- 1Key Laboratory of Non-coding RNA Transformation Research of Anhui Higher Education Institution, Yijishan Hospital of Wannan Medical College, Wuhu, China
- 2Suzhou Polytechnic Institute of Agriculture, Suzhou, China
- 3Wuhan Botanical Garden, Chinese Academy of Sciences, Wuhan, China
- 4Central Laboratory, Yijishan Hospital of Wannan Medical College, Wuhu, China
Background: Glioblastoma (GBM) is the most common and aggressive primary intracranial tumor of the central nervous system, and the prognosis of GBM remains a challenge using the standard methods of treatment—TMZ, radiation, and surgical resection. Traditional Chinese medicine (TCM) is a helpful complementary and alternative medicine. However, there are relatively few studies on TCM for GBM.
Purpose: We aimed to find the connection between TCM and anti-GBM.
Study design: Network pharmacology and inflammatory microenvironment strategy were used to predict Siraitia grosvenorii (Luo Han Guo) target for treating glioblastoma.
Methods: We mainly used network pharmacology and bioinformatics.
Results: CCL5 was significantly highly expressed in GBM with poor prognostics. Uni-cox and randomForest were used to determine that CCL5 was especially a biomarker in GBM. CCL5 was also the target for SG and TMZ. The active ingredient of Luo Han Guo — squalene and CCL5 —showed high binding efficiency. CCL5, a chemotactic ligand, was enriched and positively correlated in eosinophils. CCL5 was also the target of Luo Han Guo, and its effective active integrate compound –— squalene — might act on CCL5.
Conclusion: SG might be a new complementary therapy of the same medicine and food, working on the target CCL5 and playing an anti-GBM effect. CCL5 might affect the immune microenvironment of GBM.
Introduction
Glioblastoma (GBM) is the most common and aggressive primary intracranial tumor of the central nervous system (Barthel et al., 2019; Miller et al., 2019). Most of them are induced by genetic mutations of high penetrance genes related to rare syndromes, mainly manifested as increased intracranial pressure, neurocognitive dysfunction, and seizures, resulting in central nervous system damage and endangering the lives of patients (Zanders et al., 2019). The standard treatment for GBM is surgery, drug therapy, and radiation therapy, and the median survival time of patients is only 15 months (Kumar et al., 2019). With the changes in eating habits, living environment, and work pressure, the incidence of GBM is increasing and getting younger. Surgical resection combined with postoperative radiotherapy, chemotherapy, and immunotherapy will inevitably damage the body’s normal function and cause adverse reactions. Multi-drug resistance, especially temozolomide (TMZ), leads to frequent GBM recurrences, which is a challenge in treating GBM, and its underlying molecular mechanism is still unclear (Yin et al., 2019). Since the blood-brain barrier (BBB) can prevent the accumulation of charged or macromolecules in the tumor microenvironment at a physically relevant concentration, thereby exerting an oncolytic effect, the content of TMZ in the brain is only 40% percent of the content in the blood, and new component pharmacological methods must be developed to enhance the curative effect of the current treatment, prolong the median survival time of the patient to exceed the median survival time of 15 months (Kumar et al., 2019).
The plants of traditional Chinese medicine (TCM) were used for the treatment of various cancers (Dai et al., 2016), such as GBM (Wang et al., 2019). The use of TCM to promote health and adjuvant therapy is becoming increasingly popular worldwide (Khan and Tania, 2020). The active components of Salvia miltiorrhiza can inhibit the proliferation of U87 cells, induce apoptosis, and enhance the efficacy of TMZ (Wang et al., 2019). Lycium chinense can up-regulate CD3+T, CD8+T, and TNF-α, inhibit the proliferation of mouse C6 cells, and up-regulate CD4+CD5+T cells to prolong survival and regulate the BBB (Wang et al., 2019). Magnolol inhibits the migration and proliferation of GBM cells through the JAK-STAT3 signal pathway, mainly by inhibiting the production of GBM stem cell-like cells (Fan et al., 2019). However, the clinical application value of TCM in the treatment of GBM has not been promoted, and more molecular mechanism studies are needed to verify it. Therefore, our research aims to provide new potential for the treatment of GBM with a medicinal plant.
The TCM Siraitia grosvenori (SG) is a perennial herbaceous plant of the Cucurbitaceae family with huge resource reserves and native to southern China, also known as monk fruit and Luo Han Guo, which is a medicinal food homologous species granted by the China Food and Drug Administration with significant clinical effects (Xia et al., 2018). Mogroside has an excellent biological development, which can inhibit the excessive activation of Signal Transducer and Activator of Transcription 3 (STAT3) and promote tumor cell apoptosis (Liu et al., 2018), and targeting STAT3 can improve tumor progression and anticancer immunity response (Lee et al., 2019); reversing emergency medical technician (EMT) and destroying the cytoskeleton to inhibit hyperglycemia-induced lung cancer cell metastasis (Guan et al., 2019). Mogroside IIV and IIIV activate AMP-activated protein kinase (AMPK) and produce anti-hyperglycemic and anti-lipid properties in the body (Abdel-Hamid et al., 2020); mogroside V can cross the BBB and affect schizophrenia-like behavior (Ju et al., 2020) and can also exert neuroprotective activity (Xia et al., 2013); mogroside IVe may be potentially used as a bioactive phytochemical supplement for the treatment of colorectal cancer and laryngeal cancer (Liu et al., 2016). Monk fruit also has other pharmacological effects, such as up-regulating Sirtuin 1 (SIRT1) to reduce oxidative stress and alleviate the decline in oocyte quality during in vitro aging (Nie et al., 2019).
Network pharmacology is based on the high-throughput multi-omics data analysis to clarify the mechanism of multi-component/multi-target/multiple action pathways in medicinal plants (Hopkins, 2008). The newly network pharmacology analysis was employed to integrate active compounds, targets and pathways prediction, and network analysis which may provide novel insights into the therapeutic effects and molecular mechanisms of SG in the treatment for GBM (Abdel-Hamid et al., 2020). Then, we offered a new flowchart to explain the potential target of Siraitia grosvenorii (Luo Han Guo) for GBM (Figure 1).
