- 1Department of Plastic and burns Surgery, The Second Affiliated Hospital of Chengdu Medical College (China National Nuclear Corporation 416 Hospital), Chengdu, China
- 2Department of Cosmetic Plastic and burns Surgery, The First Affiliated Hospital of Chengdu Medical College, Chengdu, China
- 3Department of Orthopedics, The Affiliated Hospital of Yangzhou University, Yangzhou University, Yangzhou, China
- 4Department of Periodontology, Affiliated Stomatological Hospital of Zunyi MedicalUniversity, Zunyi, China
Background: Severe burns and blunt trauma can lead to multiple organ dysfunction syndrome, the leading cause of death in intensive care units. In addition to infection, the degree of immune inflammatory response also affects prognosis. However, the characteristics and clinical relevance of the common mechanisms of these major diseases are still underexplored.
Methods: In the present study, we performed microarray data analysis to identify immune-related differentially expressed genes (DEGs) involved in both disease progression in burns and blunt trauma. Six analyses were subsequently performed, including gene enrichment analysis, protein‐protein interaction (PPI) network construction, immune cell infiltration analysis, core gene identification, co-expression network analysis, and clinical correlation analysis.
Results: A total of 117 common immune-related DEGs was selected for subsequent analyses. Functional analysis emphasizes the important role of Th17 cell differentiation, Th1 and Th2 cell differentiation, Cytokine-cytokine receptor interaction and T cell receptor signaling pathway in these two diseases. Finally, eight core DEGs were identified using cytoHubba, including CD8A, IL10, CCL5, CD28, LCK, CCL4, IL2RB, and STAT1. The correlation analysis showed that the identified core DEGs were more or less significantly associated with simultaneous dysregulation of immune cells in blunt trauma and sepsis patients. Of these, the downregulation of CD8A and CD28 had a worse prognosis.
Conclusion: Our analysis lays the groundwork for future studies to elucidate molecular mechanisms shared in burns and blunt trauma. The functional roles of identified core immune-related DEGs and dysregulated immune cell subsets warrant further in-depth study.
Introduction
Burns caused by physical or chemical factors are characterized by high morbidity and mortality, causing unpredictable and severe injuries to patients. Thermal injuries caused by hot liquids, solids, or fire account for a large proportion of burns injuries (Lee, 1997). According to a 2018 report by the World Health Organization, there are about 11 million burns patients worldwide each year, and although the mortality rate has dropped from the 300,000 recorded in 2011 [(Peck, 2011)], the death toll is still as high as 180,000 [(Pereira et al., 2013)]. The improvement of the survival rate of burns patients is mainly attributed to the improvement of intensive care level, the improvement of wound care, and the effective control of infection (Cioffi et al., 1993; Finnerty et al., 2007). Most patients with severe burns require prompt and specialized burns care to reduce morbidity and mortality. Factors leading to high mortality in severe burns include hypovolemic shock, immunosuppression (Finnerty et al., 2007; Xiao et al., 2011), excessive inflammation (Stanojcic et al., 2018), and hypermetabolic response (Jeschke et al., 2011), and severe burns can also lead to severe infection, sepsis, and multiple organ function disorder syndrome (MODS), which can also lead to death in severely burnsed patients (Martin et al., 2003).
In the early stage of blunt traumatic injury, the skin and mucosal barriers and cell membrane microcarriers are generally destroyed, resulting in the release of various pathogenic factors and the activation of innate immune responses (Lord et al., 2014). These processes can help blunt traumatized patients quickly escape from the dangerous period, but may also cause serious complications disease or even death (Callcut et al., 2016; Gabbe et al., 2017; Mira et al., 2017; Sauaia et al., 2017). After severe blunt trauma in the head, chest, abdomen and other parts, the innate immune system is activated, and a series of complex and heterogeneous multisystem reactions occur (Keel and Trentz, 2005; Adib-Conquy and Cavaillon, 2009; Minei et al., 2012; Cabrera et al., 2017; Dijkink et al., 2018). For example, patients with blunt abdominal blunt trauma who underwent splenectomy had decreased T cell responses to hemagglutinin, decreased lymphocyte numbers, decreased IgM levels, and no significant changes in the expression levels of C3, C4, and C5 [(Downey et al., 1987)]. However, CD46 expression was significantly reduced 48 h after blunt trauma, with or without splenectomy (Amara et al., 2010).
Deaths from severe burns and blunt trauma occur frequently in intensive care units. In addition, severe burns are inherently a special type of trauma. From a physiological point of view, these two diseases are the result of damage to the normal physiological functions of the body due to various external factors. In the course of treatment, the exploration of biomarkers for various types of major disease progression and prognosis is necessary. The purpose of this study to combine the two diseases is to explore the genetic and immune crosstalk between major diseases caused by different etiologies.
In this study, we analyzed the microarray data to identify common functional genes involved in immune regulation under different injury conditions, and initially revealed the underlying molecular mechanism. We found that a variety of immune-related biological functions are dysfunctional after blunt trauma and burns. We then identified common immune-related genes, analyzed the proportion of immune cells, and explored their common relationships. Finally, we evaluated the diagnostic and prognostic value of common immune-related genes to determine their potential research significance and clinical application as novel biomarkers.
Materials and methods
Raw data collection
The gene expression profile of GSE11375 (Warren et al., 2009) and GSE77791 [(Tabone et al., 2018)] were downloaded from the Gene Expression Omnibus (GEO) database (http://www.ncbi.nlm.nih.gov/geo) (Edgar et al., 2002), which is a public database containing a large number of high-throughput sequencing and microarray data sets submitted by research institutes worldwide. The two datasets were based on the GPL570 platform (Affymetrix Human Genome U133 Plus 2.0 Array). The GSE11375 dataset contains 158 adult patients with severe blunt trauma and 26 healthy volunteers. The GSE77791 dataset contains 30 samples from severely burnsed patients with a total burns surface area (TBSA) range from 30 to 98 and 13 samples from healthy volunteers. Inclusion/exclusion criteria, clinical descriptions, and ethics for the severe blunt trauma (Warren et al., 2009) and burns (Venet et al., 2015; Tabone et al., 2018) cohort have been previously published elsewhere. Because this study is a secondary analysis of transcriptome data from previously published public databases, the rationale for its sample size and statistical analysis of baseline data have been confirmed in previous studies.
Data preprocessing and integration
GEO2R (Barrett et al., 2013) (www.ncbi.nlm.nih.gov/geo/ge2r) is an online analysis tool developed based on 2 R packages (GEOquery and Limma). The GEOquery package is used to read data, and the Limma package is used to calculate the differential expression multiple. We used GEO2R to compare gene expression profiles between different groups to determine the DEGs between the diseased group and the control group. Probe sets with no corresponding gene symbols or genes with more than one probe set were removed or averaged, respectively. “p < 0.01 and |logFC| ≥ 1” were defined as the thresholds for the screening of differentially expressed genes (DEGs). A list of immune-related genes was downloaded from the Immunology Database and Analysis Portal database (ImmPort; https://www.immport.org). Finally, Venn diagrams were intersected to obtain common immune-related DEGs for blunt trauma and burns.
Enrichment analyses of immune-related DEGs
In order to better understand the main biological functions of immune-related DEGs for blunt trauma and burns, we analyzed the Gene Ontology (GO) and Kyoto Encyclopedia of Genes and Genomes (KEGG) pathways via KOBAS 3.0 database (Bu et al., 2021), a Web server for gene/protein functional annotation and functional enrichment developed by Peking University, which collects 4,325 species functional annotation information. Adjusted p-value < 0.05 was considered significant.
PPI network construction and analysis of core immune-related DEGs
Search Tool for the Retrieval of Interacting Genes (STRING; http://string-db.org) (version 11.5) (Franceschini et al., 2013) can search for the relationship between proteins of interest, such as direct binding relationships, or coexisting upstream and downstream regulatory pathways, to construct a PPI network with complex regulatory relationships. Interactions with a combined score over 0.4 were considered statistically significant. Cytoscape (http://www.cytoscape.org) (version 3.9.0) (Smoot et al., 2011) was used to visualize this PPI network. The core immune-related DEGs were identified by using the cytoHubba plug-in of Cytoscape. Here, we used six common algorithms (Stress, MNC, Degree, Closeness, Radiality, EPC) to evaluate and select core immune-related DEGs. Subsequently, we constructed a co-expression network of these genes via GeneMANIA (http://www.genemania.org/) (Warde-Farley et al., 2010), which is a reliable tool for identifying internal associations in gene sets.
