- 1 College of Landscape Engineering, SuZhou Polytechnic Institute of Agriculture, Suzhou, China
- 2 Key Laboratory of Southwest China Wildlife Resources Conservation (Ministry of Education), College of Life Science, China West Normal University, Nanchong, China
Davidia involucrata is a significant living fossil with high abiotic stress tolerance. Although heat shock protein 20 (HSP20) has already been linked to heat stress, nothing is known about HSP20 family protein activities in D. involucrata. The functional dynamics of the D. involucrata HSP20 (DiHSP20) gene family were identified and characterized using a thorough genome-wide investigation. From the genome of D. involucrata, a total of 42 HSP20 genes were identified, which are distributed across 16 chromosomes. The DiHSP20 proteins were grouped into seven separate subfamilies by our phylogenetic analysis, which was validated by the conserved motif composition and gene structure studies. Segmental duplication events were shown to play a crucial role in the expansion of the DiHSP20 gene family. Synteny analysis revealed that 19 DiHSP20 genes of D. involucrata shared a syntenic connection with Arabidopsis genes, 39 with C. acuminata genes, and just 6 with O. sativa genes. Additionally, heat stress differently enhanced the expression levels of D. involucrata HSP20 genes. After 1 hour of heat treatment, the expression levels of most DiHSP20 genes, particularly DiHSP20-7, DiHSP20-29, DiHSP20-30, DiHSP20-32, and DiHSP20-34, were dramatically increased, suggestted that they might be employed as heat tolerance candidate genes. Overall, these findings add to our knowledge of the HSP20 family genes and provide helpful information for breeding heat stress resistance in D. involucrata.
Introduction
Plants are often influenced by a wide range of abiotic and biotic stresses during growth, including cold, drought, extreme heat, high salinity, and infestation by pests and pathogens (Hu et al., 2021). Over the long period of evolution, plants have evolved their unique defense mechanisms to avoid exposure to unfavorable environments, such as different physiological, molecular, morphological, and adaptive mechanisms (Wang et al., 2004). Heat shock proteins (HSP) are widely found in prokaryotic and eukaryotic organisms as a molecular chaperone and are involved in many developmental processes and responses to environmental stress. They are induced to be expressed or increased when the organism is exposed to environmental stresses, such as infection by pathogens, heat stress, reactive oxygen species (ROS), drought, and heavy metals (Kandoth et al., 2011). In general, HSP and denatured proteins produced under stress conditions maintain or restore intracellular homeostasis through folding, assembly, translocation, and degradation, thereby reducing the damage caused by stress to the organism (Maimbo et al., 2007; De Souza Resende et al., 2022).
In recent years, extreme global temperatures have been occurring more and more frequently due to anthropogenic and environmental changes. Sustained high temperatures can have a detrimental effect on plant growth and development. For example, extreme temperatures can reduce the viability of plant seeds, restrict germination and even limit plant survival (Yamori et al., 2014). As a result, it is critical to comprehend the plant’s heat tolerance. HSP is a highly conservative protein in organisms that is created when they are subjected to extreme temperatures (Gupta et al., 2010; Cui et al., 2021). Heat shock proteins are generally low in quantity under normal conditions, but when exposed to heat, they rapidly increase to account for up to 15% of total protein composition in organisms (Swindell et al., 2007; Huang et al., 2022).
Plant heat shock proteins (HSPs) respond to changes in the external environment by functioning as molecular chaperones, maintaining protein folding homeostasis, and preventing or correcting protein misfolding and degradation (Chang et al., 2006). HSP100, HSP90, HSP70, HSP60, and HSP20 are the five categories of plant HSPs based on their molecular weight and sequence homology (Waters, 2013). HSP20s are the most common and abundant proteins in plant HSPs, with molecular weights ranging from 15 to 42 kDa (Ji et al., 2019). Most HSP20s may form high-molecular-weight oligomers and are involved in protein stability. Hence, they play an essential role in the development of plant thermotolerance (Haslbeck and Vierling, 2015). For example, studies have found that heat stress will induce a large number of HSP20 genes in apple, pepper and pumpkin organisms (Guo et al., 2015; Yao et al., 2020; Hu et al., 2021). HSPs heat stress tolerance in transgenic organisms have also been confirmed by other research. For example, PtHSP17.8 from HSP20 is a heat tolerance gene in Populus trichocarpa (Li et al., 2016). The rice HSP20 gene OsHSP17.7 is involved in UV-B and heat stress (Murakami et al., 2004). Heat tolerance was improved in transgenic tobacco that overexpressed the Zea mays HSP20 gene ZmHSP16.9 (Sun et al., 2012). HSP20 genes appear to be important in modulating heat tolerance in plants, according to these research.
The alpha-crystallin domain (ACD), which contains around 90 amino acid residues, is the most conspicuous characteristic of HSP20 protein (Waters et al., 1996). The HSP20 gene family, unlike some of the other HSPs, has a lot of sequence differences and evolutionary variability (Basha et al., 2012). There are 19–94 HSP20 genes frequently researched or commercially relevant plant species (Waters, 2013). Plant HSP20s can also be classified into diverse subfamilies based on their cellular sequence similarity, location, and functionality (Waters et al., 1996). HSP20 proteins in Arabidopsis were classified into 12 subfamilies (Waters, 2013), whereas soybean HSP20 proteins were grouped into 15 subfamilies (Lopes-Caitar et al., 2013), and G. hirsutum HSP20 proteins were divided into 14 subfamilies (Ma et al., 2016).
Davidia involucrata is a living fossil that is not only recognized as an “endangered” species in China, but also serves as a symbol for the surviving relict plants of ancient provenance (Manchester et al., 2009). Because of the co-occurrence of their natural ranges and the earliest specimens by the same explorer, D. involucrata has been renowned as an exceedingly old horticultural tree and is considered as the “giant panda” of plants. As a solanaceous plant, D. involucrata is highly resistant to abiotic stresses (Chen Y. et al., 2020). The molecular regulatory mechanism governing D. involucrata responses to abiotic stressors, on the other hand, is yet unknown. Global extreme temperature have been limiting dove tree growth and development in recent years. To increase the heat resistance of D. involucrata, it is critical to research the heat resistance mechanism of D. involucrata and select intense heat resistant variants. A study of the HSP20 gene family is valuable for understanding the heat tolerance mechanism of D. involucrata.
We evaluated the phylogenetic relationships, physicochemical characteristics, cis-elements, conserved domains, gene structures, and expression patterns of HSP20 genes identified using bioinformatics approaches based on the entire genome of D. involucrata (Chen Y. et al., 2020). This research lays the groundwork for future research into the roles of the DiHSP20 gene family. Our findings may also provide light on the molecular significance of DiHSP20 during heat stress.
Materials and methods
HSP20 genes in D. involucrata
The HSP20 domain’s Hidden Markov Model (HMM) profile (PF00011) were obtained from the Protein Family Database (Pfam 34.0; http://pfam.xfam.org/). D. involucrata’s whole genome, genome annotation, and protein sequence data were obtained from the National Genomics Data Center (https://bigd.big.ac.cn/?lang=en) under accession number PRJCA001721. HMMER research (E-value < 10−5) was used to find the candidate HSP20 protein sequences (Yu et al., 2016). The positions of the conserved HSP20 domain were validated using the Simple Modular Architecture Research Tool (SMART, v9; http://smart.embl.de/smart/batch.pl), and Conserved Domain Database (CDD, v3.19; https://www.ncbi.nlm.nih.gov/Structure/bwrpsb/bwrpsb.cgi). The ultimate potential D. involucrata HSP20 proteins were found after removing redundant sequences without a similar HSP20 domain or having a molecular weight beyond the range of 15–42 kDa (Ji et al., 2019). The candidate HSP20 proteins from Oryza sativa and Arabidopsis thaliana were collected using the same way, from the Rice Genome Annotation Project (http://rice.plantbiology.msu.edu/) and TAIR database (https://www.arabidopsis.org/index.jsp).
