- 1Department of Urology, Peking University First Hospital, Beijing, China
- 2Beijing Key Laboratory of Urogenital Diseases (male) Molecular Diagnosis and Treatment Center, Beijing, China
- 3Urology and Lithotripsy Center, Peking University People’s Hospital, Peking University, Beijing, China
- 4Peking University Applied Lithotripsy Institute, Peking University, Beijing, China
Background: N6-methyladenosine (m6A) is the most extensive messenger RNA modification. Despite recent advances in the biological roles of m6A, its role in the development and progression of renal cell carcinoma (RCC) remains unclear.
Methods: In this study, we gained the transcriptome-wide m6A profile and gene expression pattern in RCC and paired adjacent peritumoral tissues by meRIP-seq and RNA-seq. m6A modifications of mRNAs were validated by meRIP-qPCR in tissues, and targeted methylation or demethylation was validated by using a CRISPR-Cas13b-based tool in RCC cell lines.
Results: Our findings showed that there were 13,805 m6A peaks among 5,568 coding gene transcripts (mRNAs) in adjacent tissues and 24,730 m6A peaks among 6,866 mRNAs in tumor tissues. Furthermore, m6A modification sites were usually located in the coding sequences (CDS), and some near the start and stop codons. Gene Ontology analysis revealed that coding genes had differential N6-methyladenosine sites and were enriched in kidney development and cancer-related signaling pathways. We also found that different levels of m6A modifications could regulate gene expression.
Conclusion: In summary, our results provided evidence for studying the potential function of RNA m6A modification and m6A-mediated gene expression regulation in human RCC.
Introduction
Renal cell carcinoma (RCC) is one of the most common malignancies in the genitourinary system. Kidney cancer is the sixth most common cancer in men, with 73,820 estimated new cases and 14,770 estimated deaths a year in the United States, according to the latest cancer data (Siegel et al., 2019). Clear cell renal cell carcinoma (ccRCC) is the most common type of renal cell carcinoma, accounting for 80%. In clinical practice, 16% of ccRCCs were diagnosed with metastasis at the initial time, with a 5-year survival rate of 12% (Rydzanicz et al., 2013). Although the oncology research and surgical treatment of RCC have developed rapidly, the prognosis of RCC has not improved significantly. For RCC in situ, 20%–30% of patients relapse after initial surgical treatment, and no treatment has been shown to reduce tumor recurrence and improve prognosis (Patel et al., 2016). In recent years, targeted agents have been shown to prolong survival and prognosis in patients with metastasis, but the median survival is still less than 3 years. Besides, in clinical practice, drug resistance and economic burden are still two prominent problems. Therefore, the study on the pathological mechanism and new therapeutic targets of RCC is still a challenging exploration.
The role of RNA in a variety of cellular processes has attracted a lot of attention and has become a rapidly developing field in the past decade. More than 100 chemically modified nucleotides have been found in different types of RNAs, such as mRNA, tRNA, rRNA, snRNA, etc. The modified RNA plays a crucial role in the post-transcriptional regulation of gene expression. In eukaryotes, m6A is the most common form of mRNA modification, whose abundance accounts for 0.1%–0.4% of the total adenosine residues (Meyer et al., 2012; Zhu H et al., 2019). In general, m6A is highly conserved between humans and mice, located in the 3′ terminal non-coding region, near the stop codon and long internal exons, and is closely associated with altered RNA stability, intracellular distribution, splicing, and translation (Csepany et al., 1990; Dominissini et al., 2012; Meyer et al., 2012). The cellular m6A state is regulated by a set of genes called “writers” (METTL3, METTLL4, and WTAP), “erasers” (FTO and ALKBH5), and “readers” (YTHDF2, YTHDF2, YTHDF3, YTHDCL, and YTHDC2) (Fustin et al., 2013; Batista et al., 2014; Lin et al., 2016; Zhang et al., 2016; Bertero et al., 2018). The writer forms a multisubunit methyltransferase complex that could upregulate m6A levels, while the eraser could downregulate m6A levels, making this event reversible (Meyer et al., 2012; Dominissini et al., 2012).
In addition, it was demonstrated that METTL3, a major RNA N6-adenosine methyltransferase, could promote hepatocellular carcinoma progression through YTHDF2-dependent post-transcriptional silencing of SOCS2. Although the m6A modification of RNA has been reported to be associated with the occurrence of different types of cancer, the relationship between m6A-related genes and RCC remains unclear (Aguilo et al., 2015; Shah et al., 2017; He et al., 2018; Panneerdoss et al., 2018; Zhu W et al., 2019; Fukumoto et al., 2019; Zhang et al., 2019). In this study, we used m6A-RIP-seq and RNA-seq to research the m6A modification profile and mRNA expression profile in RCC. In addition, we performed CRISPR-dCas13b fusion proteins to target methylation or demethylation of differentially methylated mRNAs (Wilson et al., 2020; Li et al., 2020a). This proved that abnormal RNA m6A modifications could directly modulate gene expressions in RCC. Finally, we hope this study would facilitate further investigations of potential functions of m6A modification in RCC pathogenesis.
Materials and Methods
Patients and Samples
Five pairs of primary renal cell carcinoma (RCC) samples and adjacent peritumoral tissues with informed consent of the patients were gained in the Urology Department of Peking University First Hospital (PKUFH), Beijing, China. This study followed the Helsinki declaration and was approved by the Institutional Ethical Review Board of PKUFH. Samples were obtained immediately after surgical resection and stored in liquid nitrogen after rapid freezing for the subsequent RNA isolation.
RNA m6A and mRNA Sequencing
MeRIP-seq and RNA-seq were performed by Cloudseq Biotech, Inc. (Shanghai, China) as described previously (Dominissini et al., 2013). Briefly, total RNAs were extracted from five pairs of tumor and adjacent peritumoral tissues using TRIzol (Invitrogen). Next, total RNAs were broken into almost 100 nt fragments and were incubated with anti-m6A antibody (Manga) for 2 h at 4°C. The beads were prepared and incubated with the total RNAs for 2 h at 4°C. Then the mixture was washed, and the m6A-bound RNA was purified with TE buffer. After purification, the library was constructed by Prep Kit (Illumina) on the Hiseq 3000.
Sequencing Data Analysis
After obtaining the sequencing data of control and IP samples, the read segment data should be preprocessed (such as filtering the read segment with poor sequencing quality), and then all the read segment sequence mapping of the two samples should be positioned on the reference genome, which is the basis of subsequent data processing and analysis. Then there were many read segments captured by methylation sites in the IP samples, which would be mapped to the reference genome to form a reading segment enrichment region or a “peak” near the methylation sites. Therefore, the methylation enrichment point detection algorithm is called the peak calling algorithm. The m6A methylated peaks among the transcripts were identified by MACS (Zhang et al., 2008), and metagene m6A distribution was researched by MetaPlotR (Olarerin-George and Jaffrey, 2017). The DMGs were identified by diffReps (Shen et al., 2013). To explore the DMGs and DEGs from MeRIP-seq and RNA-seq, the Gene Ontology (GO) analysis and KEGG pathway enrichment analysis were performed.
M6A-IP-qPCR and Reverse Transcription-qPCR
Ten genes with differentially methylated sites were verified by reverse transcription (RT)-qPCR according to m6A-seq. A small number of fragmented RNA was tested as the input control, while the rested RNA was incubated with anti-m6A antibody-coupled beads. Then the immunoprecipitation complex was eluted from the beads. In the end, RT-qPCR was performed on the input control and m6A -IP samples with gene-specific primers. Primers are listed in Supplementary Table S1.
Cell Line Culture and Plasmid Transfection
In this study, RCC cell lines, including 786-O, ACHN, and OSRC, and human kidney proximal tubular epithelial cells HK-2 were used. These cell lines were purchased from the American Type Culture Collection (ATCC, Manassas, VA, United States) and National Infrastructure of Cell Line Resource, China. Cell lines were cultured in RPMI 1640 or DMEM with 10% fetal bovine serum (Invitrogen, Carlsbad, CA, United States) and incubated in a 5% CO2 environment at 37°C. The plasmids were transfected with Lipo3000 (Invitrogen) according to the protocol of the manufacturer, and the dosage of plasmids was 1 μg.
Statistical Analysis
All statistical analyses were achieved and visualized using RStudio (version1.2.1335, Boston, MA, United States), GSEA (version4.0, UC San Diego and Broad Institute, United States) 23, Medcalc (version16.8, Ostend, Belgium), and GraphPad Prism (version 8.0, GraphPad, Inc., La Jolla, CA, United States). A two-tailed p < 0.05 was considered statistically significant.
