- 1Key Laboratory of Adaptation and Evolution of Plateau Biota, Northwest Institute of Plateau Biology, Chinese Academy of Sciences, Xining, China
- 2University of Chinese Academy of Sciences, Beijing, China
- 3Qinghai Province Key Laboratory of Animal Ecological Genomics, Xining, China
According to life history theory, animals living in extreme environments have evolved specific behavioral and physiological strategies for survival. However, the genetic mechanisms underpinning these strategies are unclear. As the highest geographical unit on Earth, the Qinghai–Tibet Plateau is characterized by an extreme environment and climate. During long-term evolutionary processes, animals that inhabit the plateau have evolved specialized morphological and physiological traits. The plateau pika (Ochotona curzoniae), one of the native small mammals that evolved on the Qinghai–Tibet Plateau, has adapted well to this cold and hypoxic environment. To explore the genetic mechanisms underlying the physiological adaptations of plateau pika to extremely cold ambient temperatures, we measured the differences in resting metabolic rate (RMR) and metabolism-related gene expression in individuals inhabiting three distinct altitudes (i.e., 3,321, 3,663, and 4,194 m). Results showed that the body mass and RMR of plateau pika at high- and medium-altitudes were significantly higher than those at the low-altitude. The expression levels of peroxisome proliferator-activated receptor α (pparα), peroxisome proliferator-activated receptor-γ coactivator-1α (pgc-1α), and the PR domain-containing 16 (PRDM16) in white (WAT) and brown (BAT) adipose tissues of plateau pika from high- and medium-altitudes were significantly higher than in pika from the low-altitude region. The enhanced expression levels of pgc-1α and pparα genes in the WAT of pika at high-altitude showed that WAT underwent “browning” and increased thermogenic properties. An increase in the expression of uncoupling protein 1 (UCP1) in the BAT of pika at high altitude indicated that BAT increased their thermogenic properties. The gene expression levels of pparα and pgc-1α in skeletal muscles were significantly higher in high-altitude pika. Simultaneously, the expression of the sarcolipin (SLN) gene in skeletal muscles significantly increased in high-altitude pika. Our results suggest that plateau pika adapted to an extremely cold environment via browning WAT, thereby activating BAT and enhancing SLN expression to increase non-shivering thermogenesis. This study demonstrates that plateau pika can increase thermogenic gene expression and energy metabolism to adapt to the extreme environments on the plateau.
Introduction
Animals living in different habitats are affected by various ecological factors such as photoperiod, food quantity or quality, and temperature (Van Beest et al., 2012; Hanya and Chapman, 2013; Olanrewaju et al., 2013). To adapt to a changing climate, animals have evolved specialized morphological, behavioral, and physiological traits (Zhu et al., 2017a; Mannuthy, 2017; Fox et al., 2019). For example, an experimental analysis of Trochilidae and Zonotrichia capensis showed a correlation between Hb-O2 affinity and native elevation (Projecto-Garcia et al., 2013; Cheviron et al., 2014). Tamiasciurus hudsonicus and Lepus americanus respond to environmental changes by protecting a high and stable body temperature with changes in body temperature and heart rate while reducing behavioral changes (Menzies, 2021). Moreover, as ambient temperature decreases, animals may adjust their behavior and/or physiology to reduce their energy expenditure (Humphries et al., 2005; Zub et al., 2009). Animals such as Mustela nivalis, Spermophilus parryii, and Rhabdomys pumilio can huddle together or stay in the nest to conserve energy and maintain body temperature (Geiser, 2004; Scantlebury et al., 2006; Sukhchuluun et al., 2018). Many studies have shown phenotypic and physiological adaptations to the environment, and that species-specific adaptations to extreme environments are reflected at the gene transcription level. Studies on Anolis carolinensis, Rhinopithecus bieti, Thermophis baileyi, and Sus scrofa have uncovered the gene-expression mechanisms underlying their behavioral and physiological adaptations (Li et al., 2013; Yu et al., 2016; Li T. et al., 2018; Kabelik et al., 2021).
Energy metabolism plays an important role in physiological adaptation, which influences animal distribution, abundance, reproductive success, and fitness (Yaskin, 2011; Healy et al., 2013; Tickle et al., 2018). Energy metabolism is affected by environmental and physiological factors, including body mass, food quality/quantity, and temperature, which substantially affect an animal’s heat production and thermoregulation (McNab, 2009; Tattersall et al., 2012). Elevated thermogenic capacity is crucial to an animal’s survival in a cold environment (Zhang et al., 2017). Thermogenic capacity can be measured as maximum metabolic rate, which is comprised of resting metabolic rate (RMR), shivering thermogenesis (ST), and non-shivering thermogenesis (NST) (Nespolo et al., 2001; Chi and Wang, 2011; Mineo et al., 2012). Compared with those species inhabiting cold environments, animals inhabiting warm environments, i.e., Meriones unguiculatus and Diplolaemus leopardinus, have a lower RMR (Ding et al., 2018; Vicenzi et al., 2021). Similarly, the RMRs of Tupaia belangeri and Chaetops frenatus in winter are usually higher than in summer (Zhu et al., 2012; Oswald et al., 2018). Furthermore, animals can adapt to the ambient temperature by changing their thermogenic characteristics, such as increasing protein content, cytochrome c oxidase activity, and leptin expression (Meyer et al., 2010; Wang et al., 2019).