Immune infiltration analysis
The Immune Cell Abundance Identifier (ImmuCellAI) (Miao et al., 2020) (http://bioinfo.life.hust.edu.cn/ImmuCellAI/#!/) provides comprehensive predictions of immune cell abundance by assessing the abundance of 24 immune cell types in gene expression datasets including RNA-Seq and microarray data, of which 24 The cells consist of 18 T cell subtypes and 6 other immune cells: B cells, NK cells, monocytes, macrophages, neutrophils, and DC cells. The sum of all percentages of 24 infiltrating immune cells was defined as the infiltration score. t-test was used to compare the differential immune cells between the disease group and the control group. Spearman correlation analysis was used to explore the correlation between immune cells and core immune-related DEGs.
Clinical relevance of core immune-related DEGs in blunt trauma and burns
To verify our results, the expression of core immune-related DEGs was extracted from GSE36809 (Xiao et al., 2011) and GSE19743 (Zhou et al., 2010), and the difference between disease group and normal group was analyzed by t test. To verify the diagnostic value of core immune-related DEGs, we performed receiver operating characteristic curve analysis. Since there is survival information for the burns cohort in the GSE19743 dataset, we compared the differences in core immune-related DEGs between the survival and non-survival groups.
Statistical analysis
All statistical analyses were performed using R version 4.0.2. p-value < 0.05 was considered statistically significant. Volcano plots, bubble plots, histograms and ROC plots were drawn by the R package “ggplot2”. Corrplot package was used to draw a correlation heatmap to visualize the correlation of 24 types of infiltrating immune cells.
Results
Identification of immune-related DEGs
The research flowchart of this research was shown in Figure 1. After standardizing the microarray results, 2103 DEGs and 1752 DEGs were identified in GSE11375 and GSE77791 (Figures 2A,B). Through Venn diagram calculation, we obtained 117 overlapping immune-related DEGs in GSE11375, GSE77791 and ImmPort database (Figure 2C and Supplementary Table S1).
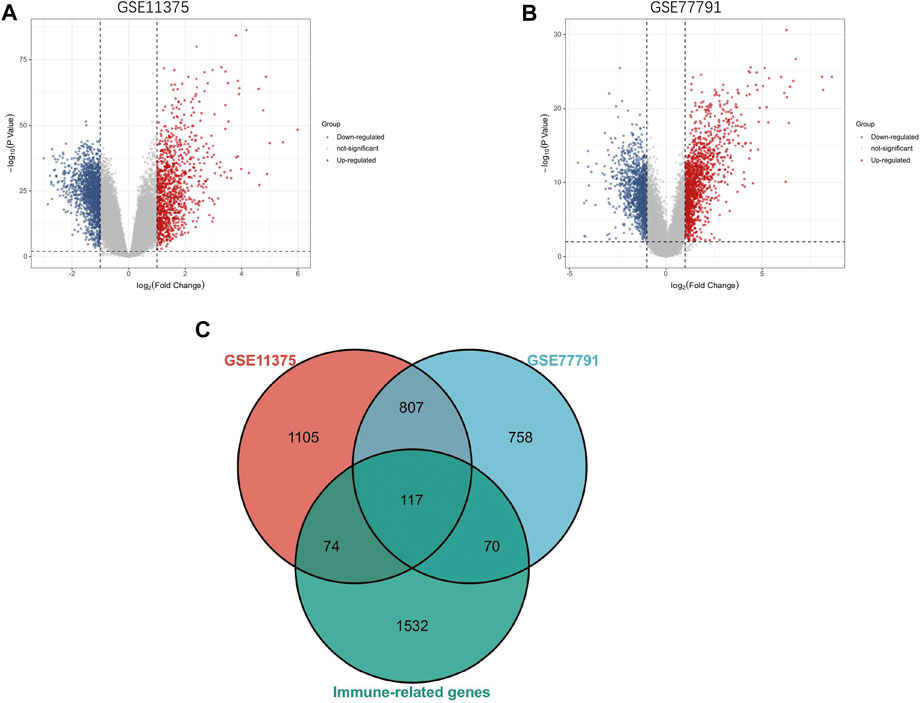
FIGURE 2. (A) The volcano map of GSE11375. (B) The volcano map of GSE77791. (C) Venn diagram show that 117 overlapping immune-related DEGs in GSE11375, GSE77791 and ImmuCellAI database.
Analysis of the functional characteristics
In order to analyze the biological functions and pathways involved in immune-related DEGs, GO and KEGG Pathway enrichment analysis were performed. GO analysis results show that immune-related DEGs were significantly enriched in protein binding, plasma membrane, inflammatory response, immune response and cytokine-mediated signaling pathway (Figure 3A). KEGG pathway analysis showed that the DEGs were mainly concentrated in Th17 cell differentiation, Th1 and Th2 cell differentiation, Cytokine-cytokine receptor interaction, T cell receptor signaling pathway and PD-L1 expression and PD-1 checkpoint pathway in cancer (Figure 3B).
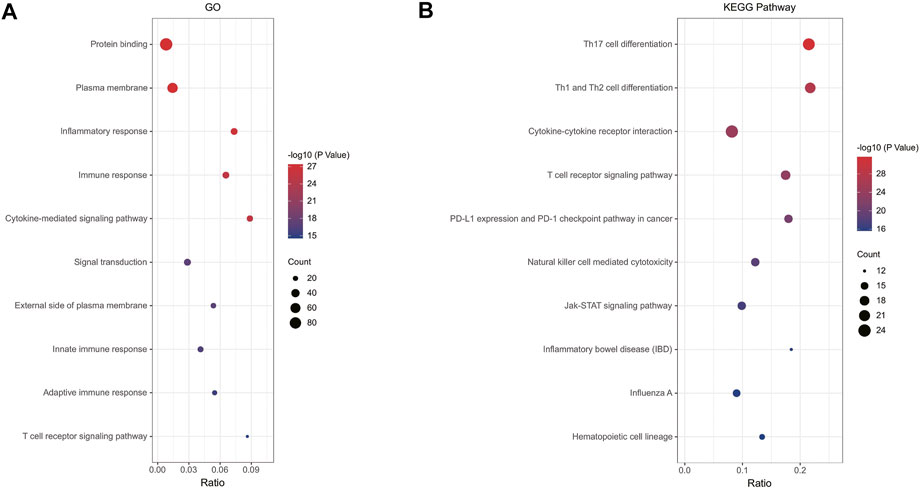
FIGURE 3. (A) Enrichment result of overlapping immune-related DEGs GO term; (B) Enrichment result of overlapping immune-related DEGs KEGG pathway. Adjusted p-value < 0.05 was considered significant.
PPI network construction and analysis of core immune-related DEGs
The PPI network of the immune-related DEGs with combined scores greater than 0.4 was constructed using Cytoscape, which contained 112 nodes and 814 interaction pairs (Figure 4A). Through the six algorithms of plug-in cytoHubba, we have calculated the top 10 core genes (Table 1). After taking the intersection of the Venn diagrams, we found 8 overlapping core genes, including CD8A, IL10, CCL5, CD28, LCK, CCL4, IL2RB, and STAT1 (Figure 4B). Table 2 shows their full names and related functions. Based on the GeneMANIA database, we analyzed the co-expression network and related functions of these genes. These genes showed the complex PPI network with the co-expression of 65.17%, physical interactions of 19.21%, pathway of 7.87%, shared protein domains of 3.03%, co-localization of 2.89% and predicted of 1.82% (Figure 4C). These genes are mainly involved in the activation of leukocyte, lymphocytes, etc.
