- 1Clinical Medicine, China-Japan Union Hospital of Jilin University Norman Bethune Third School of Jilin University, Changchun, China
- 2Department of Otorhinolaryngology Head and Neck Surgery, China-Japan Union Hospital of Jilin University Norman Bethune Third School of Jilin University, Changchun, China
MicroRNAs (miRNAs), a subset of endogenous RNAs highly conservative with short chains, play key regulatory role in the biological relevant events of the cells. Exosomes are extracellular vesicles like the plasma membrane components being able to deliver information molecules such as miRNA between cells and to regulate the fate of the target cells. The progression of chronic rhinosinusitis with nasal polyps (CRSwNP) is closely associated with significant alterations of miRNA levels in both cells and exosomes. RNA-binding proteins (RBPs) have been acknowledged to play important roles in intracellular miRNA transport to exosomes, and specific membrane proteins such as caveolin-1 critically involved in HNRNPA1 -mediated transport of miRNA to exosomes. Aberrant alteration in endogenous miRNA levels significantly contributes to the process of airway remodeling in the nasal tissue and to the occurrence and progression of inflammatory responses in CRSwNP. Exogenous miRNAs delivered via exosomes has also been shown to play an important role in activating macrophages or in regulating vascular permeability in the CRSwNP.This paper highlights the mechanism of RBP-mediated delivery of miRNAs to exosomes and the important contribution of endogenous miRNAs to the development of CRSwNP in response to inflammation and airway remodeling. Finally, we discuss the future research directions for regulation of the miRNAs to CRSwNP.Delivery of exogenous miRNAs by exosomes alters the endogenous miRNAs content in nasal mucosal epithelial cells or in associated inflammatory cells in the CRSwNP, and altered endogenous miRNAs affects the inflammatory response and airway remodeling, which then regulates the occurrence and progression of CRSwNP.RBPs and associated membrane proteins such as caveolin-1 may play a crucial role in the entry of exogenous miRNA into exosomes.
1 Introduction
MiRNAs are a set of endogenous single-stranded small molecule RNAs with length of 18–22 nt, non-translatable, and generated via two successive steps of processing from primary-miRNAs (pri-miRNAs) to precursors of miRNAs (pre-miRNA) and from pre-miRNAs to mature miRNAs (Lee et al., 2004) (Figure 1). Molecularly, miRNAs regulate gene expression at the transcriptional and post-transcriptional levels, whereas biologically, they are critically involved in almost all the known biological events such as cell proliferation and differentiation, development, reproduction, and aging (Chen and Wang, 2012; Gerasymchuk et al., 2020; Wang et al., 2021). Aberrant alterations of the miRNA abundance and the species often initiate pathogenesis of many diseases, initially making it plausible to speculate that the miRNAs could become the potential biomarkers translatable to clinic therapy for many kind of diseases. Indeed, so far many miRNAs have been acknowledged as the key regulators implicated in various disease such as cancers and neurological disorders, some of which have been developed as therapeutic targets for some stubborn diseases (Sun et al., 2018; Peng et al., 2020).
The abundance of miRNAs in cells is dynamically regulated in accordance with physiological conditions and development stage. Aberrant alteration of miRNAs may significantly contribute to initiation and/or progression of diseases. Several mechanisms account for the dynamic alteration of miRNAs abundance. The common one is up-regulation or downregulation of miRNA genes, while the enhanced or blocked miRNA package into exosomes and transportation to outside cells also become factors.
The occurrence and progression of CRSwNP is associated with alteration of intracellular miRNA levels, although the differential expression of miRNA was also detected in the nasal lavage fluid (NLF)-EV (extracellular vesicles) of CRSwNP patients (Cha et al., 2021). Some miRNAs can regulate the epithelial-mesenchymal transition (EMT) to affect the airway remodeling of CRSwNP (Liu et al., 2021b), of which is miR-155 that could be regulated by glucocorticoids, commonly serving as clinical therapy (Zheng et al., 2012). Both studies demonstrate that miRNA is involved in the development and progression of nasal polyps at both the phenotypic and molecular levels, suggesting the importance of miRNAs in CRSwNP in exploration of the occurrence mechanism of CRSwNP and clinic therapy.
The CRSwNP patients with the prevalence of 0.5%–4.3% developed clinical manifestations such as unconsciousness, dizziness, memory loss and hyposmia, and is often accompanied by asthma comorbidity and postoperative recurrence (Table 1). The CRSwNP mainly showed Th2 cell inflammation, mainly involving the effects of IL-4, IL-5, and IL-13, accompanied by changes in IL-25, IL-33, TSLP and IgE levels, with eosinophil infiltration and nasal polyps phenomenon (Fossiez et al., 1996). Besides, CRSwNP is associated with elevated levels of eosinophils, innate lymphoid cells (ILC2), macrophages and mast cells (Lee et al., 2021). NP is characterized by epithelial cell alterations, epithelial mesenchymal transformation, goblet cell hyperplasia, extracellular matrix degradation, fibrin deposition, and tissue edema.
2 The function of inflammatory cells in the CRSwNP
One of the important pathological features exhibited by CRSwNP is inflammatory cell infiltration. Infiltrating inflammatory cells include nasal epithelial cells, ILC2, eosinophils, mast cell, macrophage and Th1/Th2/Th17/Treg cell, mostly Th2 cells. The inflammatory cell infiltration is accompanied by changes of cytokines and chemokine expression. Nasal epithelial cells are capable of regulating the development of Type II inflammatory response by releasing TSLP, IL-33 and IL-25. Activated ILC2 leads to eosinophilia mainly by releasing IL-5, whereas release of IL-13 promotes airway hyperresponsiveness, goblet cell hyperplasia, mucus production, and DC activation. Th2 cells releases IL-4, IL-5, and IL-13 participates in the inflammatory response, and eosinophils releases the chemokine CCL23 to recruit M2 macrophages to release chemical mediators, such as histamine, to induce mucosal oedema and local inflammation. Activated macrophages release proinflammatory factors and recruit inflammatory cells to participate in the inflammatory response. The Th1/Th2/Th17/Treg cell balance directed towards Th2 mediates the progression of the type 2 inflammatory response.