Results
The m6A Content was Changed in Renal Cancer Cell
Recently, it has been found that RNA methylation could promote the progression of RCC (Dai et al., 2018). In order to explore the potential role of m6A modification in RCC, we measured the m6A content of total RNA in five pairs of tumor tissues and adjacent tissues using the m6A quantitative kit. We found the content was higher in tumor tissues than those in their corresponding normal tissues (Figure 1A). At the same time, we also examined the m6A contents of normal renal cell line HK-2 and renal cancer cell lines (786-O, OSRC, and ACHN), and found that m6A contents were also increased in RCC cells compared with normal cells (Figure 1B). m6A methyltransferase or demethylase could catalyze the modification of m6A. Therefore, we speculated that the abnormal m6A content might be caused by the abnormal expression of m6A methyltransferase or demethylase in RCC. In addition, many proteins have been found to be associated with m6A modification (Schwartz et al., 2014). To investigate our assumption, we examined the mRNA levels of eight genes associated with m6A modification in tissues and cell lines by RT-qPCR. Finally, the results showed that the mRNA expression levels of METL14 and ALKBH5 were increased in tumor tissues and RCC cell lines, while the mRNA expression levels of other genes were not significantly changed (Figures 1C,D).
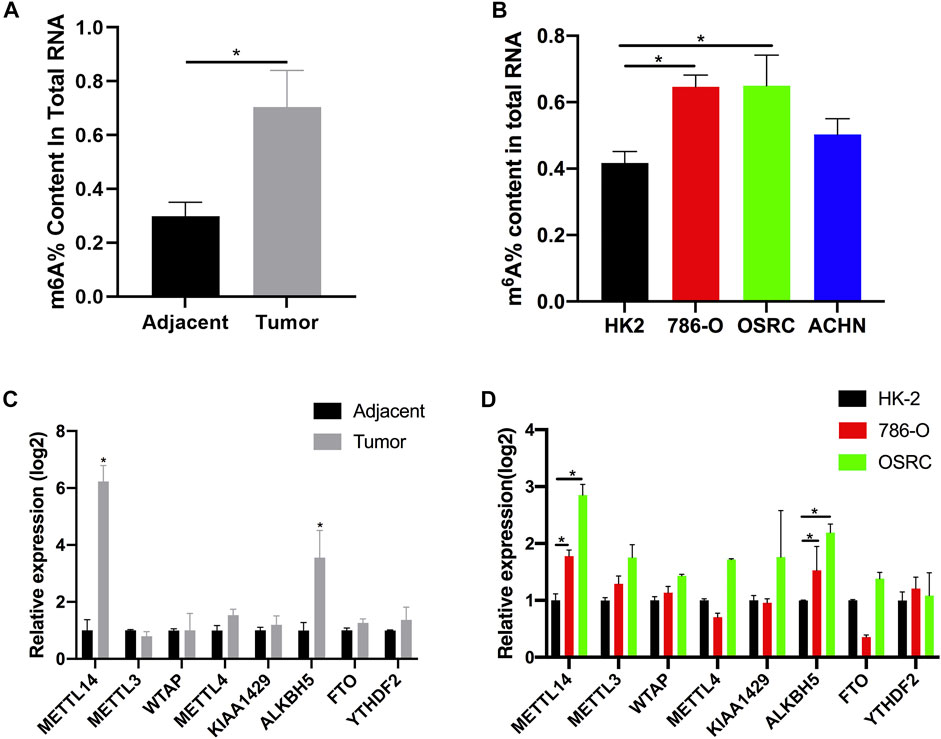
FIGURE 1. The N6-methyladenosine (m6A) contents and modification enzymes expression in renal cell carcinoma (RCC) tissues and RCC cells. (A) The m6A contents of total RNAs in tumor and adjacent tissues (n = 5). (B) The m6A contents of total RNAs in normal renal cell line (HK-2) and RCC cell lines (786-O, OSRC, and ACHN). (C) The mRNA expression levels of m6A modification enzymes in RCC and adjacent tissues (n = 5). (D) The mRNA expression levels of m6A modification enzymes in normal renal cell line (HK-2) and RCC cell lines (786-O and OSRC). *p < 0.05.
The mRNA m6A Modification was Dynamic and Differed Between Paired Renal Tumor Tissues and Adjacent Tissues
In order to investigate whether m6A modification could promote the progression of RCC, we used MeRIP-seq (Dominissini et al., 2013) to detect the differences of m6A level in the paired renal tumor tissues and adjacent tissues. MeRIP-seq analysis revealed that there were 13,805 m6A peaks among 5,568 coding gene transcripts (mRNAs) in adjacent tissues, and 24,730 m6A peaks among 6,866 mRNAs in tumor tissues. Of these, 11,510 peaks were overlapped between the adjacent tissues and tumor tissues (Figure 2A). The low overlapping m6A peaks of mRNAs suggested that there were differences in the m6A patterns between the two groups. To study whether the m6A peaks had a conserved motif (Wei et al., 1976; Schibler et al., 1977), the m6A peaks identified from the MeRIP-seq were analyzed by the HOMER motif software (Heinz et al., 2010). The results showed that there was a difference of m6A motif between tumor tissues and adjacent tissues, while their motifs were similar to those previously reported (Fu et al., 2014; Chen et al., 2015) (Figure 2B). Besides, we examined the distribution of m6A modifications in the human transcriptome. We found that 70% of methylated sequences in the adjacent group (60% of methylated transcripts in the tumor group) contained fewer than five m6A sites, while fewer transcripts contained six or more sites (Figure 2C).
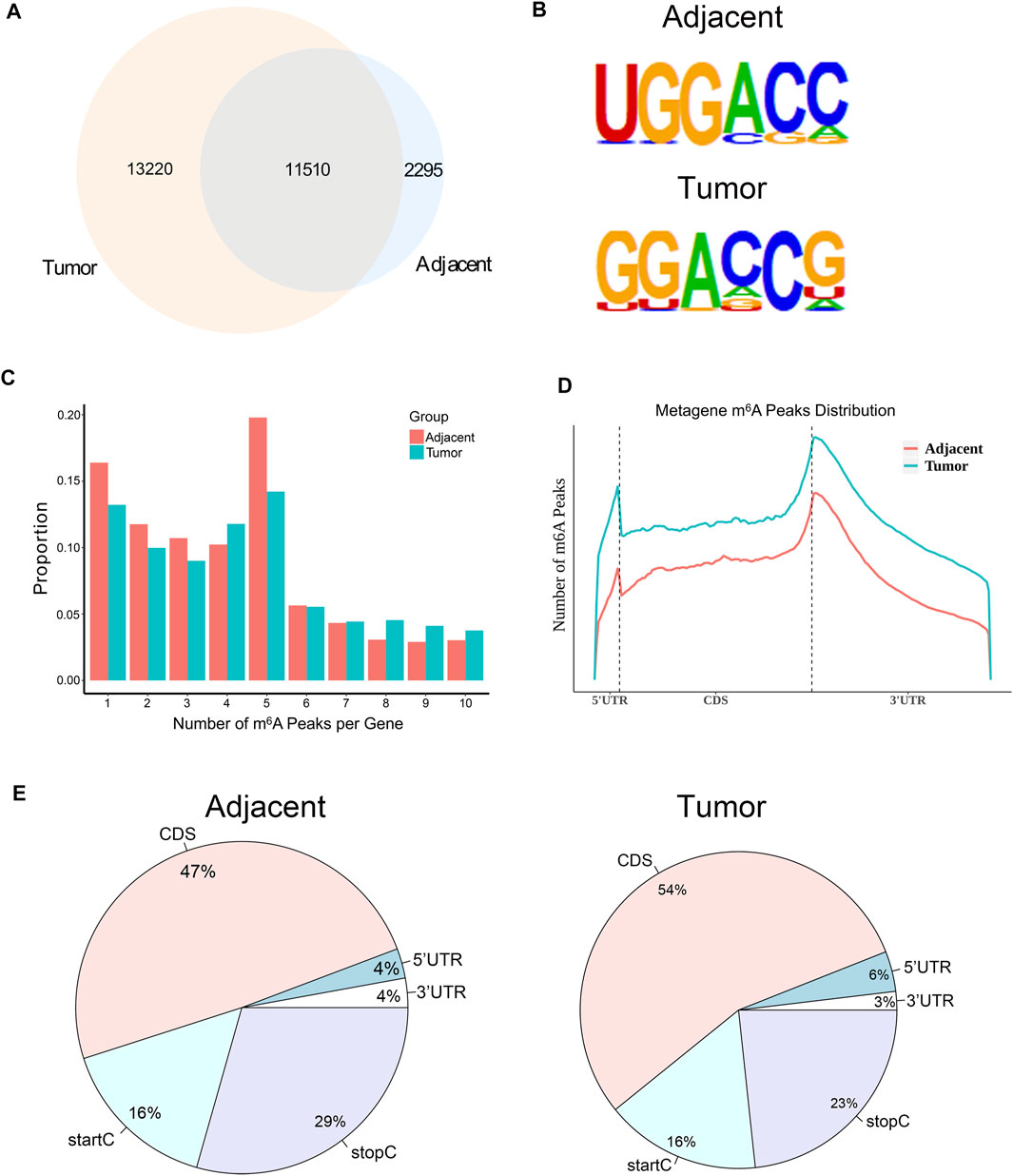
FIGURE 2. The mRNA m6A profile of five paired renal tumor tissues and adjacent tissues. (A) The Venn diagram showing the numbers of m6A peaks in tumor and adjacent tissues. (B) The sequence motif of m6A-containing peak regions in tumor and adjacent tissues, respectively. (C) The distribution of m6A modifications in the human transcriptome. (D) The metagene profiles of transcripts peaks in RCC tissues and adjacent tissues. (E) The proportion of m6A peaks located at transcripts. startC, start codon; stopC, stop codon.