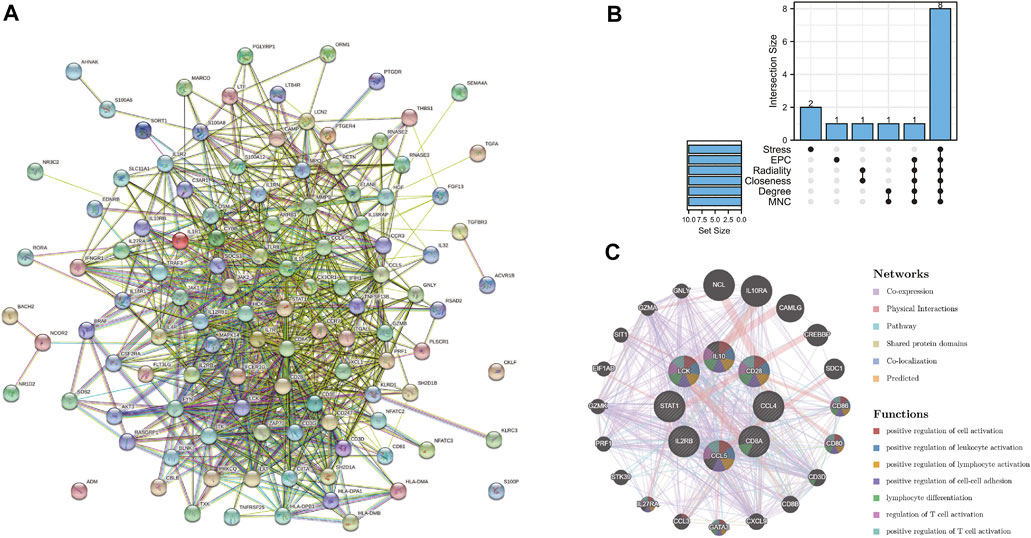
FIGURE 4. (A) PPI network constructed using the STRING database. (B) The Venn diagram showed that six algorithms have screened out 8 core immune-related DEGs. (C) Core immune-related DEGs and their co-expression genes were analyzed via GeneMANIA.
Immune infiltration analysis
After evaluating the immune cell composition of blunt trauma and burns patients, we found significant differences in immune cell profiles between diseased and control groups (Figures 5A–C). In blunt trauma and burns patients, Th2, Th17, Tfh, iTreg, and CD4_T were highly positively correlated (Figures 5B–D). In blunt trauma and burns patients, Monocyte, Macrophage, Neutrophil, and NKT were significantly increased, while NK, CD4_T, CD8_T, Gamma_delta, iTreg, Tfh, Cytotoxic, Exhausted, Central_memory, and Effector_memory were significantly decreased (Figures 6A,B). These results suggest a similar profile of immune cell components in blunt trauma and burns patients. In addition, we explored associations between immune-related DEGs and immune cell components in blunt trauma and burns patients. The results showed that the identified immune-related DEGs were more or less significantly associated with simultaneous dysregulation of immune cells in blunt trauma and sepsis patients (Figures 7A,B). For example, NKT was significantly upregulated in both blunt trauma and burns patients. Meanwhile, 7 of 8 immune-related DEGs (CD8A, IL10, CCL5, CD28, LCK, CCL4, and IL2RB) were significantly negatively correlated with NKT in blunt trauma patients (Figure 7A), and 6 of 8 immune-related DEGs (CD8A, CCL5, CD28, LCK, CCL4, and IL2RB) were significantly negatively correlated with NKT in burns patients (Figure 7B).
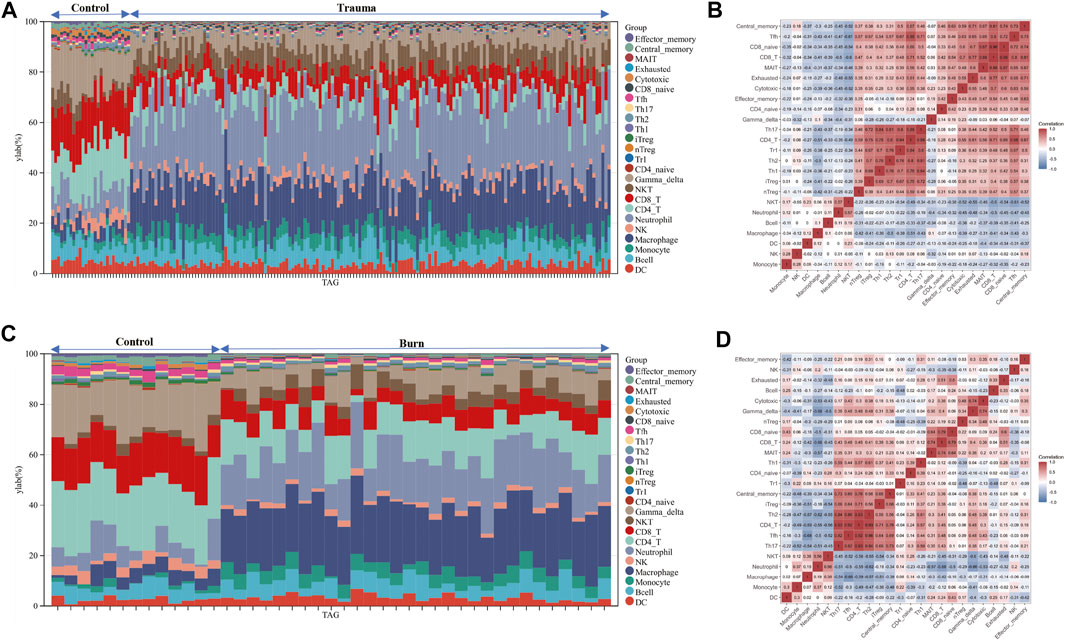
FIGURE 5. (A–C) Stacked bar chart of the immune cell. The different colors of the rectangular bars in the diagram represent different immune cells, and the length represents the proportion of immune cells. (B–D) The correlation matrix of immune cell proportions. The numbers in the squares represent the correlation coefficients between the corresponding immune cells.
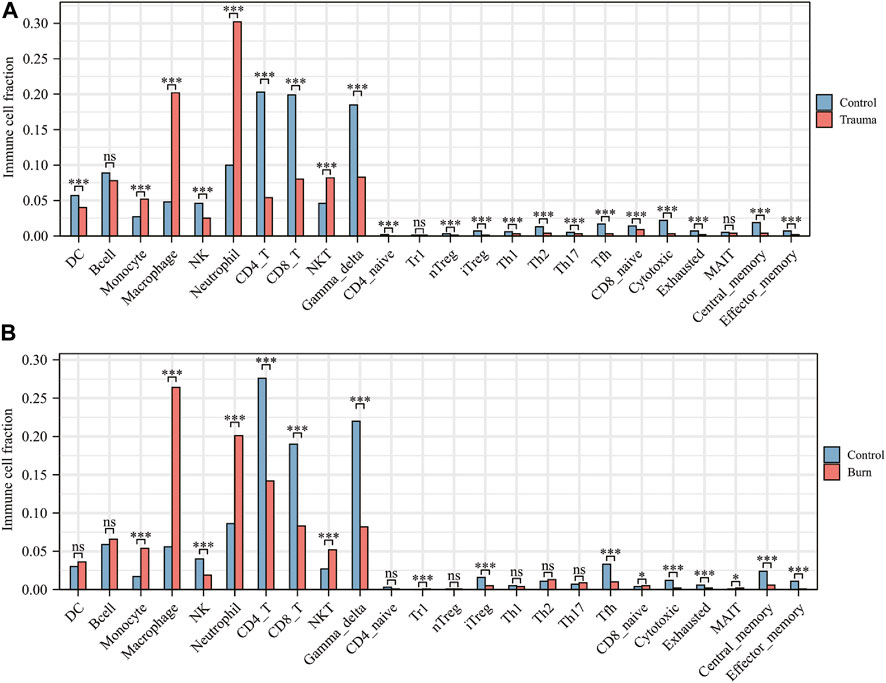
FIGURE 6. (A) Comparison of immune cell fractions between blunt trauma patients and healthy controls. (B) Comparison of immune cell fractions between burns patients and healthy controls.
