- 1Guangdong Provincial Key Laboratory of Animal Molecular Design and Precise Breeding, School of Life Science and Engineering, Foshan University, Foshan, China
- 2National Engineering Laboratory for Animal Breeding, Key Laboratory of Animal Genetics, Breeding and Reproduction, Ministry of Agriculture and Rural Affairs, College of Animal Science and Technology, China Agricultural University, Beijing, China
Ancient DNA is vitally important in evolutionary research, and obtaining authentic ancient DNA sequences is critical for a proper analysis. However, it is difficult to acquire amplicons accurately and efficiently from ancient DNA templates using current techniques. Here, we established a loop-primer-mediated amplification method (L-PCR) to obtain target ancient DNA sequences with high accuracy and efficiency. The method was tested using 66 ancient samples (including 27 pig bones or teeth and 39 chicken bones) and serially diluted modern animal DNA templates. Compared to nested PCR, L-PCR was proven to be more efficient and accurate and could obtain more amplicons from both ancient pig samples and chicken bones and detect as low as 10−3 ng/μl modern pig template DNA. The efficiency was at least 100-fold that of the nested PCR. The results suggest that L-PCR is advantageous for obtaining authentic DNA sequences from poorly preserved or recalcitrant ancient specimens.
Introduction
Ancient DNA provides a profound perspective on the evolution and origin of organisms (Krause et al., 2006; Noonan et al., 2006; Larson et al., 2010; Thalmann et al., 2013; Zhang et al., 2013; Yang et al., 2020; Zhang et al., 2020). Discreetly acquiring authentic DNA sequences is a critical requirement for reliable phylogenetic deduction and evolutionary tracing. However, relentless processes such as oxidation, deamination, and depurination modify the nitrous bases and the sugar-phosphate backbone of the DNA (Hofreiter et al., 2001a; Hofreiter et al., 2001b; Uysal Yoca Ö. et al., 2021), invariably reducing the copy number of DNA recovered from ancient samples. These base lesions accumulate in DNA over time and may cause nucleotide misincorporations when ancient DNA sequences are replicated in vitro (Stiller et al., 2006; Gutaker and Burbano, 2017). Nuclear mitochondrial pseudogenes (NUMTs), which are generally analogous to homologous mitochondrial DNA (mtDNA) sequences, act as potential interference in ancient mtDNA analyses when they are inadvertently co-amplified with authentic mtDNA sequences (den Tex et al., 2010; Diroma et al., 2021). Since the first ancient DNA research was implemented (Pääbo, 1985), nested PCR has become a basic augmentation tool for retrieving low copy number DNA, along with uracil-N-glycosylase (UNG) pretreatment, which eliminates damaged templates (Willerslev and Cooper, 2005). However, in previous ancient DNA research, primer carryover from the first-stage PCR into the second-stage PCR could still have generated nonspecific amplification products, which would then require tedious authenticity verification. Therefore, the efficient and accurate retrieval of ancient DNA sequences faces a technical roadblock.
For high-fidelity amplification, complex primer design has received considerable attention. Therefore, a scheme for exploiting the loop-primer-meditated amplification mechanism was presented (Notomi et al., 2000). In particular, with the recognition of at least six conserved target DNA regions, loop-mediated isothermal amplification (LAMP) (Notomi et al., 2000; Tomita et al., 2008; Wong et al., 2018; Kashir and Yaqinuddin, 2020) and isothermal polymerase chain reaction (isoPCR) (Søe et al., 2013) have been extensively applied as diagnostic tools for nucleic acid tests (Huy et al., 2012; Murray et al., 2013; Park et al., 2018; Vichaibun and Kanchanaphum, 2020). This amplification mechanism can effectively and specifically boost outputs by forming stem-loop products with several target repeats.
Herewith, we supposed that incorporating complex primers into PCR reaction was helping to efficiently capture authentic poorly preserved DNA sequences. Therefore, we introduced loop primers to enrich ancient DNA templates in the PCR system, which we termed L-PCR. The theoretical procedure of L-PCR assay is illustrated in Figure 1. After optimizing the reaction system using modern pig and chicken samples for each primer set, the L-PCR assay was applied to amplify 66 ancient samples (including 27 pig samples and 39 chicken bones) and serially diluted modern pig DNA templates. The results were compared with those of nested PCR to evaluate the efficiency and accuracy of the newly created method.
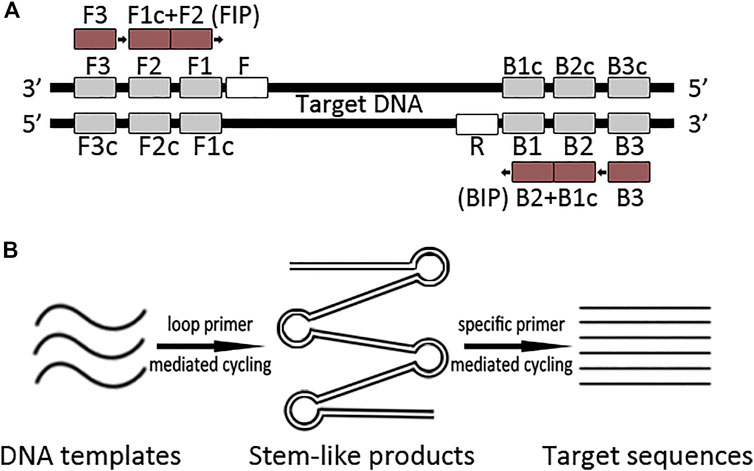
FIGURE 1. Schematic representation of the L-PCR assay. (A) Primers of the L-PCR assay contain a loop-primer set of four conserved primers which recognize six target DNA regions and a singleplex PCR primer set. (B) The L-PCR assay comprises two stages: the first stage uses a loop-primer set for specifically enriching a broader target region which comprises the target region; the second stage is a routine PCR process that uses a specific primer pair to obtain the target sequence.