As the highest plateau on Earth, the Qinghai–Tibet Plateau has an average altitude of more than 4,000 m. Its unique topography has formed extreme environmental and climatic characteristics (Sun et al., 2014). Animals that inhabit the plateau at high altitudes face the challenging environment of hypoxia and low ambient temperatures (Wang et al., 2011). The Qinghai–Tibet Plateau is one of the most sensitive regions to global climate change (Liu and Chen, 2000). A progressive reduction in temperature occurs with the ascent to high elevation, and high-altitude environments mean considerable physiological challenges to animals (Storz and Scott, 2019). Animals may adjust their physiological characteristics by spending energy to generate heat to survive in high-altitude environments. One important question is how animals regulate their metabolism and maintain their effective energy in extreme environments (O’Brien et al., 2020). Studies of passerine birds, lizards, and Parnassius butterflies inhabiting the three high-altitude regions of the Qinghai–Tibet Plateau found that their gene expression correlates with altitude, suggesting that high-altitude environments may drive similar expression patterns in high-altitude species (Yang et al., 2015; Hao et al., 2019; Su et al., 2020).
Adipose tissue, which can be divided into white adipose tissue (WAT) and brown adipose tissue (BAT) in mammals, plays an extremely important role in the regulation of energy homeostasis in animals (Harms and Seale, 2013; Elsen et al., 2014). The PR domain of 16 (PRDM16) and peroxisome proliferator-activated receptor γ coactivator-1α (pgc-1α) were key transcriptional regulators in mice and induced classic brown fat accumulation in hypothermia induction (Seale et al., 2011). Peroxisome proliferation receptor-α (pparα) mediated lipid thermogenesis by sensing pgc-1α and PRDM16 expression as a key component of brown fat thermogenesis (Hondares et al., 2011). Studies have shown that the deletion of the SLN gene in skeletal muscle causes mice to fail to maintain body temperature during exposure to acute cold, demonstrating that sarcolipin (SLN) is an important player in adaptive thermogenesis (Bal et al., 2012). There was also an increase in the transcriptional regulators of mitochondrial biogenesis, such as pparα and pgc-1α (Handschin et al., 2003; Ryder et al., 2003; Schaeffer et al., 2004). The metabolic function of the liver is controlled by insulin and other metabolic hormones. Studies have shown that under food serious shortage, cAMP-response element binding protein (CREB) and pgc-1α are key transcriptional coactivators in hepatic gluconeogenesis in two experimental mouse models; they play a key role in maintaining long-term gluconeogenesis (Herzig et al., 2001; Oh et al., 2013).
The plateau pika (Ochotona curzoniae) is a keystone species on the Qinghai–Tibet plateau (Yu et al., 2012) and plays an important role in maintaining the biodiversity and stability of the alpine meadow ecosystem (Smith and Foggin, 1999; Wilson and Smith, 2015). It inhabits the alpine regions at an altitude of 3,100–5,300 m above sea level and is well adapted to extreme hypoxia, cold, and food deprived environments (Cao et al., 2017). In this scenario, plateau animals including plateau pika face severe energetic challenges to maintain their core body temperature (Van Sant and Hammond, 2008; Zhang et al., 2012; Speakman et al., 2021). Previous studies have found that at different altitudes, the life history strategies and personalities of plateau pika varied significantly (Liu et al., 2012; Qu et al., 2013; Qu et al., 2019; Tan et al., 2020), accompanied by differences in fat accumulation and metabolic rate (Yang et al., 2006). As ambient temperatures decrease, subcutaneous WAT “browned”, and adipose tissue heat production increased (Bai et al., 2015; Li et al., 2019). However, studies on the expression of thermogenic genes in adipose tissue and other thermogenic tissues of plateau pika at different altitudes are limited. In the current study, we live-trapped plateau pika at different altitudes. We measured their metabolic rate and transcriptome expression levels in adipose tissue, liver, and skeletal muscle in order to profile gene expression patterns and investigate the role of transcriptional regulation in tissue-level metabolic adaptation to high altitudes. We aimed to test the following hypotheses: 1) the RMR of the plateau pika increases with rising altitude, and 2) metabolism-related gene expression synchronously increases with rising altitude, adapting to the extreme environments of the Qinghai–Tibet Plateau.
