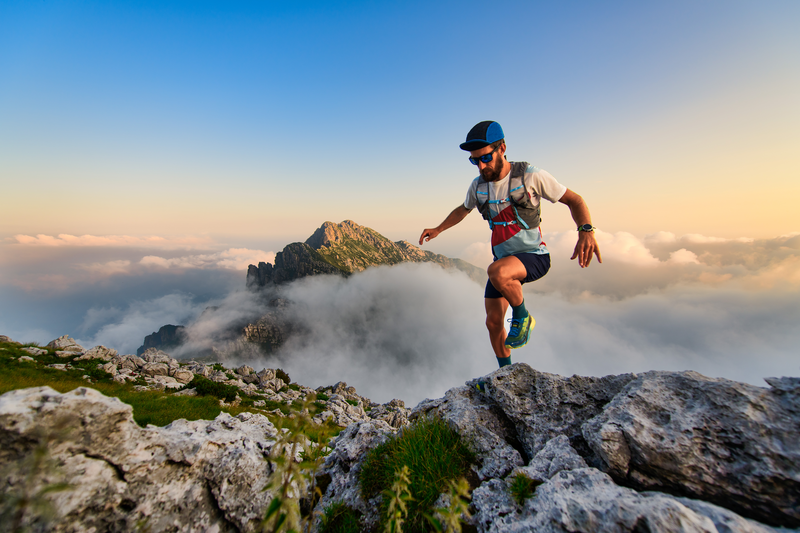
95% of researchers rate our articles as excellent or good
Learn more about the work of our research integrity team to safeguard the quality of each article we publish.
Find out more
ORIGINAL RESEARCH article
Front. Genet. , 13 October 2022
Sec. RNA
Volume 13 - 2022 | https://doi.org/10.3389/fgene.2022.1015049
This article is part of the Research Topic The Role of Extracellular Vesicles in Diseases: shedding light on their role in cell-to-cell communication View all 7 articles
Acute rejection (AR) is a common and grave complication of liver transplantation (LT). The diagnosis of AR is challenging because it has nonspecific clinical features and requires invasive procedures. Since extracellular vesicles (EVs) are promising candidates as indicators for diagnosis of various diseases, this study aimed to identify serum EV microRNAs (miRNAs) as potential biomarkers for AR in patients subjected to LT. We collected clinical information and serum samples from the liver transplant recipients with and without AR (non-AR). EVs from the serum were isolated via ultracentrifugation and identified using transmission electron microscopy, nanoparticle tracking analysis, and western blotting. EV RNA was extracted and sequenced on an Illumina HiSeq 2500/2000 platform to identify differentially expressed miRNAs between the groups. Gene Ontology (GO) and Kyoto Encyclopedia of Genes and Genomes (KEGG) enrichment analyses were performed on the target gene candidates of the differentially expressed miRNAs to test their functions in biological systems. Then, we validated 12 differentially expressed miRNAs by quantitative real-time PCR. The results demonstrated that 614 EV miRNAs were significantly altered (387 up regulated and 227 down regulated) between non-AR and AR patients. GO enrichment analysis revealed that these target genes were related to cellular processes, single-organism processes, biological regulation, metabolic processes, cells, cell parts, protein-binding processes, nucleoid binding, and catalytic activity. Furthermore, KEGG pathway analysis demonstrated that the target genes of the differentially expressed miRNAs were primarily involved in ubiquitin-mediated proteolysis, lysosomes, and protein processing in the endoplasmic reticulum. miR-223 and let-7e-5p in AR patients were significantly up-regulated compared to those in non-AR patients, whereas miR-199a-3p was significantly down-regulated, which was consistent with sequencing results. The expression of serum EV miRNAs (up-regulated: miR-223 and let-7e-5p and miR-486-3p; down regulated: miR-199a-3p, miR-148a-3p and miR-152-3p) in AR patients was significantly different from that in non-AR patients, and these miRNAs can serve as promising diagnostic biomarkers for AR in patients subjected to liver transplant.
Liver transplantation (LT) has become the most effective curative therapy for end-stage liver disease with an irreversible degradation of liver function. However, serious postoperative complications remain a major challenge limiting the long-term success of LT (Meirelles Junior et al., 2015). Rejection is an immune reaction in which a recipient lymphatic system recognizes and activates graft-specific antigens and attacks and damages grafts. Acute rejection (AR) is one of the most common and intractable complications of graft introduction, with the one-year incidence rate of 11.5% (age dependent range 9.4%–20.5%) (Kim et al., 2016; Schütz et al., 2017). It usually occurs within the first 3 months and affects the function of the transplanted liver and the prognosis of patients. Traditional methods are affected by many factors that lack specificity and sensitivity. The gold standard for diagnosing AR is percutaneous liver biopsy; however, it still faces the challenge of being invasive and delayed. The accuracy of pathological diagnosis is affected by factors, such as the experience level of the observing pathologists and the sampling quality (Jadlowiec and Taner, 2016; Rastogi, 2022). The onset of symptoms marks the transition from subclinical to clinical disease, and genetic changes occur before the onset of clinical symptoms. Therefore, a method to non-invasively identify early biomarkers at the cellular and molecular levels is urgently needed to diagnose AR (Krenzien et al., 2019).