Gene structure and sequence analysis
ExPASy’s pI/MW tool (v.3.0; http://web.expasy.org/protparam/) was used to calculate the theoretical isoelectric point (pI) and molecular weight (MW) of every HSP20 protein. The Gene Structure Display Server (GSDS 2.0; http://gsds.cbi.pku.edu.cn) was used to view the HSP20 gene structures. The MEME program (MEME Suite 5.3.3; http://meme-suite.org/tools/meme) has been used to find conserved motifs in HSP20 proteins, with the number of repeats (any, maximum number of motifs-10, and ideal motif widths set from 6 to 200 amino acid residues) being recorded. TBtools was used to visualize the findings (Chen C. et al., 2020).
Davidia involucrata HSP20 gene phylogenetic analysis and classification
The CLUSTALW (Thompson et al., 1994) tool was used to align the complete amino acid sequences of 106 HSP20 members from D. involucrata (N = 42), Arabidopsis (N = 31), and Oryza sativa (N = 33). (Supplementary Table S1). MEGA-X (Kumar et al., 2018) had been used to create a phylogenetic tree by using Maximum Likelihood (ML) approach with a 1,000-fold bootstrap test, and the best matching model is the generalized time-reversible (GTR) model in conjunction with the Shimodaira–Hasegawa (SH) test. According to the categories and in silico subcellular localization of HSP20 proteins in O. sativa and Arabidopsis, the HSP20 proteins were divided into many categories (Waters et al., 1996). To use the EvolView web program (https://evolgenius.info/evolview-v2), the phylogenetic tree was displayed and enriched.
Analysis of gene duplication and collinearity of DiHSP20s
The homologous sections of the DiHSP20 genes were initially discovered using OrthoFinder software, and a synteny graph was created using TBtools based on the found homologous gene pairs (Chen C. et al., 2020). Multiple Collinearity Scan Toolkit (MCScanX) software was used to create syntenic maps (Wang et al., 2012) to show synteny links between orthologous HSP20 genes in D. involucrata and other focused species (Arabidopsis, O. sativa and Camptotheca acuminata). The size of the more minor gene covered was equivalent to or more than 70% of the larger gene, and the difference between the two focused genes was less than 30% to determine DiHSP20 gene duplication occurrences. Tandem duplications were characterized by two or more contiguous duplication on the same chromosome in less than 100 kb, whereas segmental duplications were considered as duplicates on distinct chromosomes or at a distance more than 100 kb on the same chromosome (Kan et al., 2021).
DiHSP20 gene promoter analysis and 3D protein structure
According to the default settings, the tertiary structures of DiHSP20 proteins were estimated utilizing online prediction program SWISS-MODEL (https://swissmodel.expasy.org/). The kinds, quantities, and functionality of cis-acting elements within that 1,500 bp upstream regions of the coding area of D. involucrata HSP20 genes were evaluated employing PlantCARE tools (http://bioinformatics.psb.ugent.be/webtools/plantcare/html/) obtained from the D. involucrata genome.
Expression pattern of DiHSP20 genes in different tissues and in response to heat stress
Davidia involucrata transcriptomic data was received from the NCBI public database (Accession number: PRJNA596897). DiHSP20 genes expression was examined in various tissues, including the root, bract, leaf, and flower. The NCBI transcriptome database were also used to get the expression patterns of the DiHSP20 genes that reacted to heat stress (Accession number: PRJNA524021). For analysis of the data, the transcription data was converted to log2 [transcripts per million (TPM)+1] values (Zeng et al., 2020). TBtools software was used to create a heatmap of the obtained gene expression patterns (Chen C. et al., 2020).
Plant materials and heat stress treatment
Chengdu (102°54′E; 30°05′N) in Sichuan province, China, provided fresh and mature D. involucrata propagules. The propagules were grown in plastic pots with sand in a growth chamber (25°C temperature, 75% humidity, 14 h light/10 h darkness photoperiod) and irrigated weekly with 1/2-strength Hoagland’s nutritional solution. The seedlings were developed under heat stress treatment (42°C) for 0, 1, 6, and 12 h at the six-leaf stage. Three seedlings were included in each treatment. The leaves were sampled and instantly frozen in liquid nitrogen.
qRT-PCR validation of DiHSP20 gene expression
RNASimple Total RNA Kit (Tiangen, Beijing, China) was used to extract total RNA, and TIANScript cDNA kit (Tiangen, Beijing, China) was used to generate cDNA according to the manufacturer’s instructions. Following total RNA extraction and cDNA synthesis, quantitative analysis was carried out using a CFX96 Touch Real-Time PCR Detection System (Bio–Rad, United States) and SuperReal PreMix Plus (SYBR Green, Tiangen, China) according to the manufacturer’s instructions. The PCR program included an initial denaturation step at 95°C for 30 s, followed by 40 cycles of 95°C for 10 s, 58°C for 10 s, and 72°C for 30 s. The 2−ΔΔCT approach was used to determine the relative expression level of the focused genes (Livak and Schmittgen, 2001). All data were calculated using the expression level under heat stress divided by that under normal condition at the same time points and presented as the means ± standard error (SE) of three replicates and differences were detected using the Student’s t-test. Asterisk (* or **) indicate a significant difference at p < 0.05 or 0.01, respectively. Supplementary Table S2 lists all of the primers utilized.
Results
HSP20 proteins in D. involucrata
A HMM search of the D. involucrata genome database yielded seventy-three HSP20 proteins. After eliminating duplicated and insufficient sequences, as well as those with something like a molecular weight outside the 15–42 kDa region, 42 sequences were identified as HSP20 genes and given names based on their chromosomal positions. It content information on each DiHSP20 gene, including its name, identity, chromosomal position, amino acid (AA) number, genomic location, isoelectric point (pI), and molecular weight (MW) (Table 1). DiHSP20 genes have been identified on 15 chromosomes of D. involucrata. The DiHSP20 proteins had an average of 229 AAs and varied in size from 136 (DiHSP20-38) to 643 (DiHSP20-27). DiHSP20s had MWs ranging from 15.65 kDa (DiHSP20-38) to 42 kDa (DiHSP20-27), with an average of 25.03 kDa, and pI values of 4.76 (DiHSP20-23) to 9.83 (DiHSP20-25), with an average of 6.65.
DiHSP20 proteins phylogenetic analysis
A ML phylogenetic tree was generated to analyze the evolutionary connections of D. involucrata HSP20 proteins. HSP20 proteins were classified into seven subfamilies: cytosol I (CI), CIV, CV, mitochondria I (MI), MII, endoplasmic reticulum (ER), and plastid (P). The cytoplasmic subfamilies (CI through CV) were the biggest clade, with 29 members, whereas subfamilies CII and CIII had no DiHSP20s. Seven gene members were detected in the M (MI and MII) subfamilies, three in the ER subfamilies, and three in the P subfamilies, whereas no DiHSP20s were found in the PX/Po subfamily (Figure 1; Table 1).