Materials and methods
Modern sample and serially diluted DNA templates preparation
Five modern pig and five modern chicken blood samples were collected, respectively. The modern DNA were extracted following the standard protocol of the phenol-chloroform method. The DNA extractions were then quantified using Qubit 4 (Thermo Fisher Scientific) and applied to the subsequently optimization of amplification reaction for each primer set. The DNA samples of high quality and proper concentration were used to produce ten-fold dilution series, with concentrations ranging from 102 ng/μl to 10−6 ng/μl. Serially diluted templates were used to evaluate the efficiency of the L-PCR assay.
Ancient sample collection and DNA extraction
A total of 27 ancient pig bones or teeth and 39 ancient chicken bones were used in this study. To be specific, the pig samples were collected from three archeological sites from the middle Yellow River region including the ∼10,000-year-old Nanzhuangtou site, the ∼7,400-year-old Cishan site, and the ∼4,500-year-old Nanwa site, one ∼4,000-year-old site from the upper Yellow River region (Lajia), one ∼4,000-year-old site from the middle Yangtze River region (Qinglongquan). The chicken samples were collected from two archeological sites from the middle Yellow River region (Nanzhuangtou and Cishan), one site from the lower Yellow River region (the ∼4,000-year-old Wangyin site), and one site from the middle Yangtze River region (the ∼2,500-year-old Jiuliandun site). Detailed ancient sample information is shown in Table 1.
Ancient DNA templates were prepared in a physically isolated laboratory. Ancient samples were prepared by cautiously cleaning the adhering soils, followed by washing with 5% sodium hypochlorite solution and double-distilled water and drying under ultraviolet irradiation. Ancient samples were then milled into powder, and 200–500 mg of powder was used for DNA extraction using the QIAamp® DNA Investigator Kit (Qiagen). Amicon® Ultra-4 (Millipore) was used to concentrate the ancient DNA solution to a volume of approximately 50 μl. The DNA extractions were quantified using Qubit 4 (Thermo Fisher Scientific).
Primer design
The published mtDNA sequences of S. scrofa (AF486871) and G. gallus (NC001323) were used to design primers for obtaining the target pig or chicken mtDNA sequences, respectively. Primers for L-PCR were designed using the online Primer Explorer V4 software (http://primerexplorer.jp/e/index.html), which contained two outer primers (F3 and B3) and two inner primers (FIP and BIP). F3 and B3 were also used as the primers for the external round of nested PCR amplification. Then a pair of primers (F and R) between F3 and B3 was designed for the second round of nested PCR and L-PCR by Primer Primier 5. After amplification, F and R were also used as sequencing primers. In total, we generated two sets of primer pairs for pig mtDNA fragments of the cytochrome b gene (Cytb) and control region (CR), and three sets of chicken mtDNA fragments of the cytochrome oxidase I gene (CO I) and two fragments of the control region (CR). All primer sequences are shown in Table 2.
Optimization of amplification reaction
Based on Notomi et al. (2000) and Tomita et al. (2008), the reaction system conditions including the Mg2+ concentrations (1.0 mM–3.0 mM), Taq DNA polymerase concentrations (0.5 U–2.0 U), concentration ratios between inner to outer primers (2:1 to 8:1), the annealing temperature for each primer set (55°C–70°C), and the input volume of first stage PCR products to the second stage amplifications (0.1 μl–1.0 μl), were detected to determine the optical reaction systems for both L-PCR and nested PCR. With the optical parameters, a singleplex PCR using real-time fluorescent SYBR Green reagents (Applied Biosystems) was conducted to quantitatively compare the efficiency of L-PCR to nested PCR on serially diluted DNA templates. Amplifications of the extraction blank controls and PCR blank controls were performed in all experiments to monitor contamination.
Ancient DNA amplification
Ancient DNA extractions were amplified using both L-PCR assay and nested PCR. This resembled the optical reaction system except for special details, including 3 μl of ancient DNA extracts used in the PCR ingredients and 1U Uracil-N-glycosylase (UNG) was added to eliminate uracil from ancient DNA templates. All first-stage L-PCR and PCR reactions were initially incubated at 37°C for 10 min to facilitate the UNG, and were then performed under the cycling conditions with the optimal annealing temperatures. The subsequent singleplex PCR reactions were also executed under the aforementioned cycling conditions with their optimal annealing temperatures. All secondary amplified products detected by 2.5% agarose gel electrophoresis. The positive products were subjected to direct sequencing on an ABI 3730XL automated DNA sequencer using the BigDye Terminator v3.1 Kit (Applied Biosystems, Foster City, United States) and only samples with clear and intact sequencing histogram were regarded as successively achieved. Partial ancient samples were sent to the Ancient DNA Laboratory of the Research Center for Chinese Frontier Archaeology at Jilin University for independent replication.