Materials and Methods
Animals and Sample Collection
Plateau pikas inhabiting high-, middle-, and low-altitude regions were live trapped from Maduo, (4,194 m above sea level, n = 24), Guide, (3,663 m, n = 24), and Guinan (3,321 m, n = 24), respectively, in Qinghai Province, in December 2020. Maduo has an annual average temperature of −4°C and an average monthly temperature below −3.0°C, classifying it as an alpine steppe climate. The annual average temperature of Guide is −3.7°C; a plateau continental climate. Guinan does not experience a severely cold winter or an intensely hot summer, and the annual average temperature is 2.3°C; as such, it is also considered a plateau continental climate.
Ten pikas from each altitudinal region were immediately anesthetized and dissected after capture (five females and five males at each altitude). The adipose tissue, liver, and muscle tissue were immediately preserved in liquid nitrogen and stored at −80°C until further RNA extraction and analyses. A further sample of plateau pikas (n = 14) from each altitude were live-transported to the animal laboratory in Xining (2,261 m above sea level, outdoor temperature −2°C, indoor temperature 20°C). They were kept in 545 × 395 × 200 mm polypropylene material cages separately under 12 L: 12 D lighting conditions, and provided with artificial food (Tianjin Tongyu Feed Sales Co. Ltd.) and ad libitum water. Metabolic experiments were conducted within 24 h.
Metabolic Trials and Non-shivering Thermogenesis (NST)
The RMR of plateau pikas were expressed as oxygen consumption per hour per unit body mass [mL O2/(g·h)] and measured using an 8-channel FMS (Sable Systems International, Henderson, NV, United States) portable respiratory metabolism system. A biochemical incubator was used to control the chamber temperature, and the experimental temperature was set at 27.5°C (which is within the pika thermal neutral zone) with a standard error of 0.5°C. Metabolic measurements were conducted after the pikas had acclimatized in the chamber for 0.5 h and were resting. The RMRs of seven pikas were measured simultaneously, and a blank tube was used as the baseline for carbon dioxide, oxygen, water vapor, and temperature (Tan et al., 2020; Yu et al., 2021). Four rounds of metabolism were measured in 2 h, with each round lasting 30 min. When the chamber temperature was 27.5°C, the average of the lowest metabolic rates of each individual over at least 10 min was selected as the RMR (Boratyński et al., 2017). RMR is the minimum energy requirement for animals to maintain normal physiological activities within a thermally neutral environmental temperature, while at rest (Arnold et al., 2021). Before the experiment, the pikas were fasted for 2–3 h, and their body mass and temperature were measured using an electronic balance and rectal thermometer, respectively. A digital thermometer probe was inserted gently about 2 cm into the rectum; the measurement time did not exceed 30 s.
Noradrenaline (NE) induction is widely used to determine NST because induced heat generation and cold induction are equivalent and the mechanism is the same. The dose was 0.7 mg/kg in reference to the seasonal variation of NST in plateau pika measured by Wang (Wang and wnag, 1990). The 10 plateau pikas at each altitude were brought back to the laboratory and allowed to adapt for 24 h. The pikas were raised in a single cage under 12 L:12 D illumination in the laboratory, fed with sufficient amounts of rabbit pellet feed (Jiangsu Syu Pharmaceutical Biological Engineering Co., Ltd.), provided water ad libitum, and adapted for 2–3 h before the experiment. NST was measured using an 8-channel FMS respiratory metabolic measurement system. NE was injected subcutaneously into the back with a dose equivalent to pika body weight (0.4 mg/kg). The pikas were immediately put back into the respiratory chamber for 30 min. NE was injected with norepinephrine (1 ml containing 2 mg), having been diluted to 0.4 mg/ml by adding normal saline. The NE was produced by Shanghai Wellhope Pharmaceutical Co., Ltd. In general, the peak in metabolic response occurs 10–45 min after the NE injection. A scatterplot of oxygen consumption against determination time was generated, and the average value of 10 consecutive and stable maximum values was taken as the NST value.
Reverse Transcription (RT) and Quantitative Real-Time PCR (qPCR)
qRT-PCR was used to determine the expression of pparα, pgc-1α, CREB, PRDM16, SLN and uncoupling protein 1 (UCP1). The species-specific primer sets and 18s-actin of the genes in plateau pika were designed in accordance with the reference gene sequences in the NCBI (national center for biotechnology information) website (http://www.ncbi.nlm.nih.gov/) for reference North American pika gene sequences. Primer6.0 software was used to design primers.