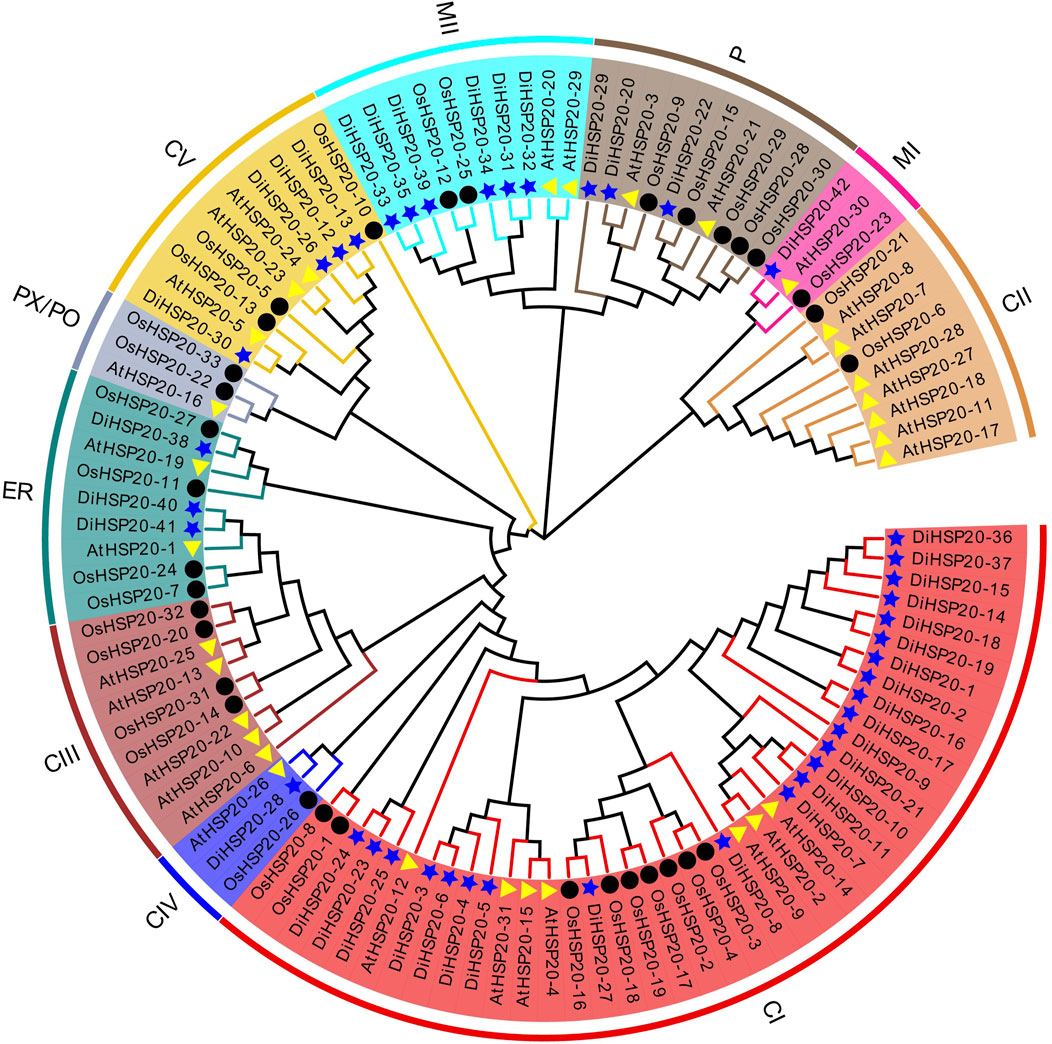
FIGURE 1. Phylogenetic analysis of Davidia involucrata, Arabidopsis, and rice HSP20s proteins. MEGA-X software was used to create a phylogenetic tree of HSP20 proteins. The ten subgroups are denoted by various colors. D. involucrata HSP20s (DiHSP20s), Arabidopsis HSP20s (AtHSP20s), and rice HSP20s (OsHSP20s) are shown by blue stars, yellow triangles, and black circles, respectively.
DiHSP20s gene structures and conserved motifs
The conserved motifs were examined by MEME to study the structural properties of the HSP20 proteins. A total of ten different motifs, numbered 1 through 10, were identified. These preserved motifs ranged in length from 6 AAs (motif 9) to 50 AAs (motif 6). The amount of conserved motifs varied from 2 to 7 for every HSP20 protein, while most DiHSP20s had 6 conserved motifs (Figure 2B).
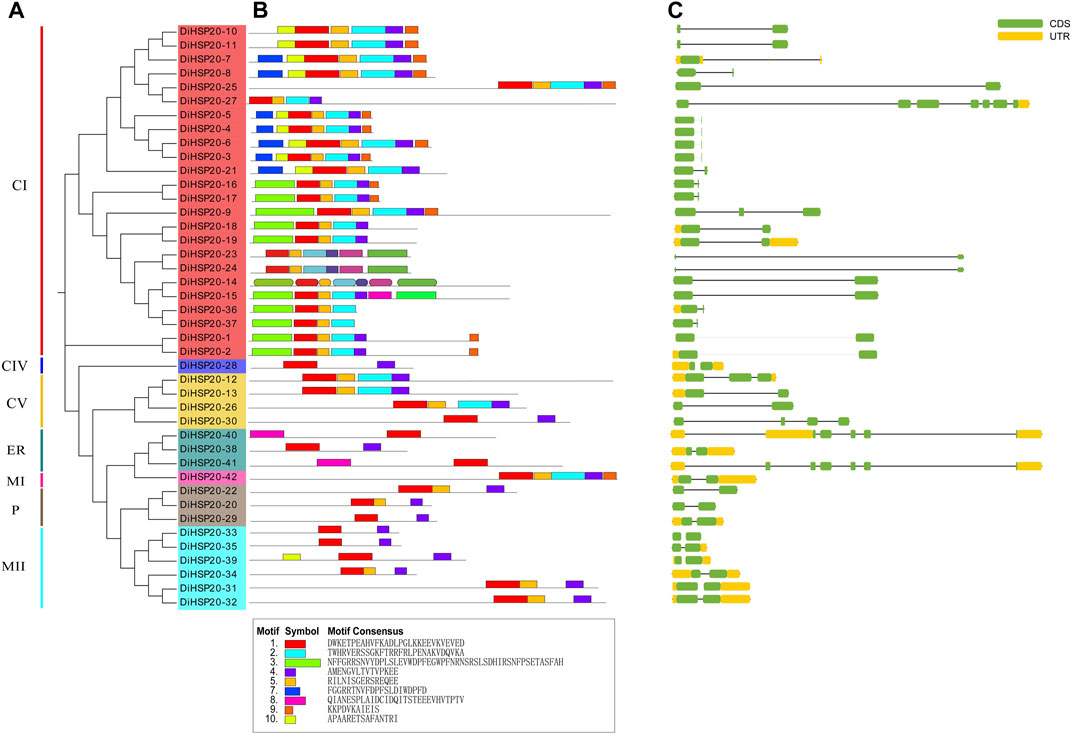
FIGURE 2. Members of the DiHSP20 family’s phylogenetic connections, structures, and motifs (A) Maximum Likelihood techniques were used to generate a phylogenetic tree comprising 42 DiHSP20 proteins. Different backdrop colors and letters are used to denote the different groupings (B) DiHSP20 proteins’ conserved motifs. Different motifs are represented by colored boxes and different numbers (C) The DiHSP20 genes’ exon/intron architecture. Green boxes, black lines, and yellow boxes correspondingly indicate exons, introns, and UTRs. With the help of TBtools, we were able to estimate the phylogenetic tree, conserved motifs, and gene structures.
The exon-intron architecture of D. involucrata HSP20 genes were studied to reveal their evolutionary relationships (Figure 2C). 23 (55 percent) of the HSP20s had no introns, 11 (26 percent) had two introns, 6 (14 percent) had one intron, and two genes (DiHSP20-7 and DiHSP20-40) had three introns. Genes with comparable exon-intron patterns were clustered together using gene structure analysis (Figures 2A,C).
The DiHSP20 proteins’ homology modeling
Protein homology modeling was done utilizing a SWISS-MODEL platform to determine a suitable theoretical structure of the DiHSP20s. Figure 3 shows the expected 3D structures. Every DiHSP20 protein was programmatically searched for a template in the program, and then a model was generated utilizing the template. According to their structural similarities, all proteins may be grouped into seven categories (groups A through G). The most proteins were found in Group A, which had 24. Only one protein was found in groups B (DiHSP20-28). Group C had 4 proteins. Group D and F had three proteins. Every DiHSP20 protein contained β-turn, and the protein structures of the same template were almost identical, implying that the expected findings were accurate.