2.1 Nasal epithelial cells
Nasal epithelium cells in CRSwNP are able to release IL-25, IL-33 and TSLP by which are able to activate type 2 innate lymphoid cells (ILC2) and to increase the production of type 2 cytokines (Fujieda et al., 2019). At the same time, TLSP can activate dendritic cells (DCs) to present antigen and costimulatory signals, and differentiate naive T cells into effector Th2 cells, causing the release of type 2 cytokine to increase (Patel et al., 2019). Type II inflammatory response can damage the epithelial cells of the nasal mucosa, improve the nasal mucosal epithelial permeability, and decrease mucosal barrier function, basement membrane thickening, stromal fibrosis, epithelium to mesenchymal transformation (EMT), airway remodeling and other phenomena.
2.2 ILC2
Epithelial-derived cytokines as well as other biological mediators such as lipid mediators enable to activate ILC2. The activated ILC2 can release IL-4, IL-5, IL-8, IL-9, IL-13, GM-CSF, and Th2 cells to enhance type 2 immune responses. Releases of IL-5 and IL-13, but only the IL-5 by the activated ILC2 could raise eosinophil levels in contrast to IL-13 that leads to airway hyper-response (AH), increasing the number of goblet cells and enhancing mucus secretion. Furthermore, IL-4, IL-5 and IL-9 released by the activated ILC2 could induce B cell proliferation and IgE production. By contrast, IL-8 and GM-CSF released by ILC2 activate neutrophils and macrophages (Poposki et al., 2017; van der Ploeg et al., 2020). IL-9 released by IL2 could maintain ILC2 survival in polyp tissue and increase the levels of mast cells. ICL2 releases cytokines, such as IL-2, IL-4, and IL-13, and induces Th2 cell proliferation and promote the occurrence of dimorphic inflammatory response (Li et al., 2021).
2.3 Th2 cells, together with eosinophils
DCs stimulate the differentiation of naive T cells into effector Th2 cells, increasing the secretion of type-2 cytokines such as IL-4, IL-5, and IL-13 (Fossiez et al., 1996). While IL-4 promotes IgE production (Punnonen et al., 1997), IL-5 is able to induce the production of eosinophilic extracellular traps (Hamilos et al., 1995). The chemokine eosinophils (Cho-C motif) ligand (CCL) 23 recruits macrophages and differentiate into M2 macrophages. CCL23 released by eosinophils recruits macrophages and differentiates into M2 macrophages (Poposki et al., 2011).
2.4 Mast cell
Activated mast cells release chemical mediators, such as histamine, to induce mucosal oedema and local inflammation. Upon stimulation with TSLP, IL-33, and IL-1, mast cells secrete IL-3 and IL-13 (Saluja et al., 2016). Both IgE and mast cells numbers increase in eosinophilic CRSwNP, and IgE is involved in regulating the regulation and activation of mast cell degranulation. Allergens were found to be able to bind to specific IgE on CRSwNP mast cells, activate mast cells and release a variety of pro-inflammatory mediators and cytokines such as prostaglandin D2, prompting the development of an inflammatory response (Cao et al., 2014).
2.5 Macrophage
Activated macrophages not only release the proinflammatory cytokines TNF and IL-1, but also could differentiate into M2 macrophages thereby releasing paclitaxel and chemokine CCL18 and recruiting eosinophils, Th2 cells and myeloid dendritic cells involved in inflammatory cell infiltration and inflammatory response of CRSwNP (Peterson et al., 2012).
2.6 Th1/Th2/Th17/treg cell
The traditional view is that Th0 cells from CRSwNP patients tend to differentiate into Th2 cells stimulated by inflammatory factors, disrupting the original Th1/Th2 cell balance and mediating the occurrence of type 2 inflammatory response (Omori and Ziegler, 2007). Recent studies have found that the Th17/Treg cell balance has an important role in the occurrence and progression of the inflammatory response. CD4 + T cells differentiate into Th17 cells in the presence of inflammatory factors, and the released IL-17 is able to trigger an inflammatory response (Fossiez et al., 1996), both of which can differentiate into Treg cells and play an immunosuppressive function in the absence of inflammatory factors and TGF-β (Fontenot et al., 2017). The development of Th17/Treg balance towards Th17 is considered an important feature of the inflammatory response as well. More importantly, the extended Th1/Th2/Th17/Treg cell pattern could be essential to understand inflammatory mechanisms at the molecular level.
3 Exosomes
3.1 Structure and components of the exosomes
Exosomes are membrane-bound vesicles with a similar structure to the plasma membrane, containing miRNA, mRNA, and proteins and being capable of transferring from the original cells to the corresponding target cells via body fluids (Jia et al., 2017). It has been confirmed that the nasal lavage fluid contained exosomes and the components have been identified to be surface markers CD9, CD63 and CD81 using flow cytometry (Lässer et al., 2011). These findings are to compare the difference in exosome components in nasal lavage fluid between normal persons and CRSwNP patients.
3.2 Generation and exportation of exosome
Exosomes are microvesicles with diameter in 30–100 nm and morphologically with a “dish” morphology consisting of a lipid bilayer. Their precursors are luminal vesicles (ILVs) in cellular polyvesicles (MVBs), and after MVB fusion with the cell membrane the precursors are released into the extracellular form of exosomes.
Exosomes contain receptors and transmembrane proteins on the cell membrane, and their formation starts as early endosomes formed by cell membrane depressions. Following that, they gradually become a late endosome, budding into multiple vesicles (MVBs), and selectively transporting intracellular proteins and specific miRNA to the luminal vesicles (ILVs). Subsequently, the multi-vesicle bodies fuse with the cell membrane to release luminal vesicles outside the cell and thereby become exosomes. Exosomes secreted by cells can be transported through extracellular fluid such as serum, lymph and taken up by target cells, accordingly causing biological consequences (Jia et al., 2017). However, the involvement of exosomes in signaling between inflammatory cells, as well as the migration of nasal polyps and inflammatory responses has been less explored. It is plausible to speculate that the understanding exosomes-mediated intercellular signaling may provide essential information for pathogenesis and clinical therapy for the nasal polyps.(Figure 2)
3.3 Differential expression of miRNA in CRSwNP
Significant difference in exosomes miRNA levels were detected between normal persons and CRSwNP patients. In the study of Xuan and Xia, the levels of miR-155, miR-375, miR-671-3p, and miR-92a-3p were associated with CRSwNP development via the same controls (Xia et al., 2015; Xuan et al., 2019). Analysis on GO enrichment and KEGG pathways showed that 192 of miRNA expression was significantly downregulated, but no substantial upregulation was observed in CRSwNP relative to normal control (Yu et al., 2021). Therefore, it could be concluded that the occurrence of CRSwNP is associated with the downregulation of miRNAs, suggesting that miRNAs with significant alteration in the expression levels could contribute to physiolpathology and be potentially developed as therapeutic targets for clinical therapy of CRSwNP patients.