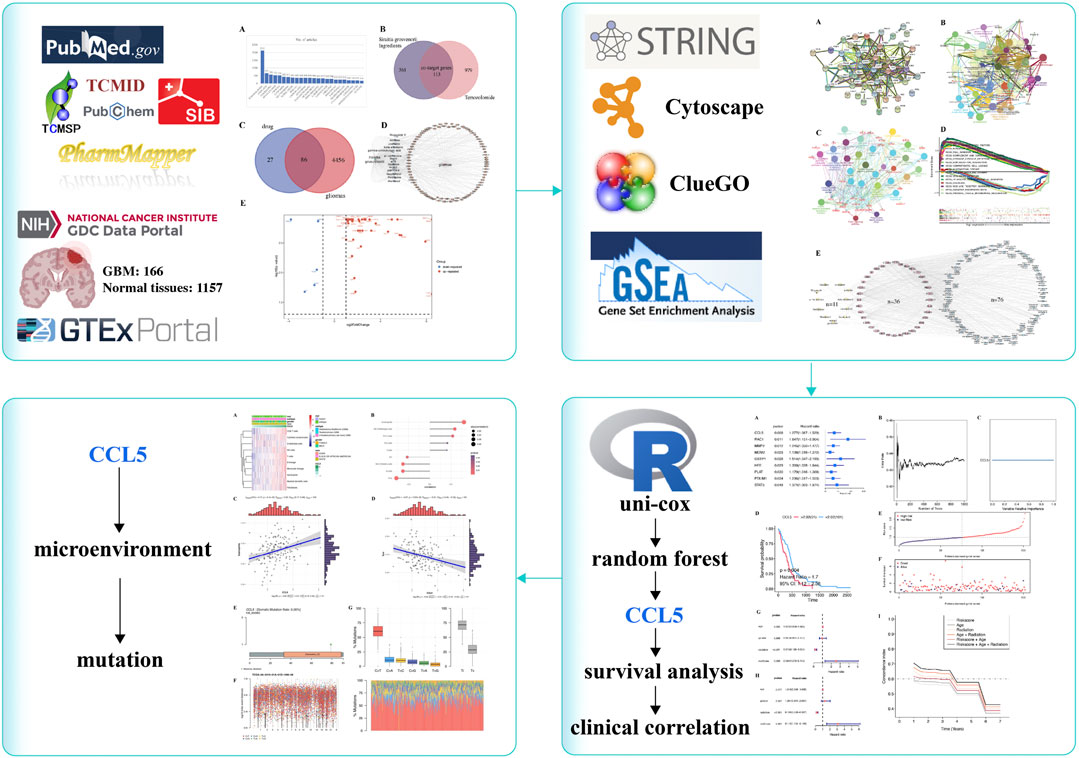
FIGURE 1. Graphical abstract. The new flowchart explains the potential target of Siraitia grosvenorii (Luo Han Guo) for glioblastoma.
Materials and Methods
The Integration of SG-TMZ-GBM (Siraitia grosvenori - Temozolomide - Glioblastoma) Targets
Through PubMed database (https://pubmed.ncbi.nlm.nih.gov) text mining, we selected the most effective clinical drug in the treatment of GBM. Based on the TCMSP (Ru et al., 2014) database (blood-brain barrier (BBB) ≥ 0.3, drug-like (DL) ≥ 0.18, oral bioavailability (OB) ≥ 30%), and TCMID (Huang et al., 2018), we collected the active ingredients and targets in monk fruit. Then, we used the chemical components to obtain the structure files by the PubChem Compound database (Kim et al., 2019) and uploaded the structure files to predict the targets across the PharmMapper (Wang et al., 2017) and Swiss Target Prediction (Gfeller et al., 2014). A Venn diagram (http://bioinformatics.psb.ugent.be/webtools/Venn/) was drawn for visualizing the SG-TMZ interacting targets. Gliomas-related targets were predicted by OMIM (Amberger et al., 2015), DrugBank (Wishart et al., 2018), and PubMed. Then, taking the intersection with the prediction targets of SG-TMZ, which is named Siraitia grosvenori - temozolomide – gene (SG-TMZ-G).
We downloaded GBM’s transcriptomic and clinical data and normal brain tissues from XENA TCGA and GTEx (https://xena.ucsc.edu/public/). Differentially expressed GBMs (DE-GBMs) were computed by limma (Smyth, 2005) with |logFoldChange (logFC)| > 2 and q-value < 0.05 as previously reported (Jiang et al., 2020a; Jiang et al., 2020b). Subsequently, common GBM-related targets were integrated between SG-TMZ-G. A volcano plot was used to show the distribution of SG-TMZ-GBM (SG-TMZ-glioblastoma).
Functional Analysis and Network Construction of SG-TMZ-GBM
STRING v11.5 was used to construct a protein-protein interaction (PPI) network, scores >0.70 were considered to have high confidence (Szklarczyk et al., 2021). Functional analyses of the gene ontology (GO), the Kyoto Encyclopedia of Genes and Genomes (KEGG) were performed by ClueGO plug-in (Bindea et al., 2009) in Cytoscape v3.8.2 (Reimand et al., 2019) with q-value ≤ 0.001. The compound-target-pathway network was built by Cytoscape (Reimand et al., 2019).
The Determination of the Key SG-TMZ-GBMs
Hazard ratios (HR) were applied using univariable Cox (uni-cox) regression analysis (p-value < 0.05). We then detected the key SG-TMZ-GBMs by “survival” and “survminer” package (Jiang et al., 2020b). Random forest was calculated by randomSurvivalForest to rank the importance of survival-related SG-TMZ-GBMs, with a relative importance >0.7 as the final feature (Liu et al., 2021). Survival analysis was built with the best cutoff value (Liu et al., 2021), the Kaplan-Meier method was used to draw survival curves, and the log-rank test was used to evaluate differences. A scatter plot of C-C Motif Chemokine Ligand 5 (CCL5) expression and survival time in GBM patients were drawn by ggrisk (Jiang et al., 2020b). The forest plot was used for performing uni-cox and multiple cox (multi-cox) regression analysis (Jiang et al., 2020b). We also used the receiver operating characteristic curve (ROC), concordance index (c-index) to evaluate the multi-clinical prognostic performance (Longato et al., 2020).
Inflammatory Microenvironment and Mutation Analysis
The microenvironment cell population-counter method was chosen to evaluate the association between CCL5 and immune cell populations (Petitprez et al., 2020). We used immune cells markers and GBM transcriptome data to validate the strong correlation between CCL5 and 24 immune cells markers (Bindea et al., 2013). Gene mutations of GBM expression by “maftools” package (Mayakonda et al., 2018). CCL5 protein expression was detected by immunohistochemistry from the HPA database (https://www.proteinatlas.org/ENSG00000271503-CCL5/pathology/glioma#).
Results
SG-TMZ-GBM Detection
PubMed text mining showed 2121 literature reports on the treatment of GBM with TMZ (Figure 2A). Through the text data mining of the Therapeutic Target Database (TTD) database and PubMed published articles, we identified 1092 target genes for treating GBM with TMZ.