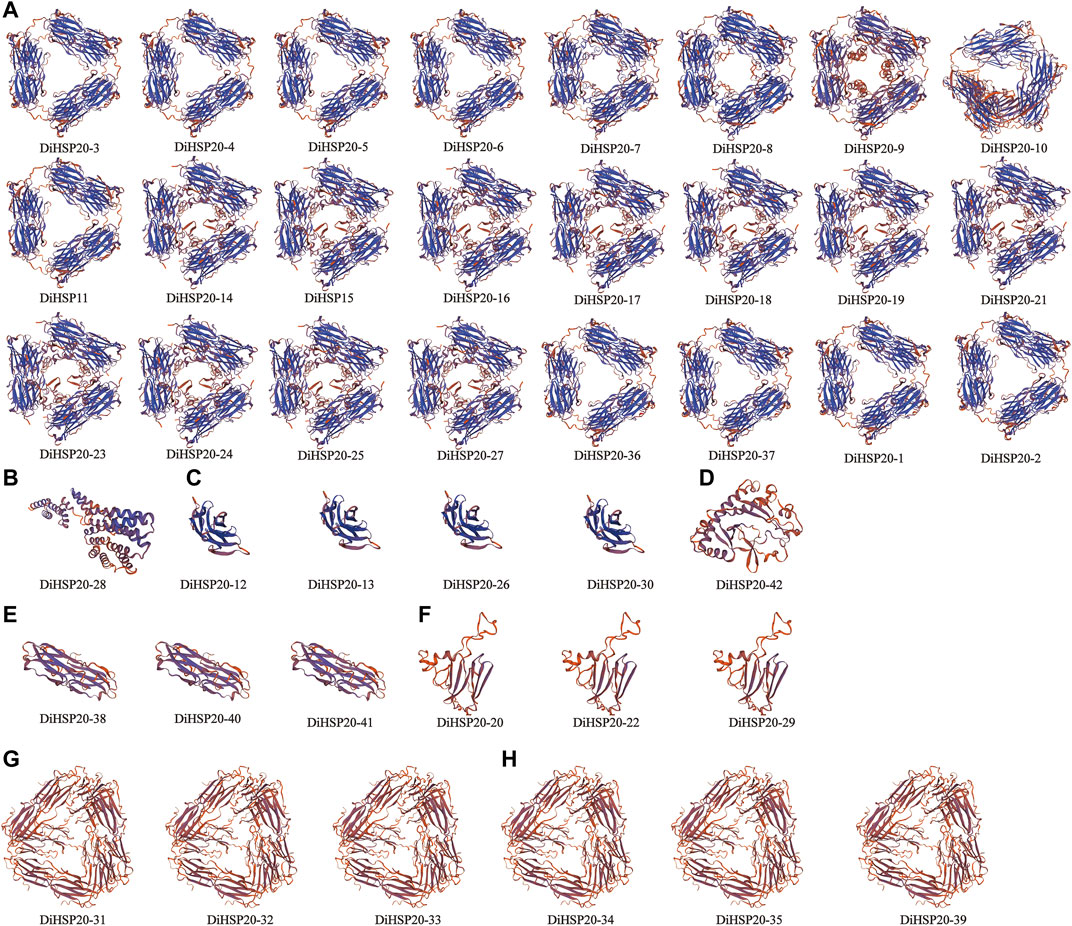
FIGURE 3. The anticipated 3-dimensional structural models of DiHSP20 proteins are depicted in cartoon form. The distinct categories are represented by capital letters (A–H).
DiHSP20s gene duplication, chromosomal location and synteny analysis
A total of 42 DiHSP20 genes were identified and distributed unevenly throughout 15 chromosomes (Chr) (Figure 4). The largest number of DiHSP20 genes per Chr was five (Chr00 and Chr16), whereas Chrs 00, 05, 08, 14, and 17 had just one gene. The investigation of gene duplication events revealed that the D. involucrata HSP20 gene family had two pairs of tandem duplication genes (DiHSP20-1/-2 and DiHSP20-40/-41) and two pairs of segmentally duplicated genes (DiHSP20-8/-14 and DiHSP20-24/-26) according to the stated criteria (Figure 4). The sequence similarity was 74.55%, 80.11%, 78.66% and 82.11%, respectively.
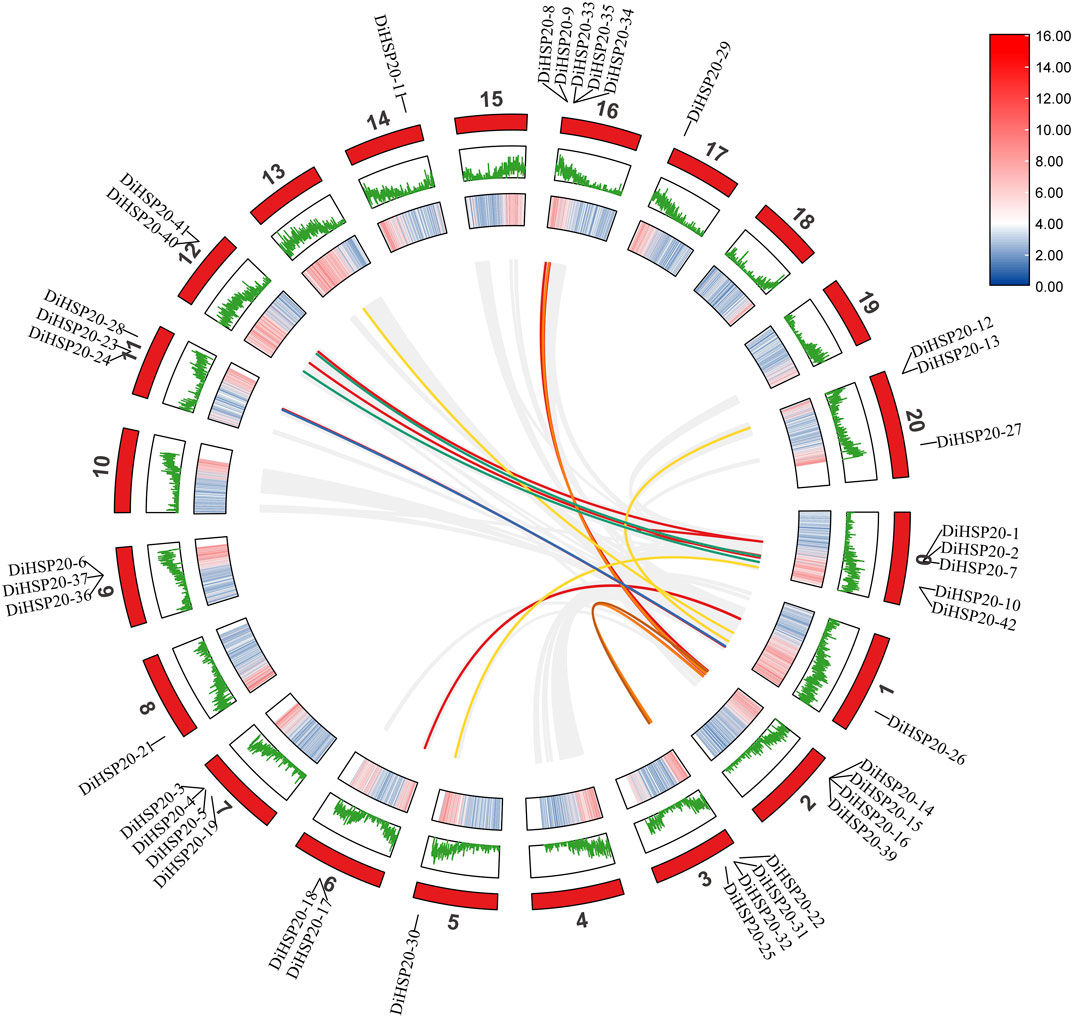
FIGURE 4. Synteny analysis between HSP20 genes of D. involucrata, as performed by MCScanX. The chromosome numbers of D. involucrata are 0–20. The outermost circle represents the length of the chromosome, the second circle represents the GC content and the third circle represents gene density. The curves indicate synteny between HSP20 genes within D. involucrata.
Orthofinder was used to discover orthologous genes and TBtools was used to create a comparative synteny map to further investigate the synteny links of DiHSP20 genes within D. involucrata (Figure 4). 30 orthologous HSP20 gene pairs have been identified based on these genes and syntenic loci in D. involucrata chromosomes, implying that the majority of HSP20 genes in D. involucrata have a similar origin and evolutionary process.
Comparative synteny maps of three related genomes (D. involucrata VS. A. thaliana, D. involucrata VS. O. sativa and D. involucrata VS. C. acuminata) were generated to better understand the evolutionary connections of the HSP20 genes with several other species. Nineteen DiHSP20 genes of D. involucrata shared a syntenic connection with Arabidopsis genes, 39 with C. acuminata genes, and just six with O. sativa genes (Figure 5). The amount of collinear gene pairings between D. involucrata and other Nyssaceae members (C. acuminata) was higher than that between more distantly related Arabidopsis or O. sativa, with D. involucrata and O. sativa having the fewest.