4 Alteration of miRNAs levels in the inflammatory cells in response to inflammation stimuli
CRSwNP mainly showed an inflammatory response in Th2 cells, along with the phenomenon of eosinophil infiltration. The level of the inhibitory cytokine IL-10 was able to affect CRSwNP progression, and the TNF-α expression is also important for CRSwNP progression. Some miRNA were found to influence the occurrence and progression of nasal polyps by regulating the levels of IL-10 or TNF-α.
4.1 The miRNA-21 was able to increase the IL-10 levels in the nasal epithelial cells to inhibit the inflammatory response
The miR-21 functions as negative feedback regulator for the CRSwNP via reducing the levels of proinflammatory cytokines. By targeting to PDCD4 mRNA, the miR-21 inhibited PDCD4 translation, thereby reducing the P65 phosphorylation of NF-kB and consequently repressing the inhibitory effect of nuclear IL-10 gene expression and elevating IL-10 levels. IL-10 could inhibit the expression of proinflammatory cytokines such as IL-25, IL-33, TSLP, IL-1, IL-6 and IL-8, thus inhibiting the progression of inflammatory response (Liu et al., 2021a). Therefore, the miR-21 confers anti-inflammatory effect via directly elevating the level of IL-10 targeting to the miR-21/PDCD4/NF-kB pathway thereby downregulating the expression of inflammatory factors.
4.2 Elevation of miR-142-3p level enhances TNF-α expression in nasal epithelial cells and exaggerates the inflammatory response
Co-elevation of both miR-142-3p and TNF-α levels were observed in human nasal epithelial cells upon stimulation with LPS in a way that the abundance of both miR-142-3p and TNF-α were positively correlated. It has been acknowledged that co-upregulation of both miR-142-3p and TNF-α in nasal epithelial cells could enhance the inflammatory response. The related molecular mechanism is the induced expression of TNF-α by the elevation of miR-142-3p promotes the release of IL-6, IL-10, and IFN-γ from monocyte macrophages and eosinophils, whereas induced release of NF-kB, C-fos and C-Jun subsequently regulates the inflammatory response, and vice versa (Yang et al., 2018). Therefore, it could be speculated that miR-142-3p might be involved in the inflammatory response through the LPS-TLR-TNF-α signaling pathway (Qing et al., 2021).
4.3 The miRNA-19a down-regulates IL-10 levels in peripheral dendritic cells and promoted the inflammatory response
In response to TLSP, peripheral dendritic cells (DCs) activate and present antigen and costimulatory signals to differentiate naive T cells into effector Th2 cells, while secrete IL-10 to reduce the inflammatory response. Significantly upregulated level of the IL-4 due to elevated miR-19a levels in CRSwNP patients could suppress IL-10 expression in dendritic cells. Furthermore, IL-4 suppresses the transcription of the IL-10 gene in response to HDAC11, the gene transcriptional repressor (Luo et al., 2017), thus significantly increasing the levels of miR-19a and IL-10 in DC cells of CRSwNP patients (Simpson et al., 2014).
5 Alteration of miRNAs in CRSwNP effects airway remodeling
5.1 Airway remodeling in the CRSwNP
In CRSwNP, airway remodeling is mainly characterized by fibrin accumulation, increasing mucin-containing mucus and epithelial mesenchymal transition. The increase of coagulation factor expression and decreased fibrinolytic activity in nasal tissue induced the accumulation of fibrin in nasal polyps. Th2 cytokines in CRSwNP can inhibit t-PA expression in nasal epithelial cells and inhibit fibrin degradation, resulting in edema eosinophilic nasal polyps in type 2 inflammation, and the increase of coagulation factor XIIIa in type 2 nasal polyps contribute to fibrin accumulation as well (Takabayashi et al., 2013a; Takabayashi et al., 2013b). Type 2 inflammatory cytokines such as IL-4, IL-13, IL-8, and IL-33 in CRSwNP patients enhanced expression of MUC5AC in nasal polyp-derived epithelial cells (Zhang et al., 2019). In response to IL-13, the expression of anion transporter pendrin protein was increased in the epithelial cells of CRSwNP, leading to the increased mucus production (Seshadri et al., 2015). EMT causes loss of cell connectivity and polarity in CRSwNP tissues, conversion of nasal epithelial cells to mesenchymal cell phenotypes, downregulation of epithelial markers expression such as e-cadherin and SIRT1 and upregulation of mesenchymal markers expression such as-SMA and MMP (Thiery et al., 2009). Of the markers, MMP can degrade specific extracellular matrix (ECM) components. Indeed, significant elevation of MMP2 and MMP9 levels observed, while the TIMP1 and TIMP4 levels are significantly attenuated in CRSwNPs, which is closely related to the occurrence of polyps in CRSwNP (Li et al., 2010).
5.2 Airway remodeling-related miRNA in CRSwNP
5.2.1 Upregulation of miR1555p promotes EMT
Elevation of miR155-5p levels in nasal epithelial cells caused by enhanced TGF-β1 levels in CRSwNP patients could more efficiently target to SIRT1, participate in EMT progression in epithelial cells and affect the airway remodeling process in nasal tissue (Yang et al., 2020). This is further evidenced by the factor that a miR155-5p inhibitor can attenuate the effect on SIRT1 and subsequently inhibit EMT, thus surpressing the polyposogenesis of CRSwNP.
5.2.2 TGF-β1 induces the nasal epithelial cell-mesenchymal transformation via miR-21
TGF-β1, a representative cytokine in tissue remodeling, could activate EMT signaling to initiate tissue remodeling of the airway epithelium and nasal tissue, leading to the altered epithelial cell morphology and upregulation of mesenchymal protein and miR-21 expression. Previous studies have observed significant upregulation of both TGF-β1mRNA and miR-21 in CRSwNP patients relative to normal control group. Consistently, the miR-21 inhibitor and an Akt-specific inhibitor could inhibit TGF-β1-induced EMT, whereas the reduction in miR-21 abundance was accompanied by elevation of the PTEN levels and decrease in Akt phosphorylation (Li et al., 2019). Therefore, it is reasonable to suggest that miR-21 or the TGF-β1-miR-21-PTEN-Akt axis may potentially developed as targets for clinical therapy of CRSwNP.