Total RNA was extracted from tissues using the Uniq-10 Column Trizol Total RNA Extraction Kit (B511321) in accordance with the kit instructions. The primer was set for 18s and six transforming genes were designed for quantitative real-time PCR. Quantitative real-time PCR was completed using the 2SG Fast qPCR Master Mix (B639271, BBI, Roche) in the LightCycler480 II type fluorescent quantitative PCR instrument (Roche, Rotkreuz, Switzerland). qRT-PCR was carried out in a 10-μL reaction system, which was composed of 5 μL of 2 SybrGreen qPCR Master Mix, 1 μL of cDNA and 0.2 μL of each primer (10 μM/L), and 3.6 μL of ddH2O. All PCR reactions were repeated. The thermal cycling conditions were as follows: 95°C for 3 min, 45 cycles at 95°C for 5 s, and 60°C for 30 s. The melting curve analysis revealed genes and 18s-amplified single-PCR and final products. We constructed a standard curve for each gene by diluting the cDNA sequence fivefold. The standard curve analysis of target genes and 18 s showed that they had similar amplification efficiency, which ensured the effectiveness of the comparative quantification method. Gene expression was calculated using the 2−ΔΔCt method and expressed as relative quantities. The nucleotide sequences of primers used for qPCR are shown in Supplementary Table S1.
Statistical Analysis
All data analyses were conducted using R 3.4.3 software. Body mass, RMR, and metabolism-related gene expression levels were analyzed using two-way analysis of variance. Prior to all statistical analyses, data were examined for assumptions of normality and homogeneity of variance by using Shapiro–Wilk and Levene tests, respectively. Differences among groups were detected using Duncan’s multiple range test. Results were presented as mean ± 0.5 standard error (SE); n is the sample size. p < 0.05 was considered statistically significant.
Results
RMR and Body Mass
The body masses of plateau pikas from the high- and middle-altitude regions were significantly higher than those from low-altitude regions (F = 7.16, p < 0.05; Figure 1A). The mass-corrected RMRs of plateau pikas were 1.55 ± 0.18, 1.52 ± 0.33, and 1.39 ± 0.17 ml/(g·h) in high-, middle-, and low-altitude regions, respectively. The RMRs of plateau pikas from high- and middle-altitude regions were significantly higher than those from the low-altitude region (F = 3.49, p < 0.05; Figure 1B).
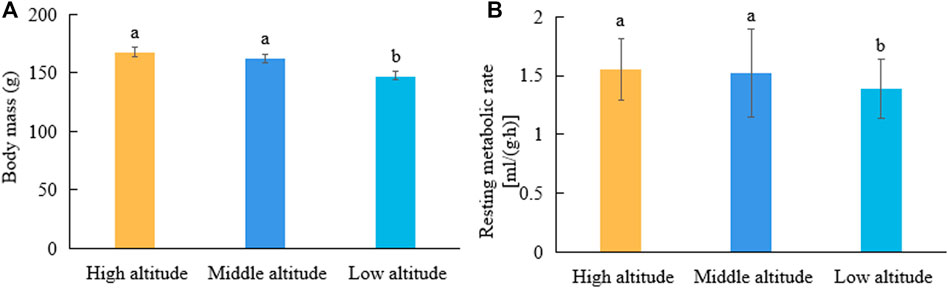
FIGURE 1. Comparisons of body mass and RMR of plateau pikas from regions with different altitudes. Different lowercase letters indicate significant differences among elevations (p < 0.05). Same lowercase letters indicate have not significant difference among elevations (ns p > 0.05).
Gene Expression in Adipose Tissue
To explore molecular signatures of the thermogenesis of WAT and BAT, we performed profiling of gene expression in the two fat tissues from the three elevation groups. No significant difference was observed in gene expression between the sexes (F = 0.36, p > 0.05), whereas significant differences were detected between the three altitudes (F = 15.56, p < 0.05). UCP1, PRDM16, and PGC-1a are the key transcriptional regulators associated with browning and BAT, they were the transcriptional co-activator that is involved in browning and mitochondrial biogenesis. The expression levels of the UCP1 protein in BAT from high- and medium-altitude regions were higher than those from the low-altitude region, which indicates that BAT is specialized for NST and energy dissipation through the action of UCP1. Evidence for an increase in NST is provided in the supplementary material (Supplementary Figure S1). Similar results were obtained for pgc-1α (Figures 2A,C). The expression levels of pgc-1α, pparα, and PRDM16 genes in the WAT and BAT of plateau pikas from high- and medium-altitude regions were significantly higher than those from the low-altitude region (p < 0.05; Figures 2A,C).