Extracellular vesicles (EVs) are nano-sized membrane vesicles carrying proteins, mRNAs, and other small RNAs found in most biological fluids (Thery, 2011). They are released into the extracellular region by most cell types and act as mediators in cell-cell communication, modulating various types of physiological processes, including immune disorders (Obregon et al., 2006; Valadi et al., 2007; McLellan, 2009; van der Vlist et al., 2012). They have also shown great potential as diagnostic or therapeutic tools (Lin et al., 2015). Some studies also highlighted the role of EVs in the alternation of the immune response in the field of transplant immunology. T cells release EV carrying miR-142-3p, which are transferred to endothelial cells and impair endothelial integrity via down-regulation of RAB11FIP2 during cardiac allograft rejection (Dewi et al., 2017). Another study using high-throughput sequencing of the EV microRNA (miRNA) profile in the peripheral blood revealed 52 known and 5 conserved EV miRNAs as diagnostic biomarkers specifically expressed in recipients with delayed graft function (Wang et al., 2019).
miRNAs play important roles in numerous cellular functions by mediating gene expression post-transcriptionally. Recent studies have shown that miRNAs showed different expression profiles in patients subjected to organ transplantation (Afshari et al., 2021; Boštjančič et al., 2021). Increasing publications suggests that miRNAs may serve as potential non-invasive biomarkers for AR during transplant (Hamdorf et al., 2017; Lin et al., 2021). miRNAs could be detected in nearly all body fluids (serum, bile, and urine) and have been proposed to be potential biomarkers for diagnosis and therapy (Cortez et al., 2011). EV miRNAs may be more stable and sensitive than circulating free miRNAs. However, to our knowledge, hardly any studies have reported EV miRNAs as biomarkers for AR after LT. Hence, the present study investigated the hypothesis that differentially expressed immune-related EV miRNAs may provide key information about allograft status and can be used as circulating markers for diagnosing AR after LT. This study also identified functions and signaling pathways of the differentially expressed EV miRNA target genes. The findings of this study contribute to the investigation of the feasibility of EV miRNAs as liquid biopsy biomarkers for patients with AR after LT.
Sixty patients who underwent LT at the First Affiliated Hospital of Xi’an Jiaotong University between 2016 and 2019 were included in this study (Supplementary Table S1). Thirty patients were diagnosed with T-cell mediated AR via liver biopsy using the Banff criteria based on clinical suspicion. Another 30 transplant patients without AR (non-AR) after surgery were considered as controls and followed up regularly every 2–4 weeks. Every patient received a graft via donation after a citizen’s death. Informed consent was obtained from all patients or their legally authorized representatives. This study was approved by the Ethics Committee of Xi’an Jiaotong University. Serum samples of patients with AR were collected immediately after onset, and then, the initial treatment was carried out. Non-AR sera were obtained on the date matching patients with AR as much as possible (7–55 days after LT). Serum samples were divided into a test set (n = 10 each for AR and non-AR) and a verification set (n = 20 each for AR and non-AR). All serum samples were stored at −80°C until use.
EVs were isolated using an ExoQuick™ Exosome Precipitation Solution (System Biosciences, United States) according to the manufacturer’s instructions. First, the serum was centrifuged at 2000 × g for 15 min to remove the cells and cell debris. The supernatant obtained was diluted in 500 μl Dulbecco’s phosphate-buffered saline (DPBS) at equal volumes and then mixed with 252 μl ExoQuick exosome precipitation solution. After incubation at 4°C for 1 h, the obtained mixture was centrifuged at 1,500 × g for 0.5 h. Finally, the supernatant was discarded and EVs pellets were diluted in 150 μl DPBS.
The EV suspension (10 μl) was loaded onto a copper grid and was stained with uranyl acetate for 1 min. The grids were washed and dried with filter paper for 10 min at room temperature, and the morphology of the EVs was observed under a transmission electron microscope (Hitachi, Japan) at a voltage of 100 kV. The average concentration and size distribution of EVs were determined using nanoparticle tracking analysis (NanoFCM, China).
Dilutions of the EV suspension were also prepared. Briefly, 10 μl of the original EV suspensions were diluted at 1/10, 1/100, and 1/1,000. Anti-mouse/rat CD81 antibodies fluorescently labeled with PE (BioLegend, United States) and APC-conjugated anti-mouse CD63 antibodies (BioLegend, United States) were incubated with 100 μl of the diluted EVs suspensions for 15 min at room temperature in the dark, and the proportion of CD 81 and CD 63 positive cells was determined using a flow cytometer (Beckman, United States).
RNA was extracted from the EV pellets using an Exosomal RNA Isolation Kit (Norgen, United States), according to the manufacturer’s protocol. RNA concentration was measured using Nanodrop 2000 (Thermo Fisher Scientific, United States).
Three micrograms of total RNA was used for the construction of a small RNA library by a NEBNext® Multiplex Small RNA Library Prep Set for Illumina® (NEB, United States) according to the manufacturer’s instructions. Index codes were added to the attribute sequences of each sample. Agilent Bioanalyzer 2100 system with DNA High Sensitivity Chips was used for quality check of the library. Clustering of the index-coded samples was performed on a cBot Cluster Generation System using a TruSeq SR Cluster Kit v3-cBot-HS (Illumina, United States) according to the manufacturer’s instructions. After cluster generation, the library preparations were sequenced on an Illumina HiSeq 2500/2000 platform and 50-bp single-end reads were generated.