Clone and sequencing of ancient DNA PCR products
To test the authenticity of L-PCR amplification of ancient DNA, the amplification products of two positive ancient samples were analyzed by agarose gel electrophoresis (1% w/v) and were subsequently purified using Agarose gel Purification and Recovery Kit (Aidlab Co., Beijing, China). After that, the purified PCR fragments were ligated into the pBLUE-T vector (Aidlab Co., Beijing, China) to get multiplex clones, and eight clones for each sample were sequenced on an ABI 3730XL automated DNA sequencer (Applied Biosystems).
Statistical analyses
The mean ± standard deviation was calculated for all data. The Wilcoxon test were used to compare the Ct value using SAS 9.2 (SAS Inst. Inc.). All values with p < 0.05 were regarded as being statistically significant.
Results
Establishment and optimization of amplification reaction system
By using modern pig and chicken DNA templates to determine the optical reaction system conditions for each primer set, the results showed that in a 25 μl reaction system 1.5 mM Mg2+ concentrations and 1U AmpliTaq Gold polymerase were suitable for all reactions. When the concentration of the inner primer was five times to the outer primer, it was expected to yield the most amplicons, and 0.2 μM forward and reverse outer primers was recommended in a 25 μl reaction system. After that, the optical annealing temperature for each primer set was determined (Table 2), which suggested that 64°C–66°C were suitable for all loop mediated primer sets and 65°C was chosen as the optical annealing temperature for the first stage L-PCR reaction of all primers. Moreover, serial volume products of the first stage amplification from 0.1 μl to 1.0 μl were input as the start template of the second stage amplification, and the result suggested 0.3 μl product input could yield most enriched targeting amplicons. To be specific, the optical reaction systems for L-PCR and nested PCR were descripted below.
For the L-PCR assay, the first-stage amplification using the loop primer set was carried out in a 25 μl reaction system containing 1U AmpliTaq Gold polymerase (Applied Biosystems), 1× PCR buffer concluded 3 mM Mg2+, 2 mM dNTPs, 1 μM forward and reverse inner primers (FIP and BIP), 0.2 μM forward and reverse outer primers (F3 and B3), and 0.5 μl DNA templates. The second-stage amplification was a singleplex PCR, in which 0.3 μl L-PCR product was used as the template, specific primers (F and R) were 0.5 μM each, and other ingredients were identical to the first stage. The L-PCR reaction was performed using the following cycling program: 94°C for 5 min, followed by 40 cycles of 94°C for 30 s, 65°C for 40 s, 72°C for 30 s, and a final extension of 10 min at 72°C. The subsequent singleplex PCR was performed under the same conditions except for the optical annealing temperature for each primer pair. For the nested PCR, the first-phase PCR was conducted with F3 and B3 primers using the optical annealing temperature for each primer set and the same ingredients as those used in L-PCR. For the second-phase PCR, 0.3 μl PCR product was added as a DNA template, and all other ingredients were identical to those in the first-phase PCR. The cycling programs were identical to those of singleplex PCR of L-PCR.
Efficiency of L-PCR compared to nested PCR using serially diluted templates
A serially diluted DNA templates was used to test the enrichment efficiency of L-PCR assay and nested PCR with pig Cytb primer set. The results showed that good quality amplification products could be obtained by L-PCR for DNA templates with initial concentration of no less than 10° ug/μl, and even certain amplification products could be obtained with initial DNA templates concentration from 102–10° pg/μl DNA templates by agarose gel analyses. Meanwhile, the results revealed that the nested PCR was able to achieve high quality amplification products with initial DNA templates concentration from 102–102 ug/μL DNA templates.
Moreover, the comparison of the quantitative determinations revealed that the L-PCR assay could reach the yield plateau at a lower Ct value (p < 0.01) than the nested PCR method at a template DNA concentration range of 102 ng/μl to 10° ug/μl (Figure 2A). Within the limited 40 reaction cycles, L-PCR amplification with 10° ug/μl DNA templates reached a yield of 1,000 relative fluorescence units (RFUs), while at least 10° ng/μl DNA templates were needed for nested PCR to meet the same threshold (Figure 2B). Further, the L-PCR assay with an initial DNA concentration of 10° ng/μl could almost meet the equipotent yields of nested PCR with 102 ng/μl DNA templates. These results demonstrated that the L-PCR assay could successfully detect total DNA in concentrations as low as 10° ug/μl and its productivity is at least 100-fold higher than that of conventional nested PCR.