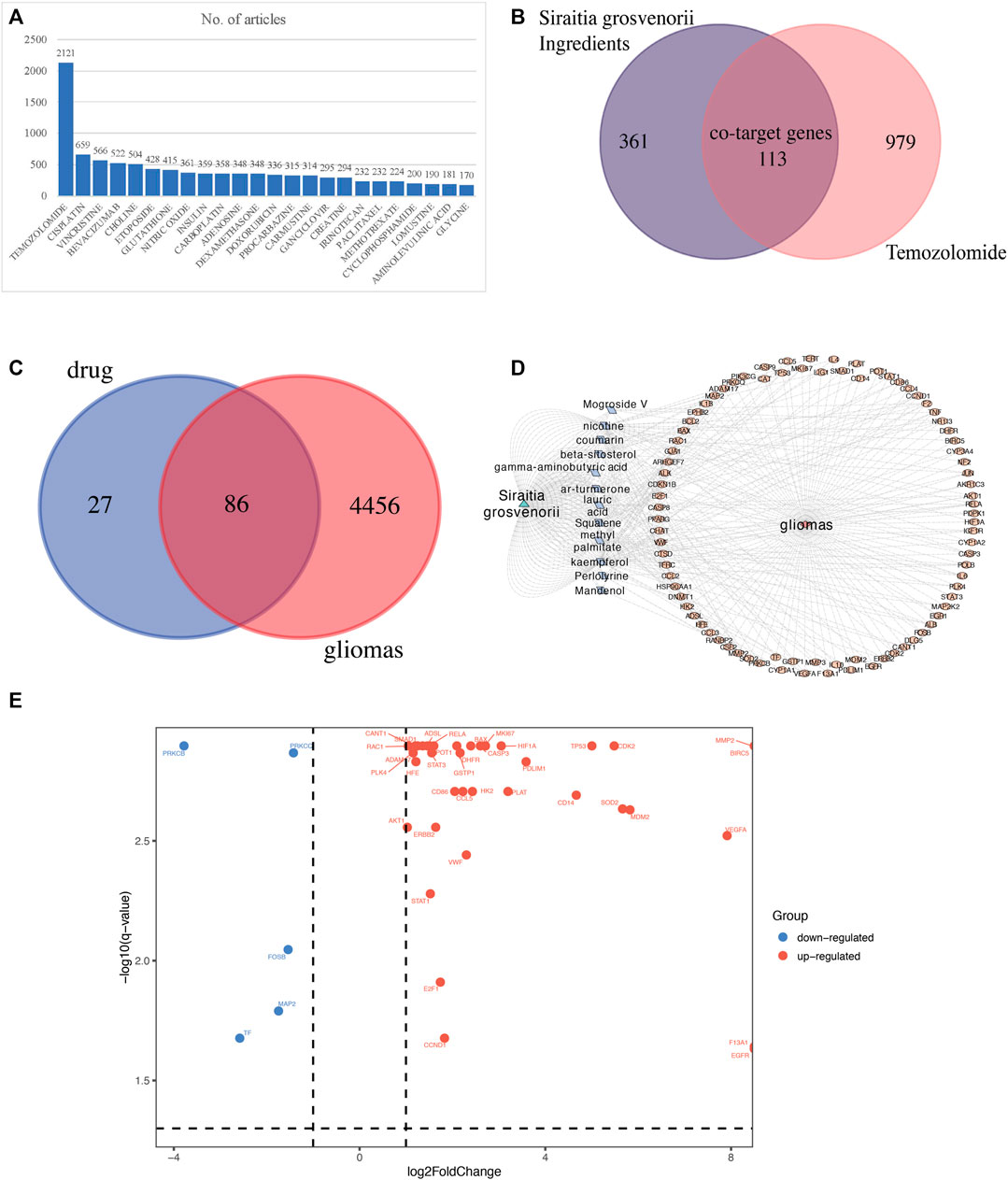
FIGURE 2. SG-TMZ-GBM detection. (A) The analysis result on the clinical drug in treating gliomas in PubMed database (updated by 2021-8-10); (B) co-target genes predicted between the ingredients of Siraitia grosvenorii and temozolomide; (C) co-target genes predicted between the ingredients of Siraitia grosvenorii -temozolomide and gliomas; (D) co-target genes predicted network between the ingredients of Siraitia grosvenorii and gliomas, a triangle means Siraitia grosvenorii, a diamond means the 12 ingredients of Siraitia grosvenorii, an oval means co-target genes in gliomas; (E) volcano plot for differentially expressed target genes.
We obtained 12 chemical compositions in SG by TCMSP and TCMID, and gained the compound structure by PubChem, and predicted the 474 target genes of SG by the Swiss Target Prediction and PharmMapper. A total of 113 SG-TMZ targets were found by taking the intersection (Figure 2B). We further discovered 4542 target genes related to gliomas through PALM-IST, filtered 86 target genes as SG-TMZ-G (Figure 2C), and drew a network diagram (Figure 2D). For example, IL6 was a co-target gene in the gamma-aminobutyric acid, lauric acid, and methyl palmitate of SG and GBM; CCL5 was a co-target gene in squalene. According to the cutoff log2FoldChange> 2 and q-value <0.05, we screened the interaction of differentially expressed genes (DEGs) in GBM-normal brain tissues and SG-TMZ-G, Volcano plot for 42 SG-TMZ-GBM targets were detected for the following research (Figure 2E).
Luo Han Guo Compound-Target-Disease Interaction Network and Functional Enrichment Analysis
We imported 42 SG-TMZ-GBMs into the STRING database to construct a protein-protein interaction (PPI) network, the primary connection in the network which might have pharmacological effects in GBM. In addition, the four targets, including Protection of Telomeres 1 (POT1), Adenylosuccinate Lyase (ADSL), FosB Proto-Oncogene, AP-1 Transcription Factor Subunit (FOSB), and Calcium Activated Nucleotidase 1 (CANT1), did not interact with other targets (Figure 3A). Tumor Protein P53 (TP53) and MDM2 Proto-Oncogene (MDM2), Cyclin Dependent kinase 2 (CDK2) and Cyclin D1 (CCND1) (scores >0.70) were considered to have high confidence. We further explore the correlation between 42 SG-TMZ-GBMs and glioblastoma by GO (Figure 3B), KEGG (Figure 3C), and GSEA (Figure 3D) enrichment analyses. We discovered that 54 significant GO enrichment results, such as “lactation,” “response to iron ion,” “apoptotic mitochondrial changes,” CCL5 was enriched in “human cytomegalovirus infection,” “toll-like receptor signaling pathway” and “epithelial cell signaling in helicobacter pylori infection,” Vascular Endothelial Growth Factor A (VEGFA), Rac Family Small GTPase 1 (RAC1), Protein kinase C Beta (PRKCB), and AKT Serine/Threonine kinase 1 (AKT1) were enriched in Vascular Endothelial Growth Factor (VEGF) signaling pathway (Figure 3B); pathway analysis revealed that SG-TMZ-GBMs were associated with cancer-related pathway, including glioma, non-small cell lung cancer, pancreatic cancer, and thyroid cancer, AKT1, BCL2 Associated X, Apoptosis Regulator (BAX), CCND1, E2F Transcription Factor 1 (E2F1), MDM2, PRKCB, and TP53 were enriched in “glioma” pathway, suggesting Luo Han Guo may play a role in cancer treatment; PRKCB, RELA Proto-Oncogene, NF-KB Subunit (RELA), STAT3, and VEGFA were enriched in “AGE-RAGE signaling pathway” and “HIF-1 signaling pathway” might be related in the inflammation-related diseases (Figure 3C). The GSEA KEGG enrichment analysis is shown in Figure 3D, and we found the top three significantly activated KEGG pathways were “KEGG hematopoietic cell lineage”, “KEGG leishmania infection”, and “KEGG nod like receptor signaling pathway”. A compound-target-pathway network was established based on the target recognition and pathway analysis, with nodes mapping compounds, targets, or pathways, and indicated interactions by Cytoscape (Figure 3E).