5.2.3 miR29b3p promotes α-tubulin deacetylation-mediated tissue remodeling
MMP9 is able to bind to integrin proteins and regulate α-tubulin acetylation and deacetylation. Acetyl-α-tubulin could affect cell morphology and got involved in the formation of nasal polyps in CRSwNP. MiR29b-3p and TMP1 play counterpart roles in terms of MM9 expression regulation in ways that miR-29b-3p stimulates expression of MMP9, while TIMP1 reduces MMP9 abundance. miR29b3p affects acetyl-α-tubulin levels by enhancing the interaction between MMP9 and integrin 1, the lack of which is associated with epithelial mesenchymal transformation induced by TGF-β (Liu et al., 2021b). Thus, regulation of α-tubulin acetylation levels by miR-293p could be another promising strategy for the treatment of nasal polyps (Table 2).
6 The miRNA is secreted to the exosome machinery
6.1 The role of RNA-binding protein in miRNA secretion
Cells selectively transport miRNA into exosomes, and the proteins involved in this process mainly include RNA-binding proteins such as hnRNPA2B1 with Argonaute-2, as well as exosome assembly-synthesized membrane proteins such as caveolin-1 and sphingophospholipase 2. RNA-binding protein (RBP) is a class of proteins that can specifically bind RNA molecules to mediate their entry into exosomes. Studies have found significant differences in concentrations between the intracellular miRNA and the secreted miRNA, indicating the essential role of RBP in miRNA entry into exosome.
6.2 The RNA-binding protein involved in the regulation of miRNA secretion to the exosomes
A number of RBPs have been characterized to be associated with the secretion of miRNA into exosomes, including hnRNPs, Ago2, YBX-1, MEX3C, MVP, and La proteins. hnRNPs play diversified roles in regulating transcriptional and post-transcriptional gene expression, Including RNA splicing, polyadenylation, capping, modification, export, localization and translation. hnRNPs bind to short RNA single strands through specific domains. For example, hnRNP-q contains a N-terminal domain that recognizes the GGCU sequence highly affinitive to the miRNAs. The miRNA binding domain in hnRNP-q could initiate and regulate transportation of miR-3470a and miR-194-2-3p into exosomes (Santangelo et al., 2016). In consideration of the essential roles of hnRNP-q, hnRNP-q downregulation could repress miRNA transportation into exosomes, leading to accumulation of miRNA in the cytoplasm.
Like hnRNP-q, ARGONAUNT2 (AGO2) is highly affinitive to miRNAs and can serve as carrier for transportation of miRNAs into exosomes. The AGO2 mediated miRNAs transportation is regulated by the KRAS-MEK-ERK pathway via alteration of the AGO2 phosphorylation levels. This was confirmed by the elevated miR-100 content of hyperphosphorylated KRAS mutations in vitro (Cha et al., 2015). The RNA-binding domain of YBX-1 enables it to interact with miR-133 and miR-223 for transportation of both miRNAs into exosomes. To provide evidence, reducing YBX-1 expression levels in H/R EPC reduced the abundance of miR-133 in exosomes, while the miR-133 abundance in the cytoplasm remains largely unaltered (Lin et al., 2019). Unlike hnRNP-q and AGO2 with miRNA binding domains for miRNA transportation into exosomes, it was found that MEX3C is not a direct target of miR-451a during miR-451a transportation to exosomes. The RBP MEX3C was involved in forming the MEX3C-Ago2 complex, mediating miR-451a entry into exosomes (Lu et al., 2017). This finding suggests the two RBP involved participation in the process of miRNA transport into exosomes, providing new perspectives on miRNA transport. Another RBP, Dome principal protein (MVP) is involved in the transport of RNA from nucleus to cytoplasm and from cytoplasm to exosomes. MVP overexpression in CT26 colon cancer cells leads to decreased abundance of miR-193a in the cells and increased levels in exosomes, suggesting the important role the MVP plays in the miR-193a transportation to exosomes. The La protein, a transcription factor of the RNA polymerase III and a RBP, was found to play important role in transportation of some miR-122 into exosomes. Downregulation of the La content in the cytoplasm would cause a decrease in miR-122 abundance in exosomes and vice versa (Temoche-Diaz et al., 2019).
6.3 The HNRNP-A1 recognizes the miRNA containing the AGAGGG motif and allows for delivery to the exosomes
HNRNP-A1 was found to specifically recognize miRNAs that contain AGAGGG motifs such as miR-320 to mediate miRNA secretion to exosomes. Upon knockdown of HNRNPA1, miR-320 level increased significantly in the cytosol, and accordingly dramatic decrease in exosomes, suggesting the essential role of HNRNPA1 in maintenance of some miRNAs accumulation in exosomes (Gao et al., 2019). This further suggests that the synchronously dynamic fluctuation of miRNAs in cells and exosomes is mediated by RNPs. (Figure 3)
6.4 Membrane protein caveolin-1 is involved in HNRNPA2B1 regulation of miRNA transport to exosomes
RBPs, such as hnRNPs, Ago2, YBX-1, YBX-1, MEX3C, MVP and La proteins all bind miRNA and facilitate their transfer to exosomes. The membrane protein caveolin-1 was involved in the RBP- mediated miRNA entry into exosomes. Upon induction of oxidative stress, Y-14 of caveolin-1was phosphorylated in the lung epithelial cells to enable the CSD of caveolin-1 to interact with the RGG of HNRNPA2B1 to form a cav-1/HNRNPA2B1 complex (Lee et al., 2019). Importantly, previous study demonstrated the involvement of membrane proteins in the RBP-mediated entry of miRNA into exosomes.(Figure 4)
7 Effect of exosome-delivered miRNA on target cells
7.1 The miR-22-3p in the CRSwNP exosomes regulates vascular permeability by targeting the VE-calcium adhesion proteins
MiR-22-3p targets mRNA 3′-UTR, coding for VE-calcium adhesion protein. Significant upregulation of miR-22-3p expression in nasal lavage fluid of CRSwNP patients could downregulate expression of VE-cadherin, leading to abnormal vascular permeability and consequently causing the aberrant tissue edema and nasal polyp growth (Zhang et al., 2020). Different study further supports the conclusion that miR-22-3p in exosomes downregulates expression of the VE-calcium adhesion proteins to regulate CRSwNP occurrence and progression (Gu et al., 2017). Altogether suggests that exosome-delivered miRNA is capable of regulating CRSwNP progression via targeting adhesion protein molecules。
7.2 Exosome-delivered miR-17/93 is able to downregulate Irf2bp2 in macrophages to activate them
It was found that miR17/93-containing exosomes released by lung epithelial cells under oxidative stress were able to be ingested by macrophages, and thedelivered miR17/93 was able to reduce Irf2bp2 levels in macrophages. The Irf2bp2 suppresses macrophage activation, and the reduced level of Irf2bp2 in macrophages can activate macrophages and promote macrophage migration and the synthesis and release of cytokines such as TNF and IL-1 (Chen et al., 2015). It is confirmed that exogenously delivered miRNA can regulate the expression level of intracellular related proteins followed by causing the corresponding biological consequences. It is of great significance for miRNA transmission in CRSwNP to explore the occurrence mechanism and to develop clinical therapy for CRSwNP. (Figure 5)
8 Future
CRSwNP is an inflammatory respiratory disease with high global incidence and high recurrence, and limited information for its pathogenesis is available. Nasal epithelial cells, myeloid dendritic cells, ICL2 cells, eosinophils, mast cells, macrophages and Th2 cells are all involved in the inflammatory process of CRSwNP. The new Th1/Th2/Th17/Treg cell pattern opened a novel angle towards the mechanism of inflammatory response and progression.