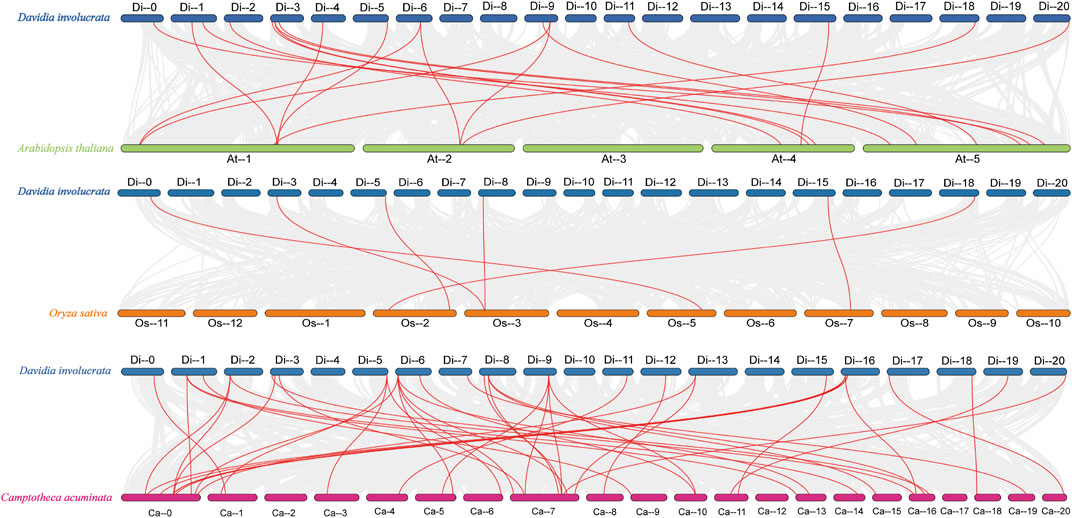
FIGURE 5. Synteny analyses of HSP20 genes between D. involucrata and three other representative plant species (Arabidopsis, rice, C. acuminata) (A) D. involucrata VS. A. thaliana. (B) D. involucrata VS. O. sativa. (C) D. involucrata VS. C. acuminata. Gray lines indicate significantly collinear blocks within and among plant genomes, while red lines highlight syntenic HSP20 gene pairs. The chromosome number is indicated at the top of each chromosome.
Promoter analysis of DiHSP20 genes
To further understand the mechanism of cis-regulatory elements in DiHSP20, cis-elements were detected in the 1.5 kb upstream of every DiHSP20’s translation start site (ATG) (Figure 6). Three categories of cis-elements, including stress responsive, plant development-related and hormone responsive elements have been discovered. The most cis-elements found among the 31 DiHSP20 genes were connected with hormone responsiveness, such as ABRE. Auxin responsiveness (AuxRR-core and TGA-element), abscisic acid responsiveness (ABRE), gibberellin responsive elements (GARE-motif, P-box, and TATC-box), and salicylic acid-responsive (TCAelement) were all found in abundance in the promoter region. The hormone responsive elements ABRE, TCAelement, and TGA-element made up the majority of the elements, whereas the AuxRR-core element was only discovered in the promoter region of five DiHSP20 genes. The category for stress-related issues abiotic stress-related elements (LTR, TC-rich repeats, and MBS) and biotic stress-related elements (LTR, TC-rich repeats, and MBS) are found in cis-elements (WUN-motif). Circadian control (circadian), palisade mesophyll cell differentiation (HD-Zip 1), endosperm expression (GCN 4 motif), meristem expression (CAT-box), flavonoid biosynthesis genes regulation (MBSI), and seed-specific regulation (RYelement) were all identified as plant development-related elements. Only the promoter regions of DiHSP20-25 and DiHSP20-41 included the HD-Zip 1 element, whereas the promoter regions of DiHSP20-28, DiHSP20-42, and DiHSP20-22 contained the RY-element element (Figure 6).
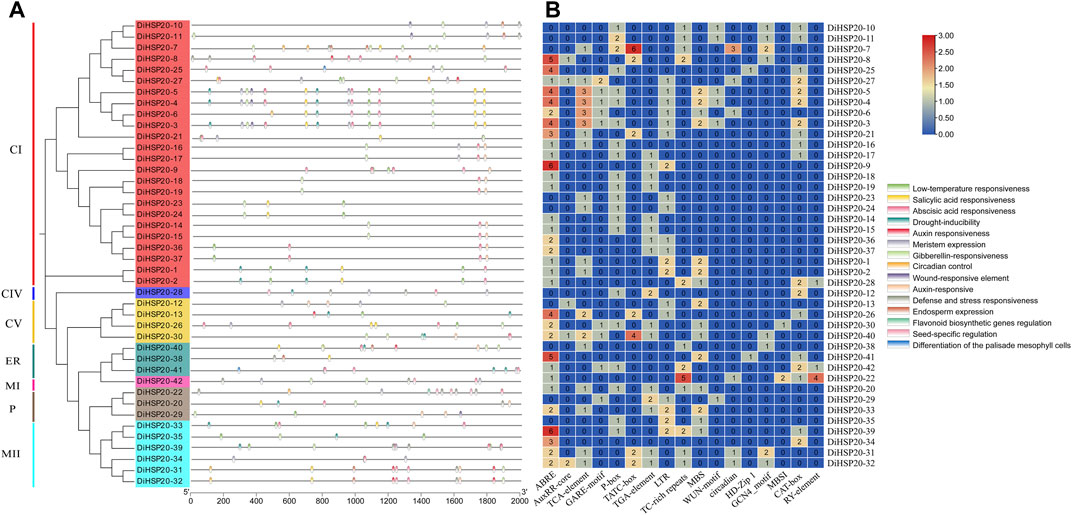
FIGURE 6. Cis-elements evaluation of the DiHSP20 gene promoter regions (A) The distinct colors and numbers in the DiHSP20 genes showed the variety of different promoter elements. (B) Different kinds of cis-elements and relative positions in every DiHSP20 gene are represented by colored blocks.
DiHSP20 gene expression patterns in different organs and in response to heat stress
The expression patterns of all 42 DiHSP20 genes were studied using a conventional transcriptome analysis approach based on publically accessible transcriptomic data from D. involucrata tissues such as bark, bracts, leaves, and flowers. Among the 42 DiHSP20s, 39 were expressed in tissues above (TPM >0). A few really DiHSP20 genes were expressed preferentially in all of the tissues studied. DiHSP20 genes were most significantly expressed in the bark, particularly DiHSP20-7, DiHSP20-16, DiHSP20-1, DiHSP20-17, and DiHSP20-30. The most highly expressed DiHSP20 genes in bract were DiHSP20-7, DiHSP20-40 and DiHSP20-41. The most highly expressed DiHSP20-34, DiHSP20-7, DiHSP20-16 and DiHSP20-17 in Flower. The most highly expressed DiHSP20 in leaf were DiHSP20-8, DiHSP20-9 and DiHSP20-7. DiHSP20-23, DiHSP20-25, and DiHSP20-33, on the other hand, were minimally expressed in any of the tissues examined (Figure 7A).
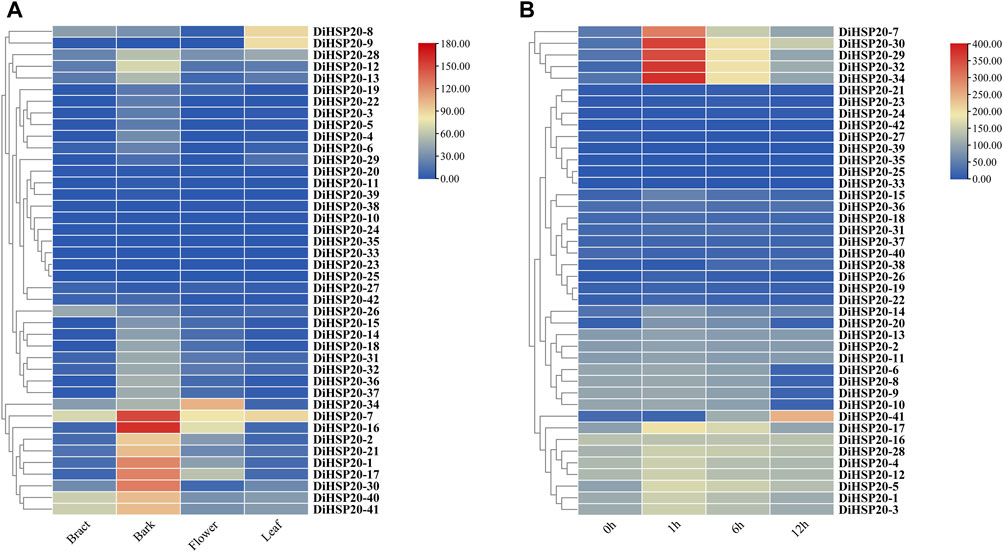
FIGURE 7. DiHSP20 gene expression in D. involucrata (A) Based on publically accessible transcriptome data, the transcript levels of the DiHSP20 genes in four tissues of D. involucrata were studied. From blue to red, the color scale reflects increasing degrees of expressiveness. (B) Based on publically accessible transcriptome data, the transcript levels of DiHSP20 genes in response to heat stress were studied.