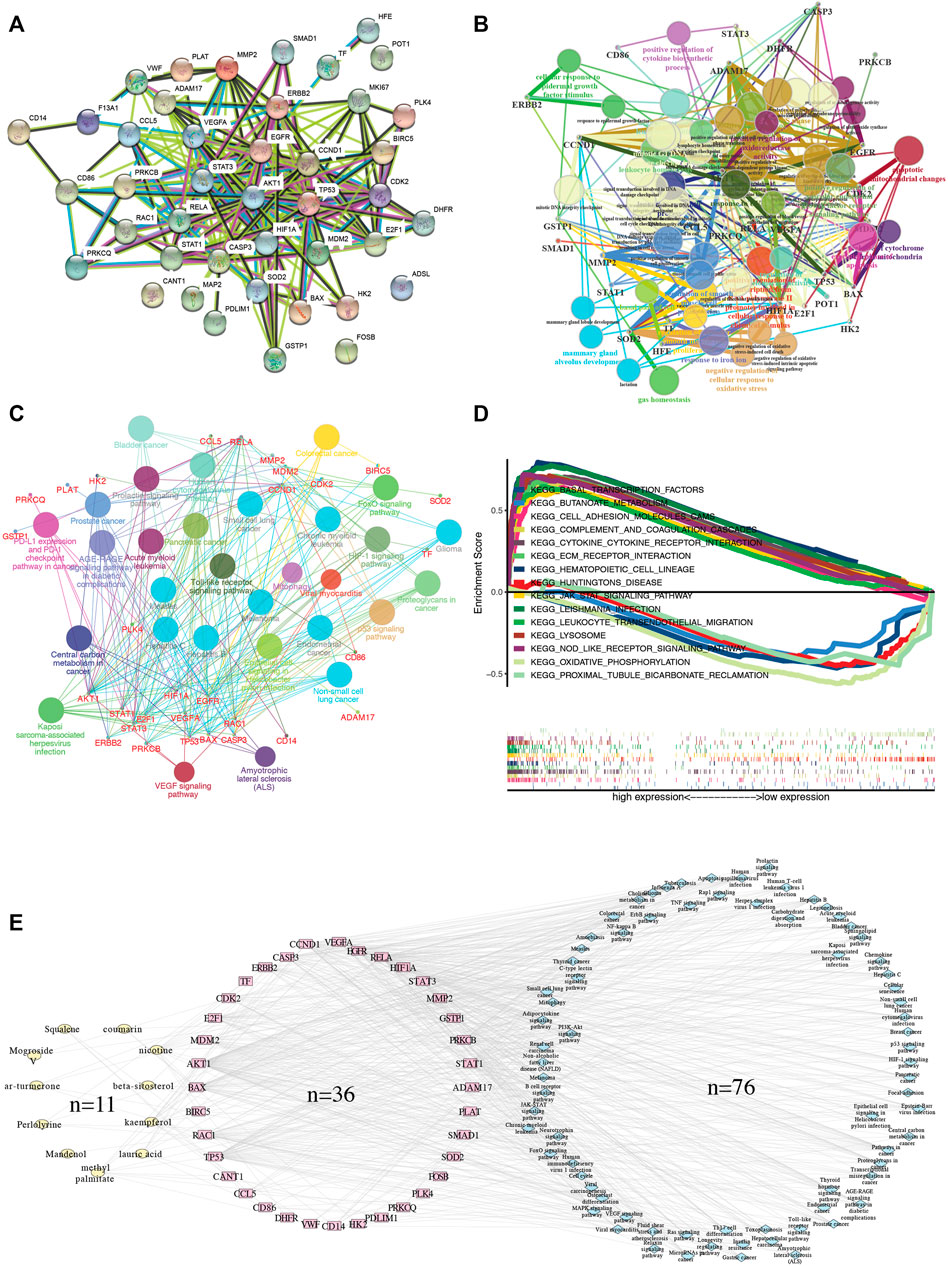
FIGURE 3. Targeted gene functional analysis. (A) PPI network analysis; (B) GO enrichment analysis; (C) KEGG pathway enrichment analysis; (D) the study of GSEA KEGG enrichment plot. (E) Compound-target-KEGG pathway network. The network was generated by Cytoscape 3.8.2. Yellow circles represent 11 ingredient targets from Siraitia grosvenorii. Red circles represent 36 common targets between ingredient targets from Siraitia grosvenorii and GBM significant targets. Blue circles represent 76 KEGG pathways.
The Determination of the Key SG-TMZ-GBMs
Uni-cox analysis revealed that 9 SG-TMZ-GBMs were determined as the significant survival-related risk genes. CCL5 was the most significant gene (p-value = 0.008) (Figure 4A). We found CCL5 was the key SG-TMZ-GBM (importance = 1) by random forest calculation (Figures 4B,C) and the survival analysis with p-value = 0.004 (Figure 4D). To further explore the effect of CCL5 on tshe GBM prognosis, a scatter plot of CCL5 expression and survival time in GBM patients was created (Figures 4E,F). Uni-cox and multi-cox regression analysis revealed that radiation (p-value < 0.001) and risk score (p-value = 0.008) were independent risk factors for overall survival analysis (Figures 4G,H). ROC c-index analysis illustrated that risk score + age + radiation, age + radiation, and radiation were the top three (Figure 4I).