In order to determine the priority position of m6A in transcripts, we then studied the metagene profiles of the peaks in RCC tissues and adjacent normal tissues. We found that most m6A peaks were located at the end of the 5′UTRs and start of the 3′UTRs (Figure 2D). At the same time, we found that the proportion of m6A peaks in CDS was the highest and that in UTRs was the lowest in both tissues (Figure 2E). These results of the m6A modification distributions were similar to those reported previously (Dominissini et al., 2012; Meyer et al., 2012).
mRNA Containing Differential m6A Sites was Enriched in Kidney Development and Cancer-Related Signaling Pathways
Totally, we had identified 4,404 differential m6A sites (DMMSs) within 1,877 nuclear mRNAs, of which 43% (1,887/4,404) were significantly downmethylated sites (tumor vs. adjacent). Compared with the adjacent group, we found 923 significantly hypomethylated coding genes and 954 significantly hypermethylated coding genes in the tumor group (Figure 3A). Besides, we analyzed the relative density of differential m6A modification sites on chromosomes; the top three chromosomes were 19, 17, and 22 (Figure 3B). To explore the functions of m6A in RCC, differentially methylated genes were performed for Gene Ontology enrichment analysis and Kyoto Encyclopedia of Genes and Genomes pathway analysis. The results indicated that differentially methylated genes in the tumor group were mostly enriched in many system development-associated processes (Figures 3C,D). Furthermore, differentially methylated genes were found to be significantly involved in cancer pathways, such as the transcriptional misregulation and TGF-β signaling pathway (Figures 3E,F).
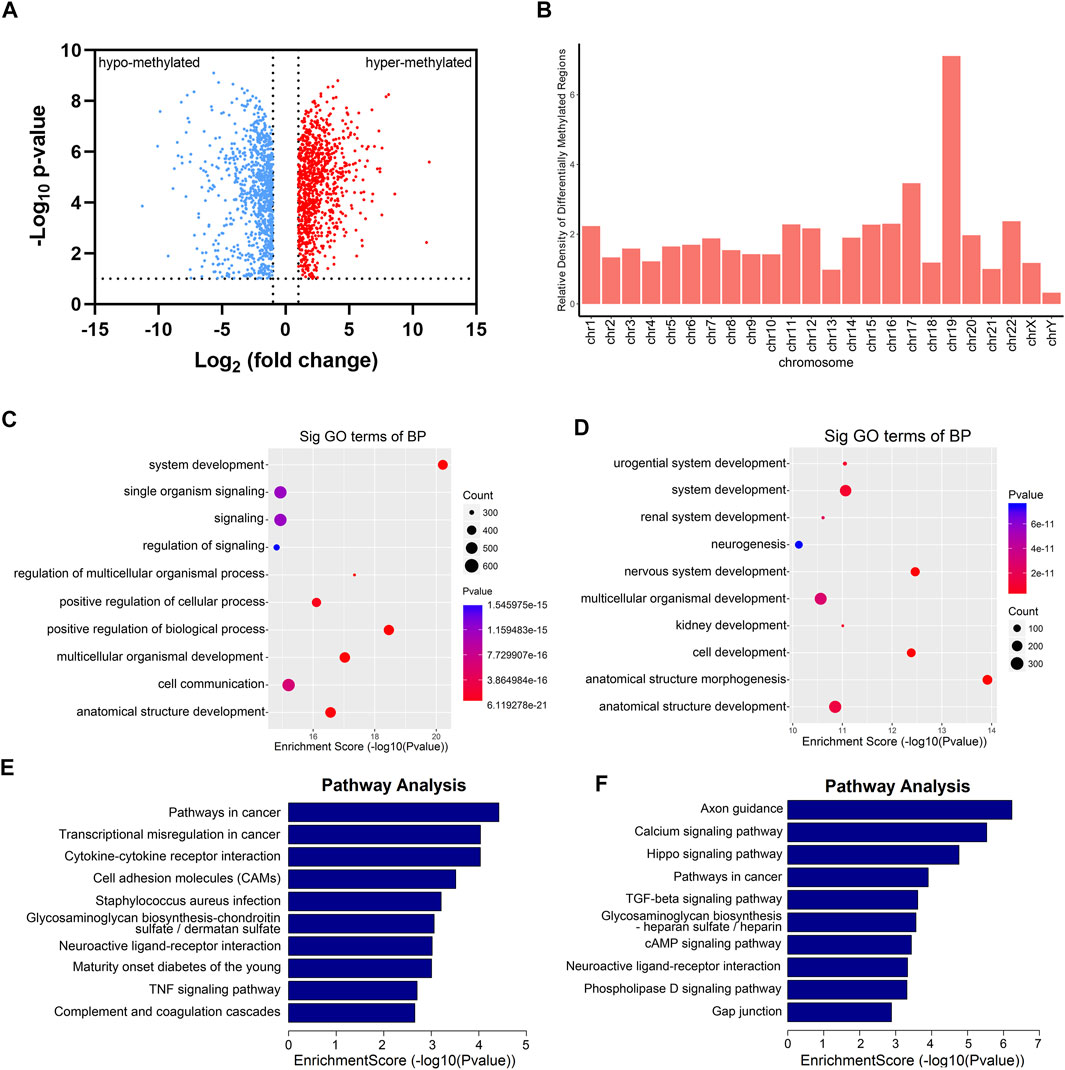
FIGURE 3. Gene Ontology (GO) and KEGG analysis of DMGs between five paired renal tumor and adjacent tissues. (A) Volcano blot of differentially methylated mRNAs (DMGs) in tumor compared with adjacent tissues. (B) The container of differentially methylated m6A sites harbored by different chromosomes. (C) GO analysis of hypermethylated genes in RCC tumor tissues. (D) GO analysis of hypomethylated genes in RCC tumor tissues. (E) KEGG analysis of hypermethylated genes in RCC tumor tissues. (F) KEGG analysis of hypomethylated genes in RCC tumor tissues.
Differentially Expressed RNAs were Involved in Important Biological Pathways
From the RNA-seq data, we found that 1,469 genes were upregulated in tumor tissues, and 1,402 were upregulated in adjacent tissues (Figures 4A,B). Next, we performed Gene Ontology enrichment analysis and Kyoto Encyclopedia of Genes and Genomes pathway analysis to investigate the function of the differentially expressed RNAs. We found that the differentially expressed RNAs were mainly related to single-organism process and urogenital system development (Figures 4C,D). Moreover, pathway analysis showed that systemic lupus erythematosus, tight junction, and MAPK signaling pathway were significantly changed in tumor samples (Figures 4E,F).