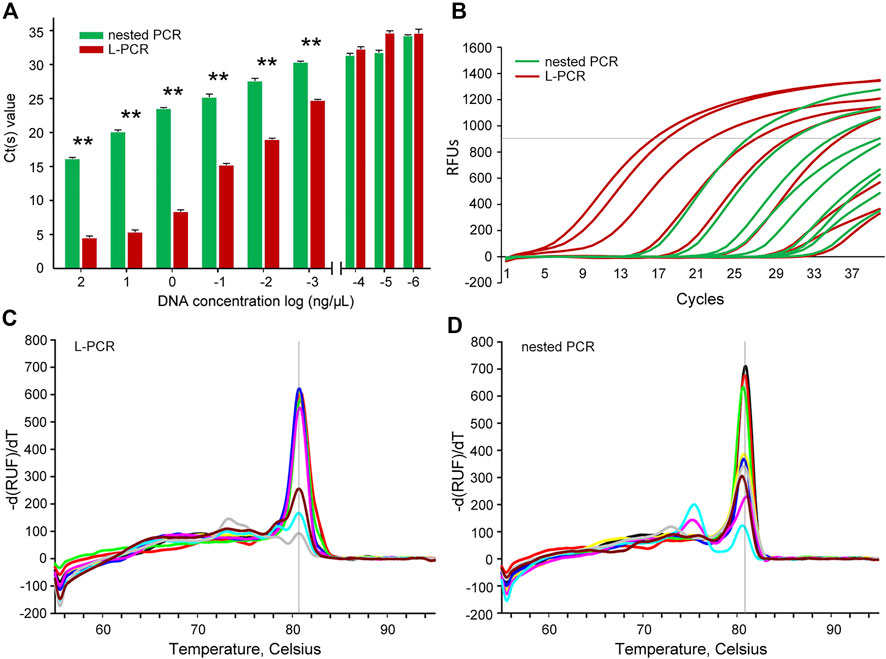
FIGURE 2. Analytical efficiency and accuracy between the nested PCR and L-PCR assay. (A,B) The Ct value comparison between the nested PCR and the L-PCR assay with serially diluted templates. (C) The melt temperatures for the L-PCR assay. (D) The melt temperatures for the nested PCR assay. The asterisk (*) indicates p < 0.05.
Determination of the accuracy of L-PCR assay
The melt curve analyses were carried out to detect the specificity of amplification at such low concentrations. The melt temperatures of the L-PCR second-stage amplification were clustered as one single sharp peak at approximately 80°C for the start DNA concentration of 102 ng/μl to 10° ug/μl (Figure 2C), suggesting high fidelity of L-PCR assay. When the template concentration of L-PCR was in the case of undetectable dilutions, the melt temperature curves began to appear multi-peak and the peak value was low (Figure 2C), which suggested that the non-specific amplification increased, and reliability decreased. In contrast, the nested PCR had high amplification specificity only when the template concentration is 10° ng/μL or higher (Figure 2D). When the template concentration was lower than 10° ng/μl, the curve of melting temperature had two obvious peaks (Figure 2D), indicating that its specificity decreased with lower start template concentration than 10° ng/μl. This result supported the assumption that with multiple recognition regions on the target sequence, the L-PCR assay could perform well with rigorous and precise amplification beyond its limit of detection. For this, it was suggested that melt temperatures were not necessary to determine the fidelity while using the L-PCR assay, similar to the nested PCR (Uemura et al., 2008; Spielmann et al., 2019).
Ancient sample amplification using nested PCR method and L-PCR assay
In order to test the practical effect of L-PCR method in ancient DNA research, it was applied to amplify target sequences of Cytb gene and control region from ancient pig samples, and COI gene and control region fragments from ancient chicken samples. For both L-PCR and nested PCR, a weak correlation between the quality of ancient DNA templates and success of amplification. However, the results showed that the yield ratio did not decrease consistently with the increasing age in the overall comparison (Figure 3). Despite this, more sequences were achieved from specimens of ancient pigs than ancient chickens because chicken remains lack teeth and have a thinner bone wall. And both the numbers and ratios of the samples obtained using L-PCR assay were higher than the nested PCR, regardless of whether the DNA of pig and chicken samples came from as DNA templates (Figure 3).
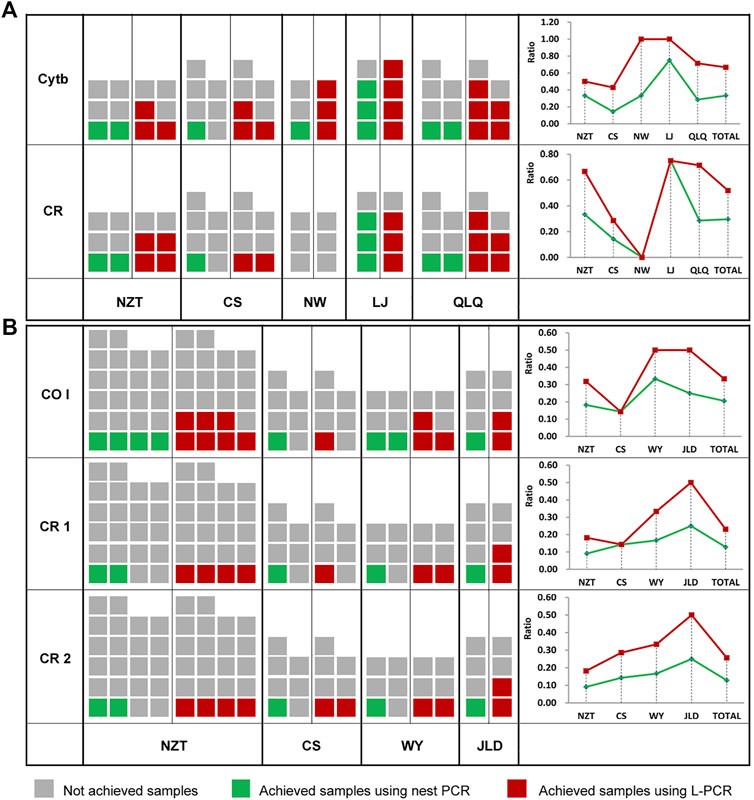
FIGURE 3. Comparison of ancient sample amplification using a nested PCR method and the L-PCR assay. (A) Numbers and ratios of ancient pig samples (B) Numbers and ratios of the ancient chicken samples.