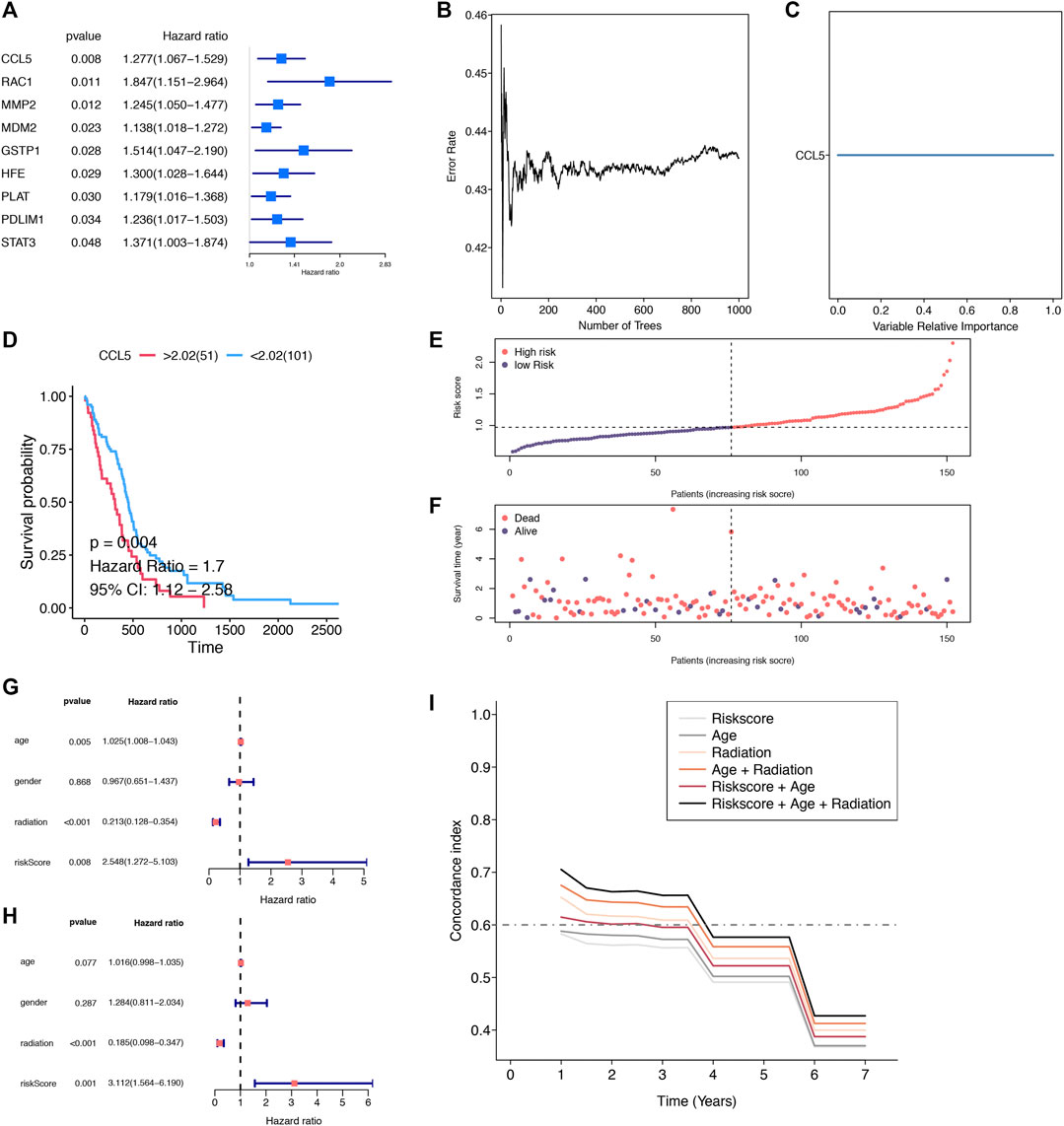
FIGURE 4. The determination of the key SG-TMZ-GBMs. (A) Uni-cox analysis; (B) error tree of randomForest; (C) variable relative importance of randomForest; (D) survival analysis; (E) the curve of risk score; (F) survival status by ggrisk; (G) uni-cox with clinical information; (H) multi-cox with clinical information; and (I) ROC-concordance index.
Inflammatory Microenvironment and Mutation Analysis
The microenvironment cell population-counter method evaluated the association between CCL5 and 10 immune cell populations from transcriptomic data. A strong correlation between CCL5 and CD8 T cells, T cells, B lineage, and fibroblasts were seen (Figure 5A). Then we further found the significant correlation (p-value < 0.05) between CCL5 and 9 of 24 immune cells markers (Figure 5B), such as the positive correlation in eosinophils (Figure 5C) and the negative correlation in Tcm (Figure 5D). In addition, exploring somatic mutations is helpful to understand the occurrence and development of GBM. The lollipop map shows the mutation distribution and protein domain of CCL5 with somatic mutation (Figure 5E). The distribution of the mutation spectrum of GBM samples can also be identified by a rainfall map (Figure 5F). The transition plot classified single nuclear variants into six categories (Figure 5G). Among them, the C > T mutation accounted for more than 50% of the total mutations. Furthermore, CCL5 protein expression can be detected by immunohistochemistry from the HPA database (Figure 5H).
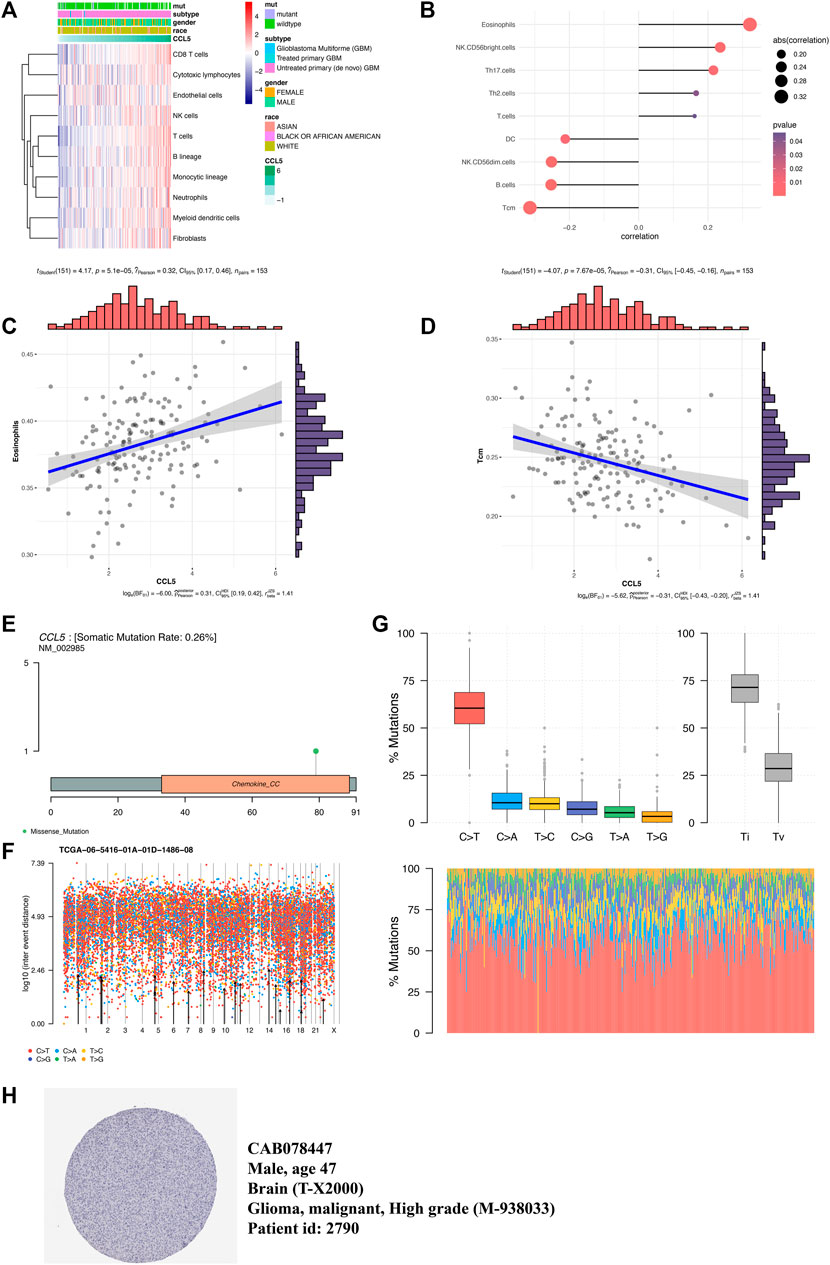
FIGURE 5. Inflammatory microenvironment and mutation analysis. (A) Association between CCL5 expression and 10 immune cell populations in GBM. (B) The association between CCL5 expression and 24 immune cell markers in GBM. (C) A scatter plot of the positive correlation between CCL5 expression and eosinophils. (D) A scatter plot of the negative correlation between CCL5 expression and Tcm. (E) The lollipop map shows the mutation distribution and protein domain of CCL5 with somatic mutation. (F) The rainfall map of TCGA-AC-A23H-01A-11D-A159-09 in the GBM sample. (G) The transition and crosscut graphs show the distribution of SNV in GBM with six transition and crosscut events. The stacked bar graph (bottom) shows the mutation spectrum distribution of each sample. (H) CCL5 protein expression can be detected by immunohistochemistry from the HPA database.