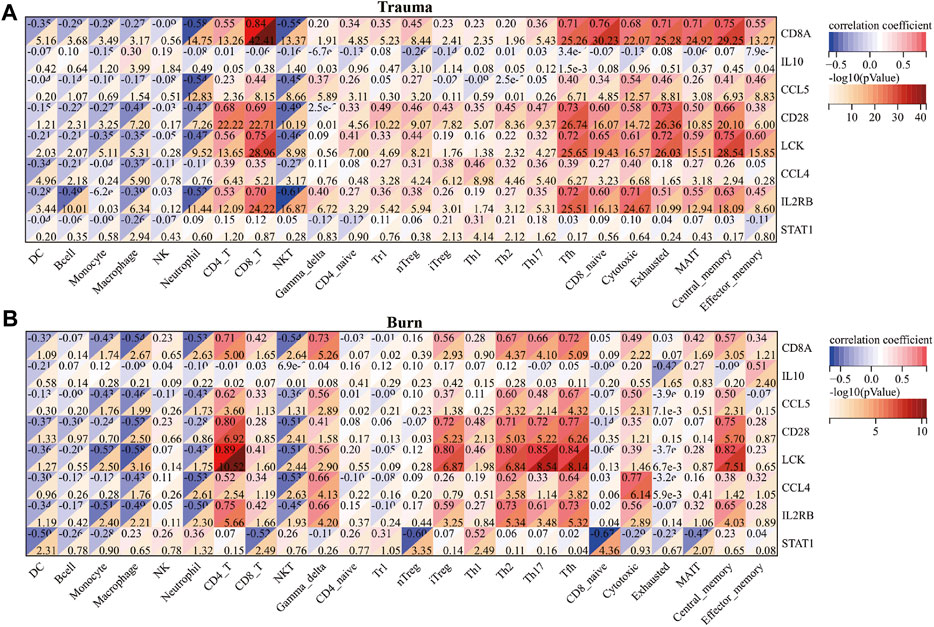
FIGURE 7. (A) Correlations between core immune-related DEGs and immune cell components in blunt trauma patients. (B) Correlation between core immune-related DEGs and immune cell components in burns patients.
Clinical relevance of core immune-related DEGs in blunt trauma and burns
The results of t-test analysis showed that 8 immune-related DEGs conformed to the expression trends in the aforementioned blunt trauma and burns datasets (Figures 8A,B). To verify the diagnostic value of immune-related DEGs, we performed receiver operating characteristic curve analysis. The results showed that these genes have good diagnostic potential for both blunt trauma and burns (Figures 9A,B). Since there is survival information for the burns cohort in the GSE19743 dataset, we compared the differences in core immune-related DEGs between the survival and non-survival groups to explore the prognostic potential of these immune-related DEGs for burns patients. The results showed that the expression of CD8A and CD28 was higher in the survival group, indicating that the downregulation of CD8A and CD28 had a worse prognosis (Figure 9C).
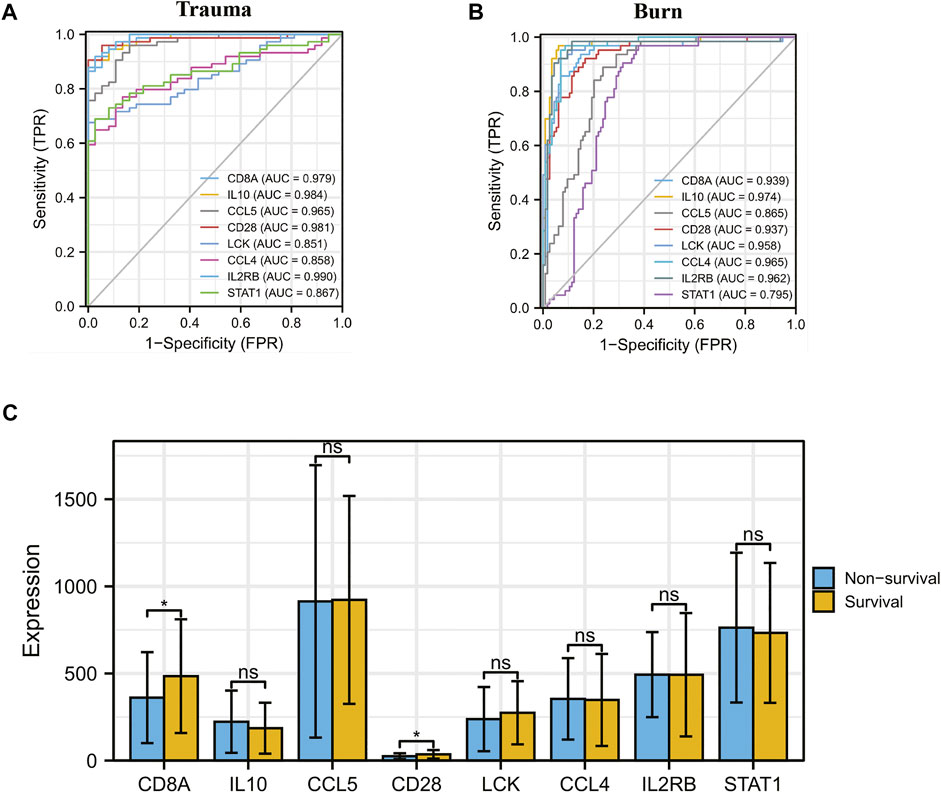
FIGURE 9. (A,B) ROC curve analysis of core immune-related DEGs in blunt trauma and burns. (C) Differences in expression of core immune-related DEGs between survival and non-survival of burns patients.
Discussion
In this study, we identified 2103 DEGs and 1752 DEGs in the datasets GSE11375 and GSE77791, respectively. Through Venn diagram calculations, we obtained 117 overlapping immune-related DEGs in GSE11375, GSE77791, and ImmPort databases. We then performed GO and KEGG pathway enrichment analysis. The GO analysis results showed that immune-related DEGs were significantly enriched in protein binding, plasma membrane, inflammatory response, immune response, and cytokine-mediated signaling pathways. These data all indicate that after the body is blunt traumatized and burnsed, the immune regulation is imbalanced, and a series of biochemical reactions occur.
Existing experimental and clinical studies have shown that a few hours after severe burns can produce an extremely dysregulated host inflammatory response (Xiao et al., 2011; Kallinen et al., 2012; Osuka et al., 2014; Sood et al., 2016; Nielson et al., 2017; Greenhalgh, 2019), mainly including cytokine release, elevated protein levels in acute phase, and hypermetabolic state of the body (Jeschke et al., 2008; Rowan et al., 2015). Studies have also shown that after blunt trauma, in addition to activating the immune system, the coagulation and complement systems are also activated (Ganter et al., 2007; Burk et al., 2012; Ekdahl et al., 2016), thereby preventing bleeding and bacterial invasion. In blunt traumatized patients, complement activation products are deposited on the surface of erythrocytes, inhibiting erythrocyte deformability and inhibiting oxygen delivery (Muroya et al., 2014). Inflammatory mediators such as tissue factor, TNF, and C5a, released by damaged tissues and expressed on leukocytes, act as procoagulant factors (Kambas et al., 2008), promoting the generation of thrombin, which causes endothelial cells to release cytokines that promote cell contraction and expression of adhesion molecules (Aird, 2003). The result of these responses is vascular inflammation, perfusion disturbance and tissue hypoxia, which in turn aggravate the thrombo-inflammatory response (Ekdahl et al., 2016; Kral et al., 2016). Damage-associated pattern molecules (DAMPs) and reactive oxygen species (ROS) produced by blunt trauma also induce endothelial cells to produce adhesion molecules to extravasate into damaged tissues (Sun et al., 2013; Vestweber, 2015).
In addition to hypovolemic shock and hypermetabolic response, burns also have important effects on the immune system (Xiao et al., 2011; Finnerty et al., 2013; Seok et al., 2013; Sood et al., 2016). By comparing the number of immune cells between blunt trauma patients and healthy individuals, it was found that the numbers of monocytes, macrophages, neutrophils, and NKT cells were significantly increased in both blunt trauma and burns patients. In response to burns injury, immune cells, including monocytes, macrophages, and neutrophils, are activated within hours to recognize endogenous factors such as DAMPs, activate downstream NF-κB inflammation-related signaling pathways, and promote inflammatory mediators (IL1, IL6, IL8, IL18, and TNF) release, ultimately leading to the development of systemic inflammatory response syndrome (Singer et al., 2016). In addition, antigen presentation by macrophages or killing of invading pathogens by neutrophils, proliferation of T cells, and inhibition of IL2 production can lead to impaired adaptive immune system and enhanced susceptibility (Baker et al., 1979; Antonacci et al., 1984; Wood et al., 1984; Kupper et al., 1985; Schluter et al., 1991; Messingham et al., 2002; Murphy et al., 2004; Miyazaki et al., 2015; Hampson et al., 2017). In addition to the reduced antigen-presenting function of macrophages, the production of IL12 and IL15 is relatively reduced in the early stages of blunt trauma (Stephan et al., 1987; Ayala et al., 1996; Kawasaki et al., 2006; Kawasaki et al., 2009; Hietbrink et al., 2013). Interestingly, there are also studies reporting that IL4 and IL10 can significantly inhibit the antigen presentation of macrophages and the bactericidal activity of NK cells and neutrophils (Donnelly et al., 1991; Fiorentino et al., 1991; Oswald et al., 1992). Our comparison of the associations between immune-related DEGs and immune cell components in blunt trauma and burns patients also found that immune-related DEGs were more or less associated with simultaneous dysregulation of immune cells in blunt trauma patients, for example, NKT in both blunt trauma and burns patients was significantly up-regulated, while IL10 was negatively correlated with the number of NKTs in blunt trauma patients.