Briefly, clean reads were obtained from raw data by removing reads containing adapter dimers, junk, and low-quality reads, and a certain range of lengths from clean reads was chosen for further analyses. The small RNA tags were mapped to search for known miRNAs using miRBase 20.0 as reference. Custom scripts were used to obtain the miRNA counts and base bias at the first position of the identified miRNA with a certain length and at each position of all identified miRNAs, respectively. Novel miRNAs were predicted using two software packages (miREvo and mirdeep2) by exploring their secondary structures. Differential miRNA expression analysis was performed using the DEGseq (2010) R package. A q value <0.01 and log2 (fold change) > 1 were set by default as the threshold for significant differential expression. To predict the genes targeted by the most abundant miRNAs, computational target prediction algorithms (miRanda 3.3a) were used to identify miRNA-binding sites. Finally, Gene Ontology (GO) and Kyoto Encyclopedia of Genes and Genomes (KEGG) enrichment analyses were performed to reveal functions and pathways.
To validate the sequencing results, qRT-PCR was performed using the SYBR Green PCR kit (GenStar, China), according to the manufacturer’s instructions. Assays were performed on AR and non-AR samples (n = 20 each) for 12 immune-related miRNAs that met the defined criteria. Each reaction was performed in a 20 μl volume containing 2 μl cDNA, 1 μl each primer, 10 μl SYBR Green, and 6 μl RNase-free water. All reactions were performed in triplicate using an IQ5 Multicolor Real-Time PCR Detection System (Bio-Rad, United States). The 2−ΔΔCt method was used for quantitation and U6 was used as the miRNA control. The Ct value range of qRT-PCR is from 14 to 32, and fold change <0.5 or >2 was considered to be significantly down-regulated or up-regulated, respectively. The primer sequences used are listed in Supplementary Table S2.
All experiments were performed in triplicate and statistical analyses were performeded using GraphPad Prism (San Diego, United States). For miRNA expression, Student’s t-test was used to analyze the differences between two independent groups. Data were reported as the mean ± SD, and p-values < 0.05 was considered statistically significant.
Immunosuppressive treatment consists of tacrolimus/cyclosporine, mycophenolate mofetil, and prednisone was administered to recipients after transplantation to prevent AR. Basic information regarding the liver transplant recipients is shown in Supplementary Table S1. We found that the AR and non-AR patients did not differ significantly in terms of sex, etiology, Child-Pugh score, model for end-stage liver disease scores (MELD scores), cold/warm ischemia time, and ABO compatibility. However, the mean immunosuppressive concentration in AR patients during the first week of treatment was significantly lower than non-AR patients (p < 0.05) (Supplementary Table S1). Selected serum samples were then collected for EV isolation and sequencing analysis to screen potential biomarkers for the diagnosis of AR (Figure 1).
We isolated EV from the serum of all participants by ultracentrifugation as previously reported (Thery et al., 2006) (Figure 2A). Transmission electron microscopy revealed the morphology of the EV isolated from patient serum (Figure 2B), which was consistent with previous reports. Nanoparticle tracking analysis revealed an average diameter of approximately 85.52 nm (Figure 2C), suggesting sufficient EV purification. Flow cytometry was also performed to detect two commonly used EV protein markers: CD63 and CD81. The results showed that both protein markers were highly expressed in the isolated EV (Figure 2D). Taken together, we confirmed the successful purification of EV from all serum samples.
FIGURE 2. Characterization of EVs. (A)The procedures for EVs isolation used in this study. (B) Morphological characterization of EVs isolated from serum by transmission electron microscopy. Typical EVs were amplified with red arrows. Scale bar = 100 nm (upper). (C) Average size distribution of EVs was revealed by nanoparticle tracking analysis. (D) EVs protein markers (CD 63 and CD 81) were identified by flow cytometry.
We obtained 13,067,031 and 15,270,653 total reads from non-AR and AR patients with solexa high-throughput sequencing. After removing the reads containing poly-N, length <15 or >35, and low-quality reads, 11,982,753 (91.7%) and 13,990,228 (91.6%) high-quality clean reads were extracted from the two groups, respectively (Table 1). The distributions of sequence length were then analyzed, and a certain length range was selected. The lengths of the clean reads peaked at 21–22 nt, which are within the range for miRNAs (Figures 3A,B). Approximately 62% and 18.78% of sRNA could be mapped to the genome in non-AR and AR patients using Bowtie software (Langmead et al., 2009) (Table 2). In addition, density statistics of the reads of each chromosome on the genome of each sample were determined, and the distribution of the reads on each chromosome was checked using Circos mapping (Figures 3C,D). Next, the reads of rRNA, tRNA, snoRNA, and other snRNAs were annotated and removed for subsequent analysis (Supplementary Figure S1; Supplementary Table S3).