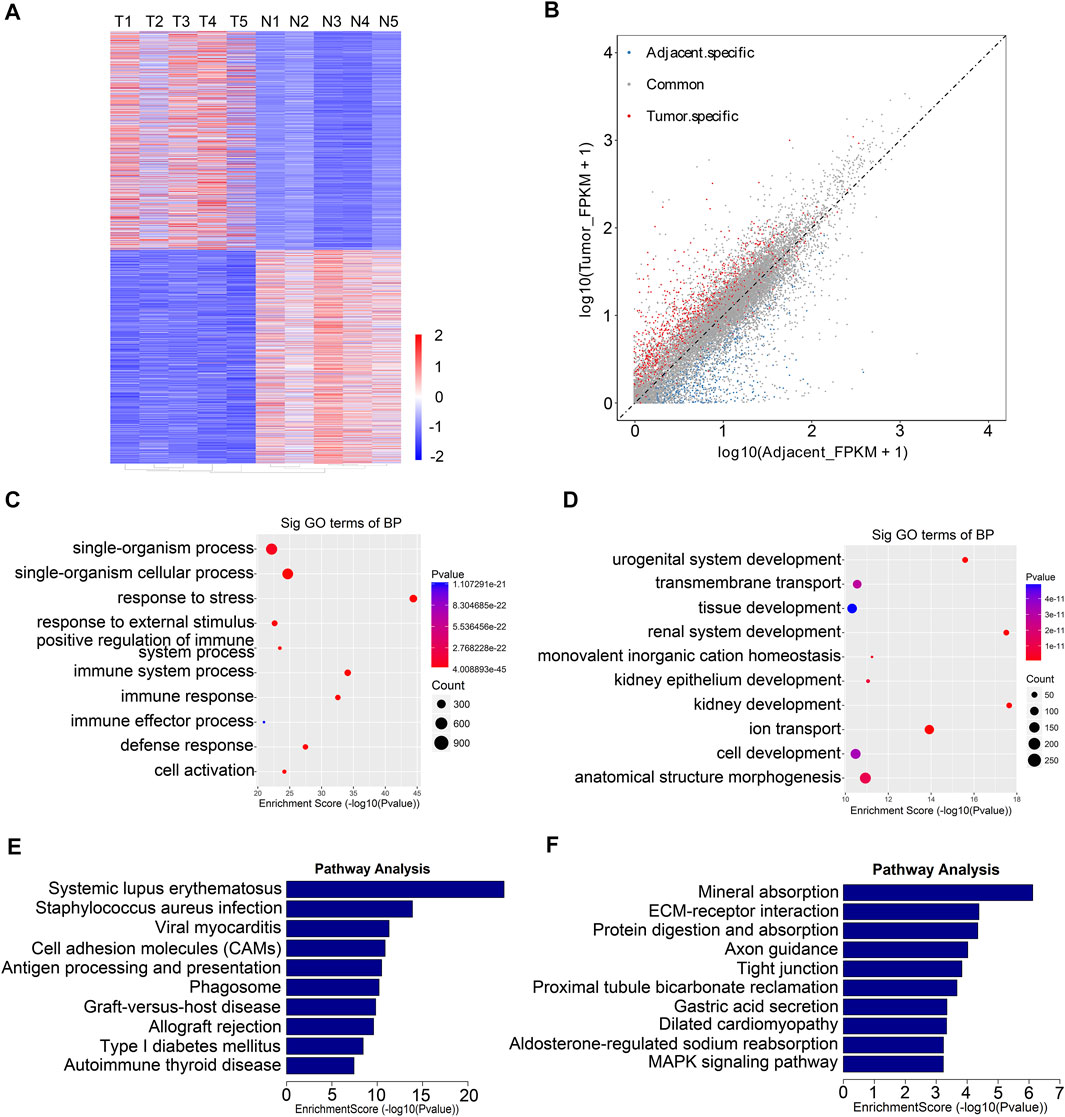
FIGURE 4. Functional analysis of differentially expressed genes between five paired renal tumor and adjacent tissues. (A) Heatmap of differentially expressed mRNAs in renal tumor tissues and adjacent tissues (T, tumor group; N, adjacent normal group). (B) Scatter plots showing the tissue specific DEGs (fold changes ≥2 and p < 0.05). (C) GO analysis of upregulated genes in RCC tumor tissues. (D) GO analysis of downregulated genes in RCC tumor tissues. (E) KEGG analysis of upregulated genes in RCC tumor tissues. (F) KEGG analysis of downregulated genes in RCC tumor tissues.
Conjoint Analysis of MeRIP-Seq and RNA-Seq
After conjoint analysis of meRIP-seq and RNA-seq, 369 hyper-up genes, 372 hypo-down genes, 14 hyper-down genes, and two hypo-up genes were found in RCC tissues compared with adjacent tissues (Figure 5A). This result promoted us to investigate the cancer-related genes in the RCC. For instance, cell division cycle-associated 2 (CDCA2) promotes the proliferation and development of colon cancer, within which m6A was hypermethylated (tumor vs. adjacent) near the stop codon (Figure 5B) (Feng et al., 2019), and the m6A peaks of choline-O-acetyltransferase (CHAT) (Yokomori et al., 1983; Pahud et al., 2001) were enriched around the 5′UTR of CHAT in tumors (Figure 5C), while mucin 15 (MUC15), a significantly hypermethylated peak enriched in coding sequence (CDS) was shown in the adjacent groups (Figure 5D), which had been reported to promote the progression of cancer (Zhang et al., 2020). It was reported that HRG could prevent the cancer development by inducing macrophage polarization (Rolny et al., 2011), and its m6A peak was enriched around the beginning of the 3′UTR in the adjacent groups in our study (Figure 5E).
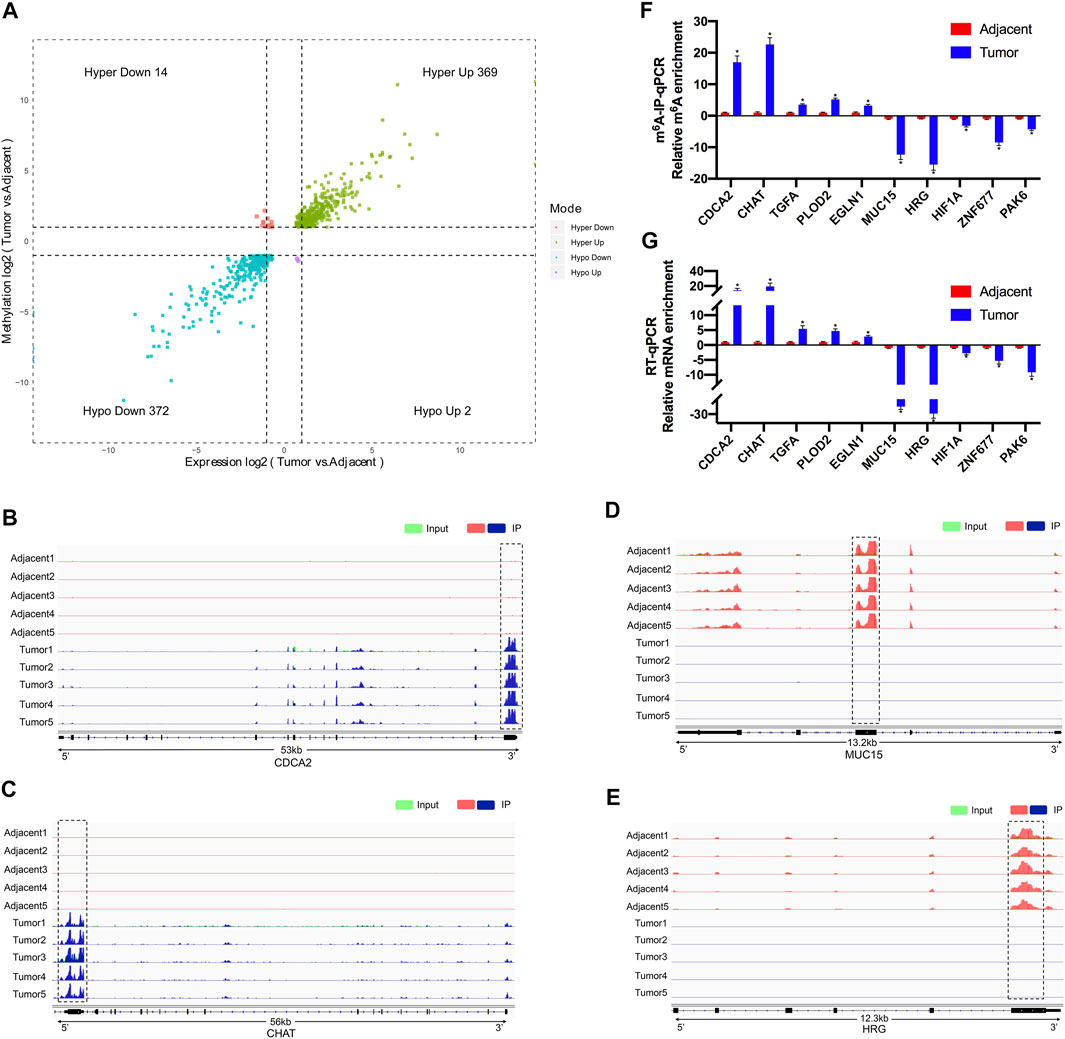
FIGURE 5. Conjoint analysis of differentially methylated genes and differentially expressed genes between five paired renal tumor and adjacent tissues. (A) Four quadrant diagram showing the differentially methylated genes and differentially expressed genes. (B,C) Integrative Genome Viewer (IGV) software showing the m6A peaks in hypermethylated and upregulated gene cell division cycle-associated 2 (CDCA2) (B) and choline-O-acetyltransferase (CHAT) (C). (D,E) Integrative Genome Viewer (IGV) software showed the m6A peaks in hypomethylated and downregulated genes mucin 15 (MUC15) (D) and histidine-rich glycoprotein (HRG) (E). (F) Validations of the m6A enrichment of five hypermethylated genes (CDCA2, CHAT, TGFA, PLOD2, and EGLN1) and five hypomethylated genes (MUC15, HRG, HIF1A, ZNF677, and PAK6) by m6A-immunoprecipitation (IP)-qPCR (n = 5). (G) Validations of the mRNA expression level of five upregulated genes (CDCA2, CHAT, TGFA, PLOD2, and EGLN1) and five downregulated genes (MUC15, HRG, HIF1A, ZNF677, and PAK6) by RT-qPCR (n = 5). *p < 0.05.