We found significant differences in immune cell profiles between diseased and control groups by assessing the number of immune cells in blunt trauma and burns patients. Compared with the control group, the numbers of Th2 and Th17 cells were significantly increased in blunt trauma and burns patients. In addition to impairing the function of the innate immune system, severe burns reduce the number of T lymphocytes that play a dominant role in the adaptive immune system (Heideman and Bengtsson, 1992; Sheridan et al., 1999). Low expression of IL2 and INF-γ and high expression of IL4 and IL10 increase the number of Th2, while suppressing Th1 activity (Schwacha, 2003; Gosain and Gamelli, 2005). The reduction of the Th1 to Th2 ratio is an important factor in suppressing the adaptive immune response (Church et al., 2006). In addition, the ratio of CD4+ helper T cells to CD8+ suppressor T cells also decreases after severe burns (Burleson et al., 1988). Similarly, burns disrupt the balance between Th17 and regulatory T cells, leading to immune dysregulation. Besides, we have compared the immune cell fraction between the surviving and non-surviving groups for burns. The results showed no significant difference in immune cells (Supplementary Figure S1). We estimate that the reason may be due to the small sample size, with only 8 samples in the non-survival group. However, due to current technical limitations, we cannot distinguish the effects of the cell number and immune-related DEGs expression difference on the immune cell fraction. According to Figure 7B, most of the core immune-related DEGs and Th17 fractions were significantly positively correlated (especially LCK and CD28). We speculate that the expression changes of core immune-related DEGs have a greater impact on immune cell fractions, which further experimental studies are needed to confirm.
In the following t-test analysis, we found that eight immune-related DEGs (CD8A, IL10, CCL5, CD28, LCK, CCL4, IL2RB, and STAT1) conformed to the above-mentioned centralized expression trend of the wound and burns dataset. It shows that after the body is injured, the expression of immune-related DEG changes, which in turn mediates the body’s inflammatory response. After severe blunt trauma, a “genetic storm” and functional rearrangement of leukocytes is activated (Lederer et al., 2008; Xiao et al., 2011), mainly through the release of cytokines (IL10), induction of ROS, and mediation of phagocytosis (Munford and Pugin, 2001; Keel and Trentz, 2005; Itagaki et al., 2015; Timmermans et al., 2016; Hazeldine et al., 2017; Seshadri et al., 2017). Notably, the systemic inflammatory response includes not only multiple immune system activation signatures, but also prominent suppressive signatures that evolve within minutes or hours of blunt trauma (Itagaki et al., 2015; Timmermans et al., 2016; Hazeldine et al., 2017).
To verify the diagnostic value of immune-related DEGs, we performed receiver operating characteristic curve analysis. The results showed that these genes have good diagnostic value for both blunt trauma and burns. The expression of CD8A and CD28 was higher in the survival group, indicating that patients with low expression of CD8A and CD28 had poor prognosis. CD28 is an indispensable co-stimulatory molecule for the activation of T cells, which is crucial for the activation of CD8+ CTL [(Schwartz, 1992)]. Current studies have shown that CD28 has important effects on T cell function, including transcriptional regulation, post-translational modification, and remodeling of the actin cytoskeleton (Esensten et al., 2016; Zumerle et al., 2017; Porciello et al., 2018), which in turn participates in the regulation of T cell physiological functions, such as the regulation of proliferation signals. Activation, activation of telomerase, and enhancement of T cell migration and homing (Bour-Jordan et al., 2011; Esensten et al., 2016; Zhang and Vignali, 2016). Therefore, downregulation of CD28 is a hallmark of CD8+ T cell senescence, and CD28− T cells exhibit marked immunosuppression (Esensten et al., 2016). Importantly, the key regulatory role of CD28 in inducing immune responses has been preliminarily confirmed in animal experiments. After mice were given CD28 antagonists, it was found that CD28 antagonists were able to induce antigen-specific tolerance and prevent autoimmune diseases and organs. The progression of transplant rejection (Lenschow et al., 1992). These results indicate that CD28 is likely to be a promising target for regulating the body’s immune response.
We should acknowledge some limitations of this study. The data of this study are all from public databases and lack the support of clinical data. But based on future trends toward rapid point-of-care testing with limited hands-on time, without the need for specialized laboratories, and the development of statistical methods to analyze gene expression data over time to help address these questions, our study provides some potential targets. Finally, we propose further studies on the regulatory mechanisms and functional roles of dysregulated immune-related DEGs and immune cells to elucidate the temporal association of dysregulated immune-related DEGs and immune cells with MODS in severe disease.
Conclusion
In conclusion, through a comprehensive analysis of microarray data, our study found that the host immune response is altered after burns and blunt trauma, which may be mediated by specific core immune-related DEGs and immune cells. This study provides potential research targets and directions for further research on the occurrence and development of immune regulation after burns and blunt trauma.
Data availability statement
The datasets presented in this study can be found in online repositories. The names of the repository/repositories and accession number(s) can be found in the article/Supplementary Material.
Author contributions
WS and DY: conceptualization. XC, DL, and KW: data curation. WS, KW, BH, and XC: formal analysis and methodology. DY, XC, and BH: supervision. MZ: collect literature. WS, XC, and DL: writing (review and editing). The final manuscript read and approved by all authors. All authors contributed to the article and approved the submitted version.
Funding
This work was supported by the National Natural Science Foundation of China (32071238), the Fundamental Research Funds for the Central Universities and Young Talent Program of China National Nuclear Corporation (CNNC2021136), Natural Science Project of Chengdu Medical College (CYZYB21-07) and Medical Research Project of Chengdu 2021 (2021085).
Conflict of interest
The authors declare that the research was conducted in the absence of any commercial or financial relationships that could be construed as a potential conflict of interest.
Publisher’s note
All claims expressed in this article are solely those of the authors and do not necessarily represent those of their affiliated organizations, or those of the publisher, the editors and the reviewers. Any product that may be evaluated in this article, or claim that may be made by its manufacturer, is not guaranteed or endorsed by the publisher.
Supplementary material
The Supplementary Material for this article can be found online at: https://www.frontiersin.org/articles/10.3389/fgene.2022.1038222/full#supplementary-material
Abbreviations
DEGs, differentially expressed genes; PPI, protein‐protein interaction; MODS, organ function disorder syndrome; GEO, Gene Expression Omnibus; TBSA, total burns surface area; GO, Gene ontology; KEGG, Kyoto Encyclopedia of Genes and Genomes; ImmuCellAI, The Immune Cell Abundance Identifier; DAMPs, damage-associated pattern molecules; ROS, reactive oxygen species.