FIGURE 3. The length distribution of the clean reads sequence and location of clean reads on each chromosome. (A,C) non-AR patients. (B,D) AR patients.
Mapped small RNA tags and miRBase 20.0 were used to identify known miRNAs. A total of 515 and 397 mature miRNAs were identified, respectively (Table 3). miRNA expression levels were estimated as transcript per million (TPM) and differential expression analysis was performed between the non-AR and AR patients (Supplementary Figure S2; Supplementary Table S4; Supplementary Table S5). Compared to the non-AR patients, 387 miRNAs (including miR-96-5p, miR-1290, miR-19b-3p, and miR-30b-5p) were significantly upregulated, whereas 227 miRNAs (including miR-134-5p, miR-455-5p, miR-10a-5p, and miR-194-5p) were downregulated in patients with AR (Figures 4A,B).
FIGURE 4. Volcano plot and heat map of differential expressed miRNAs obtained from the comparison of non-AR and AR patients. (A) Each point in the volcano plot represents one miRNA. Red points represent upregulated ones, green points represent downregulated ones, and dark points represent no significant changes in miRNAs. (B) The abscissa represents different groups, the ordinate represents the different miRNAs compared in the groups, and the color blocks at different positions represent the relative miRNA expressions.
Target genes of miRNAs were predicted using RNAhybrid and miRanda. The distribution of candidate genes of differentially expressed miRNAs in GO was compared with that of the references, and the number of genes of the significantly enriched GO terms was counted to determine the biological functions that were significantly correlated (Supplementary Table S6). The enrichment results showed that the target genes were related to cellular processes, single-organism processes, biological regulation, metabolic processes, cells, cell parts, protein binding processes, nucleoid binding, and catalytic activity (Figure 5; Supplementary Figure S3).
FIGURE 5. GO function classification target genes of known differential expressed miRNAs in AR patients compared with non-AR patients. Abscissa: class classification. The three different classifications represent the three basic classifications of GO terms. From left to right they are biological process, cellular component, and molecular function.
The target candidate genes were mapped to reference pathways recorded in the KEGG database to identify the biological pathways through which the differentially expressed miRNAs were involved in AR (Supplementary Table S7). KEGG pathway analysis revealed 20 major pathways occupied by the most abundant target genes of differentially expressed miRNAs. In patients with AR, the target gene enrichment pathways included ubiquitin-mediated proteolysis, lysosomes, and protein processing in the endoplasmic reticulum (Figure 6). These results revealed the potential function of miRNA targets, which may form a regulatory network and play a vital role in the disease process of AR.
FIGURE 6. KEGG pathway analysis of the target genes of known differential expressed miRNAs in AR patients compared with non-AR patients. Horizontal axis: Rich factor. The larger the point, the higher the enrichment degree, the more candidate target genes in this pathway, and the color of the point correspond to a different q value range. Vertical axis: The definition of the pathway.
To narrow down the potential candidates, miRNAs were screened from immune-related literatures published on PubMed first. Then, 12 hsa-miRNAs with a significant and consistent fold-change were selected for further validation by RT-qPCR. Notably, three of these miRNAs (miR-223, let-7e-5p, and miR-486-3p) were up regulated, whereas miR-199a-3p, miR-148a-3p, and miR-152-3p were significantly down regulated, exhibiting a similar trend from the RNA sequencing data (Figure 7). We then analyzed the correlation between these differentially expressed miRNAs and clinical information, and found that miR-223 was positively correlated with lymphocytes in patients with AR (Table 4).
FIGURE 7. Validation of differential expressed miRNAs by qRT-PCR. miRNA expression of fold change detected by qRT-PCR in AR patients compared with non-AR patients (n = 3, p* < 0.05, p** <0.01, p*** <0.001, ns = no significance).
AR is a complex immunological process mediated by a variety of cells (including lymphocytes, dendritic cells, and macrophages) and antibodies (Charlton et al., 2018). The main mechanism for AR may be defined as when the recipient lymphatic system recognizes and activates graft-specific antigens, attacks and damages grafts, while humoral immunity gradually develops (Dogan et al., 2018). Most biomarkers are proinflammatory factors, immunomodulatory cytokines, and inflammation-related proteins that could overlap across diseases (Naesens and Anglicheau, 2018; Mirzakhani et al., 2019; Kohut et al., 2020). For example, IL-2 promotes proliferation and differentiation of T cells and its subsets, stimulates the maturation of B cells, and mediates graft rejection (Holzknecht and Platt, 2000). Cytotoxic T lymphocytes act as effector cells that mediate graft rejection. The CD4/CD8 ratio can be used to monitor immune function since a low CD4/CD8 ratio in graft tissues or serum is associated with AR (Shenoy et al., 2012). Many clinical trials have shown that intracellular ATP levels in CD4+ T cells can act as markers for identifying infection or allograft rejection (Israeli et al., 2008). However, iATP seems to be more effective in identifying over-immunosuppressed patients with infection than rejection, and should be used in combination with other biomarkers to improve the efficacy of diagnosis (Millan et al., 2009). With the development of technology, some relatively noninvasive and continuous detection methods have been developed, such as cytokines, CD families, and proteomics, among others (Perottino et al., 2022). Lun et al. (2002) and Chae et al. (2018) found that IL-2, IL-6, and IL-17 production by T cells significantly increased during AR. Massoud et al. (2011) previously screened and found 41 highly expressed proteins (such as Hsp60, Hsp70, CC1q, CC3, CC4, CD33, and other immune-activating mediators) in the serum of patients with AR after LT compared to those with non-AR. After verifying independent samples, complement C4 was found to be a good predictor of AR in liver transplant recipients. Besides, many studies found that graft-derived cell-free DNA acted as a non-invasive early rejection and graft damage marker for LT (Schütz et al., 2017; Baumann et al., 2022; Levitsky et al., 2022). However, these studies are still in the experimental research stage; hence their detection efficiency is relatively limited and they have not yet been applied in large-scale clinical practice.