Changes in cellular endogenous miRNA content have important effects on both inflammatory cytokine production and airway remodeling in CRSwNP. Regulation of intracellular miRNA levels has significant potential in treating CRSwNP and studying the pathogenesis of CRSwNP.
Given that the exosomes could be isolated from the Nasal lavage fluid in CRSwNP patients and as an easy way miRNAs could be profiled to identify and characterize, the exosomes and the contained miRNAs have become an efficient tool convenient for investigating the miRNA-mediated pathology for CRSwNP.
Exosome-delivered miR-22-3p in CRSwNP regulates vascular permeability by targeting ve-calcium adhesion proteins, and the exosome-delivered miR-17/93 in lung epithelial cells is able to downregulate Irf2bp2 in macrophages to activate macrophages. Cell-secreted exosomes have been shown to regulate the inflammatory response in the target cells, of which miRNA plays an important role. Extracellular stimulation signals transmitted by miRNA in exosomes can activate or inhibit inflammatory cells, cause intracellular-related biological events such as generation and release of the related inflammatory cytokines, and regulate the occurrence and progression of CRS. The study of miRNA in exosomes provides new angle for the development of novel drugs for therapy of CRSwNP.
Studies on the process of RBP in miRNA transport to exosomes revealed the causes of synchronized changes between endogenous miRNA and foreign miRNA in exosomes. Moreover, RBP is important for the entry of endogenous miRNA into exosomes. Several specific membrane proteins such as caveolin-1 have also been shown to be involved in RBP-mediated miRNA entry into exosomes, miRNA entry processes may involve more intracellular components, and miRNA transport into exosomes with greater research potential.
Altered miRNAs levels have important effects on inflammatory progression and airway remodeling in nasal polyps. Exogenous exosome-delivered miRNAs targets nasal mucosal epithelial cells as well as associated inflammatory cells in CRSwNP, changes their cellular endogenous miRNA content, and regulates the occurrence and progression of CRSwNP by regulating inflammatory responses and airway remodeling. This provides new ideas and methods to study the pathogenesis, treatment and prevention of nasal polyps, as well as to realize the precise treatment of nasal polyps and reduce the recurrence rate of nasal polyps after surgery (Figure 6).
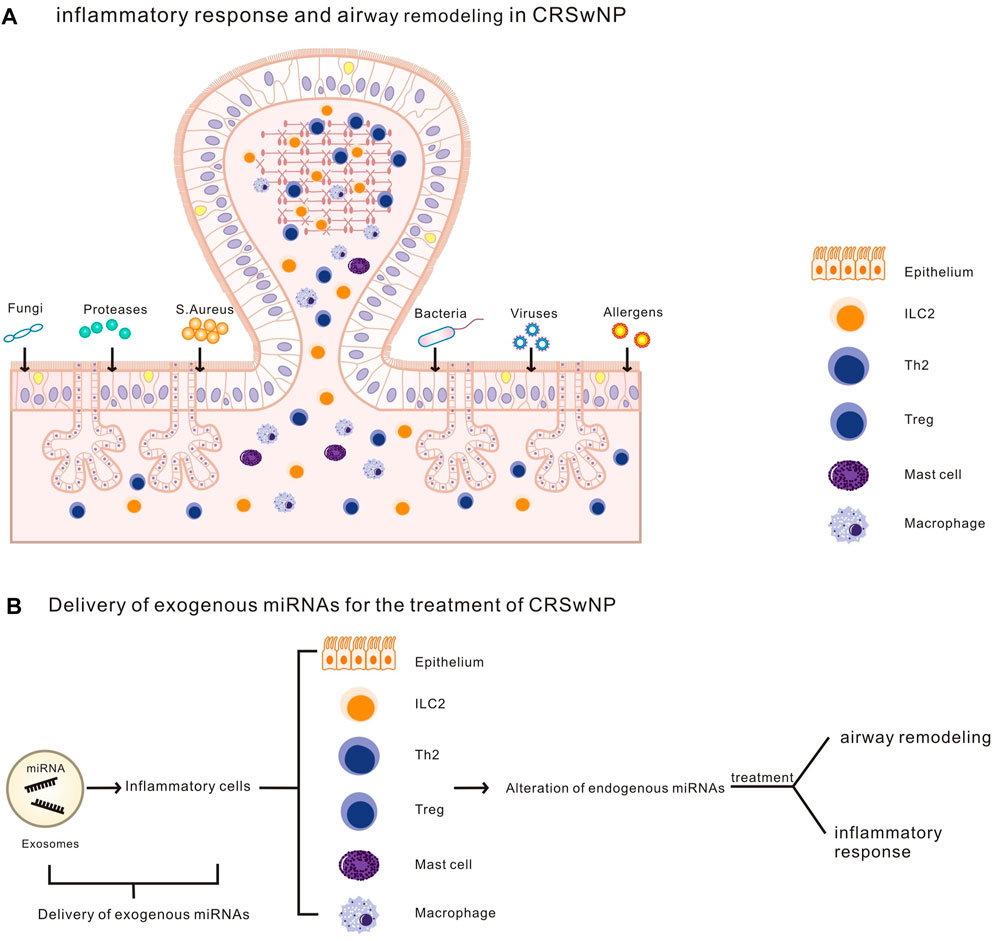
FIGURE 6. (A). Airway remodeling and an inflammatory response in CRSwNP.(B).Exosomes deliver exogenous miRNA to inflammatory cells (including nasal epithelial cells and other CRSwNP-related inflammatory cells), altering cellular endogenous miRNA levels, regulating airway remodeling and inflammatory response in CRSwNP.