References
Adib-Conquy, M., and Cavaillon, J. M. (2009). Compensatory anti-inflammatory response syndrome. Thromb. Haemost. 101 (1), 36–47. doi:10.1160/th08-07-0421
Aird, W. C. (2003). The role of the endothelium in severe sepsis and multiple organ dysfunction syndrome. Blood 101 (10), 3765–3777. doi:10.1182/blood-2002-06-1887
Amara, U., Kalbitz, M., Perl, M., Flierl, M. A., Rittirsch, D., Weiss, M., et al. (2010). Early expression changes of complement regulatory proteins and C5A receptor (CD88) on leukocytes after multiple injury in humans. Shock 33 (6), 568–575. doi:10.1097/SHK.0b013e3181c799d4
Antonacci, A. C., Calvano, S. E., Reaves, L. E., Prajapati, A., Bockman, R., Welte, K., et al. (1984). Autologous and allogeneic mixed-lymphocyte responses following thermal injury in man: The immunomodulatory effects of interleukin 1, interleukin 2, and a prostaglandin inhibitor, WY-18251. Clin. Immunol. Immunopathol. 30 (2), 304–320. doi:10.1016/0090-1229(84)90064-3
Ayala, A., Ertel, W., and Chaudry, I. H. (1996). Trauma-induced suppression of antigen presentation and expression of major histocompatibility class II antigen complex in leukocytes. Shock 5 (2), 79–90. doi:10.1097/00024382-199602000-00001
Baker, C. C., Miller, C. L., and Trunkey, D. D. (1979). Predicting fatal sepsis in burn patients. J. Trauma 19 (9), 641–648. doi:10.1097/00005373-197909000-00001
Barrett, T., Wilhite, S. E., Ledoux, P., Evangelista, C., Kim, I. F., Tomashevsky, M., et al. (2013). NCBI GEO: Archive for functional genomics data sets-update. Nucleic Acids Res. 41, D991–D995. doi:10.1093/nar/gks1193
Bour-Jordan, H., Esensten, J. H., Martinez-Llordella, M., Penaranda, C., Stumpf, M., and Bluestone, J. A. (2011). Intrinsic and extrinsic control of peripheral T-cell tolerance by costimulatory molecules of the CD28/B7 family. Immunol. Rev. 241 (1), 180–205. doi:10.1111/j.1600-065X.2011.01011.x
Bu, D., Luo, H., Huo, P., Wang, Z., Zhang, S., He, Z., et al. (2021). KOBAS-I: Intelligent prioritization and exploratory visualization of biological functions for gene enrichment analysis. Nucleic Acids Res. 49, W317–W325. doi:10.1093/nar/gkab447
Burk, A. M., Martin, M., Flierl, M. A., Rittirsch, D., Helm, M., Lampl, L., et al. (2012). Early complementopathy after multiple injuries in humans. Shock 37 (4), 348–354. doi:10.1097/SHK.0b013e3182471795
Burleson, D. G., Mason, A. D., and Pruitt, B. A. (1988). Lymphoid subpopulation changes after thermal injury and thermal injury with infection in an experimental model. Ann. Surg. 207 (2), 208–212. doi:10.1097/00000658-198802000-00016
Cabrera, C. P., Manson, J., Shepherd, J. M., Torrance, H. D., Watson, D., Longhi, M. P., et al. (2017). Signatures of inflammation and impending multiple organ dysfunction in the hyperacute phase of trauma: A prospective cohort study. PLoS Med. 14 (7), e1002352. doi:10.1371/journal.pmed.1002352
Callcut, R. A., Wakam, G., Conroy, A. S., Kornblith, L. Z., Howard, B. M., Campion, E. M., et al. (2016). Discovering the truth about life after discharge: Long-term trauma-related mortality. J. Trauma Acute Care Surg. 80 (2), 210–217. doi:10.1097/TA.0000000000000930
Church, D., Elsayed, S., Reid, O., Winston, B., and Lindsay, R. (2006). Burn wound infections. Clin. Microbiol. Rev. 19 (2), 403–434. doi:10.1128/CMR.19.2.403-434.2006
Cioffi, W. G., deLemos, R. A., Coalson, J. J., Gerstmann, D. A., and Pruitt, B. A. (1993). Decreased pulmonary damage in primates with inhalation injury treated with high-frequency ventilation. Ann. Surg. 218 (3), 328–335. doi:10.1097/00000658-199309000-00012
Dijkink, S., van der Wilden, G. M., Krijnen, P., Dol, L., Rhemrev, S., King, D. R., et al. (2018). Polytrauma patients in The Netherlands and the USA: A bi-institutional comparison of processes and outcomes of care. Injury 49 (1), 104–109. doi:10.1016/j.injury.2017.10.021
Donnelly, R. P., Fenton, M. J., Kaufman, J. D., and Gerrard, T. L. (1991). IL-1 expression in human monocytes is transcriptionally and posttranscriptionally regulated by IL-4. J. Immunol. 146 (10), 3431–3436.
Downey, E. C., Shackford, S. R., Fridlund, P. H., and Ninnemann, J. L. (1987). Long-term depressed immune function in patients splenectomized for trauma. J. Trauma 27 (6), 661–663. doi:10.1097/00005373-198706000-00010
Edgar, R., Domrachev, M., and Lash, A. E. (2002). Gene expression Omnibus: NCBI gene expression and hybridization array data repository. Nucleic Acids Res. 30 (1), 207–210. doi:10.1093/nar/30.1.207
Ekdahl, K. N., Teramura, Y., Hamad, O. A., Asif, S., Duehrkop, C., Fromell, K., et al. (2016). Dangerous liaisons: Complement, coagulation, and kallikrein/kinin cross-talk act as a linchpin in the events leading to thromboinflammation. Immunol. Rev. 274 (1), 245–269. doi:10.1111/imr.12471
Esensten, J. H., Helou, Y. A., Chopra, G., Weiss, A., and Bluestone, J. A. (2016). CD28 costimulation: From mechanism to therapy. Immunity 44 (5), 973–988. doi:10.1016/j.immuni.2016.04.020
Finnerty, C. C., Herndon, D. N., and Jeschke, M. G. (2007). Inhalation injury in severely burned children does not augment the systemic inflammatory response. Crit. Care 11 (1), R22. doi:10.1186/cc5698
Finnerty, C. C., Jeschke, M. G., Qian, W. J., Kaushal, A., Xiao, W., Liu, T., et al. (2013). Determination of burn patient outcome by large-scale quantitative discovery proteomics. Crit. Care Med. 41 (6), 1421–1434. doi:10.1097/CCM.0b013e31827c072e
Fiorentino, D. F., Zlotnik, A., Mosmann, T. R., Howard, M., and O'Garra, A. (1991). IL-10 inhibits cytokine production by activated macrophages. J. Immunol. 147 (11), 3815–3822.