Mounting evidence has proven that miRNAs play critical roles in immune cell development, immune responses, and immune tolerance. As novel diagnostic markers, miRNAs found in EV can be used as noninvasive sensors for acute and chronic transplant rejection (Hamdorf et al., 2017). The significance of this potential value in liver transplant rejection is supported by an animal study conducted by Morita et al. (2014) They investigated the miRNAs involved in the AR of liver allografts in mice and found that levels of miR-146a, -15b, -223, -23a, -27a, -34a, and -451 were significantly increased in the grafts, while those of miR-101a, -101b, and -148a were decreased. A study on human renal transplantation that detected differentially expressed miRNAs found that miR-223 was overexpressed in AR biopsies compared with that in normal allograft biopsies (Ledeganck et al., 2019). Indeed, among the miRNAs overexpressed in AR biopsies, miR-223 levels were the highest; they were also highly expressed in normal human peripheral blood mononuclear cells (PBMCs). Further investigation showed that the activation of PBMCs with the mitogen phytohemagglutinin resulted in a reduction in miR-223 expression (Anglicheau et al., 2009). Let-7, as a highly conserved miRNA family, plays an anti-inflammatory role. Intranasal administration of let-7 mimics reduced IL-13 levels in allergic lungs and alleviated asthma features, such as airway hyper responsiveness, airway inflammation, and goblet cell metaplasia (Kumar et al., 2011). In hematopoietic stem cell transplantation, the study performed by Rachel et al. revealed that miR-199 expression was lower in the EV fraction of patients who subsequently developed acute graft-versus-host disease (aGvHD), verifying the capacity of circulating miR-199 as a diagnostic and prognostic aGvHD biomarker (Crossland et al., 2016). To our knowledge, for the first time in patients subjected to LT, the present study showed a significant elevation in miR-223 and let-7e-5p levels and a reduction of miR-199a-3p levels in EV from serum of patients with those in AR compared with non-AR. This was consistent with the results of other studies of immune disorders; hence, we propose that these EV miRNAs might be novel diagnostic biomarkers for AR.
Selecting one or more closely associated miRNAs would be helpful in exploring the role of EV miRNAs in the mechanism of AR. It is well known that miRNAs regulate gene expression by targeting mRNAs and act as master regulators of various signaling pathways. Therefore, the first principle is to select miRNAs that closely match the genetic background of the target diseases. AR has been shown to be closely related to immune disorders. Based on the results of GO enrichment analysis, we selected 12 miRNAs closely related to immunity for further experimental validation, of which 3 (miR-223, let-7e-5p, and miR-199a-3p) were significantly associated with AR. Second, the choice should be based on KEGG enrichment analyses. Our present data showed that ubiquitin-mediated proteolysis, a widespread and important type of protein regulation at the post-translational level is a reliable pathway. Thus, miR-223 and miR-199a should be chosen for further study, as they have been associated with esophageal squamous cell carcinoma (Kurashige et al., 2012) and human end-stage dilated cardiomyopathy (Baumgarten et al., 2013). However, this study had some limitations. First, we did not examine the correlation between the target genes and candidate miRNAs in patients with AR. This may help explain how EV miRNAs affect the expression of target genes and potential signaling pathways, and will be addressed in our future research. Second, the sample size was relatively limited, so further research involving more samples or establishing multicenter collaboration would be beneficial. Moreover, single biomarkers have insufficient sensitivity and specificity. Therefore, multiple biomarker panels, such as patient clinical manifestations, laboratory results, and other biomarkers, could provide a more robust diagnosis of AR in LT. We hope that our results may provide a reliable serum biomarker for diagnosing AR and uncovering its potential molecular mechanisms in the future.
In conclusion, this study was the first attempt to screen EVs-based biomarkers for patients with AR after LT, and we analyzed the analyzing differential miRNA expression profiles through high-throughput sRNA sequencing and experimental validation. The expression levels of serum EV miRNAs (up-regulated: miR-223 and let-7e-5p and miR-486-3p; down regulated: miR-199a-3p, miR-148a-3p and miR-152-3p) in patients with AR are significantly different from those in patients with non-AR and can serve as promising diagnostic biomarkers for AR in patients subjected to liver transplant.
All data are available within this manuscript and its Supplementary Material. sRNA-seq data has been uploaded to SRA repository (PRJNA885293).
The studies involving human participants were reviewed and approved by Xi’an Jiaotong University. The patients/participants provided their written informed consent to participate in this study. Written informed consent was obtained from the individual(s) for the publication of any potentially identifiable images or data included in this article.