Author contributions
SJ and MC conceived the idea of the study; HZ interpreted the results; NZ wrote the paper; all authors discussed the results and revised the manuscript. All authors agree to be accountable for the content of the work.
Funding
This work was supported by grants from the National Natural Science Foundation of China (82071016, 81870701), the Program National Natural Science Foundation of Jilin Provincial (20200201600JC, 20200201411JC, 20200201200JC), Jilin Province Health Science and Technology Talent Project (2019scz004, and 2020scz24).
Conflict of interest
The authors declare that the research was conducted in the absence of any commercial or financial relationships that could be construed as a potential conflict of interest.
Publisher’s note
All claims expressed in this article are solely those of the authors and do not necessarily represent those of their affiliated organizations, or those of the publisher, the editors and the reviewers. Any product that may be evaluated in this article, or claim that may be made by its manufacturer, is not guaranteed or endorsed by the publisher.
References
Cao, P. P., Zhang, Y. N., Liao, B., Ma, J., Wang, B. F., Wang, H., et al. (2014). Increased local IgE production induced by common aeroallergens and phenotypic alteration of mast cells in Chinese eosinophilic, but not non-eosinophilic, chronic rhinosinusitis with nasal polyps. Clin. Exp. Allergy 44 (5), 690–700. doi:10.1111/cea.12304
Cha, D. J., Franklin, J. L., Dou, Y., Liu, Q., Higginbotham, J. N., Demory Beckler, M., et al. (2015). KRAS-dependent sorting of miRNA to exosomes. ELife 4, e07197. doi:10.7554/eLife.07197
Cha, S., Seo, E. H., Lee, S. H., Kim, K. S., Oh, C. S., Moon, J. S., et al. (2021). MicroRNA expression in extracellular vesicles from nasal lavage fluid in chronic rhinosinusitis. Biomedicines 9 (5), 471. doi:10.3390/biomedicines9050471
Chen, H. H., Keyhanian, K., Zhou, X., Vilmundarson, R. O., Almontashiri, N. A., Cruz, S. A., et al. (2015). IRF2BP2 reduces macrophage inflammation and susceptibility to atherosclerosis. Circ. Res. 117 (8), 671–683. doi:10.1161/CIRCRESAHA.114.305777
Chen, J., and Wang, D. Z. (2012). microRNAs in cardiovascular development. J. Mol. Cell. Cardiol. 52 (5), 949–957. doi:10.1016/j.yjmcc.2012.01.012
Fontenot, J. D., Gavin, M. A., and Rudensky, A. Y. (2017). Pillars article: Foxp3 programs the development and function of CD4+CD25+ regulatory T cells. Nat. Immunol. 2003. 4: 330-336. J. Immunol. 198 (3), 986–992.
Fossiez, F., Djossou, O., Chomarat, P., Flores-Romo, L., Ait-Yahia, S., Maat, C., et al. (1996). T cell interleukin-17 induces stromal cells to produce proinflammatory and hematopoietic cytokines. J. Exp. Med. 183 (6), 2593–2603. doi:10.1084/jem.183.6.2593
Fujieda, S., Imoto, Y., Kato, Y., Ninomiya, T., Tokunaga, T., Tsutsumiuchi, T., et al. (2019). Eosinophilic chronic rhinosinusitis. Allergol. Int. 68 (4), 403–412. doi:10.1016/j.alit.2019.07.002
Gao, X., Wan, Z., Wei, M., Dong, Y., Zhao, Y., Chen, X., et al. (2019). Chronic myelogenous leukemia cells remodel the bone marrow niche via exosome-mediated transfer of miR-320. Theranostics 9 (19), 5642–5656. doi:10.7150/thno.34813
Gerasymchuk, M., Cherkasova, V., Kovalchuk, O., and Kovalchuk, I. (2020). The role of microRNAs in organismal and skin aging. Int. J. Mol. Sci. 21 (15), 5281. doi:10.3390/ijms21155281
Gu, W., Zhan, H., Zhou, X. Y., Yao, L., Yan, M., Chen, A., et al. (2017). MicroRNA-22 regulates inflammation and angiogenesis via targeting VE-cadherin. FEBS Lett. 591 (3), 513–526. doi:10.1002/1873-3468.12565
Hamilos, D. L., Leung, D. Y., Wood, R., Cunningham, L., Bean, D. K., Yasruel, Z., et al. (1995). Evidence for distinct cytokine expression in allergic versus nonallergic chronic sinusitis. J. Allergy Clin. Immunol. 96 (4), 537–544. doi:10.1016/s0091-6749(95)70298-9
Jia, Y., Chen, Y., Wang, Q., Jayasinghe, U., Luo, X., Wei, Q., et al. (2017). Exosome: Emerging biomarker in breast cancer. Oncotarget 8 (25), 41717–41733. doi:10.18632/oncotarget.16684
Lässer, C., O'Neil, S. E., Ekerljung, L., Ekström, K., Sjöstrand, M., and Lötvall, J. (2011). RNA-containing exosomes in human nasal secretions. Am. J. Rhinol. Allergy 25 (2), 89–93. doi:10.2500/ajra.2011.25.3573
Lee, H., Li, C., Zhang, Y., Zhang, D., Otterbein, L. E., and Jin, Y. (2019). Caveolin-1 selectively regulates microRNA sorting into microvesicles after noxious stimuli. J. Exp. Med. 216 (9), 2202–2220. doi:10.1084/jem.20182313
Lee, K., Tai, J., Lee, S. H., and Kim, T. H. (2021). Advances in the knowledge of the underlying airway remodeling mechanisms in chronic rhinosinusitis based on the endotypes: A review. Int. J. Mol. Sci. 22 (2), 910. doi:10.3390/ijms22020910
Lee, Y., Kim, M., Han, J., Yeom, K. H., Lee, S., Baek, S. H., et al. (2004). MicroRNA genes are transcribed by RNA polymerase II. Embo J. 23, 4051–4060. doi:10.1038/sj.emboj.7600385