Clone and sequencing of ancient DNA PCR products
Two ancient pig samples from the ∼4,000-year-old Qinglongquan Site were amplified by L-PCR with Cytb gene primer. A total of eight clones for each sample were sequenced and the multiple alignment showed that only a few damage sites were found in different clones of the two samples and the consensus sequence was identical to the endogenous sequence (Figure 4), indicating that L-PCR had high fidelity to ancient DNA research. However, since some damage sites did exist in different clones, it should be recommend adding proper UNG enzyme in the first amplification reaction system of L-PCR to eliminate possible damage templates.
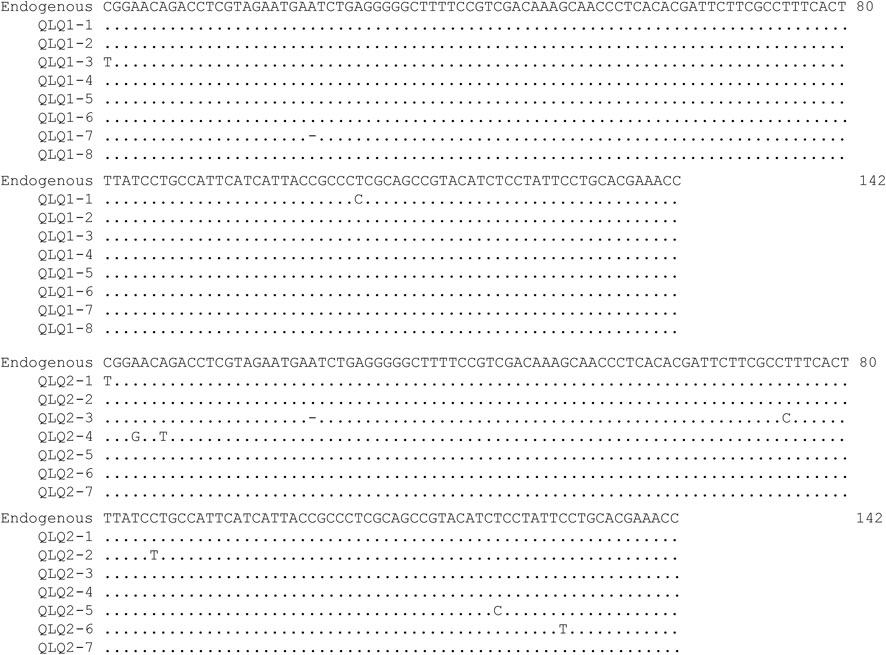
FIGURE 4. Alignment of the achieved Cytb clones of two Qinglongquan pig samples using L-PCR without UNG treatment. The “Endogenous” sequence is determined by the results of amplification after UNG treatment. Nucleotides identical to the endogenous sequence are indicated by dots.
Discussion
Previous storage experiments have revealed that diluted DNA templates are more prone to drop under the minimum fragment threshold than concentrated DNA, even after a short period of storage. Therefore, the serially diluted DNA templates were used to simulate the degradable and fragmented ancient DNA templates; the results demonstrated that the L-PCR assay was efficient and accurate for ancient DNA amplification (Figure 2).
Currently, the use of UNG and multiple clones after amplification are conventional troubleshooting methods for assuring the authenticity of ancient DNA sequences. However, eliminating damaged templates using UNG also greatly aggravates the dilemma of the paucity of ancient DNA and the proposed after-amplification authenticity validation, such as multiple clones, requires increased time inputs but with fewer data outputs. Primers used in L-PCR were recognized with six distinct conserved regions on the target sequence, giving rise to amplification of the target sequence with high selectivity from the beginning, excluding damaged templates, and eliminating false amplification of possible homologous NUMTs or other contaminants. Similar to LAMP, the first-stage amplification of the L-PCR assay generated stem-loop DNAs with several inverted repeats and cauliflower-like structures (Notomi et al., 2000; Hardinge and Murray, 2019), thereby providing a very high molecular DNA nanostructure without primer carryover and avoiding false positives due to base lesions (Briggs et al., 2010; Hardinge and Murray, 2019).