WW, WL, and BG contributed to the data interpretation and drafting manuscript. LC, BW, CL, and YQ contributed to sample collection and data interpretation. BG and CH contributed to the study design and revising of the manuscript. All authors contributed to the article and approved the submitted version.
This work was supported by the Natural Science Foundation of Shaanxi Province (2022JQ-902, 2019JQ-390), and the Scientific Research and Sharing Platform Construction Project of Shaanxi Province (2022PT-07).
The authors declare that the research was conducted in the absence of any commercial or financial relationships that could be construed as a potential conflict of interest.
All claims expressed in this article are solely those of the authors and do not necessarily represent those of their affiliated organizations, or those of the publisher, the editors and the reviewers. Any product that may be evaluated in this article, or claim that may be made by its manufacturer, is not guaranteed or endorsed by the publisher.
The Supplementary Material for this article can be found online at: https://www.frontiersin.org/articles/10.3389/fgene.2022.1015049/full#supplementary-material
r, Correlation Coefficient; EO, Eosnophils; LYM, Lymphocyte; ALT, Alanine Aminotransferase; AST, Aspartate Aminotransferase; GGT, Gamma-Glutamyl Transferase; ALP, Alkaline Phosphatase; TBIL, Total Bilirubin.
Afshari, A., Yaghobi, R., Karimi, M. H., and Mowla, J. (2021). Alterations in MicroRNA gene expression profile in liver transplant patients with hepatocellular carcinoma. BMC Gastroenterol. 21 (1), 262. doi:10.1186/s12876-020-01596-2
Anglicheau, D., Sharma, V. K., Ding, R., Hummel, A., Snopkowski, C., Dadhania, D., et al. (2009). MicroRNA expression profiles predictive of human renal allograft status. Proc. Natl. Acad. Sci. U. S. A. 106 (13), 5330–5335. doi:10.1073/pnas.0813121106
Baumann, A. K., Beck, J., Kirchner, T., Hartleben, B., Schütz, E., Oellerich, M., et al. (2022). Elevated fractional donor-derived cell-free DNA during subclinical graft injury after liver transplantation. Liver Transplant. 16. doi:10.1002/lt.26479
Baumgarten, A., Bang, C., Tschirner, A., Engelmann, A., Adams, V., von Haehling, S., et al. (2013). TWIST1 regulates the activity of ubiquitin proteasome system via the miR-199/214 cluster in human end-stage dilated cardiomyopathy. Int. J. Cardiol. 168 (2), 1447–1452. doi:10.1016/j.ijcard.2012.12.094
Boštjančič, E., Večerić-Haler, Ž., and Kojc, N. (2021). The role of immune-related miRNAs in the pathology of kidney transplantation. Biomolecules 11 (8), 1198. doi:10.3390/biom11081198
Chae, M. S., Kim, J. W., Chung, H. S., Park, C. S., Lee, J., Choi, J. H., et al. (2018). The impact of serum cytokines in the development of early allograft dysfunction in living donor liver transplantation. Medicine 97 (16), e0400. doi:10.1097/MD.0000000000010400
Charlton, M., Levitsky, J., Aqel, B., O'Grady, J., Hemibach, J., Rinella, M., et al. (2018). International liver transplantation society consensus statement on immunosuppression in liver transplant recipients. Transplantation 102 (5), 727–743. doi:10.1097/Tp.0000000000002147
Cortez, M. A., Bueso-Ramos, C., Ferdin, J., Lopez-Berestein, G., Sood, A. K., and Calin, G. A. (2011). MicroRNAs in body fluids--the mix of hormones and biomarkers. Nat. Rev. Clin. Oncol. 8 (8), 467–477. doi:10.1038/nrclinonc.2011.76
Crossland, R. E., Norden, J., Pearce, K. F., Juric, M. K., Lendrem, C., Bibby, L. A., et al. (2016). Serum and extracellular vesicle micrornas MiR-423, MiR-199 and MiR-93*As biomarkers for acute graft versus host disease. Front. Immunol. 128 (22), 1446. doi:10.3389/fimmu.2017.01446
Dewi, I. S., Celik, S., Karlsson, A., Hollander, Z., Lam, K., McManus, J. W., et al. (2017). Exosomal miR-142-3p is increased during cardiac allograft rejection and augments vascular permeability through down-regulation of endothelial RAB11FIP2 expression. Cardiovasc. Res. 113 (5), 440–452. doi:10.1093/cvr/cvw244
Dogan, N., Husing-Kabar, A., Schmidt, H. H., Cicinnati, V. R., Beckebaum, S., and Kabar, I. (2018). Acute allograft rejection in liver transplant recipients: Incidence, risk factors, treatment success, and impact on graft failure. J. Int. Med. Res. 46 (9), 3979–3990. doi:10.1177/0300060518785543