Discussion
GBM is the most frequent and the least treatable type of brain tumor, and the prognosis of GBM remains a challenge using the standard methods of treatment—TMZ, radiation, and surgical resection (Jiang et al., 2020b). TMZ is a novel methylating agent that demonstrated activity against recurrent GBM and is ineffective due to drug resistance (Wu et al., 2021). TCMs were considered anti-GBM auxiliary drugs, such as Solanum nigrum L. (Li et al., 2021), Panax ginseng, licorice, Lycium barbarum, Salvia miltiorrhiza bunge, Coptis rhizoma, and Sophora flavescens (Wang et al., 2019). TCM is a helpful complementary and alternative medicine, however, there are few studies on TCM for GBM (Wang et al., 2019). The anti-GBM effects of TCM extract provided the new medium for the treatment of GBM (Li et al., 2021).
We tried to find a new TCM complementary method to treat GBM and hope that through combining Chinese and Western medicine, TMZ resistance could be reversed and anti-tumor therapeutic effects could be achieved. Luo Han Guo is a TCM with the same medicine and food. The multiple compounds in Luo Han Guo not only act on the same target protein, but a single compound also acts on various target proteins and multiple pathways, which reflects the “multiple components, multiple targets, and multiple pathways” of Luo Han Guo’s synergistic effect. Luo Han Guo may work with ar-turmerone, methyl palmitate, lauric acid, beta-sitosterol, gamma-aminobutyric acid, coumarin, mogroside V, and squalene. GO functional enrichment analyses reflected that most of the active ingredients in SG might target nerve cells.
Through network pharmacology and bioinformatics analysis, we found that the CCL5 molecule is a potential target of SG, TMZ, and GBM, maybe the key to the clinical development of TMZ resistance (Figure 1). CCL5-CCR5 paracrine signaling could be an effective therapeutic strategy to improve chemotherapeutic efficacy against GBM (Zhang et al., 2021). CCL5 of glioma-associated microglia/macrophages regulates glioma migration and invasion via calcium-dependent matrix metalloproteinase 2 (Yu-Ju Wu et al., 2020). Knockdown or pharmacological inhibition of CCL5 increased the sensitivity of GBM cells treated with pericyte conditioned media to TMZ (Sprowls and Lathia, 2021). CCL5 was significantly highly expressed in GBM with poor prognostic. Uni-cox and randomForest were used to determine that CCL5 was a significantly important biomarker in GBM. CCL5 was also the target for SG and TMZ. The active ingredient of Luo Han Guo — squalene and CCL5 —show high binding efficiency. SG may be used as a new complementary therapy of the same medicine and food, acting on the target CCL5 and playing an anti-glioblastoma effect. Increasing the effective content of squalene in SG also needs further research. The radiation-related factors were the most critical in ROC c-index analysis. CCL5 plays a vital role in maintaining chemotherapy and radiation resistance.
Compared to genetically distinct syngeneic GBM models, the difference in mouse GBM models was eosinophils, reported in GBM (Khalsa and Shah, 2021). Eosinophils were associated with prognostic risk in the GBM microenvironment (Liang et al., 2020). We found that the SG-TMZ-GBM target, CCL5, a chemotactic ligand, is enriched and positively correlated in eosinophils. CCL5 is also the target of Luo Han Guo, and its effective active integrate compound – squalene—might act on CCL5, thereby affecting the immune microenvironment of GBM.
Data Availability Statement
The original contributions presented in the study are included in the article/Supplementary Material. Further inquiries can be directed to the corresponding author.
Author Contributions
Data curation, L.J., J.L., X.Z., and Y.C.; formal analysis, L.J., J.L., D.B., and Y.C.; funding acquisition, L.J.; validation, L.J.; writing—original draft, L.J.; writing—review and editing, L.J., Y.C., and K.L.
Funding
This project was supported by the Talent Scientific Research Start-up Foundation of Yijishan Hospital, Wannan Medical College (grant no. YR202001); the Opening Foundation of Key Laboratory of Non-coding RNA Transformation Research of Anhui Higher Education Institution (grant no. RNA202004); the Key Projects of Natural Science Research of Universities in Anhui Province (grant no. KJ 2020A0622).
Conflict of Interest
The authors declare that the research was conducted in the absence of any commercial or financial relationships that could be construed as a potential conflict of interest.
Publisher’s Note
All claims expressed in this article are solely those of the authors and do not necessarily represent those of their affiliated organizations, or those of the publisher, the editors, and the reviewers. Any product that may be evaluated in this article, or claim that may be made by its manufacturer, is not guaranteed or endorsed by the publisher.
References
Abdel-Hamid, M., Romeih, E., Huang, Z., Enomoto, T., Huang, L., and Li, L. (2020). Bioactive Properties of Probiotic Set-Yogurt Supplemented with Siraitia Grosvenorii Fruit Extract. Food Chem. 303, 125400. doi:10.1016/j.foodchem.2019.125400
Amberger, J. S., Bocchini, C. A., Schiettecatte, F., Scott, A. F., and Hamosh, A. (2015). OMIM.org: Online Mendelian Inheritance in Man (OMIM), an Online Catalog of Human Genes and Genetic Disorders. Nucleic Acids Res. 43, D789–D798. doi:10.1093/nar/gku1205
Barthel, F. P., Johnson, K. C., Johnson, K. C., Varn, F. S., Moskalik, A. D., Tanner, G., et al. (2019). Longitudinal Molecular Trajectories of Diffuse Glioma in Adults. Nature 576, 112–120. doi:10.1038/s41586-019-1775-1
Bindea, G., Mlecnik, B., Hackl, H., Charoentong, P., Tosolini, M., Kirilovsky, A., et al. (2009). ClueGO: a Cytoscape Plug-In to Decipher Functionally Grouped Gene Ontology and Pathway Annotation Networks. Bioinformatics 25, 1091–1093. doi:10.1093/bioinformatics/btp101
Bindea, G., Mlecnik, B., Tosolini, M., Kirilovsky, A., Waldner, M., Obenauf, A. C., et al. (2013). Spatiotemporal Dynamics of Intratumoral Immune Cells Reveal the Immune Landscape in Human Cancer. Immunity 39, 782–795. doi:10.1016/j.immuni.2013.10.003
Dai, S. X., Li, W. X., Han, F. F., Guo, Y. C., Zheng, J. J., Liu, J. Q., et al. (2016). In Silico identification of Anti-cancer Compounds and Plants from Traditional Chinese Medicine Database. Sci. Rep. 6, 25462–25511. doi:10.1038/srep25462
Fan, Y., Xue, W., Schachner, M., and Zhao, W. (2019). Honokiol Eliminates Glioma/glioblastoma Stem Cell-like Cells via JAK-STAT3 Signaling and Inhibits Tumor Progression by Targeting Epidermal Growth Factor Receptor. Cancers (Basel) 11, 22. doi:10.3390/cancers11010022
Gfeller, D., Grosdidier, A., Wirth, M., Daina, A., Michielin, O., and Zoete, V. (2014). SwissTargetPrediction: a Web Server for Target Prediction of Bioactive Small Molecules. Nucleic Acids Res. 42, W32–W38. doi:10.1093/nar/gku293
Guan, J., Lin, L., and Ouyang, M. (2019). Zhengyuan Capsule Alleviates Chemotherapy-Related Fatigue in Nude Mice with Human Lung Adenocarcinoma A549 Xenografts. Cancer 1, 1–7.