Hostile sample deposit conditions, including high or fluctuating temperatures, excessive acidity, inappropriate salinity, and immoderate water percolation, easily leads to DNA lesions, such as strand breaks, oxidative lesions, cross-links, and other unknown types of damage in ancient DNA. Fragmentation and DNA modifications therefore hindered the elongation of DNA strands by DNA polymerase (Pääbo et al., 2004; Berdis, 2017; Garafutdinov et al., 2020). Thus, the DNA preservation conditions are of great relevance in obtaining target DNA in ancient DNA research. In the present study, the deposit conditions of all archeological sites were pre-investigated; therefore, we designed amplicons with variable lengths and different regions. However, our results suggested that the yield ratio did not decrease consistently with increasing age. Moreover, regardless of whether DNA templates came from younger or older pig and chicken samples, the L-PCR assay were able to yield higher numbers and ratios of ancient DNA sequence from the samples. With L-PCR enrichment of ancient templates, the L-PCR assay presents an effective method of generating valuable sequences in ancient DNA research.
In addition, single nucleotide polymorphism (SNP) sites have been applied as DNA markers in, for example, species identification in forensic detection (Hou et al., 2014; Jiang et al., 2020; Sinha et al., 2020), traditional Chinese medicine research (Cao et al., 2014; Hou et al., 2017), and agricultural product quality control (Fukuta et al., 2004; McClure et al., 2018; Raatz et al., 2019). However, objects investigated in these fields are usually characterized by unknown composition, seriously damaged DNA, or DNA that is degraded to low copy numbers over time or under multifarious processes. These intrinsic challenges generally limit the utilization of conventional detection approaches. With high productivity and high fidelity, the L-PCR assay can be regarded as a tool that addresses these challenges.
In conclusion, the L-PCR assay combines the merits of PCR and loop primer-mediated amplification. Possessing high efficiency and fidelity, this assay is an effective tool for obtaining fragmented but valuable authentic DNA sequences from poorly preserved or recalcitrant ancient specimens. It can also be applied to improve molecular diagnosis using poor DNA templates.
Data availability statement
The datasets presented in this study can be found in online repositories. The names of the repository/repositories and accession number(s) can be found below: DRYAD (http://datadryad.org) with DOI https://doi.org/10.5061/dryad.zw3r228b4.
Ethics statement
The animal study was reviewed and approved by the China Agricultural University Laboratory Animal Welfare and Animal Experimental Ethical Inspection board.
Author contributions
XBZ and HX designed this project; HX, ZW, LY and XGZ performed the experiments; HX analyzed the data; HX, LY and XBZ interpreted the data and drafted the manuscript. All authors contributed critically to the drafts and gave final approval for publication.
Funding
This study was funded by the National Natural Science Foundations of China (Grant number: 31961133031), the Young S&T Talent Training Program of Guangdong Provincial Association for S&T (Grant number: SKXRC202205), and the Foundation for Creative Team and Key Laboratory in Higher Education of Guangdong (Grant number: 2019KCXTD006 and 2019KSYS011).
Acknowledgments
We express sincere thanks to Jing Yuan (Institute of Archaeology, Chinese Academy of Social Science), Jianqiang Gao (Hebei Provincial Institute of Cultural Relic), Baoquan Yu (Xushui County Office for Preservation of Ancient Monuments), Yunbing Luo (Hubei Provincial Institute of Cultural Relics and Archaeology) for generously providing the ancient samples.
Conflict of interest
The authors declare that the research was conducted in the absence of any commercial or financial relationships that could be construed as a potential conflict of interest.
Publisher’s note
All claims expressed in this article are solely those of the authors and do not necessarily represent those of their affiliated organizations, or those of the publisher, the editors and the reviewers. Any product that may be evaluated in this article, or claim that may be made by its manufacturer, is not guaranteed or endorsed by the publisher.
References
Berdis, A. J. (2017). Inhibiting DNA polymerases as a therapeutic intervention against cancer. Front. Mol. Biosci. 4, 78. doi:10.3389/fmolb.2017.00078
Briggs, A. W., Stenzel, U., Meyer, M., Krause, J., Kircher, M., and Paabo, S. (2010). Removal of deaminated cytosines and detection of in vivo methylation in ancient DNA. Nucleic Acids Res. 38, e87. doi:10.1093/nar/gkp1163
Cao, M., Wang, J., Yao, L., Xie, S., Du, J., and Zhao, X. (2014). Authentication of animal signatures in traditional Chinese medicine of Lingyang Qingfei Wan using routine molecular diagnostic assays. Mol. Biol. Rep. 41, 2485–2491. doi:10.1007/s11033-014-3105-x
den Tex, R. J., Maldonado, J. E., Thorington, R., and Leonard, J. A. (2010). Nuclear copies of mitochondrial genes: Another problem for ancient DNA. Genetica 138, 979–984. doi:10.1007/s10709-010-9481-9