To further confirm the results of our m6A-seq data, we analyzed gene-specific m6A-IP qPCR assays for several hypermethylated genes (CDCA2, CHAT, TGFA, PLOD2, and EGLN1) and hypomethylated genes (MUC15, HRG, HIF1A, ZNF677, and PAK6), which might participate in RCC development. We verified the same m6A level changes of 10 genes, confirming the validity of the meRIP-seq results (Figure 5F). Next, mRNA levels of the abovementioned 10 genes were measured in the five pairs of adjacent and tumor samples by RT-qPCR (Figure 5G). Results showed a similar tendency of m6A-methylated levels and mRNA expressions in the two groups, which suggested a relationship between m6A mRNA methylation and gene transcription.
dCas13b-ALKBH5 Fusion Protein Induced Demethylation of m6A-Modified mRNAs in vitro
To study mRNA modifications in vitro, we performed dCas13b-ALKBH5 fusion protein for targeted mRNA demethylation (Li et al., 2020a). We designed two guide RNAs targeting the 3′UTR or CDS of CDCA2 mRNA, and another two gRNAs targeting 5′UTR or CDS of CHAT mRNA. These two mRNAs were hypermethylated in RCC, then all four gRNAs were transfected in 786-O RCC cell line with dCas13b-ALKBH5 fusion protein, respectively (Figure 6A). The results of m6A-IP qPCR revealed that the m6A levels of the targeted site were decreased after transfecting with dCas13b-ALKBH5 and gRNAs, compared with NT-gRNA (Figures 6B,C). Intriguingly, the mRNA expression levels of the targeted CDCA2 and CHAT were decreased after transfecting gRNAs (Figures 6D,E). YTHDF1, which is responsible for binding to the translational machinery and RNA translation activation, might be inhibited by dCas13b-ALKBH5 (Wang et al., 2015). Collectively, these results suggested that dCas13b-ALKBH5 could demethylate m6A levels of targeted hypermethylated mRNAs.
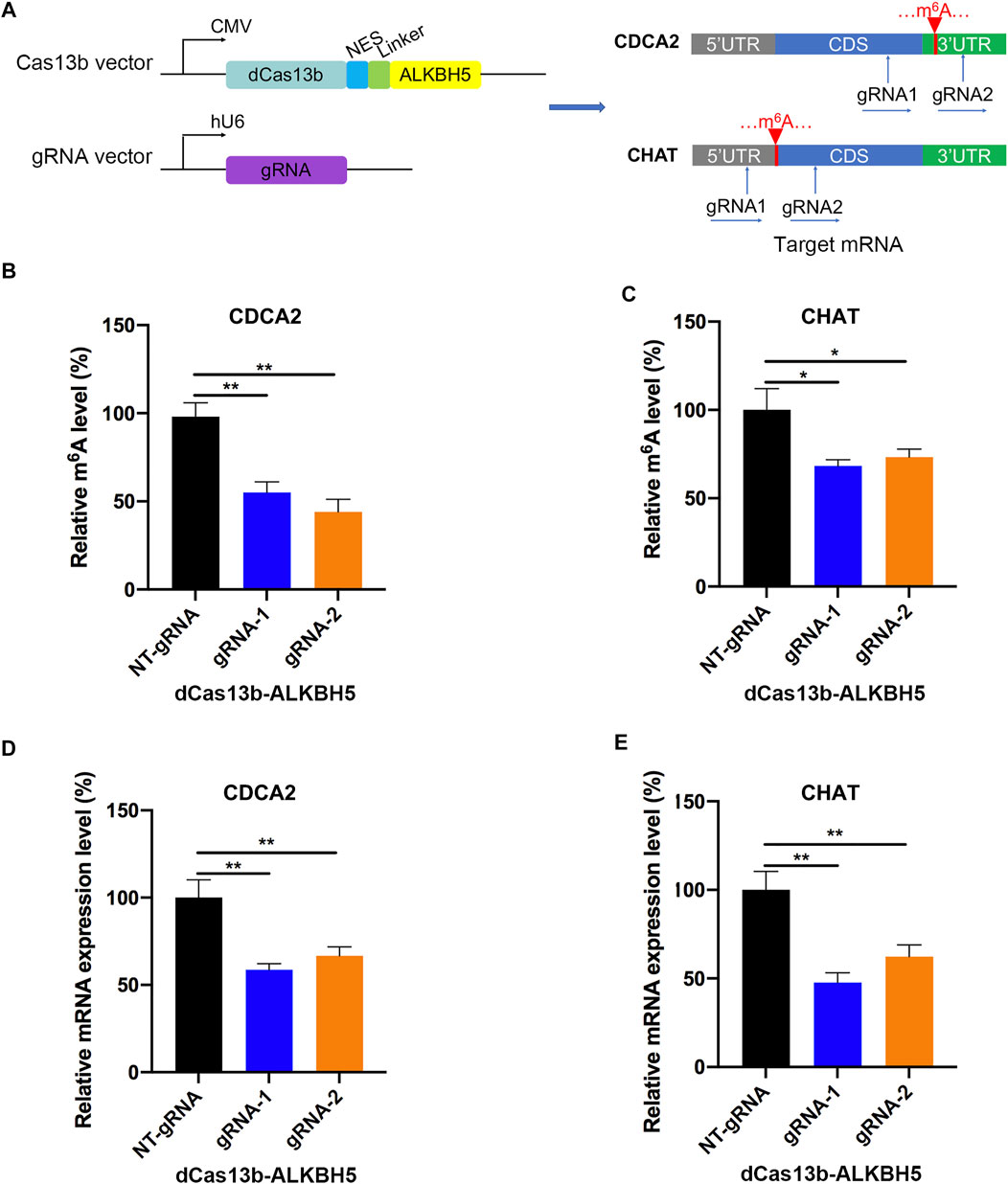
FIGURE 6. dCas13b-ALKBH5 induces demethylation of m6A hypermethylated mRNA in RCC cells. (A) The design of dCas13b-ALKBH5 fusion protein and sgRNAs targeted with CDCA2 and CHAT mRNA m6A sites. (B,C) The m6A level of CDCA2 (B) and CHAT (C) mRNA in RCC cell line 786-O after transfected with dCas13b-ALKBH5 and gRNAs. (D,E) The mRNA levels of CDCA2 (D) and CHAT (E) in 786-O cell after transfection with dCas13b-ALKBH5 and gRNAs. **p < 0.01.
dCas13b-M3M14 Fusion Protein Induced Methylation of m6A-Modified mRNAs in vitro
In addition, we constructed the dCas13b-M3M14 fusion protein (Wilson et al., 2020) to promote m6A modifications in RCC cells. The mRNAs of HRG and MUC15 were hypomethylated in RCC and were targeted by two gRNAs at different positions (Figure 7A). First, we measured the m6A levels of HRG and MUC15 in 786-O RCC cell lines transfected with dCas13b-M3M14 and gRNAs or NT-gRNAs. The results showed that dCas13b-M3M14 significantly increased the m6A levels of HRG and MUC15, suggesting that gRNAs could efficiently recognize their targeted mRNAs (Figures 7B,C). We then verified the effect of dCas13b-M3M14 fusion protein (gRNAs for HRG and MUC15) on the mRNA expressions of HRG and MUC15. The results of RT-qPCR showed that dCas13b-M3M14 fusion protein transfected with gRNAs significantly upregulated the mRNA levels of their targeted mRNAs (Figures 7D,E). This might be due to dCas13b-M3M14 fusion protein increasing the binding of targeted mRNA and YTHDF1. Finally, this dCas13b-M3M14 fusion protein could mediate efficient installation of m6A in endogenous RNA transcripts.