Hopkins, A. L. (2008). Network Pharmacology: the Next Paradigm in Drug Discovery. Nat. Chem. Biol. 4, 682–690. doi:10.1038/nchembio.118
Huang, L., Xie, D., Yu, Y., Liu, H., Shi, Y., Shi, T., et al. (2018). TCMID 2.0: a Comprehensive Resource for TCM. Nucleic Acids Res. 46, D1117–D1120. doi:10.1093/nar/gkx1028
Jiang, L., Zhong, M., Chen, T., Zhu, X., Yang, H., and Lv, K. (2020). Gene Regulation Network Analysis Reveals Core Genes Associated with Survival in Glioblastoma Multiforme. J. Cel. Mol. Med. 24, 10075–10087. doi:10.1111/jcmm.15615
Jiang, L., Zhu, X., Yang, H., Chen, T., and Lv, K. (2020). Bioinformatics Analysis Discovers Microtubular Tubulin Beta 6 Class V (TUBB6) as a Potential Therapeutic Target in Glioblastoma. Front. Genet. 11, 566579. doi:10.3389/fgene.2020.566579
Ju, P., Ding, W., Chen, J., Cheng, Y., Yang, B., Huang, L., et al. (2020). The Protective Effects of Mogroside V and its Metabolite 11-Oxo-Mogrol of Intestinal Microbiota against MK801-Induced Neuronal Damages. Psychopharmacology 237, 1011–1026. doi:10.1007/s00213-019-05431-9
Khalsa, J. K., and Shah, K. (2021). Immune Profiling of Syngeneic Murine and Patient GBMs for Effective Translation of Immunotherapies. Cells 10, 491. doi:10.3390/cells10030491
Khan, M. A., and Tania, M. (2020). Cordycepin in Anticancer Research: Molecular Mechanism of Therapeutic Effects. Cmc 27, 983–996. doi:10.2174/0929867325666181001105749
Kim, S., Chen, J., Cheng, T., Gindulyte, A., He, J., He, S., et al. (2019). PubChem 2019 Update: Improved Access to Chemical Data. Nucleic Acids Res. 47, D1102–D1109. doi:10.1093/nar/gky1033
Kumar, V., Radin, D., and Leonardi, D. (2019). Studies Examining the Synergy between Dihydrotanshinone and Temozolomide against MGMT+ Glioblastoma Cells In Vitro: Predicting Interactions with the Blood-Brain Barrier. Biomed. Pharmacother. 109, 386–390. doi:10.1016/j.biopha.2018.10.069
Lee, H., Jeong, A. J., and Ye, S.-K. (2019). Highlighted STAT3 as a Potential Drug Target for Cancer Therapy. BMB Rep. 52, 415–423. doi:10.5483/bmbrep.2019.52.7.152
Li, J.-H., Li, S.-Y., Shen, M.-X., Qiu, R.-Z., Fan, H.-W., and Li, Y.-B. (2021). Anti-tumor Effects of Solanum nigrum L. Extraction on C6 High-Grade Glioma. J. Ethnopharmacology 274, 114034. doi:10.1016/j.jep.2021.114034
Liang, P., Chai, Y., Zhao, H., and Wang, G. (2020). Predictive Analyses of Prognostic-Related Immune Genes and Immune Infiltrates for Glioblastoma. Diagnostics 10, 177. doi:10.3390/diagnostics10030177
Liu, C., Dai, L., Liu, Y., Dou, D., Sun, Y., and Ma, L. (2018). Pharmacological Activities of Mogrosides. Future Med. Chem. 10, 845–850. doi:10.4155/fmc-2017-0255
Liu, C., Dai, L., Liu, Y., Rong, L., Dou, D., Sun, Y., et al. (2016). Antiproliferative Activity of Triterpene Glycoside Nutrient from Monk Fruit in Colorectal Cancer and Throat Cancer. Nutrients 8, 360. doi:10.3390/nu8060360
Liu, H., Tang, C., and Yang, Y. (2021). Identification of Nephrogenic Therapeutic Biomarkers of Wilms Tumor Using Machine Learning. J. Oncol. 2021. doi:10.1155/2021/6471169
Longato, E., Vettoretti, M., and Di Camillo, B. (2020). A Practical Perspective on the Concordance index for the Evaluation and Selection of Prognostic Time-To-Event Models. J. Biomed. Inform. 108, 103496. doi:10.1016/j.jbi.2020.103496
Mayakonda, A., Lin, D.-C., Assenov, Y., Plass, C., and Koeffler, H. P. (2018). Maftools: Efficient and Comprehensive Analysis of Somatic Variants in Cancer. Genome Res. 28, 1747–1756. doi:10.1101/gr.239244.118
Miller, A. M., Shah, R. H., Pentsova, E. I., Pourmaleki, M., Briggs, S., Distefano, N., et al. (2019). Tracking Tumour Evolution in Glioma through Liquid Biopsies of Cerebrospinal Fluid. Nature 565, 654–658. doi:10.1038/s41586-019-0882-3
Nie, J., Sui, L., Zhang, H., Zhang, H., Yan, K., Yang, X., et al. (2019). Mogroside V Protects Porcine Oocytes from In Vitro Ageing by Reducing Oxidative Stress through SIRT1 Upregulation. Aging 11, 8362–8373. doi:10.18632/aging.102324
Petitprez, F., Levy, S., Sun, C. M., Meylan, M., Linhard, C., Becht, E., et al. (2020). The Murine Microenvironment Cell Population Counter Method to Estimate Abundance of Tissue-Infiltrating Immune and Stromal Cell Populations in Murine Samples Using Gene Expression. Genome Med. 12, 86–15. doi:10.1186/s13073-020-00783-w
Reimand, J., Isserlin, R., Voisin, V., Kucera, M., Tannus-Lopes, C., Rostamianfar, A., et al. (2019). Pathway Enrichment Analysis and Visualization of Omics Data Using g:Profiler, GSEA, Cytoscape and EnrichmentMap. Nat. Protoc. 14, 482–517. doi:10.1038/s41596-018-0103-9
Ru, J., Li, P., Wang, J., Zhou, W., Li, B., Huang, C., et al. (2014). TCMSP: a Database of Systems Pharmacology for Drug Discovery from Herbal Medicines. J. Cheminform. 6, 13–16. doi:10.1186/1758-2946-6-13
Smyth, G. K. (2005). Limma: Linear Models for Microarray Data, Bioinformatics and Computational Biology Solutions Using R and Bioconductor. Springer, 397–420.