The expression of DiHSP20s following heat treatment was investigated using publically accessible transcriptome data to study the potential roles of DiHSP20s in response to heat stress. The results showed that the expression levels of most DiHSP20 genes, especially DiHSP20-7, DiHSP20-29, DiHSP20-30, DiHSP20-32 and DiHSP20-34 were sharply upregulated after 1 hour of heat treatment, the expression of which increased more than two-fold. The expression of most DiHSP20 genes was heavily up-regulated at 1 h after heat stress, while it started to be down-regulated at 6 h and continued to decrease after 12 h. However, there were also some genes whose expression levels did not change after heat stress (Figure 7B).
Five DEGs (DiHSP20-7, DiHSP20-29, DiHSP20-30, DiHSP20-32, and DiHSP20-34) were selected for expression analysis of the candidate DiHSP20 genes that are critical for heat tolerance, and their expression levels were evaluated by qRT–PCR. Under 42°C, the expression of all of the investigated DiHSP20 genes was extremely upregulated, as shown in Figure 8. At 1 hour, the expression of all candidate genes reached a peak and the level was much higher than the control group’s. After six and 12 h, the expression decreased sequentially, but the expression at this time was also much higher than that before the heat stress.
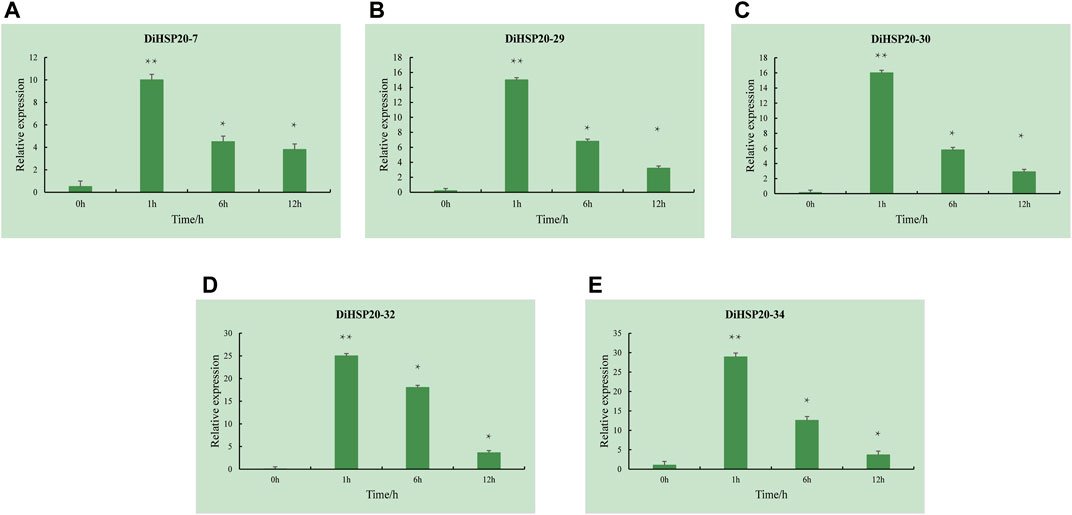
FIGURE 8. Expression profiles of 5 selected DiHSP20 genes in response to heat stress. Asterisk (p or pp) indicate a significant difference at p < 0.05 or 0.01, respectively.
Discussion
Climate variability is one of the world’s most pressing environmental and economic problems, with the potential to significantly reduce forest output and quality throughout forestal systems. Plant heat-shock proteins have been extensively found in plants and are rapidly produced in response to stress (Wang et al., 2004). Numerous studies have demonstrated the members of the HSP20 transcription factor generate heat shock proteins and play an important role in plant abiotic stress (Yu et al., 2016; Zhao et al., 2018). The roles of the HSP20 family proteins in numerous species have already been identified, such as the model species rice (Sarkar et al., 2009) and Arabidopsis (Scharf et al., 2001), along with soybean (Lopes-Caitar et al., 2013) and potato (Zhao et al., 2018). A detailed identification and characterization of the HSP20 gene in D. involucrata would offer essential information to this accumulated understanding while exposing their roles in D. involucrata stress tolerance.
It is now possible to evaluate members of the HSP20 gene family at the complete genome level thanks to the effective and efficient genome sequencing of D. involucrata (Chen Y. et al., 2020). Bioinformatics approaches were used to identify 42 HSP20 gene family members from the D. involucrata genome database. We discovered that the sample size of HSP20 genes in D. involucrata was not much different from Arabidopsis (N = 31) or rice (N = 33), but less than Gossypium hirsutum (N = 94) (Ma et al., 2016). These data point to the likelihood of a gene increase occurrence throughout the process of evolution from diploid (Arabidopsis, rice, D. involucrata) to tetraploid (G. hirsutum).
We built a phylogenetic relationships depending on the AA sequence data of D. involucrata, Arabidopsis and rice HSP20s to identify the evolutionary connections of HSP20 genes. Our phylogenetic study revealed that the HSP20 family in D. involucrata may be split into seven subfamilies, with 29 HSP20 genes in the cytoplasm belonging to CI, CIV, and CV, 7 genes in mitochondria belonging to MI and MII, 3 in the endoplasmic reticulum, and 3 in the plastids. These clustering patterns are almost similar to the distribution features of HSP20 family members in Arabidopsis (Scharf et al., 2001) and rice (Sarkar et al., 2009), showing a tight link between HSP20s from D. involucrata, Arabidopsis, and rice. This means that depending on the function of homologous genes in other species, the biological role of D. involucrata HSP20s might be inferred. Furthermore, over half of DiHSP20s were categorized into the CI-CV subfamilies, indicating that the cytoplasm may be the HSP20 family’s principal functional region in D. involucrata. Interestingly, none of the HSP20 genes belonged to the CII and CIII family, which contradicted the findings from the other focused species (Lopes-Caitar et al., 2013; Guo et al., 2015; Yao et al., 2020). The CII and CIII subfamilies may have appeared prior to D. involucrata speciation as a result of multiple gene duplication. Surprisingly, DiHSP20 members from D. involucrata were much more strongly related to members of the same subfamily from different species than to other HSP20s from the same species, indicating high level of synteny across members of the same HSP20 subfamily from various plant species.
Exon/intron structure has played a key role in the development of multiple gene families (Xu et al., 2012). Approximately 79 percent of DiHSP20 genes (mostly from the CI and CV subfamilies) contain only one intron or have no intron at all (Figure 2). This is not surprising, given that plants prefer to keep genes with no or few introns (Mattick and Gagen, 2001). Under environmental stress, the HSP20 gene family is one of the most quickly expressed genes (Sarkar et al., 2009; Yu et al., 2016). We investigated the expression levels of D. involucrata HSP20 genes in response to heat stress and discovered that the majority of HSP20 genes were significantly up-regulated.
The presence of a homologous domain was also explored in order to learn more about the development of HSP20 genes in D. involucrata. The results show that 6 conserved motifs were found in the majority of DiHSP20s, and motif 1 was found in approximately all of the proteins. Moreover, we discovered that most HSP20 genes from the same subfamilies have conserved motifs and similar gene architectures, which was similarly discovered in tomato and apple (Yu et al., 2016; Yao et al., 2020). This occurrence validated the subfamilies’ close evolutionary connection and categorization. These finding of the DiHSP20 family’s conserved motifs and gene architectures may aid in the discovery of other activities of DiHSP20 genes in D. involucrata, including in responses to various types of stressors.