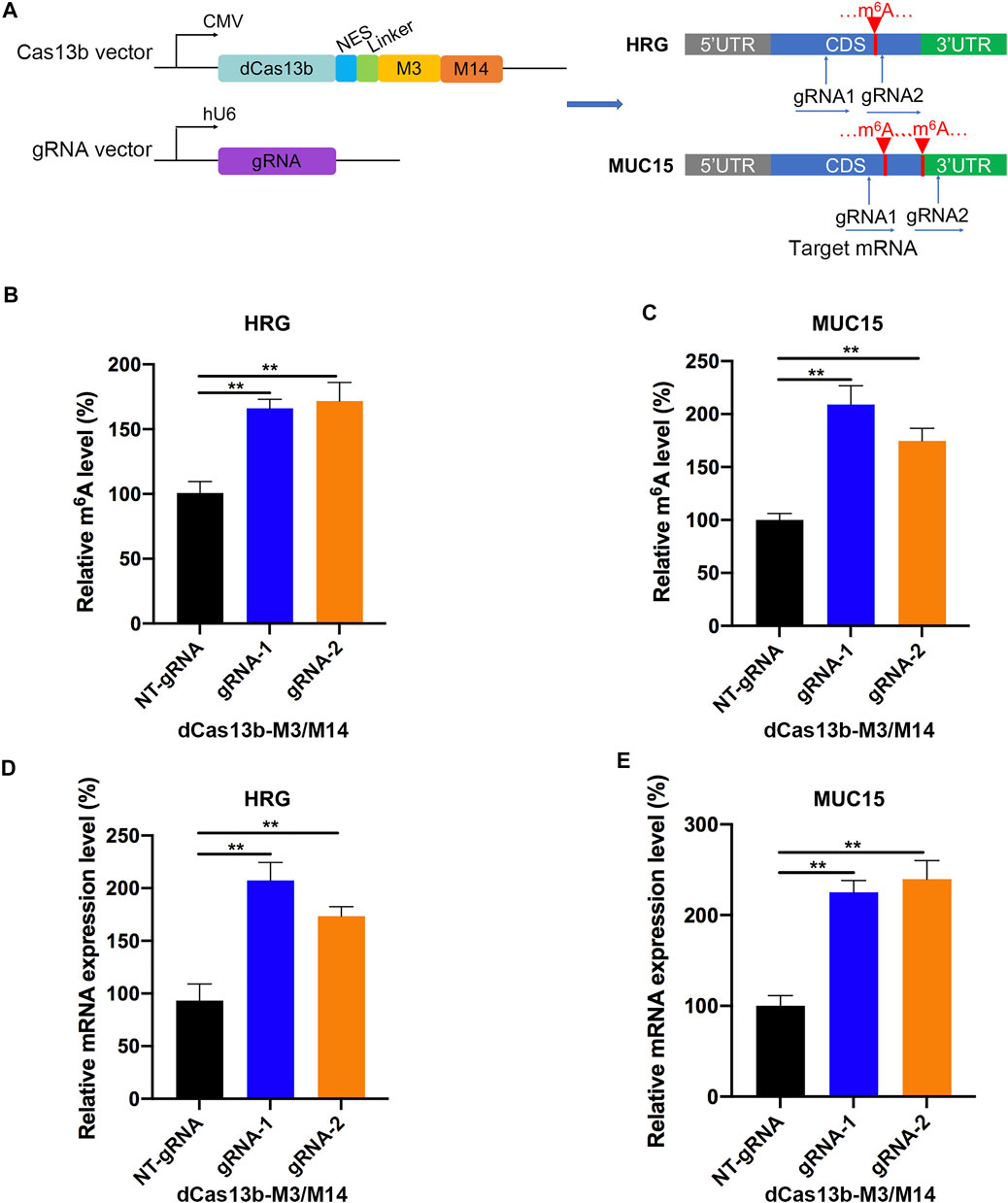
FIGURE 7. dCas13b-M3M14 induces methylation of m6A hypomethylated mRNA in RCC cells. (A) The design of dCas13b-M3M14 fusion protein and sgRNAs targeted with MUC15 and HRG mRNA m6A sites. (B,C) The m6A level of MUC15 (B) and HRG (C) mRNA in RCC cell line 786-O after transfection with dCas13b-ALKBH5 and gRNAs. (D,E) The mRNA levels of MUC15 (D) and HRG (E) in 786-O cell after transfection with dCas13b-ALKBH5 and gRNAs. **p < 0.01.
Discussion
m6A is identified as a dynamic and reversible RNA modification in eukaryotes, due to the “writer” (methyltransferase) and “eraser” (demethylase) proteins. It has been reported that m6A modification could take part in many cellular activities and reaction, including heat shock (Zhou et al., 2015), ultraviolet light (Robinson et al., 2019), hypoxic stress (Fry et al., 2017), and oxidative stress (Anders et al., 2018). Moreover, there were numbers of evidences confirming that m6A modification could promote the development of tumors. In this study, we identified many differentially methylated genes in RCC samples and tumor-adjacent normal tissues based on meRIP-seq technology, analyzed and validated gene expression and cancer-related pathways modulated by abnormal m6A RNA modifications.
We figured out that m6A modification in tumor tissues and normal tissues mainly occurred in the GGACC motif, which was similar to the previous data, and the m6A peaks of transcripts were mainly located at the CDS site. Almost 80% of the methylated genes had one to five m6A sites, and others contained over eight m6A sites in mRNAs. In the current study, differentially methylated mRNAs between tumor and normal tissues were shown to be involved in many important biological pathways. As observed, the gene function analyses of DMMSs showed that the hyper- and hypomethylated genes in the tumor group were significantly enriched in many phylogenetic processes, such as multicellular organismal development and kidney development, and they were also involved in the cancer pathways, such as the transcriptional misregulation in cancer and TGF-β signaling pathway, which supported the importance of m6A in tumorigenesis. It was reported that m6A could play an important role in carcinogenesis and the development of gastric cancer, and the genes with higher m6A levels were mainly enriched in transcriptional misregulation in carcinogenesis pathways, whereas the genes with decreased methylation mainly regulate digestion and absorption of protein (Sang et al., 2020). In addition, the dysregulated expression of m6A was also involved in transcriptional misregulation in cancer and other malignancy-related pathways including primary immunodeficiency, regulation of autophagy, and response to oxidative stress (Zheng et al., 2020). It was also reported that the expression of m6A methyltransferase METTL3 and m6A modification were increased during TGF-β-induced epithelial–mesenchymal transition in A549 and LC2/ad lung cancer cells, and mechanistic investigations revealing that METTL3 could be indispensable for TGF-β-induced epithelial–mesenchymal transition of lung cancer cells through the regulation of JUNB (Wanna-Udom et al., 2020). Moreover, m6A modification was involved in the epithelial–mesenchymal transition of cancer cells by regulating the expression and secretion of TGF-β1 (Li et al., 2020b). These studies revealed that differential m6A modifications were involved in important biological pathways, which were consistent with our study.
Combined analysis of m6A-seq and mRNA-seq data uncovered 369 hyper-up genes and 372 hypo-down genes in tumor tissues compared with adjacent normal tissues, which might play critical roles in the RCC development. Moreover, some of the genes have been reported to facilitate tumor growth and metastasis in different types of cancers. To further investigate mRNA m6A modification of these specific genes, we used CRISPR-dCas13b fusion proteins to regulate the methylation levels of mRNA. For instance, the methylation levels of CDCA2 and CHAT were found to be approximately 20 times higher in the tumor group than that in the control group. Then, we applied dCas13b-ALKBH5 fusion protein to induce the demethylation of CDCA2 and CHAT mRNAs in RCC cell lines, and found that the m6A levels and mRNA expression levels were significantly reduced after transfecting with their targeted gRNAs. Furthermore, another two hypomethylated genes, MUC15 and HRG, exhibited downregulated mRNA levels in the tumor group. Therefore, we performed dCas13b-M3M14 fusion protein combined with two gRNAs to methylate mRNA m6A level of MUC15 and HRG in RCC cell line. The data showed hypermethylated m6A levels and upregulated mRNA levels of MUC15 and HRG, which were in line with our expectations.
However, the study was still partially flawed. We cannot avoid the potential selection bias, since the m6A-RIP-seq and RNA-seq were based on five paired ccRCC tumor tissues and adjacent tissues. Further functional experiments and mechanism explorations of m6A modification in ccRCC should be performed. Despite the defects listed above, the presented findings still provided a link between mRNA m6A modifications and renal tumorigenesis, which could be expected to be a new target for gene targeted therapy of renal cell carcinoma.