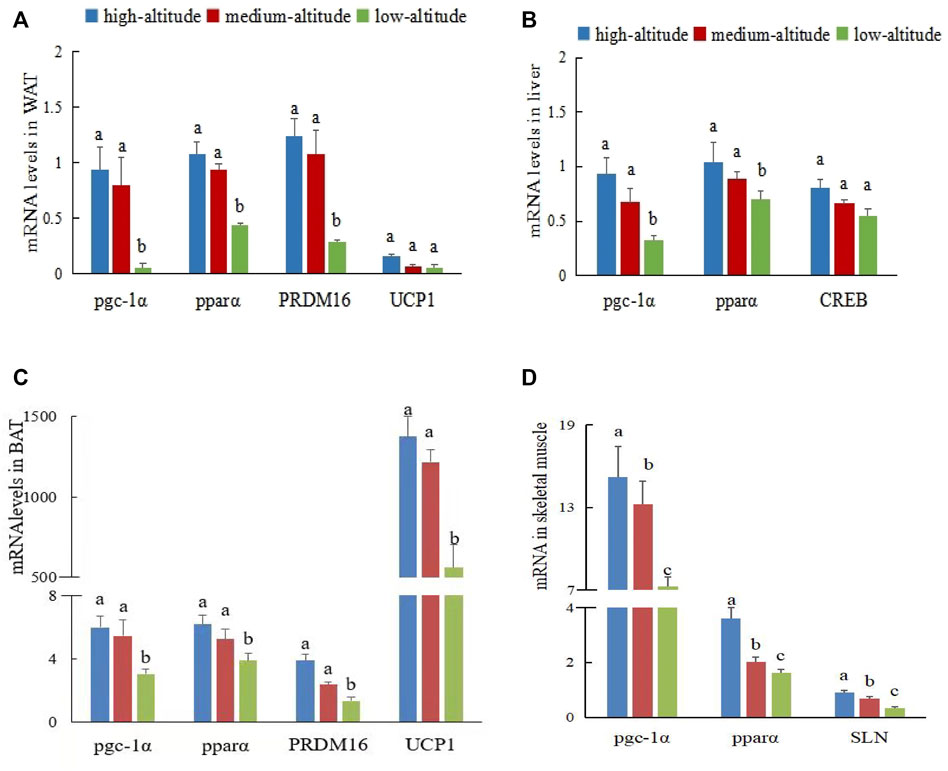
FIGURE 2. Gene expression of different tissues in plateau pika from regions with different altitudes. Note: (A) Expression of pgc-1α, pparα,PRDM16 and UCP1 genes in WAT. (B) Expression of pgc-1α, pparα and CREB genes in liver. (C) Expression of pgc-1α, pparα, PRDM16, and UCP1 genes in BAT. (D) Expression of pgc-1α, pparα, and SLN genes in skeletal muscle. Different lowercase letters indicate significant differences among elevations (p < 0.05). Same lowercase letters indicate have not significant difference among elevations (ns p > 0.05).
Gene Expression in Liver
Liver is a metabolic organ, and its metabolic function is controlled by metabolic hormones such as insulin. To explore the molecular characteristics of hepatic gluconeogenesis in liver, we detected the differential expression of energy metabolism molecules in three plateau pika samples at different altitudes. No significant difference was observed between the sexes (F = 1.587, p > 0.05), whereas a significant difference in gene expression levels was found between altitudes (F = 13.59, p < 0.05). The main transcription factors inducing gluconeogenesis include CREB, FoxO1, and several nuclear receptors. PGC-1α is a key transcriptional coactivator for FoxO1 in hepatic gluconeogenesis, which plays a key role in maintaining long-term gluconeogenesis under conditions of scarce food resource. The expression levels of pgc-1α and pparα genes in the liver of plateau pikas from the high-and medium-altitude regions were significantly higher than those from the low-altitude region, but the expression levels of the CREB gene were not significantly different between the three regions (p > 0.05, Figure 2B).
Gene Expression in the Skeletal Muscle
As the largest organ, skeletal muscle is also a major contributor to metabolic rate and can significantly affect metabolism and body weight by increasing muscle energy expenditure through non-shivering thermogenesis. We studied the differential expression of thermogenic molecules in the skeletal muscle of pikas at the three altitudes. No significant difference was observed in gene expression between the sexes (F = 0.78, p > 0.05), but gene expressions in the three regions were significantly different (F = 11.56, p < 0.05). The expression levels of pgc-1α, pparα, and SLN genes in the muscle of plateau pikas from the high-altitude region were significantly higher than those from the medium-altitude region (F = 15.49, p < 0.05). The expression levels of pgc-1α, pparα, and SLN genes of plateau pikas from the medium-altitude region were significantly higher than those from the low-altitude region (F = 14.91, p < 0.05; Figure 2D).