Franceschini, A., Szklarczyk, D., Frankild, S., Kuhn, M., Simonovic, M., Roth, A., et al. (2013). STRING v9.1: Protein-protein interaction networks, with increased coverage and integration. Nucleic Acids Res. 41, D808–D815. doi:10.1093/nar/gks1094
Gabbe, B. J., Simpson, P. M., Cameron, P. A., Ponsford, J., Lyons, R. A., Collie, A., et al. (2017). Long-term health status and trajectories of seriously injured patients: A population-based longitudinal study. PLoS Med. 14 (7), e1002322. doi:10.1371/journal.pmed.1002322
Ganter, M. T., Brohi, K., Cohen, M. J., Shaffer, L. A., Walsh, M. C., Stahl, G. L., et al. (2007). Role of the alternative pathway in the early complement activation following major trauma. Shock 28 (1), 29–34. doi:10.1097/shk.0b013e3180342439
Gosain, A., and Gamelli, R. L. (2005). A primer in cytokines. J. Burn Care Rehabil. 26 (1), 7–12. doi:10.1097/01.bcr.0000150214.72984.44
Greenhalgh, D. G. (2019). Management of burns. N. Engl. J. Med. 380 (24), 2349–2359. doi:10.1056/NEJMra1807442
Hampson, P., Dinsdale, R. J., Wearn, C. M., Bamford, A. L., Bishop, J. R. B., Hazeldine, J., et al. (2017). Neutrophil dysfunction, immature granulocytes, and cell-free dna are early biomarkers of sepsis in burn-injured patients: A prospective observational cohort study. Ann. Surg. 265 (6), 1241–1249. doi:10.1097/SLA.0000000000001807
Hazeldine, J., Naumann, D. N., Toman, E., Davies, D., Bishop, J. R. B., Su, Z., et al. (2017). Prehospital immune responses and development of multiple organ dysfunction syndrome following traumatic injury: A prospective cohort study. PLoS Med. 14 (7), e1002338. doi:10.1371/journal.pmed.1002338
Heideman, M., and Bengtsson, A. (1992). The immunologic response to thermal injury. World J. Surg. 16 (1), 53–56. doi:10.1007/BF02067115
Hietbrink, F., Koenderman, L., Althuizen, M., Pillay, J., Kamp, V., and Leenen, L. P. (2013). Kinetics of the innate immune response after trauma: Implications for the development of late onset sepsis. Shock 40 (1), 21–27. doi:10.1097/SHK.0b013e318295a40a
Itagaki, K., Kaczmarek, E., Lee, Y. T., Tang, I. T., Isal, B., Adibnia, Y., et al. (2015). Mitochondrial DNA released by trauma induces neutrophil extracellular traps. PLoS One 10 (3), e0120549. doi:10.1371/journal.pone.0120549
Jeschke, M. G., Chinkes, D. L., Finnerty, C. C., Kulp, G., Suman, O. E., Norbury, W. B., et al. (2008). Pathophysiologic response to severe burn injury. Ann. Surg. 248 (3), 387–401. doi:10.1097/SLA.0b013e3181856241
Jeschke, M. G., Gauglitz, G. G., Kulp, G. A., Finnerty, C. C., Williams, F. N., Kraft, R., et al. (2011). Long-term persistance of the pathophysiologic response to severe burn injury. PLoS One 6 (7), e21245. doi:10.1371/journal.pone.0021245
Kallinen, O., Maisniemi, K., Bohling, T., Tukiainen, E., and Koljonen, V. (2012). Multiple organ failure as a cause of death in patients with severe burns. J. Burn Care Res. 33 (2), 206–211. doi:10.1097/BCR.0b013e3182331e73
Kambas, K., Markiewski, M. M., Pneumatikos, I. A., Rafail, S. S., Theodorou, V., Konstantonis, D., et al. (2008). C5a and TNF-alpha up-regulate the expression of tissue factor in intra-alveolar neutrophils of patients with the acute respiratory distress syndrome. J. Immunol. 180 (11), 7368–7375. doi:10.4049/jimmunol.180.11.7368
Kawasaki, T., Choudhry, M. A., Schwacha, M. G., Bland, K. I., and Chaudry, I. H. (2009). Effect of interleukin-15 on depressed splenic dendritic cell functions following trauma-hemorrhage. Am. J. Physiol. Cell Physiol. 296 (1), C124–C130. doi:10.1152/ajpcell.00447.2008
Kawasaki, T., Fujimi, S., Lederer, J. A., Hubbard, W. J., Choudhry, M. A., Schwacha, M. G., et al. (2006). Trauma-hemorrhage induces depressed splenic dendritic cell functions in mice. J. Immunol. 177 (7), 4514–4520. doi:10.4049/jimmunol.177.7.4514
Keel, M., and Trentz, O. (2005). Pathophysiology of polytrauma. Injury 36 (6), 691–709. doi:10.1016/j.injury.2004.12.037
Kral, J. B., Schrottmaier, W. C., Salzmann, M., and Assinger, A. (2016). Platelet interaction with innate immune cells. Transfus. Med. Hemother. 43 (2), 78–88. doi:10.1159/000444807
Kupper, T. S., Green, D. R., Durum, S. K., and Baker, C. C. (1985). Defective antigen presentation to a cloned T helper cell by macrophages from burned mice can be restored with interleukin-1. Surgery 98 (2), 199–206.
Lederer, J. A., Brownstein, B. H., Lopez, M. C., Macmillan, S., Delisle, A. J., Macconmara, M. P., et al. (2008). Comparison of longitudinal leukocyte gene expression after burn injury or trauma-hemorrhage in mice. Physiol. Genomics 32 (3), 299–310. doi:10.1152/physiolgenomics.00086.2007
Lee, R. C. (1997). Injury by electrical forces: Pathophysiology, manifestations, and therapy. Curr. Probl. Surg. 34 (9), 677–764. doi:10.1016/s0011-3840(97)80007-x
Lenschow, D. J., Zeng, Y., Thistlethwaite, J. R., Montag, A., Brady, W., Gibson, M. G., et al. (1992). Long-term survival of xenogeneic pancreatic islet grafts induced by CTLA4lg. Science 257 (5071), 789–792. doi:10.1126/science.1323143
Lord, J. M., Midwinter, M. J., Chen, Y. F., Belli, A., Brohi, K., Kovacs, E. J., et al. (2014). The systemic immune response to trauma: An overview of pathophysiology and treatment. Lancet 384 (9952), 1455–1465. doi:10.1016/S0140-6736(14)60687-5
Martin, G. S., Mannino, D. M., Eaton, S., and Moss, M. (2003). The epidemiology of sepsis in the United States from 1979 through 2000. N. Engl. J. Med. 348 (16), 1546–1554. doi:10.1056/NEJMoa022139
Messingham, K. A., Faunce, D. E., and Kovacs, E. J. (2002). Alcohol, injury, and cellular immunity. Alcohol 28 (3), 137–149. doi:10.1016/s0741-8329(02)00278-1
Miao, Y., Zhang, Q., Lei, Q., Luo, M., Xie, G., Wang, H., et al. (2020). ImmuCellAI: A unique method for comprehensive T-cell subsets abundance prediction and its application in cancer immunotherapy. Adv. Sci. 7 (7), 1902880. doi:10.1002/advs.201902880
Minei, J. P., Cuschieri, J., Sperry, J., Moore, E. E., West, M. A., Harbrecht, B. G., et al. (2012). The changing pattern and implications of multiple organ failure after blunt injury with hemorrhagic shock. Crit. Care Med. 40 (4), 1129–1135. doi:10.1097/CCM.0b013e3182376e9f
Mira, J. C., Cuschieri, J., Ozrazgat-Baslanti, T., Wang, Z., Ghita, G. L., Loftus, T. J., et al. (2017). The epidemiology of chronic critical illness after severe traumatic injury at two level-one trauma centers. Crit. Care Med. 45 (12), 1989–1996. doi:10.1097/CCM.0000000000002697
Miyazaki, H., Kinoshita, M., Ono, S., Seki, S., and Saitoh, D. (2015). Burn-evoked reactive oxygen species immediately after injury are crucial to restore the neutrophil function against postburn infection in mice. Shock 44 (3), 252–257. doi:10.1097/SHK.0000000000000404
Munford, R. S., and Pugin, J. (2001). Normal responses to injury prevent systemic inflammation and can be immunosuppressive. Am. J. Respir. Crit. Care Med. 163 (2), 316–321. doi:10.1164/ajrccm.163.2.2007102
Muroya, T., Kannan, L., Ghiran, I. C., Shevkoplyas, S. S., Paz, Z., Tsokos, M., et al. (2014). C4d deposits on the surface of RBCs in trauma patients and interferes with their function. Crit. Care Med. 42 (5), e364–e372. doi:10.1097/CCM.0000000000000231
Murphy, T. J., Paterson, H. M., Mannick, J. A., and Lederer, J. A. (2004). Injury, sepsis, and the regulation of Toll-like receptor responses. J. Leukoc. Biol. 75 (3), 400–407. doi:10.1189/jlb.0503233
Nielson, C. B., Duethman, N. C., Howard, J. M., Moncure, M., and burns, Wood J. G. (2017). Burns: Pathophysiology of systemic complications and current management. J. Burn Care Res. 38 (1), e469–e481. doi:10.1097/BCR.0000000000000355
Osuka, A., Ogura, H., Ueyama, M., Shimazu, T., and Lederer, J. A. (2014). Immune response to traumatic injury: Harmony and discordance of immune system homeostasis. Acute Med. Surg. 1 (2), 63–69. doi:10.1002/ams2.17