Data Availability Statement
The original contributions presented in the study are publicly available. This data can be found here: https://www.ncbi.nlm.nih.gov/bioproject/PRJNA719065.
Ethics Statement
The studies involving human participants were reviewed and approved by the Institutional Ethical Review Board of PKUFH. The patients/participants provided their written informed consent to participate in this study.
Author Contributions
QZ, XY, and LY conceptualized and designed the study. YG collected and analyzed the sequencing data. AL constructed plasmids and performed validation experiments. YG, JL, and XW processed tissue samples. ZZ and QL performed the data interpretation. All authors contributed to the writing of the manuscript and for the final approval of the manuscript.
Funding
This work was supported by the National Natural Science Foundation of China (Grant Nos. 82072826 and 81872088 to QZ; Nos. 62076007 to XY; Nos. 82103239 to YG).
Conflict of Interest
The authors declare that the research was conducted in the absence of any commercial or financial relationships that could be construed as a potential conflict of interest.
Publisher’s Note
All claims expressed in this article are solely those of the authors and do not necessarily represent those of their affiliated organizations, or those of the publisher, the editors, and the reviewers. Any product that may be evaluated in this article, or claim that may be made by its manufacturer, is not guaranteed or endorsed by the publisher.
Supplementary Material
The Supplementary Material for this article can be found online at: https://www.frontiersin.org/articles/10.3389/fgene.2021.795611/full#supplementary-material
References
Aguilo, F., Zhang, F., Sancho, A., Fidalgo, M., Di Cecilia, S., Vashisht, A., et al. (2015). Coordination of m(6)A mRNA Methylation and Gene Transcription by ZFP217 Regulates Pluripotency and Reprogramming. Cell Stem Cell 17, 689–704. doi:10.1016/j.stem.2015.09.005
Anders, M., Chelysheva, I., Goebel, I., Trenkner, T., Zhou, J., Mao, Y., et al. (2018). Dynamic m(6)A Methylation Facilitates mRNA Triaging to Stress Granules. Life Sci. Alliance 1, e201800113. doi:10.26508/lsa.201800113
Batista, P. J., Molinie, B., Wang, J., Qu, K., Zhang, J., Li, L., et al. (2014). m6A RNA Modification Controls Cell Fate Transition in Mammalian Embryonic Stem CellsA RNA Modification Controls Cell Fate Transition in Mammalian Embryonic Stem Cells. Cell Stem Cell 15, 707–719. doi:10.1016/j.stem.2014.09.019
Bertero, A., Brown, S., Madrigal, P., Osnato, A., Ortmann, D., Yiangou, L., et al. (2018). The SMAD2/3 Interactome Reveals that TGFβ Controls m(6)A mRNA Methylation in Pluripotency. Nature 555, 256–259. doi:10.1038/nature25784
Chen, T., Hao, Y.-J., Zhang, Y., Li, M.-M., Wang, M., Han, W., et al. (2015). m(6)A RNA Methylation Is Regulated by MicroRNAs and Promotes Reprogramming to PluripotencyA RNA Methylation Is Regulated by microRNAs and Promotes Reprogramming to Pluripotency. Cell Stem Cell 16, 289–301. doi:10.1016/j.stem.2015.01.016
Csepany, T., Lin, A., Baldick, C. J., and Beemon, K. (1990). Sequence Specificity of mRNA N6-Adenosine Methyltransferase. J. Biol. Chem. 265, 20117–20122. doi:10.1016/s0021-9258(17)30477-5
Dai, D., Wang, H., Zhu, L., Jin, H., and Wang, X. (2018). N6-methyladenosine Links RNA Metabolism to Cancer Progression. Cell Death Dis. 9, 124. doi:10.1038/s41419-017-0129-x
Dominissini, D., Moshitch-Moshkovitz, S., Salmon-Divon, M., Amariglio, N., and Rechavi, G. (2013). Transcriptome-wide Mapping of N(6)-Methyladenosine by m(6)A-Seq Based on Immunocapturing and Massively Parallel Sequencing. Nat. Protoc. 8, 176–189. doi:10.1038/nprot.2012.148
Dominissini, D., Moshitch-Moshkovitz, S., Schwartz, S., Salmon-Divon, M., Ungar, L., Osenberg, S., et al. (2012). Topology of the Human and Mouse m6A RNA Methylomes Revealed by m6A-Seq. Nature 485, 201–206. doi:10.1038/nature11112
Feng, Y., Qian, W., Zhang, Y., Peng, W., Li, J., Gu, Q., et al. (2019). CDCA2 Promotes the Proliferation of Colorectal Cancer Cells by Activating the AKT/CCND1 Pathway In Vitro and In Vivo. BMC Cancer 19, 576. doi:10.1186/s12885-019-5793-z
Fry, N. J., Law, B. A., Ilkayeva, O. R., Holley, C. L., and Mansfield, K. D. (2017). N(6)-methyladenosine Is Required for the Hypoxic Stabilization of Specific mRNAs. RNA 23, 1444–1455. doi:10.1261/rna.061044.117
Fu, Y., Dominissini, D., Rechavi, G., and He, C. (2014). Gene Expression Regulation Mediated through Reversible m(6)A RNA Methylation. Nat. Rev. Genet. 15, 293–306. doi:10.1038/nrg3724
Fukumoto, T., Zhu, H., Nacarelli, T., Karakashev, S., Fatkhutdinov, N., Wu, S., et al. (2019). N(6)-Methylation of Adenosine of FZD10 mRNA Contributes to PARP Inhibitor Resistance. Cancer Res. 79, 2812–2820. doi:10.1158/0008-5472.CAN-18-3592
Fustin, J.-M., Doi, M., Yamaguchi, Y., Hida, H., Nishimura, S., Yoshida, M., et al. (2013). RNA-methylation-dependent RNA Processing Controls the Speed of the Circadian Clock. Cell 155, 793–806. doi:10.1016/j.cell.2013.10.026
He, Y., Hu, H., Wang, Y., Yuan, H., Lu, Z., Wu, P., et al. (2018). ALKBH5 Inhibits Pancreatic Cancer Motility by Decreasing Long Non-coding RNA KCNK15-AS1 Methylation. Cell Physiol. Biochem. 48, 838–846. doi:10.1159/000491915
Heinz, S., Benner, C., Spann, N., Bertolino, E., Lin, Y. C., Laslo, P., et al. (2010). Simple Combinations of Lineage-Determining Transcription Factors Prime Cis-Regulatory Elements Required for Macrophage and B Cell Identities. Mol. Cel. 38, 576–589. doi:10.1016/j.molcel.2010.05.004
Li, J., Chen, F., Peng, Y., Lv, Z., Lin, X., Chen, Z., et al. (2020). N6-Methyladenosine Regulates the Expression and Secretion of TGFβ1 to Affect the Epithelial-Mesenchymal Transition of Cancer Cells. Cells 9, 296. doi:10.3390/cells9020296
Li, J., Chen, Z., Chen, F., Xie, G., Ling, Y., Peng, Y., et al. (2020). Targeted mRNA Demethylation Using an Engineered dCas13b-ALKBH5 Fusion Protein. Nucleic Acids Res. 48, 5684–5694. doi:10.1093/nar/gkaa269
Lin, S., Choe, J., Du, P., Triboulet, R., and Gregory, R. I. (2016). The m(6)A Methyltransferase METTL3 Promotes Translation in Human Cancer Cells. Mol. Cel. 62, 335–345. doi:10.1016/j.molcel.2016.03.021
Meyer, K. D., Saletore, Y., Zumbo, P., Elemento, O., Mason, C. E., and Jaffrey, S. R. (2012). Comprehensive Analysis of mRNA Methylation Reveals Enrichment in 3′ UTRs and Near Stop Codons. Cell 149, 1635–1646. doi:10.1016/j.cell.2012.05.003
Olarerin-George, A. O., and Jaffrey, S. R. (2017). MetaPlotR: a Perl/R Pipeline for Plotting Metagenes of Nucleotide Modifications and Other Transcriptomic Sites. Bioinformatics 33, 1563–1564. doi:10.1093/bioinformatics/btx002
Pahud, G., Bontron, S., and Eder-Colli, L. (2001). Modulation of Choline Acetyltransferase Synthesis by Okadaic Acid, a Phosphatase Inhibitor, and KN-62, a CaM Kinase Inhibitor, in NS-20Y Neuroblastoma. Neurochem. Int. 38, 75–82. doi:10.1016/s0197-0186(00)00064-4
Panneerdoss, S., Eedunuri, V. K., Yadav, P., Timilsina, S., Rajamanickam, S., Viswanadhapalli, S., et al. (2018). Cross-talk Among Writers, Readers, and Erasers of m(6)A Regulates Cancer Growth and Progression. Sci. Adv. 4, eaar8263. doi:10.1126/sciadv.aar8263
Patel, D. N., Figlin, R. A., and Kim, H. L. (2016). Adjuvant Treatment for Renal Cell Carcinoma: Do We Finally Have a Major Breakthrough? Clin. Adv. Hematol. Oncol. 14, 907–914.