Hamdorf, M., Kawakita, S., and Everly, M. (2017). The potential of MicroRNAs as novel biomarkers for transplant rejection. J. Immunol. Res. 2017, 4072364. doi:10.1155/2017/4072364
Holzknecht, Z. E., and Platt, J. L. (2000). The fine cytokine line between graft acceptance and rejection. Nat. Med. 6 (5), 497–498. doi:10.1038/74963
Israeli, M., Klein, T., Sredni, B., Avitzur, Y., Mor, E., Bar-Nathen, N., et al. (2008). ImmuKnow: A new parameter in immune monitoring of pediatric liver transplantation recipients. Liver Transpl. 14 (6), 893–898. doi:10.1002/lt.21426
Jadlowiec, C. C., and Taner, T. (2016). Liver transplantation: Current status and challenges. World J. Gastroenterol. 22 (18), 4438–4445. doi:10.3748/wjg.v22.i18.4438
Kim, W. R., Lake, J. R., Smith, J. M., Skeans, M. A., Schladt, D. P., Edwards, E. B., et al. (2016). Liver. Am. J. Transpl. 16 (2), 69–98. doi:10.1111/ajt.13668
Kohut, T. J., Barandiaran, J. F., and Keating, B. J. (2020). Genomics and liver transplantation: Genomic biomarkers for the diagnosis of acute cellular rejection. Liver Transpl. 26 (10), 1337–1350. doi:10.1002/lt.25812
Krenzien, F., Keshi, E., Splith, K., Griesel, S., Kamali, K., Sauer, I. M., et al. (2019). Diagnostic biomarkers to diagnose acute allograft rejection after liver transplantation: Systematic review and meta-analysis of diagnostic accuracy studies. Front. Immunol. 10, 758. doi:10.3389/fimmu.2019.00758
Kumar, M., Ahmad, T., Sharma, A., Mabalirajan, U., Kulshreshtha, A., Agrawal, A., et al. (2011). Let-7 microRNA-mediated regulation of IL-13 and allergic airway inflammation. J. Allergy Clin. Immunol. 128 (5), 1077–1085. e1071-1010. doi:10.1016/j.jaci.2011.04.034
Kurashige, J., Watanabe, M., Iwatsuki, M., Kinoshita, K., Saito, S., Hiyoshi, Y., et al. (2012). Overexpression of microRNA-223 regulates the ubiquitin ligase FBXW7 in oesophageal squamous cell carcinoma. Br. J. Cancer 106 (1), 182–188. doi:10.1038/bjc.2011.509
Langmead, B., Trapnell, C., Pop, M., and Salzberg, S. L. (2009). Ultrafast and memory-efficient alignment of short DNA sequences to the human genome. Genome Biol. 10 (3), R25. doi:10.1186/gb-2009-10-3-r25
Ledeganck, K. J., Gielis, E. M., Abramowicz, D., Stenvinkel, P., Shiels, P. G., and VanCraenenbroeck, A. H. (2019). MicroRNAs in AKI and kidney transplantation. Clin. J. Am. Soc. Nephrol. 14 (3), 454–468. doi:10.2215/CJN.08020718
Levitsky, J., Kandpal, M., Guo, K., Kleiboeker, S., and Sinha, R. (2022). Donor-derived cell-free DNA levels predict graft injury in liver transplant recipients. Am. J. Transpl. 22 (2), 532–540. doi:10.1111/ajt.16835
Lin, J., Li, J., Huang, B., Liu, J., Chen, X., Chen, X. M., et al. (2015). Exosomes: Novel biomarkers for clinical diagnosis. ScientificWorldJournal. 2015, 657086. doi:10.1155/2015/657086
Lin, Y., Wang, L., Ge, W., Hui, Y., Zhou, Z., Hu, L., et al. (2021). Multi-omics network characterization reveals novel microRNA biomarkers and mechanisms for diagnosis and subtyping of kidney transplant rejection. J. Transl. Med. 19 (1), 346. doi:10.1186/s12967-021-03025-8
Lun, A., Cho, M. Y., Muller, C., Staffa, G., Bechstein, W. O., Radke, C., et al. (2002). Diagnostic value of peripheral blood T-cell activation and soluble IL-2 receptor for acute rejection in liver transplantation. Clin. Chim. Acta. 320 (1-2), 69–78. doi:10.1016/s0009-8981(02)00045-1
Massoud, O., Heimbach, J., Viker, K., Krishnan, A., Poterucha, J., Sanchez, W., et al. (2011). Noninvasive diagnosis of acute cellular rejection in liver transplant recipients: A proteomic signature validated by enzyme-linked immunosorbent assay. Liver Transpl. 17 (6), 723–732. doi:10.1002/lt.22266
McLellan, A. D. (2009). Exosome release by primary B cells. Crit. Rev. Immunol. 29 (3), 203–217. doi:10.1615/critrevimmunol.v29.i3.20
Meirelles Junior, R. F., Salvalaggio, P., Rezende, M. B., Evangelista, A. S., Guardia, B. D., Matielo, C. E., et al. (2015). Liver transplantation: History, outcomes and perspectives. Einstein (Sao Paulo) 13 (1), 149–152. doi:10.1590/S1679-45082015RW3164
Millan, O., Sanchez-Fueyo, A., Rimola, A., Guillen, D., Hidalgo, S., Benitez, C., et al. (2009). Is the intracellular ATP concentration of cd4+t-cells a predictive biomarker of immune status in stable transplant recipients? Transplantation 88 (3), S78–S84. doi:10.1097/TP.0b013e3181afeba6