Gene duplication phenomena are important in genomic reconfigurations and contractions (Vision et al., 2000), making plants possible to adapt to changing climatic conditions by increasing functional divergences (Flagel and Wendel, 2009). The 42 DiHSP20 genes were irregularly distributed on D. involucrata’s 15 chromosomes, with 10 clusters containing at least two DiHSP20 genes apiece (Figure 4). In several parts, particularly in Chrs 00 and 16, a group of family members congregated into clusters. Two sets of projected tandem duplicated HSP20 genes and two pairs of expected segmental duplicated HSP20 genes were detected, implying that the two duplication events had contributed to the expansion of the DiHSP20 family.
Furthermore, the promoter regions of DiHSP20 genes contained multiple hormone-responsive, stress-responsive, and plant development-related components (Figure 6), revealing that the HSP20 genes in D. involucrata have several or distinct roles. HSP20s have also been shown to play a critical part in the regulation of plants’ responses to environmental stress (Sarkar et al., 2009; Yu et al., 2016). The majority of the 42 DiHSP20 genes were found to be up-regulated in response to heat stress, after 1 hour of heat treatment, the expression of DiHSP20-7, DiHSP20-29, DiHSP20-30, DiHSP20-32, and DiHSP20-34 was markedly increased (Figure 7B). The expression levels of five DEGs (DiHSP20-7, DiHSP20-29, DiHSP20-30, DiHSP20-32, and DiHSP20-34) were assessed using qRT–PCR to corroborate the potential DiHSP20 genes that are crucial for heat tolerance. It is worth mentioning that after 1 h of heat stress, the relative expression levels of the 5 HSP20 genes were substantially up-regulated. These genes may play a role in the heat stress biological pathway and could be exploited as candidate genes for D. involucrata heat resistance breeding.
Conclusion
A genome-wide analysis of HSP20 proteins in D. involucrata was undertaken in this work, and 42 DiHSP20 genes were found. According to their evolutionary links, the 42 DiHSP20 genes are unequally distributed across 16 chromosomes and were grouped into seven subfamilies. The fundamental properties, genome location, gene architectures, homologous motifs, gene duplication phenomena, and cis-elements of the 42 genes were studied, to provide a critical understanding of the HSP20 gene family’s evolutionary relationships. The expression of DiHSP20 genes was investigated using qRT-PCR, and the findings indicated that heat stress up-regulated the expression of 5 candidate D. involucrata HSP20 genes. Our findings offer a foundation for identifying key potential HSP20 genes implicated in D. involucrata heat stress responses (Yang et al., 2008).
Data availability statement
The datasets presented in this study can be found in online repositories. The names of the repository/repositories and accession number(s) can be found in the article/Supplementary Material.
Author contributions
ZH performed the experiments and wrote the study, AL designed the research, Cb H revise the manuscript.
Funding
Financial support for this research was provided by the Fundamental Research Funds of Sichuan Provincial Science Program Project (2022NSFSC1141), the Jiangsu Forestry Science and Technology Innovation and Promotion Project (LYKJ[2021]02), and the PhD Fundamental Research Funds of SuZhou Polytechnic Institute of Agriculture (BS[2022]08).
Conflict of interest
The authors declare that the research was conducted in the absence of any commercial or financial relationships that could be construed as a potential conflict of interest.
Publisher’s note
All claims expressed in this article are solely those of the authors and do not necessarily represent those of their affiliated organizations, or those of the publisher, the editors and the reviewers. Any product that may be evaluated in this article, or claim that may be made by its manufacturer, is not guaranteed or endorsed by the publisher.
Supplementary material
The Supplementary Material for this article can be found online at: https://www.frontiersin.org/articles/10.3389/fgene.2022.1011676/full#supplementary-material
References
Basha, E., O’Neill, H., and Vierling, E. (2012). Small heat shock proteins and α-crystallins: Dynamic proteins with flexible functions. Trends biochem. Sci. 37, 106–117. doi:10.1016/j.tibs.2011.11.005
Chang, Y. Y., Liu, H. C., Liu, N. Y., Hsu, F. C., and Ko, S. S. (2006). Arabidopsis Hsa32, a novel heat shock protein, is essential for acquired thermotolerance during long recovery after acclimation. Plant Physiol. 140, 1297–1305. doi:10.1104/pp.105.074898
Chen, Y., Ma, T., Zhang, L., Kang, M., Zhang, Z., Zheng, Z., et al. (2020a). Genomic analyses of a “living fossil”: The endangered dove‐tree. Mol. Ecol. Resour. 20 (3), 756–769. doi:10.1111/1755-0998.13138
Chen, C., Chen, H., Zhang, Y., Thomas, H. R., Frank, M. H., He, Y., et al. (2020b). TBtools: An integrative Toolkit developed for interactive analyses of big biological data. Mol. Plant 13, 1194–1202. doi:10.1016/j.molp.2020.06.009
Cui, F., Taier, G., Wang, X., and Wang, K. (2021). Genome-wide analysis of the HSP20 gene family and expression patterns of HSP20 genes in response to abiotic stresses in cynodon transvaalensis. Front. Genet. 12, 732812. doi:10.3389/fgene.2021.732812
De Souza Resende, J. S., Benedito dos Santos, T., and Graciele Hülse de Souza, S. (2022). Small heat shock protein (Hsp20) gene family in Phaseolus vulgaris L.: Genome-wide identification, evolutionary and expression analysis. Plant gene. 31, 100370. doi:10.1016/j.plgene.2022.100370
Flagel, L. E., and Wendel, J. F. (2009). Gene duplication and evolutionary novelty in plants. New Phytol. 183, 557–564. doi:10.1111/j.1469-8137.2009.02923.x
Guo, M., Liu, J. H., Lu, J. P., Zhai, Y. F., Wang, H., Gong, Z. H., et al. (2015). Genome-wide analysis of the CaHsp20 gene family in pepper: Comprehensive sequence and expression profile Analysis under heat stress.