Discussion
Adaptive evolution is a hot topic in evolutionary ecology. Elucidating the selection pressures that drive the evolution of metabolic rate is fundamental to understanding the evolution of the morphology, physiology, behavior, and life histories of animals (McKechnie and Swanson, 2010). In the present study, the metabolic rates of plateau pikas from the high- and middle-altitude regions were significantly higher than those from the low-altitude region. The expression levels of pparα, PRDM16, and UCP1 in the WAT and BAT of plateau pikas from high and medium altitudes are significantly higher than in those from low altitude. Simultaneously, the expression levels of SLN genes in skeletal muscle and liver significantly increase in high-altitude pikas. These data support the contention that through long-term adaptation, the plateau pika has adapted to high altitude and evolved efficient approaches to deal with the extreme cold and harsh environments on the Qinghai–Tibet Plateau.
RMR and Body Mass
Selective pressures affecting metabolism are complex and can influence metabolic rate through multiple pathways (Zheng et al., 2014b) such as body size, climate, activity, and habits (Killen et al., 2016). Body mass is the most direct indicator of animal energy reserves (Swanson et al., 2017). For example, statistical tests found that the metabolic rate of 533 species of birds was positively correlated with body mass (McNab, 2009). In the present study, the body masses of plateau pikas from high- and middle-altitudes were significantly higher than those from the low altitude. Significant correlations between body mass and metabolic rate were found in the hamster subfamily (Bozinovic, 1992). According to Bergmann’s law, the increase in body mass decreases the surface-to-volume ratio, thereby reducing heat loss and living costs (Zheng et al., 2014a). Many variables associated with physiology are correlated with latitude, indicating that climate is an important factor for the evolution of life-history traits (Tószögyová, 2020). Meta-analyses about the metabolic rate of 69 species of tropical birds and 59 species of temperate birds found that tropical migrants in temperate habitats have lower metabolic rates than do temperate residents (Wiersma et al., 2007). Compared with Cricetulus barabensis kept at room temperature, the energy intake of individuals adapted to a low temperature was higher while the energy intake of individuals adapted to a high temperature was lower (Zhou et al., 2015). The RMRs of plateau pikas in the current study inhabiting high altitude are significantly higher than those of pikas inhabiting low altitude. High metabolic rates may be caused by the biochemical activities of several tissues including the liver, BAT, and skeletal muscle, which all have high mitochondrial oxidative phosphorylation rates (Burton et al., 2011; Selman et al., 2013). Increased metabolism plays an important role in thermal regulation in animals living in an extremely cold environment (Gordon, 2012; McKie et al., 2019).
Gene Expression in WAT and BAT
Cold and hypoxia are defining features of the Qinghai–Tibet Plateau environment, and plateau pika have developed tolerances to this harsh environment (Xie et al., 2014; Wei et al., 2016). Consistent with previous studies that demonstrated that pika have tolerance to hypoxia and low-temperatures (Yang et al., 2006; Zhu et al., 2018), a previous study showed that plateau pika can effectively endure extremely cold environments (Li et al., 2001). Earlier studies found that plateau pika have high NST to cope with the cold environment on the plateau in comparison to Ochotonidae from other regions (Wang et al., 2006; Luo et al., 2008). NST is related to tissue heat production, especially adipose tissue, which is important in regulating body temperature and energy homeostasis in cold environments (Zhu et al., 2017b). As two major types of adipose tissue, WAT is involved in energy storage and BAT is involved in energy expenditure and thermogenesis. BAT and WAT can be conditionally interconverted in response to neuroendocrinal factors, β-3-adrenergic stimulation, and cold stress exposure. WAT responds quickly to environmental changes under cold conditions and takes on the characteristics of BAT. When animals inhabit a cold environment, WAT may possibly transform into beige and brown adipocytes to increase NST in order to adapt to cold conditions (Nedergaard et al., 2007) (Figure 3A).
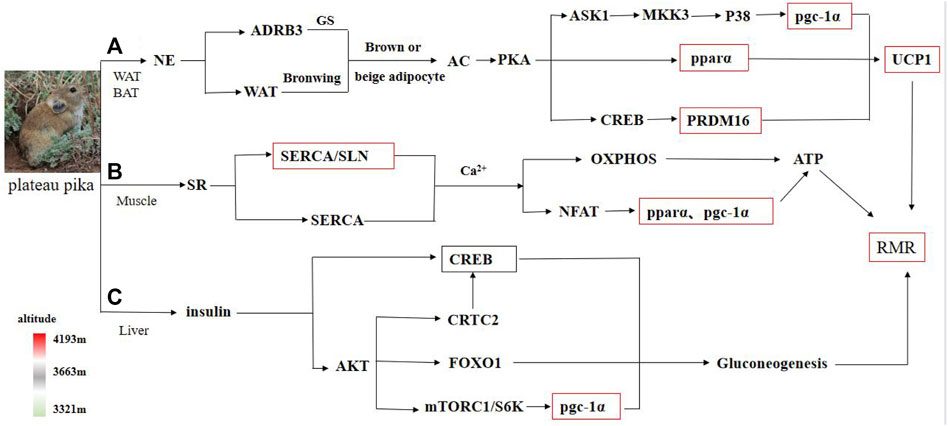
FIGURE 3. Schematic of energy metabolism in various tissues of plateau pika. Note: The red box represents significant change in gene expression, whereas the green box represents no significant change in gene expression among regions with different altitudes. (A–C) represent the metabolic process of adipose tissue, muscle tissue and liver tissue, respectively.