Li, X., Li, C., Zhu, G., Yuan, W., and Xiao, Z. A. (2019). TGF-β1 induces epithelial-mesenchymal transition of chronic sinusitis with nasal polyps through MicroRNA-21. Int. Arch. Allergy Immunol. 179 (4), 304–319. doi:10.1159/000497829
Li, X., Meng, J., Qiao, X., Liu, Y., Liu, F., Zhang, N., et al. (2010). Expression of TGF, matrix metalloproteinases, and tissue inhibitors in Chinese chronic rhinosinusitis. J. Allergy Clin. Immunol. 125 (5), 1061–1068. doi:10.1016/j.jaci.2010.02.023
Li, Y., Wang, W., Ying, S., Lan, F., and Zhang, L. (2021). A potential role of group 2 innate lymphoid cells in eosinophilic chronic rhinosinusitis with nasal polyps. Allergy Asthma Immunol. Res. 13 (3), 363–374. doi:10.4168/aair.2021.13.3.363
Lin, F., Zeng, Z., Song, Y., Li, L., Wu, Z., Zhang, X., et al. (2019). YBX-1 mediated sorting of miR-133 into hypoxia/reoxygenation-induced EPC-derived exosomes to increase fibroblast angiogenesis and MEndoT. Stem Cell Res. Ther. 10 (1), 263. doi:10.1186/s13287-019-1377-8
Liu, R., Du, J., Zhou, J., Zhong, B., Ba, L., Zhang, J., et al. (2021). Elevated microRNA-21 is a brake of inflammation involved in the development of nasal polyps. Front. Immunol. 12, 530488. doi:10.3389/fimmu.2021.530488
Liu, Z., Liu, H., Yu, D., Gao, J., Ruan, B., and Long, R. (2021). Downregulation of miR-29b-3p promotes α-tubulin deacetylation by targeting the interaction of matrix metalloproteinase-9 with integrin β1 in nasal polyps. Int. J. Mol. Med. 48 (1), 126. doi:10.3892/ijmm.2021.4959
Lu, P., Li, H., Li, N., Singh, R. N., Bishop, C. E., Chen, X., et al. (2017). MEX3C interacts with adaptor-related protein complex 2 and involves in miR-451a exosomal sorting. PLoS One 12 (10), e0185992. doi:10.1371/journal.pone.0185992
Luo, X. Q., Shao, J. B., Xie, R. D., Zeng, L., Li, X. X., Qiu, S. Q., et al. (2017). Micro RNA-19a interferes with IL-10 expression in peripheral dendritic cells of patients with nasal polyposis. Oncotarget 8 (30), 48915–48921. doi:10.18632/oncotarget.16555
Omori, M., and Ziegler, S. (2007). Induction of IL-4 expression in CD4(+) T cells by thymic stromal lymphopoietin. J. Immunol. 178 (3), 1396–1404. doi:10.4049/jimmunol.178.3.1396
Patel, N. N., Kohanski, M. A., Maina, I. W., Workman, A. D., Herbert, D. R., and Cohen, N. A. (2019). Sentinels at the wall: Epithelial-derived cytokines serve as triggers of upper airway type 2 inflammation. Int. Forum Allergy Rhinol. 9 (1), 93–99. doi:10.1002/alr.22206
Peng, L., Sang, H., Wei, S., Li, Y., Jin, D., Zhu, X., et al. (2020). circCUL2 regulates gastric cancer malignant transformation and cisplatin resistance by modulating autophagy activation via miR-142-3p/ROCK2. Mol. Cancer 19 (1), 156. doi:10.1186/s12943-020-01270-x
Peterson, S., Poposki, J. A., Nagarkar, D. R., Chustz, R. T., Peters, A. T., Suh, L. A., et al. (2012). Increased expression of CC chemokine ligand 18 in patients with chronic rhinosinusitis with nasal polyps. J. Allergy Clin. Immunol. 129 (1), 119–127. e1-9. doi:10.1016/j.jaci.2011.08.021
Poposki, J. A., Klingler, A. I., Tan, B. K., Soroosh, P., Banie, H., Lewis, G., et al. (2017). Group 2 innate lymphoid cells are elevated and activated in chronic rhinosinusitis with nasal polyps. Immun. Inflamm. Dis. 5 (3), 233–243. doi:10.1002/iid3.161
Poposki, J. A., Uzzaman, A., Nagarkar, D. R., Chustz, R. T., Peters, A. T., Suh, L. A., et al. (2011). Increased expression of the chemokine CCL23 in eosinophilic chronic rhinosinusitis with nasal polyps. J. Allergy Clin. Immunol. 128 (1), 73–81. e4. doi:10.1016/j.jaci.2011.03.017
Punnonen, J., Yssel, H., and de Vries, J. E. (1997). The relative contribution of IL-4 and IL-13 to human IgE synthesis induced by activated CD4+ or CD8+ T cells. J. Allergy Clin. Immunol. 100 (1), 792–801. doi:10.1016/s0091-6749(97)70276-8
Qing, X., Zhang, Y., Peng, Y., He, G., Liu, A., and Liu, H. (2021). Mir-142-3p regulates inflammatory response by contributing to increased TNF-α in chronic rhinosinusitis with nasal polyposis. Ear Nose Throat J. 100 (1), NP50–NP56. doi:10.1177/0145561319847972
Saluja, R., Zoltowska, A., Ketelaar, M. E., and Nilsson, G. (2016). IL-33 and Thymic Stromal Lymphopoietin in mast cell functions. Eur. J. Pharmacol. 778, 68–76. doi:10.1016/j.ejphar.2015.04.047
Santangelo, L., Giurato, G., Cicchini, C., Montaldo, C., Mancone, C., Tarallo, R., et al. (2016). The RNA-binding protein SYNCRIP is a component of the hepatocyte exosomal machinery controlling MicroRNA sorting. Cell Rep. 17 (3), 799–808. doi:10.1016/j.celrep.2016.09.031
Seshadri, S., Lu, X., Purkey, M. R., Homma, T., Choi, A. W., Carter, R., et al. (2015). Increased expression of the epithelial anion transporter pendrin/SLC26A4 in nasal polyps of patients with chronic rhinosinusitis. J. Allergy Clin. Immunol. 136 (6), 1548–1558. e7. doi:10.1016/j.jaci.2015.05.024
Simpson, L. J., Patel, S., Bhakta, N. R., Choy, D. F., Brightbill, H. D., Ren, X., et al. (2014). A microRNA upregulated in asthma airway T cells promotes TH2 cytokine production. Nat. Immunol. 15 (12), 1162–1170. doi:10.1038/ni.3026