Gupta, S. C., Sharma, A., Mishra, M., Mishra, R. K., and Chowdhuri, D. K. (2010). Heat shock proteins in toxicology: How close and how far? Life Sci. 86 (11/12), 377–384. doi:10.1016/j.lfs.2009.12.015
Haslbeck, M., and Vierling, E. (2015). A first line of stress defense: Small heat shock proteins and their function in protein homeostasis. J. Mol. Biol. 427, 1537–1548. doi:10.1016/j.jmb.2015.02.002
Hu, Y., Zhang, T., Liu, Y., Li, Y., Wang, M., Zhu, B., et al. (2021). Pumpkin (Cucurbita moschata) HSP20 gene family identification and expression under heat stress[J]. Front. Genet. 12, 2062. doi:10.3389/fgene.2021.753953
Huang, J., Hai, Z., Wang, R., Yu, Y., Chen, X., Liang, W., et al. (2022). Genome-wide analysis of HSP20 gene family and expression patterns under heat stress in cucumber (Cucumis sativus L.). Front. Plant Sci. 13, 968418. doi:10.3389/fpls.2022.968418
Ji, X. R., Yu, Y. H., Ni, P. Y., Zhang, G. H., and Guo, D. L. (2019). Genome-wide identification of small heat-shock protein (HSP20) gene family in grape and expression profile during berry development. BMC Plant Biol. 19, 433. doi:10.1186/s12870-019-2031-4
Kan, J., Gao, G., He, Q., Gao, Q., Jiang, C., Ahmar, S., et al. (2021). Genome-wide characterization of WRKY transcription factors revealed gene duplication and diversification in populations of wild to domesticated barley. Int. J. Mol. Sci. 22, 5354. doi:10.3390/ijms22105354
Kandoth, P. K., Ithal, N., Recknor, J., Maier, T., Nettleton, D., Baum, T. J., et al. (2011). The soybean Rhg1 locus for resistance to the soybean cyst nematode Heterodera glycines regulates the expression of a large number of stress- and defense-related genes in degenerating feeding cells. Plant Physiol. 155, 1960–1975. doi:10.1104/pp.110.167536
Kumar, S., Stecher, G., Li, M., Knyaz, C., and Tamura, K. (2018). Mega X: Molecular evolutionary Genetics analysis across computing platforms. Mol. Biol. Evol. 35, 1547–1549. doi:10.1093/molbev/msy096
Li, J., Zhang, J., Jia, H., Li, Y., Xu, X., Wang, L., et al. (2016). The Populus trichocarpa PtHSP17.8 involved in heat and salt stress tolerances. Plant Cell Rep. 35, 1587–1599. doi:10.1007/s00299-016-1973-3
Livak, K. J., and Schmittgen, T. D. (2001). Analysis of relative gene expression data using real-time quantitative PCR and the 2(-Delta Delta C(T)) Method. Methods 25, 402–408. doi:10.1006/meth.2001.1262
Lopes-Caitar, V. S., de Carvalho, M. C., Darben, L. M., Kuwahara, M. K., Nepomuceno, A. L., Dias, W. P., et al. (2013). Genome-wide analysis of the hsp 20 gene family in soybean: Comprehensive sequence, genomic organization and expression profile Analysis under abiotic and biotic stresses. BMC Genomics 14 (1), 577. doi:10.1186/1471-2164-14-577
Ma, W., Zhao, T., Li, J., Liu, B., Fang, L., Hu, Y., et al. (2016). Identification and characterization of the GhHsp20 gene family in Gossypium hirsutum. Sci. Rep. 6, 32517. doi:10.1038/srep32517
Maimbo, M., Ohnishi, K., Hikichi, Y., Yoshioka, H., and Kiba, A. (2007). Induction of a small heat shock protein and its functional roles in nicotiana plants in the defense response against ralstonia solanacearum. Plant Physiol. 145 (4), 1588–1599. doi:10.1104/pp.107.105353
Manchester, S. R., Chen, Z. D., Lu, A. M., and Uemura, K. (2009). Eastern Asian endemic seed plant genera and their paleogeographic history throughout the Northern Hemisphere. J. Syst. Evol. 47 (1), 1–42. doi:10.1111/j.1759-6831.2009.00001.x
Mattick, J. S., and Gagen, M. J. (2001). The evolution of controlled multitasked gene networks: The role of introns and other noncoding RNAs in the development of complex organisms. Mol. Biol. Evol. 18 (9), 1611–1630. doi:10.1093/oxfordjournals.molbev.a003951
Murakami, T., Matsuba, S., Funatsuki, H., Kawaguchi, K., Saruyama, H., Tanida, M., et al. (2004). Over-expression of a small heat shock protein, sHSP17.7, confers both heat tolerance and UV-B resistance to rice plants. Mol. Breed. 13, 165–175. doi:10.1023/b:molb.0000018764.30795.c1
Sarkar, N. K., Kim, Y. K., and Grover, A. (2009). Rice sHsp genes: Genomic organization and expression profiling under stress and development. BMC Genomics 10, 393. doi:10.1186/1471-2164-10-393
Scharf, K. D., Siddique, M., and Vierling, E. (2001). The expanding family of Arabidopsis thaliana small heat stress proteins and a new family of proteins containing α-crystallin domains (acd proteins). Cell Stress Chaperones 6 (3), 225–237. doi:10.1379/1466-1268(2001)006<0225:tefoat>2.0.co;2
Sun, L., Liu, Y., Kong, X., Zhang, D., Pan, J., Zhou, Y., et al. (2012). ZmHSP16.9, a cytosolic class I small heat shock protein in maize (Zea mays), confers heat tolerance in transgenic tobacco. Plant Cell Rep. 31, 1473–1484. doi:10.1007/s00299-012-1262-8
Swindell, W. R., Huebner, M., and Weber, A. P. (2007). Transcriptional profiling of Arabidopsis heat shock proteins and transcription factors reveals extensive overlap between heat and non-heat stress response pathways. BMC Genomics 8, 125. doi:10.1186/1471-2164-8-125
Thompson, J. D., Higgins, D. G., and Gibson, T. J. (1994). Clustalw: Improving the sensitivity of progressive multiple sequence alignment through sequence weighting, position-specific gap penalties and weight matrix choice. Nucleic Acids Res. 22, 4673–4680. doi:10.1093/nar/22.22.4673
Vision, T. J., BrownTanksley, D. G. S. D., and Tanksley, S. D. (2000). The origins of genomic duplications in Arabidopsis. Science 290, 2114–2117. doi:10.1126/science.290.5499.2114
Wang, W., Vinocur, B., Shoseyov, O., and Altman, A. (2004). Role of plant heat-shock proteins and molecular chaperones in the abiotic stress response. Trends Plant Sci. 9 (5), 244–252. doi:10.1016/j.tplants.2004.03.006
Wang, Y., Tang, H., DeBarry, J. D., Tan, X., Li, J., Wang, X., et al. (2012). MCScanX: A Toolkit for detection and evolutionary analysis of gene synteny and collinearity. Nucleic Acids Res. 40 (7), e49. doi:10.1093/nar/gkr1293
Waters, E. R., Lee, G. J., and Vierling, E. (1996). Evolution, structure and function of the small heat shock proteins in plants. J. Exp. Bot. 47, 325–338. doi:10.1093/jxb/47.3.325
Waters, E. R. (2013). The evolution, function, structure, and expression of the plant sHsps. J. Exp. Bot. 64 (2), 391–403. doi:10.1093/jxb/ers355
Xu, G., Guo, C., Shan, H., and Kong, H. (2012). Divergence of duplicate genes in exon-intron structure. Proc. Natl. Acad. Sci. U. S. A. 109, 1187–1192. doi:10.1073/pnas.1109047109
Yamori, W., Hikosaka, K., and andWay, D. A. (2014). Temperature response of photosynthesis in C3, C4, and CAM plants: Temperature acclimation and temperature adaptation. Photosynth. Res. 119, 101–117. doi:10.1007/s11120-013-9874-6
Yang, S., Zhang, X., Yue, J. X., Tian, D., and Chen, J. Q. (2008). Recent duplications dominate NBS-encoding gene expansion in two woody species. Mol. Genet. Genomics 280, 187–198. doi:10.1007/s00438-008-0355-0
Yao, F., Song, C., Wang, H., Song, S., Jiao, J., Wang, M., et al. (2020). Genome-wide characterization of the HSP20 gene family identifies potential members involved in temperature stress response in apple. Front. Genet. 11, 609184. doi:10.3389/fgene.2020.609184
Yu, J., Cheng, Y., Feng, K., Ruan, M., Ye, Q., Wang, R., et al. (2016). Genome-wide identification and expression profiling of tomato Hsp20 gene family in response to biotic and abiotic stresses. Front. Plant Sci. 7, 1215. doi:10.3389/fpls.2016.01215
Zeng, Z., Lu, J., Wu, D., Zuo, R., Li, Y., Huang, H., et al. (2020). Poly( ADP -ribose) glycohydrolase silencing-mediated H2B expression inhibits benzo(a)pyreneinduced carcinogenesis. Environ. Toxicol. 36, 291–297. doi:10.1002/tox.23034
Keywords: Davidia involucrata, heat shock protein 20, heat stress, gene family, defense mechanisms
Citation: Hou Z, Li A and Huang C (2022) Genome-wide identification, characterization and expression of HSP 20 gene family in dove. Front. Genet. 13:1011676. doi: 10.3389/fgene.2022.1011676
Received: 04 August 2022; Accepted: 15 September 2022;
Published: 04 October 2022.
Edited by:
Aditya Pratap, Indian Institute of Pulses Research (ICAR), IndiaReviewed by:
Chandra Mohan Singh, Banda University of Agriculture and Technology, IndiaAwdhesh Kumar Mishra, Yeungnam University, South Korea
Copyright © 2022 Hou, Li and Huang. This is an open-access article distributed under the terms of the Creative Commons Attribution License (CC BY). The use, distribution or reproduction in other forums is permitted, provided the original author(s) and the copyright owner(s) are credited and that the original publication in this journal is cited, in accordance with accepted academic practice. No use, distribution or reproduction is permitted which does not comply with these terms.
*Correspondence: Changbing Huang, cbhuang@szai.edu.cn
† These authors have contributed equally to this work