Sprowls, S. A., and Lathia, J. D. (2021). Neutralizing Shapeshifting Pericytes Enhances Glioblastoma Therapeutic Efficacy. Cell Res, 1–2. doi:10.1038/s41422-021-00538-1
Szklarczyk, D., Gable, A. L., Nastou, K. C., Lyon, D., Kirsch, R., Pyysalo, S., et al. (2021). The STRING Database in 2021: Customizable Protein-Protein Networks, and Functional Characterization of User-Uploaded Gene/measurement Sets. Nucleic Acids Res. 49, D605–D612. doi:10.1093/nar/gkaa1074
Wang, J., Qi, F., Wang, Z., Zhang, Z., Pan, N., Huai, L., et al. (2019). A Review of Traditional Chinese Medicine for Treatment of Glioblastoma. Bst 13, 476–487. doi:10.5582/bst.2019.01323
Wang, X., Shen, Y., Wang, S., Li, S., Zhang, W., Liu, X., et al. (2017). PharmMapper 2017 Update: a Web Server for Potential Drug Target Identification with a Comprehensive Target Pharmacophore Database. Nucleic Acids Res. 45, W356–W360. doi:10.1093/nar/gkx374
Wishart, D. S., Feunang, Y. D., Guo, A. C., Lo, E. J., Marcu, A., Grant, J. R., et al. (2018). DrugBank 5.0: a Major Update to the DrugBank Database for 2018. Nucleic Acids Res. 46, D1074–D1082. doi:10.1093/nar/gkx1037
Wu, W., Klockow, J. L., Zhang, M., Lafortune, F., Chang, E., Jin, L., et al. (2021). Glioblastoma Multiforme (GBM): An Overview of Current Therapies and Mechanisms of Resistance. Pharmacol. Res. 171, 105780. doi:10.1016/j.phrs.2021.105780
Xia, M., Han, X., He, H., Yu, R., Zhen, G., Jia, X., et al. (2018). Improved De Novo Genome Assembly and Analysis of the Chinese Cucurbit Siraitia Grosvenorii, Also Known as Monk Fruit or Luo-han-guo. GigaScience 7, giy067. doi:10.1093/gigascience/giy067
Xia, X., Zhong, Z., Xiao, Y., and Liang, L. (2013). Protective Effect of Mogroside against H_2O_2 Induced Apoptosis in PC12 Cell. Chin. J. Hosp. Pharm. 33, 786–789.
Yin, J., Zeng, A., Zhang, Z., Shi, Z., Yan, W., and You, Y. (2019). Exosomal Transfer of miR-1238 Contributes to Temozolomide-Resistance in Glioblastoma. EBioMedicine 42, 238–251. doi:10.1016/j.ebiom.2019.03.016
Yu-Ju Wu, C., Chen, C.-H., Lin, C.-Y., Feng, L.-Y., Lin, Y.-C., Wei, K.-C., et al. (2020). CCL5 of Glioma-Associated Microglia/macrophages Regulates Glioma Migration and Invasion via Calcium-dependent Matrix Metalloproteinase 2. Neuro Oncol. 22, 253–266. doi:10.1093/neuonc/noz189
Zanders, E. D., Svensson, F., and Bailey, D. S. (2019). Therapy for Glioblastoma: Is it Working? Drug Discov. Today 24, 1193–1201. doi:10.1016/j.drudis.2019.03.008
Zhang, X.-N., Yang, K.-D., Chen, C., He, Z.-C., Wang, Q.-H., Feng, H., et al. (2021). Pericytes Augment Glioblastoma Cell Resistance to Temozolomide through CCL5-CCR5 Paracrine Signaling. Cel Res, 1–16. doi:10.1038/s41422-021-00528-3
Glossary
ADSL adenylosuccinate lyase
AKT1 AKT serine/threonine kinase 1
AMPK AMP-activated protein kinase;
BAX BCL2 associated X, apoptosis regulator
BBB blood-brain barrier
c-index concordance index
CCL5 C-C motif chemokine ligand 5
CANT1 calcium activated nucleotidase 1
CDK2 CDKCyclin dependent kinase 2
CCND1 cyclin D1
DEGs differentially expressed genes
DL drug-like
DE-GBMs differentially expressed GBMs
EMT emergency medical technician
E2F1 E2F transcription factor 1
FOSB FosB proto-oncogene, AP-1 transcription factor subunit
GBM glioblastoma
GO gene ontology
HR hazard ratios
KEGG kyoto encyclopedia of genes and Genomes
logFC logFoldChange
MDM2 MDM2 proto-oncogene
multi-cox multivariate cox
OB oral bioavailability
PPI protein-protein interaction
POT1 protection of telomeres 1
PRKCB protein kinase C beta
RELA RELA proto-oncogene, NF-KB subunit
RAC1 Rac family small GTPase 1
ROC receiver operating characteristic curve
SG Siraitia grosvenorii
SG-TMZ-GBM Siraitia grosvenorii - temozolomide – glioblastoma
STAT3 signal transducer and activator of transcription 3
SIRT1 sirtuin 1
TP53 tumor protein P53
TCM traditional chinese medicine
TMZ temozolomide; uni-cox, univariable Cox
VEGFA vascular endothelial growth factor A
VEGF vascular endothelial growth factor
Keywords: Siraitia grosvenorii, CCL5, glioblastoma, in silico, network pharmacology
Citation: Li J, Bi D, Zhang X, Cao Y, Lv K and Jiang L (2021) Network Pharmacology and Inflammatory Microenvironment Strategy Approach to Finding the Potential Target of Siraitia grosvenorii (Luo Han Guo) for Glioblastoma. Front. Genet. 12:799799. doi: 10.3389/fgene.2021.799799
Received: 22 October 2021; Accepted: 15 November 2021;
Published: 20 December 2021.
Edited by:
Meng Zhou, Wenzhou Medical University, ChinaReviewed by:
Fuhai Li, Washington University in St. Louis, United StatesShuanglong Yi, ShanghaiTech University, China
Wei Li, Central South University, China
Copyright © 2021 Li, Bi, Zhang, Cao, Lv and Jiang. This is an open-access article distributed under the terms of the Creative Commons Attribution License (CC BY). The use, distribution or reproduction in other forums is permitted, provided the original author(s) and the copyright owner(s) are credited and that the original publication in this journal is cited, in accordance with accepted academic practice. No use, distribution or reproduction is permitted which does not comply with these terms.
*Correspondence: Lan Jiang, amlhbmdsYW5oaUAxNjMuY29t; Kun Lv, bHZrdW5AeWpzeXkuY29t; Yunpeng Cao, eGZjeXBlbmdAMTI2LmNvbQ==
†These authors share first authorship