Diroma, M. A., Modi, A., Lari, M., Sineo, L., Caramelli, D., and Vai, S. (2021). New insights into mitochondrial DNA reconstruction and variant detection in ancient samples. Front. Genet. 12, 619950. doi:10.3389/fgene.2021.619950
Fukuta, S., Mizukami, Y., Ishida, A., Ueda, J., Hasegawa, M., Hayashi, I., et al. (2004). Real-time loop-mediated isothermal amplification for the CaMV-35S promoter as a screening method for genetically modified organisms. Eur. Food Res. Technol. 218, 496–500. doi:10.1007/s00217-003-0862-5
Garafutdinov, R. R., Sakhabutdinova, A. R., Kupryushkin, M. S., and Pyshnyi, D. V. (2020). Prevention of DNA multimerization using phosphoryl guanidine primers during isothermal amplification with Bst exo- DNA polymerase. Biochimie 168, 259–267. doi:10.1016/j.biochi.2019.11.013
Gutaker, R. M., and Burbano, H. A. (2017). Reinforcing plant evolutionary genomics using ancient DNA. Curr. Opin. Plant Biol. 36, 38–45. doi:10.1016/j.pbi.2017.01.002
Hardinge, P., and Murray, J. A. H. (2019). Reduced false positives and improved reporting of loop-mediated isothermal amplification using quenched fluorescent primers. Sci. Rep. 9, 7400. doi:10.1038/s41598-019-43817-z
Hofreiter, M., Jaenicke, V., Serre, D., von Haeseler, A., and Pääbo, S. (2001). DNA sequences from multiple amplifications reveal artifacts induced by cytosine deamination in ancient DNA. Nucleic Acids Res. 29, 4793–4799. doi:10.1093/nar/29.23.4793
Hofreiter, M., Serre, D., Poinar, H. N., Kuch, M., and Pääbo, S. (2001). Ancient DNA. Nat. Rev. Genet. 2, 353–359. doi:10.1038/35072071
Hou, F., Cao, J., Wang, X., Wang, S., Peng, C., and Guo, J. (2017). Specific authentication of Hippocampus based on SNP markers. Biochem. Syst. Ecol. 71, 131–136. doi:10.1016/j.bse.2017.01.005
Hou, G., Jiang, X., Yang, Y., Jia, F., Li, Q., Zhao, J., et al. (2014). A 21-locus autosomal SNP multiplex and its application in forensic science. J. Forensic Sci. 59, 5–14. doi:10.1111/1556-4029.12259
Huy, N. T., Hang le, T. T., Boamah, D., Lan, N. T., Van Thanh, P., Watanabe, K., et al. (2012). Development of a single-tube loop-mediated isothermal amplification assay for detection of four pathogens of bacterial meningitis. FEMS Microbiol. Lett. 337, 25–30. doi:10.1111/1574-6968.12002
Jiang, H. H., Li, B., Ma, Y., Bai, S. Y., Dahmer, T. D., Linacre, A., et al. (2020). Forensic validation of a panel of 12 SNPs for identification of Mongolian wolf and dog. Sci. Rep. 10, 13249. doi:10.1038/s41598-020-70225-5
Kashir, J., and Yaqinuddin, A. (2020). Loop mediated isothermal amplification (LAMP) assays as a rapid diagnostic for COVID-19. Med. Hypotheses 141, 109786. doi:10.1016/j.mehy.2020.109786
Krause, J., Dear, P. H., Pollack, J. L., Slatkin, M., Spriggs, H., Barnes, I., et al. (2006). Multiplex amplification of the mammoth mitochondrial genome and the evolution of Elephantidae. Nature 439, 724–727. doi:10.1038/nature04432
Larson, G., Liu, R., Zhao, X., Yuan, J., Fuller, D., Barton, L., et al. (2010). Patterns of East Asian pig domestication, migration, and turnover revealed by modern and ancient DNA. Proc. Natl. Acad. Sci. U. S. A. 107, 7686–7691. doi:10.1073/pnas.0912264107
McClure, M. C., McCarthy, J., Flynn, P., McClure, J. C., Dair, E., O'Connell, D. K., et al. (2018). SNP data quality control in a national beef and dairy cattle system and highly accurate SNP based parentage verification and identification. Front. Genet. 9, 84. doi:10.3389/fgene.2018.00084
Murray, L., Edwards, L., Tuppurainen, E. S., Bachanek-Bankowska, K., Oura, C. A., Mioulet, V., et al. (2013). Detection of capripoxvirus DNA using a novel loop-mediated isothermal amplification assay. BMC Vet. Res. 9, 90. doi:10.1186/1746-6148-9-90
Noonan, J. P., Coop, G., Kudaravalli, S., Smith, D., Krause, J., Alessi, J., et al. (2006). Sequencing and analysis of Neanderthal genomic DNA. Science 314, 1113–1118. doi:10.1126/science.1131412
Notomi, T., Okayama, H., Masubuchi, H., Yonekawa, T., Watanabe, K., Amino, N., et al. (2000). Loop-mediated isothermal amplification of DNA. Nucleic Acids Res. 28, E63. doi:10.1093/nar/28.12.e63
Pääbo, S. (1985). Molecular cloning of Ancient Egyptian mummy DNA. Nature 314, 644–645. doi:10.1038/314644a0
Pääbo, S., Poinar, H., Serre, D., Jaenicke-Despres, V., Hebler, J., Rohland, N., et al. (2004). Genetic analyses from ancient DNA. Annu. Rev. Genet. 38, 645–679. doi:10.1146/annurev.genet.37.110801.143214
Park, Y. R., Kim, H. R., Kim, S. H., Lee, K. K., Lyoo, Y. S., Yeo, S. G., et al. (2018). Loop-mediated isothermal amplification assay for the rapid and visual detection of novel porcine circovirus 3. J. Virol. Methods 253, 26–30. doi:10.1016/j.jviromet.2017.12.006
Raatz, B., Mukankusi, C., Lobaton, J. D., Male, A., Chisale, V., Amsalu, B., et al. (2019). Analyses of african common bean (Phaseolus vulgaris L.) germplasm using a SNP fingerprinting platform: Diversity, quality control and molecular breeding. Genet. Resour. Crop Evol. 66, 707–722. doi:10.1007/s10722-019-00746-0
Sinha, M., and Rao, I. A. (2020). “SNP testing in forensic science,” in Forensic DNA typing: Principles, applications and advancements. Editors P. Shrivastava, H. R. Dash, J. A. Lorente, and J. Imam (Singapore: Springer Singapore), 365–376.