The intermittent cold exposure experiment demonstrated that plateau pika kept in warm temperatures have little classical brown fat, but the “browning” of WATs is detected rapidly upon cold exposure. The expression of several brown fat differentiation markers, including UCP1, increases simultaneously. The increase in UCP1 expression enhances adaptive thermogenesis (Bai et al., 2015). The study about Tupaia belangeri shows that RMR and the expression levels of pparα, pgc-1α, and PRDM16 increase significantly under cold acclimation, suggesting that browning may appear in WAT (Hou et al., 2020). A genomic, proteomic, and morphological study of energy metabolism in highland pikas and Tupaia belangeri in summer and winter studies revealed that subcutaneous WAT in winter show BAT morphological and histological features (Li et al., 2019). Furthermore, BAT-specific genes, such as UCP1, Cox4, and pgc-1α, are highly expressed in WAT in winter (Li J. et al., 2018). These results suggest that plateau pika adapt to a cold environment by browning scarfskin WAT and adding BAT to increase heat production. Our results suggest that a high expression of pgc-1α may be involved in the critical adaptation mechanisms in pika to cope with the harsh environment of the Qinghai–Tibet plateau. pgc-1α is essential for brown fat thermogenesis and complementary mitochondrial biogenesis, and is also involved in the browning of WAT (Finck and Kelly, 2006). High mRNA expression levels of pgc-1α are observed in high-altitude groups, suggesting high levels of thermogenesis within the tissues. Overall, our study indicated that plateau pikas inhabiting the high altitudes of the Qinghai–Tibet Plateau can regulate their relative gene expression in adipose tissue to, in turn, regulate metabolic level and thermogenic-related physiological performance.
Gene Expression in Skeletal Muscle
The skeletal muscle is a major determinant of basal metabolic rate (Maurya et al., 2018). Skeletal muscle also plays a central role in temperature homeostasis and can be recruited to produce heat through NST (Nowack et al., 2017). As an uncoupler of the sarcoplasmic reticulum calcium ATPase (SERCA) pump, SLN can enhance futile cycling and increase ATP hydrolysis, thereby creating chronic energy demand (Sahoo et al., 2013). The SLN/SERCA interaction plays a dual role: it creates energy demands in muscle and activates Ca2+-dependent signaling, such as pgc-1α and pparα, to increase ATP production through increased mitochondrial biogenesis (Shaikh et al., 2016) (Figure 3B). In genetically engineered SLN mouse models, SLN knockout mice have reduced cold adaptive thermogenesis (Bal et al., 2018). The loss of SLN predisposes mice to diet-induced obesity, indicating that SLN may regulate their energy balance (Bal et al., 2012). Compared to SLN gene-lacking mice, the overexpression of the SLN gene leads to a loss of body mass and increases in the depletion of fat deposits (Rotter et al., 2018). The Rotter et al. (2018) study was conducted at thermoneutrality, which can minimize the contribution to metabolic rate of thermogenic mechanisms. Thus, a high energy consumption may be due to SLN-mediated energy expenditure (Maurya et al., 2015). In the present study, the SLN gene expression level of plateau pika from the high-altitude region was significantly higher than that of plateau pika from the middle-altitude region, which in turn was significantly higher than the SLN gene expression of pika from the low-altitude region. This result suggests that the SLN gene is important in regulating the heat production of plateau pikas at different altitudes.
Gene Expression of Liver
The liver is an essential metabolic organ, and its metabolic function is regulated by insulin and other metabolic hormones. Numerous transcription factors and coactivators, including CREB, pparg, and pgc-1 regulate the expression of enzymes that catalyze key steps of metabolic pathways, thus managing the energy metabolism of liver (Rui, 2014). When food is scarce, the hepatic gluconeogenesis pathway is enhanced by decreasing the concentration of insulin and increasing the concentration of insulin counter-regulatory hormones, such as glucagon (Han et al., 2016). pgc-1α, CREB/CRTC2, and FoxO1 genes are critical in coordinating the fasting-mediated activation of gluconeogenesis in the liver (Oh et al., 2013) (Figure 3C). In our study, the pgc-1α and pparα in the liver of plateau pika from the high-altitude region were significantly higher than those of pika from the low-altitude region, whereas no significant difference in the CREB gene was detected among the three regions, suggesting that ATP depletion is due to activity-induced energy demands and the storage of fatty acids, cholesterol, glycogen, and proteins, especially in liver (Ke et al., 2018).