Mirzakhani, M., Mohammadnia-Afrouzi, M., Shahbazi, M., Mirhosseini, S. A., Hosseini, H. M., and Amani, J. (2019). The exosome as a novel predictive/diagnostic biomarker of rejection in the field of transplantation. Clin. Immunol. 203, 134–141. doi:10.1016/j.clim.2019.04.010
Morita, M., Chen, J. J., Fujino, M., Kitazawa, Y., Sugioka, A., Zhong, L., et al. (2014). Identification of microRNAs involved in acute rejection and spontaneous tolerance in murine hepatic allografts. Sci. Rep. 4, 6649. doi:10.1038/srep06649
Naesens, M., and Anglicheau, D. (2018). Precision transplant medicine: Biomarkers to the rescue. J. Am. Soc. Nephrol. 29 (1), 24–34. doi:10.1681/Asn.2017010004
Obregon, C., Rothen-Rutishauser, B., Gitahi, S. K., Gehr, P., and Nicod, L. P. (2006). Exovesicles from human activated dendritic cells fuse with resting dendritic cells, allowing them to present alloantigens. Am. J. Pathol. 169 (6), 2127–2136. doi:10.2353/ajpath.2006.060453
Perottino, G., Harrington, C., and Levitsky, J. (2022). Biomarkers of rejection in liver transplantation. Curr. Opin. Organ Transpl. 27 (2), 154–158. doi:10.1097/MOT.0000000000000959
Rastogi, A. (2022). Liver transplant biopsy interpretation: Diagnostic considerations and conundrums. Indian J. Pathol. Microbiol. 65 (2), 245–257. doi:10.4103/ijpm.ijpm_1090_21
Schütz, E., Fischer, A., Beck, J., Harden, M., Koch, M., Wuensch, T., et al. (2017). Graft-derived cell-free DNA, a noninvasive early rejection and graft damage marker in liver transplantation: A prospective, observational, multicenter cohort study. PLoS Med. 14 (4), e1002286. doi:10.1371/journal.pmed.1002286
Shenoy, K. V., Solomides, C., Cordova, F., Rogers, T. J., Ciccolella, D., and Criner, G. J. (2012). Low CD4/CD8 ratio in bronchus-associated lymphoid tissue is associated with lung allograft rejection. J. Transpl. 2012, 928081. doi:10.1155/2012/928081
Thery, C., Amigorena, S., Raposo, G., and Clayton, A. (2006). Isolation and characterization of exosomes from cell culture supernatants and biological fluids. Curr. Protoc. Cell Biol. 3, Unit 3.22. doi:10.1002/0471143030.cb0322s30
Thery, C. (2011). Exosomes: Secreted vesicles and intercellular communications. F1000 Biol. Rep. 3, 15. doi:10.3410/B3-15
Valadi, H., Ekstrom, K., Bossios, A., Sjostrand, M., Lee, J. J., and Lotvall, J. O. (2007). Exosome-mediated transfer of mRNAs and microRNAs is a novel mechanism of genetic exchange between cells. Nat. Cell Biol. 9 (6), 654–659. doi:10.1038/ncb1596
van der Vlist, E. J., Arkesteijn, G. J., van de Lest, C. H., Stoorvogel, W., Nolte-'t Hoen, E. N., and Wauben, M. H. (2012). CD4(+) T cell activation promotes the differential release of distinct populations of nanosized vesicles. J. Extracell. Vesicles 1, 18364. doi:10.3402/jev.v1i0.18364
Keywords: acute rejection, liver transplantaion, biomarker, extracellular vesicle, microRNA
Citation: Wang W, Li W, Cao L, Wang B, Liu C, Qin Y, Guo B and Huang C (2022) Serum extracellular vesicle MicroRNAs as candidate biomarkers for acute rejection in patients subjected to liver transplant. Front. Genet. 13:1015049. doi: 10.3389/fgene.2022.1015049
Received: 10 August 2022; Accepted: 28 September 2022;
Published: 13 October 2022.
Edited by:
Yuan Zhou, Peking University, ChinaReviewed by:
Sina Naserian, Hôpital Paul Brousse, FranceCopyright © 2022 Wang, Li, Cao, Wang, Liu, Qin, Guo and Huang. This is an open-access article distributed under the terms of the Creative Commons Attribution License (CC BY). The use, distribution or reproduction in other forums is permitted, provided the original author(s) and the copyright owner(s) are credited and that the original publication in this journal is cited, in accordance with accepted academic practice. No use, distribution or reproduction is permitted which does not comply with these terms.
*Correspondence: Bo Guo, Ym9fZ3VvQHhqdHUuZWR1LmNu; Chen Huang, aGNoZW5AeGp0dS5lZHUuY24=
Disclaimer: All claims expressed in this article are solely those of the authors and do not necessarily represent those of their affiliated organizations, or those of the publisher, the editors and the reviewers. Any product that may be evaluated in this article or claim that may be made by its manufacturer is not guaranteed or endorsed by the publisher.
Research integrity at Frontiers
Learn more about the work of our research integrity team to safeguard the quality of each article we publish.