Søe, M. J., Rohde, M., Mikkelsen, J., and Warthoe, P. (2013). IsoPCR: An analytically sensitive, nested, multiplex nucleic acid amplification method. Clin. Chem. 59, 436–439. doi:10.1373/clinchem.2012.193664
Spielmann, G., Ziegler, S., Haszprunar, G., Busch, U., Huber, I., and Pavlovic, M. (2019). Using loop-mediated isothermal amplification for fast species delimitation in eels (genus Anguilla), with special reference to the European eel (Anguilla anguilla). Food control. 101, 156–162. doi:10.1016/j.foodcont.2019.02.022
Stiller, M., Green, R. E., Ronan, M., Simons, J. F., Du, L., He, W., et al. (2006). Patterns of nucleotide misincorporations during enzymatic amplification and direct large-scale sequencing of ancient DNA. Proc. Natl. Acad. Sci. U. S. A. 103, 13578–13584. doi:10.1073/pnas.0605327103
Thalmann, O., Shapiro, B., Cui, P., Schuenemann, V. J., Sawyer, S. K., Greenfield, D. L., et al. (2013). Complete mitochondrial genomes of ancient canids suggest a European origin of domestic dogs. Science 342, 871–874. doi:10.1126/science.1243650
Tomita, N., Mori, Y., Kanda, H., and Notomi, T. (2008). Loop-mediated isothermal amplification (LAMP) of gene sequences and simple visual detection of products. Nat. Protoc. 3, 877–882. doi:10.1038/nprot.2008.57
Uemura, N., Makimura, K., Onozaki, M., Otsuka, Y., Shibuya, Y., Yazaki, H., et al. (2008). Development of a loop-mediated isothermal amplification method for diagnosing Pneumocystis pneumonia. J. Med. Microbiol. 57, 50–57. doi:10.1099/jmm.0.47216-0
Uysal Yoca Ö., E. H., Yüce, Z., and Uysal Yoca, O. (2021). Ancient DNA research: Ongoing challenges and contribution to medical sciences. J. Basic Clin. Health Sci. 5, 182–189. doi:10.30621/jbachs.854258
Vichaibun, V., and Kanchanaphum, P. (2020). Quantitative LAMP and PCR detection of Salmonella in chicken samples collected from local markets around pathum thani province, Thailand. Int. J. Food Sci. 2020, 8833173. doi:10.1155/2020/8833173
Willerslev, E., and Cooper, A. (2005). Ancient DNA. Proc. Biol. Sci. 272, 3–16. doi:10.1098/rspb.2004.2813
Wong, Y. P., Othman, S., Lau, Y. L., Radu, S., and Chee, H. Y. (2018). Loop-mediated isothermal amplification (LAMP): A versatile technique for detection of micro-organisms. J. Appl. Microbiol. 124, 626–643. doi:10.1111/jam.13647
Yang, M. A., Fan, X., Sun, B., Chen, C., Lang, J., Ko, Y. C., et al. (2020). Ancient DNA indicates human population shifts and admixture in northern and southern China. Science 369, 282–288. doi:10.1126/science.aba0909
Zhang, D., Xia, H., Chen, F., Li, B., Slon, V., Cheng, T., et al. (2020). Denisovan DNA in late pleistocene sediments from baishiya karst cave on the Tibetan plateau. Science 370, 584–587. doi:10.1126/science.abb6320
Keywords: loop-primer, L-PCR, nested PCR, ancient DNA (aDNA), poorly preserved DNA
Citation: Xiang H, Wang Z, Yang L, Zhang X and Zhao X (2022) Using loop-primer mediated PCR to enhance the detection of poorly preserved DNA. Front. Genet. 13:1000123. doi: 10.3389/fgene.2022.1000123
Received: 22 July 2022; Accepted: 21 November 2022;
Published: 30 November 2022.
Edited by:
Michael David Martin, Norwegian University of Science and Technology, NorwayReviewed by:
Jinquan Li, Inner Mongolia Agricultural University, ChinaIzhar Ul-Haq Khan, Agriculture and Agri-Food Canada (AAFC), Canada
Copyright © 2022 Xiang, Wang, Yang, Zhang and Zhao. This is an open-access article distributed under the terms of the Creative Commons Attribution License (CC BY). The use, distribution or reproduction in other forums is permitted, provided the original author(s) and the copyright owner(s) are credited and that the original publication in this journal is cited, in accordance with accepted academic practice. No use, distribution or reproduction is permitted which does not comply with these terms.
*Correspondence: Xingbo Zhao, emh4YkBjYXUuZWR1LmNu; Hai Xiang, eGhAZm9zdS5lZHUuY24=