. (2004). Health promotion, social epidemiology, and social capital: Associations and perspectives for public health. Cad. Saúde Pública 20, 1354–1360. doi:10.1590/S0102-311X2004000500030
Taha, H. A., Sheir, R. E., Shafy, S. S. A., and Mohamed, L. M. (2014). Genotypic analysis of Asp299Gly and Thr399Ile polymorphisms of TLR4 in Egyptian patients with rheumatoid arthritis and systemic lupus erythematosus. Egypt. J. Intern. Med. 26, 15–20. doi:10.4103/1110-7782.132884
Taha, S. I., Shata, A. K., Baioumy, S. A., Fouad, S. H., Anis, S. G., Mossad, I. M., et al. (2021). Toll-like receptor 4 polymorphisms (896A/G and 1196C/T) as an indicator of COVID-19 severity in a convenience sample of Egyptian patients. J. Inflamm. Res. 14, 6293–6303. doi:10.2147/JIR.S343246
Takeuchi, O., Hoshino, K., Kawai, T., Sanjo, H., Takada, H., Ogawa, T., et al. (1999). Differential roles of TLR2 and TLR4 in recognition of gram-negative and gram-positive bacterial cell wall components. Immunity 11, 443–451. doi:10.1016/S1074-7613(00)80119-3
Torres-García, D., Cruz-Lagunas, A., García-Sancho Figueroa, M. C., Fernández-Plata, R., Baez-Saldaña, R., Mendoza-Milla, C., et al. (2013). Variants in toll-like receptor 9 gene influence susceptibility to tuberculosis in a Mexican population. J. Transl. Med. 11, 220. doi:10.1186/1479-5876-11-220
Varzari, A., Deyneko, I. V., Vladei, I., Grallert, H., Schieck, M., Tudor, E., et al. (2019). Genetic variation in TLR pathway and the risk of pulmonary tuberculosis in a Moldavian population. Infect. Genet. Evol. 68, 84–90. doi:10.1016/j.meegid.2018.12.005
Vaure, C., and Liu, Y. (2014). A comparative review of toll-like receptor 4 expression and functionality in different animal species. Front. Immunol. 5, 316. doi:10.3389/fimmu.2014.00316
Vidyant, S., Chatterjee, A., and Dhole, T. N. (2019). A single-nucleotide polymorphism in TLR4 is linked with the risk of HIV-1 infection. Br. J. Biomed. Sci. 76, 59–63. doi:10.1080/09674845.2018.1559486
Waldman, E. A., Silva, L. J. da, and Monteiro, C. A. (1999). Trajetória das doenças infecciosas: Da eliminação da poliomielite à reintrodução da cólera. Inf. Epidemiol. Sus 8, 05–47. doi:10.5123/S0104-16731999000300002
World Health Organization, (2000). The world health report : 2000 : Health systems : Improving performance. Available at: https://apps.who.int/iris/handle/10665/42281 (Accessed July 22, 2021).
Keywords: immunogenetics, infectious diseases, association studies, single nucleotide polymorphism, toll-like receptor 4 (TLR4)
Citation: Silva MJA, Santana DS, de Oliveira LG, Monteiro EOL and Lima LNGC (2022) The relationship between 896A/G (rs4986790) polymorphism of TLR4 and infectious diseases: A meta-analysis. Front. Genet. 13:1045725. doi: 10.3389/fgene.2022.1045725
Received: 15 September 2022; Accepted: 10 November 2022;
Published: 24 November 2022.
Edited by:
Fatma Savran Oguz, Istanbul University, TurkeyReviewed by:
Marcia Holsbach Beltrame, Federal University of Paraná, BrazilEbru Karpuzoglu, University of Georgia, United States
Copyright © 2022 Silva, Santana, de Oliveira, Monteiro and Lima. This is an open-access article distributed under the terms of the Creative Commons Attribution License (CC BY). The use, distribution or reproduction in other forums is permitted, provided the original author(s) and the copyright owner(s) are credited and that the original publication in this journal is cited, in accordance with accepted academic practice. No use, distribution or reproduction is permitted which does not comply with these terms.
*Correspondence: Marcos Jessé Abrahão Silva, amVzc2VhYnJhaGFvMTBAZ21haWwuY29t