Sun, P., Liu, D. Z., Jickling, G. C., Sharp, F. R., and Yin, K. J. (2018). MicroRNA-based therapeutics in central nervous system injuries. J. Cereb. Blood Flow. Metab. 38 (7), 1125–1148. doi:10.1177/0271678X18773871
Takabayashi, T., Kato, A., Peters, A. T., Hulse, K. E., Suh, L. A., Carter, R., et al. (2013). Excessive fibrin deposition in nasal polyps caused by fibrinolytic impairment through reduction of tissue plasminogen activator expression. Am. J. Respir. Crit. Care Med. 187 (1), 49–57. doi:10.1164/rccm.201207-1292OC
Takabayashi, T., Kato, A., Peters, A. T., Hulse, K. E., Suh, L. A., Carter, R., et al. (2013). Increased expression of factor XIII-A in patients with chronic rhinosinusitis with nasal polyps. J. Allergy Clin. Immunol. 132 (3), 584–592. e4. doi:10.1016/j.jaci.2013.02.003
Temoche-Diaz, M. M., Shurtleff, M. J., Nottingham, R. M., Yao, J., Fadadu, R. P., Lambowitz, A. M., et al. (2019). Distinct mechanisms of microRNA sorting into cancer cell-derived extracellular vesicle subtypes. Elife 8, e47544. doi:10.7554/eLife.47544
Thiery, J. P., Acloque, H., Huang, R. Y., and Nieto, M. A. (2009). Epithelial-mesenchymal transitions in development and disease. Cell 139 (5), 871–890. doi:10.1016/j.cell.2009.11.007
van der Ploeg, E. K., Carreras Mascaro, A., Huylebroeck, D., Hendriks, R. W., and Stadhouders, R. (2020). Group 2 innate lymphoid cells in human respiratory disorders. J. Innate Immun. 12 (1), 47–62. doi:10.1159/000496212
Wang, L., Liu, Y., Yu, Z., Gong, J., Deng, Z., Ren, N., et al. (2021). Mir-139-5p inhibits glioma cell proliferation and progression by targeting GABRA1. J. Transl. Med. 19 (1), 213. doi:10.1186/s12967-021-02880-9
Xia, G., Bao, L., Gao, W., Liu, S., Ji, K., and Li, J. (2015). Differentially expressed miRNA in inflammatory mucosa of chronic rhinosinusitis. J. Nanosci. Nanotechnol. 15 (3), 2132–2139. doi:10.1166/jnn.2015.9161
Xuan, L., Luan, G., Wang, Y., Lan, F., Zhang, X., Hao, Y., et al. (2019). MicroRNAs regulating mucin type O-glycan biosynthesis and transforming growth factor β signaling pathways in nasal mucosa of patients with chronic rhinosinusitis with nasal polyps in Northern China. Int. Forum Allergy Rhinol. 9 (1), 106–113. doi:10.1002/alr.22230
Yang, L., Wang, Y., Shi, S., Xie, L., Liu, T., Wang, Y., et al. (2018). The TNF-α-induced expression of miR-130b protects cervical cancer cells from the cytotoxicity of TNF-α. FEBS Open Bio 8 (4), 614–627. doi:10.1002/2211-5463.12395
Yang, N., Cheng, H., Mo, Q., Zhou, X., and Xie, M. (2020). miR-155-5p downregulation inhibits epithelial-to-mesenchymal transition by targeting SIRT1 in human nasal epithelial cells. Mol. Med. Rep. 22 (5), 3695–3704. doi:10.3892/mmr.2020.11468
Yu, J., Kang, X., Xiong, Y., Luo, Q., Dai, D., and Ye, J. (2021). Gene expression profiles of circular RNAs and MicroRNAs in chronic rhinosinusitis with nasal polyps. Front. Mol. Biosci. 8, 643504. doi:10.3389/fmolb.2021.643504
Zhang, W., Zhang, T., Yan, Y., Zhang, J., Zhou, Y., Pei, Y., et al. (2020). Exosomal miR-22-3p derived from chronic rhinosinusitis with nasal polyps regulates vascular permeability by targeting VE-cadherin. Biomed. Res. Int. 2020, 1237678. doi:10.1155/2020/1237678
Zhang, Y., Derycke, L., Holtappels, G., Wang, X. D., Zhang, L., Bachert, C., et al. (2019). Th2 cytokines orchestrate the secretion of MUC5AC and MUC5B in IL-5-positive chronic rhinosinusitis with nasal polyps. Allergy 74 (1), 131–140. doi:10.1111/all.13489
Zheng, Y., Xiong, S., Jiang, P., Liu, R., Liu, X., Qian, J., et al. (2012). Glucocorticoids inhibit lipopolysaccharide-mediated inflammatory response by downregulating microRNA-155: A novel anti-inflammation mechanism. Free Radic. Biol. Med. 52 (8), 1307–1317. doi:10.1016/j.freeradbiomed.2012.01.031
Keywords: microRNA, chronic rhinosinusitis with nasal polyps, RNA binding protein, inflammatory response, airway remodeling, exosomes
Citation: Zhipu N, Zitao H, Jichao S and Cuida M (2022) Research advances in roles of microRNAs in nasal polyp. Front. Genet. 13:1043888. doi: 10.3389/fgene.2022.1043888
Received: 14 September 2022; Accepted: 14 November 2022;
Published: 25 November 2022.
Edited by:
Liqi Shu, Brown University, United StatesReviewed by:
Chenyan Jiang, shanghai ninth people’s hospital, ChinaRunjie Shi, Shanghai Jiao Tong University, China
Copyright © 2022 Zhipu, Zitao, Jichao and Cuida. This is an open-access article distributed under the terms of the Creative Commons Attribution License (CC BY). The use, distribution or reproduction in other forums is permitted, provided the original author(s) and the copyright owner(s) are credited and that the original publication in this journal is cited, in accordance with accepted academic practice. No use, distribution or reproduction is permitted which does not comply with these terms.
*Correspondence: Sha Jichao, shajichao@jlu.edu.cn; Meng Cuida, mengcuida@jlu.edu.cn