Robinson, M., Shah, P., Cui, Y.-H., and He, Y.-Y. (2019). The Role of Dynamic m(6)A RNA Methylation in Photobiology. Photochem. Photobiol. 95, 95–104. doi:10.1111/php.12930
Rolny, C., Mazzone, M., Tugues, S., Laoui, D., Johansson, I., Coulon, C., et al. (2011). HRG Inhibits Tumor Growth and Metastasis by Inducing Macrophage Polarization and Vessel Normalization through Downregulation of PlGF. Cancer Cell 19, 31–44. doi:10.1016/j.ccr.2010.11.009
Rydzanicz, M., Wrzesiński, T., Bluyssen, H. A. R., and Wesoły, J. (2013). Genomics and Epigenomics of clear Cell Renal Cell Carcinoma: Recent Developments and Potential Applications. Cancer Lett. 341, 111–126. doi:10.1016/j.canlet.2013.08.006
Sang, L., Sun, L., Wang, A., Zhang, H., and Yuan, Y. (2020). The N6-Methyladenosine Features of mRNA and Aberrant Expression of m6A Modified Genes in Gastric Cancer and Their Potential Impact on the Risk and Prognosis. Front. Genet. 11, 561566. doi:10.3389/fgene.2020.561566
Schibler, U., Kelley, D. E., and Perry, R. P. (1977). Comparison of Methylated Sequences in Messenger RNA and Heterogeneous Nuclear RNA from Mouse L Cells. J. Mol. Biol. 115, 695–714. doi:10.1016/0022-2836(77)90110-3
Schwartz, S., Mumbach, M. R., Jovanovic, M., Wang, T., Maciag, K., Bushkin, G. G., et al. (2014). Perturbation of m6A Writers Reveals Two Distinct Classes of mRNA Methylation at Internal and 5′ Sites. Cel. Rep. 8, 284–296. doi:10.1016/j.celrep.2014.05.048
Shah, A., Rashid, F., Awan, H. M., Hu, S., Wang, X., Chen, L., et al. (2017). The DEAD-Box RNA Helicase DDX3 Interacts with m(6)A RNA Demethylase ALKBH5. Stem Cell Int. 2017, 8596135. doi:10.1155/2017/8596135
Shen, L., Shao, N.-Y., Liu, X., Maze, I., Feng, J., and Nestler, E. J. (2013). diffReps: Detecting Differential Chromatin Modification Sites from ChIP-Seq Data with Biological Replicates. Plos One 8, e65598. doi:10.1371/journal.pone.0065598
Siegel, R. L., Miller, K. D., and Jemal, A. (2019). Cancer Statistics, 2019. CA A. Cancer J. Clin. 69, 7–34. doi:10.3322/caac.21551
Wang, X., Zhao, B. S., Roundtree, I. A., Lu, Z., Han, D., Ma, H., et al. (2015). N(6)-methyladenosine Modulates Messenger RNA Translation Efficiency. Cell 161, 1388–1399. doi:10.1016/j.cell.2015.05.014
Wanna-Udom, S., Terashima, M., Lyu, H., Ishimura, A., Takino, T., Sakari, M., et al. (2020). The m6A Methyltransferase METTL3 Contributes to Transforming Growth Factor-Beta-Induced Epithelial-Mesenchymal Transition of Lung Cancer Cells through the Regulation of JUNB. Biochem. Biophysical Res. Commun. 524, 150–155. doi:10.1016/j.bbrc.2020.01.042
Wei, C. M., Gershowitz, A., and Moss, B. (1976). 5'-Terminal and Internal Methylated Nucleotide Sequences in HeLa Cell mRNA. Biochemistry 15, 397–401. doi:10.1021/bi00647a024
Wilson, C., Chen, P. J., Miao, Z., and Liu, D. R. (2020). Programmable M(6)A Modification of Cellular RNAs with a Cas13-Directed Methyltransferase. Nat. Biotechnol. 38, 1431. doi:10.1038/s41587-020-0572-6
Yokomori, K., Tsuchida, Y., and Saito, S. (1983). Tyrosine Hydroxylase and Choline Acetyltransferase Activity in Human Neuroblastoma Correlations with Clinical Features. Cancer 52, 263–272. doi:10.1002/1097-0142(19830715)52:2<263:aid-cncr2820520213>3.0.co;2-o
Zhang, C., Samanta, D., Lu, H., Bullen, J. W., Zhang, H., Chen, I., et al. (2016). Hypoxia Induces the Breast Cancer Stem Cell Phenotype by HIF-dependent and ALKBH5-Mediated m(6)A-Demethylation of NANOG mRNA. Proc. Natl. Acad. Sci. USA 113, E2047–E2056. doi:10.1073/pnas.1602883113
Zhang, J., Guo, S., Piao, H.-y., Wang, Y., Wu, Y., Meng, X.-y., et al. (2019). ALKBH5 Promotes Invasion and Metastasis of Gastric Cancer by Decreasing Methylation of the lncRNA NEAT1. J. Physiol. Biochem. 75, 379–389. doi:10.1007/s13105-019-00690-8
Zhang, S., Zhang, W., Xiao, Y., Qin, T., Yue, Y., Qian, W., et al. (2020). Targeting MUC15 Protein in Cancer: Molecular Mechanisms and Therapeutic Perspectives. Ccdt 20, 647–653. doi:10.2174/1568009620666200601140639
Zhang, Y., Liu, T., Meyer, C. A., Eeckhoute, J., Johnson, D. S., Bernstein, B. E., et al. (2008). Model-based Analysis of ChIP-Seq (MACS). Genome Biol. 9, R137. doi:10.1186/gb-2008-9-9-r137
Zheng, Z., Mao, S., Guo, Y., Zhang, W., Liu, J., Li, C., et al. (2020). N6-methyladenosine RNA M-ethylation R-egulators P-articipate in M-alignant P-rogression and H-ave P-rognostic V-alue in clear C-ell R-enal C-ell C-arcinoma. Oncol. Rep. 43, 1591–1605. doi:10.3892/or.2020.7524
Zhou, J., Wan, J., Gao, X., Zhang, X., Jaffrey, S. R., and Qian, S.-B. (2015). Dynamic m(6)A mRNA Methylation Directs Translational Control of Heat Shock Response. Nature 526, 591–594. doi:10.1038/nature15377
Zhu, H., Gan, X., Jiang, X., Diao, S., Wu, H., and Hu, J. (2019). ALKBH5 Inhibited Autophagy of Epithelial Ovarian Cancer through miR-7 and BCL-2. J. Exp. Clin. Cancer Res. 38, 163. doi:10.1186/s13046-019-1159-2
Keywords: m6A, gene expression, Programmable Modification, CRISPR-Cas13b, Renal Cell Carcinoma
Citation: Gan Y, Li A, Liu J, Wang X, Zhang Z, Li Q, Ye X, Yao L and Zhang Q (2022) m6A-mRNA Methylation Regulates Gene Expression and Programmable m6A Modification of Cellular RNAs With CRISPR-Cas13b in Renal Cell Carcinoma. Front. Genet. 12:795611. doi: 10.3389/fgene.2021.795611
Received: 15 October 2021; Accepted: 09 December 2021;
Published: 21 January 2022.
Edited by:
Dapeng Xiong, Cornell University, United StatesReviewed by:
Dandan Yang, Brigham and Women’s Hospital and Harvard Medical School, United StatesHeqiao Zhang, ShanghaiTech University, China
Copyright © 2022 Gan, Li, Liu, Wang, Zhang, Li, Ye, Yao and Zhang. This is an open-access article distributed under the terms of the Creative Commons Attribution License (CC BY). The use, distribution or reproduction in other forums is permitted, provided the original author(s) and the copyright owner(s) are credited and that the original publication in this journal is cited, in accordance with accepted academic practice. No use, distribution or reproduction is permitted which does not comply with these terms.
*Correspondence: Lin Yao, poparies@163.com; Xiongjun Ye, yexiongjun@bjmu.edu.cn; Qian Zhang, zhangqianbjmu@126.com
†These authors have contributed equally to this work