Oswald, I. P., Wynn, T. A., Sher, A., and James, S. L. (1992). Interleukin 10 inhibits macrophage microbicidal activity by blocking the endogenous production of tumor necrosis factor alpha required as a costimulatory factor for interferon gamma-induced activation. Proc. Natl. Acad. Sci. U. S. A. 89 (18), 8676–8680. doi:10.1073/pnas.89.18.8676
Peck, M. D. (2011). Epidemiology of burns throughout the world. Part I: Distribution and risk factors. burns 37 (7), 1087–1100. doi:10.1016/j.burns.2011.06.005
Pereira, R. F., Barrias, C. C., Granja, P. L., and Bartolo, P. J. (2013). Advanced biofabrication strategies for skin regeneration and repair. Nanomedicine (Lond) 8 (4), 603–621. doi:10.2217/nnm.13.50
Porciello, N., Grazioli, P., Campese, A. F., Kunkl, M., Caristi, S., Mastrogiovanni, M., et al. (2018). A non-conserved amino acid variant regulates differential signalling between human and mouse CD28. Nat. Commun. 9 (1), 1080. doi:10.1038/s41467-018-03385-8
Rowan, M. P., Cancio, L. C., Elster, E. A., Burmeister, D. M., Rose, L. F., Natesan, S., et al. (2015). Burn wound healing and treatment: Review and advancements. Crit. Care 19, 243. doi:10.1186/s13054-015-0961-2
Sauaia, A., Moore, F. A., and Moore, E. E. (2017). Postinjury inflammation and organ dysfunction. Crit. Care Clin. 33 (1), 167–191. doi:10.1016/j.ccc.2016.08.006
Schluter, B., Konig, W., Koller, M., Erbs, G., and Muller, F. E. (1991). Differential regulation of T- and B-lymphocyte activation in severely burned patients. J. Trauma Inj. Infect. Crit. Care 31 (2), 239–246. doi:10.1097/00005373-199102000-00015
Schwacha, M. G. (2003). Macrophages and post-burn immune dysfunction. burns 29 (1), 1–14. doi:10.1016/s0305-4179(02)00187-0
Schwartz, R. H. (1992). Costimulation of T lymphocytes: The role of CD28, CTLA-4, and B7/BB1 in interleukin-2 production and immunotherapy. Cell 71 (7), 1065–1068. doi:10.1016/s0092-8674(05)80055-8
Seok, J., Warren, H. S., Cuenca, A. G., Mindrinos, M. N., Baker, H. V., Xu, W., et al. (2013). Genomic responses in mouse models poorly mimic human inflammatory diseases. Proc. Natl. Acad. Sci. U. S. A. 110 (9), 3507–3512. doi:10.1073/pnas.1222878110
Seshadri, A., Brat, G. A., Yorkgitis, B. K., Keegan, J., Dolan, J., Salim, A., et al. (2017). Phenotyping the immune response to trauma: A multiparametric systems Immunology approach. Crit. Care Med. 45 (9), 1523–1530. doi:10.1097/CCM.0000000000002577
Sheridan, R. L., Weber, J. M., Pasternak, M. M., Mulligan, J. M., and Tompkins, R. G. (1999). A 15-year experience with varicella infections in a pediatric burn unit. burns 25 (4), 353–356. doi:10.1016/s0305-4179(99)00003-0
Singer, M., Deutschman, C. S., Seymour, C. W., Shankar-Hari, M., Annane, D., Bauer, M., et al. (2016). The third international consensus definitions for sepsis and septic shock (Sepsis-3). JAMA 315 (8), 801–810. doi:10.1001/jama.2016.0287
Smoot, M. E., Ono, K., Ruscheinski, J., Wang, P. L., and Ideker, T. (2011). Cytoscape 2.8: New features for data integration and network visualization. Bioinforma. Oxf. Engl. 27 (3), 431–432. doi:10.1093/bioinformatics/btq675
Sood, R. F., Gibran, N. S., Arnoldo, B. D., Gamelli, R. L., Herndon, D. N., Tompkins, R. G., et al. (2016). Early leukocyte gene expression associated with age, burn size, and inhalation injury in severely burned adults. J. Trauma Acute Care Surg. 80 (2), 250–257. doi:10.1097/TA.0000000000000905
Stanojcic, M., Abdullahi, A., Rehou, S., Parousis, A., and Jeschke, M. G. (2018). Pathophysiological response to burn injury in adults. Ann. Surg. 267 (3), 576–584. doi:10.1097/SLA.0000000000002097
Stephan, R. N., Saizawa, M., Conrad, P. J., Dean, R. E., Geha, A. S., and Chaudry, I. H. (1987). Depressed antigen presentation function and membrane interleukin-1 activity of peritoneal macrophages after laparotomy. Surgery 102 (2), 147–154.
Sun, S., Sursal, T., Adibnia, Y., Zhao, C., Zheng, Y., Li, H., et al. (2013). Mitochondrial DAMPs increase endothelial permeability through neutrophil dependent and independent pathways. PLoS One 8 (3), e59989. doi:10.1371/journal.pone.0059989
Tabone, O., Mommert, M., Jourdan, C., Cerrato, E., Legrand, M., Lepape, A., et al. (2018). Endogenous retroviruses transcriptional modulation after severe infection, trauma and burn. Front. Immunol. 9, 3091. doi:10.3389/fimmu.2018.03091
Timmermans, K., Kox, M., Vaneker, M., van den Berg, M., John, A., van Laarhoven, A., et al. (2016). Plasma levels of danger-associated molecular patterns are associated with immune suppression in trauma patients. Intensive Care Med. 42 (4), 551–561. doi:10.1007/s00134-015-4205-3
Venet, F., Plassais, J., Textoris, J., Cazalis, M., Pachot, A., Bertin-Maghit, M., et al. (2015). Low-dose hydrocortisone reduces norepinephrine duration in severe burn patients: A randomized clinical trial. Crit. Care 19, 21. doi:10.1186/s13054-015-0740-0
Vestweber, D. (2015). How leukocytes cross the vascular endothelium. Nat. Rev. Immunol. 15 (11), 692–704. doi:10.1038/nri3908
Warde-Farley, D., Donaldson, S. L., Comes, O., Zuberi, K., Badrawi, R., Chao, P., et al. (2010). The GeneMANIA prediction server: Biological network integration for gene prioritization and predicting gene function. Nucleic Acids Res. 38, W214–W220. doi:10.1093/nar/gkq537
Warren, H., Elson, C., Hayden, D., Schoenfeld, D., Cobb, J., Maier, R., et al. (2009). A genomic score prognostic of outcome in trauma patients. Mol. Med. 15, 220–227. doi:10.2119/molmed.2009.00027
Wood, J. J., Rodrick, M. L., O'Mahony, J. B., Palder, S. B., Saporoschetz, I., D'Eon, P., et al. (1984). Inadequate interleukin 2 production. A fundamental immunological deficiency in patients with major burns. Ann. Surg. 200 (3), 311–320. doi:10.1097/00000658-198409000-00008
Xiao, W., Mindrinos, M. N., Seok, J., Cuschieri, J., Cuenca, A. G., Gao, H., et al. (2011). A genomic storm in critically injured humans. J. Exp. Med. 208 (13), 2581–2590. doi:10.1084/jem.20111354
Zhang, Q., and Vignali, D. A. (2016). Co-Stimulatory and Co-inhibitory pathways in autoimmunity. Immunity 44 (5), 1034–1051. doi:10.1016/j.immuni.2016.04.017
Zhou, B., Xu, W., Herndon, D., Tompkins, R., Davis, R., Xiao, W., et al. (2010). Analysis of factorial time-course microarrays with application to a clinical study of burn injury. Proc. Natl. Acad. Sci. U. S. A. 107 (22), 9923–9928. doi:10.1073/pnas.1002757107
Keywords: burns, blunt trauma, bioinformatics, differentially expressed genes, immune cell infiltration, core immune-related genes
Citation: Chen X, Wang K, Li D, Zhao M, Huang B, Su W and Yu D (2022) Genetic and immune crosstalk between severe burns and blunt trauma: A study of transcriptomic data. Front. Genet. 13:1038222. doi: 10.3389/fgene.2022.1038222
Received: 06 September 2022; Accepted: 15 September 2022;
Published: 30 September 2022.
Edited by:
Shibiao Wan, St. Jude Children’s Research Hospital, United StatesReviewed by:
Xiangxiang Hu, University of North Carolina at Chapel Hill, United StatesZhaoyue Zhang, The Rockefeller University, United States
Xun Li, University of Maryland, United States
Copyright © 2022 Chen, Wang, Li, Zhao, Huang, Su and Yu. This is an open-access article distributed under the terms of the Creative Commons Attribution License (CC BY). The use, distribution or reproduction in other forums is permitted, provided the original author(s) and the copyright owner(s) are credited and that the original publication in this journal is cited, in accordance with accepted academic practice. No use, distribution or reproduction is permitted which does not comply with these terms.
*Correspondence: Biao Huang, hbcd1997@163.com; Wenxing Su, wenxingsu@126.com; Daojiang Yu, ydj51087@163.com
†These authors have contributed equally to this work