In conclusion, the RMR, and the expression of skeletal muscle thermogenic genes and lipid transcription factor genes in plateau pika increases with rising altitude on the Qinghai–Tibet Plateau. Therefore, plateau pikas inhabiting high-altitude environments can survive extreme environments by increasing their metabolic rate, and gene expression of skeletal muscle thermogenesis and adipose tissue. Browning increases the expression of UCPI to promote BAT cell differentiation, thermogenesis, and metabolism. These physiological and gene expression changes confer plateau pika the ability to survive in an extreme environment.
Data Availability Statement
The datasets presented in this study can be found in online repositories. The names of the repository/repositories and accession number(s) can be found in the article/Supplementary Material.
Ethics Statement
The animal study was reviewed and approved by The Ethics Committee of Northwest Institute of Plateau Biology, Chinese Academy of Sciences (NWIPB-20201201). Written informed consent was obtained from the owners for the participation of their animals in this study.
Author Contributions
JQ and HZ conceived the study. ZHJ and LZ collected samples. HZ, JL and LZ completed the majority of the experiments. HZ written the manuscript. JQ wrote and reviewed the manuscript. All author contributed to the articles and approved the final manuscript.
Funding
This work was supported by the Second Tibetan Plateau Scientific Expedition and Research (STEP) program (2019QZKK05010410, 2019QZKK05010212), Strategic Priority Research Program of Chinese Academy of Sciences (XDA2002030302, XDA26020201), National Natural Science Foundation of China (31770459), CAS “Light of West China” for Interdisciplinary Innovation Team, Qinghai Province Natural Sciences Foundation (2021-ZJ-929), Joint Grant from Chinese Academy of Sciences-People’s Government of Qinghai Province on Sanjiangyuan National Park (LHZX-2020-01).
Conflict of Interest
The authors declare that the research was conducted in the absence of any commercial or financial relationships that could be construed as a potential conflict of interest.
Publisher’s Note
All claims expressed in this article are solely those of the authors and do not necessarily represent those of their affiliated organizations, or those of the publisher, the editors and the reviewers. Any product that may be evaluated in this article, or claim that may be made by its manufacturer, is not guaranteed or endorsed by the publisher.
Acknowledgments
We thank all team members for their helpful discussions. We also thank reviewers for their helpful comments and suggestions.
Supplementary Material
The Supplementary Material for this article can be found online at: https://www.frontiersin.org/articles/10.3389/fgene.2021.784811/full#supplementary-material
References
Arnold, P. A., Delean, S., Cassey, P., and White, C. R. (2021). Meta-analysis Reveals that Resting Metabolic Rate Is Not Consistently Related to Fitness and Performance in Animals. J. Comp. Physiol. B 191, 1097–1110. doi:10.1007/s00360-021-01358-w
Bai, Z., Wuren, T., Liu, S., Han, S., Chen, L., and McClain, D. (2015). Intermittent Cold Exposure Results in Visceral Adipose Tissue “browning” in the Plateau Pika (Ochotona Curzoniae). Comp. Biochem. Physiol. A: Mol. Integr. Physiol. 184, 171–178. doi:10.1016/j.cbpa.2015.01.019
Bal, N. C., Maurya, S. K., Sopariwala, D. H., Sahoo, S. K., Gupta, S. C., Shaikh, S. A., et al. (2012). Sarcolipin Is a Newly Identified Regulator of Muscle-Based Thermogenesis in Mammals. Nat. Med. 18 (10), 1575–1579. doi:10.1038/nm.2897
Bal, N. C., Sahoo, S. K., Maurya, S. K., and Periasamy, M. (2018). The Role of Sarcolipin in Muscle Non-shivering Thermogenesis. Front. Physiol. 9, 1217. doi:10.3389/fphys.2018.01217
Boratyński, J. S., Jefimow, M., and Wojciechowski, M. S. (2017). Individual Differences in the Phenotypic Flexibility of Basal Metabolic Rate in Siberian Hamsters Are Consistent on Short-And Long-Term Timescales. Physiol. Biochem. Zool. 90 (2), 139–152. doi:10.1086/689870
Bozinovic, F. (1992). Rate of Basal Metabolism of Grazing Rodents from Different Habitats. J. Mammalogy 73 (2), 379–384. doi:10.2307/1382072
Burton, T., Killen, S., Armstrong, J., and Metcalfe, N. (2011). What Causes Intraspecific Variation in Resting Metabolic Rate and what Are its Ecological Consequences? Proc. R. Soc. B: Biol. Sci. 278 (1724), 3465–3473. doi:10.1098/rspb.2011.1778
Cao, X.-F., Bai, Z.-Z., Ma, L., Ma, S., and Ge, R.-L. (2017). Metabolic Alterations of Qinghai–Tibet Plateau Pikas in Adaptation to High Altitude. High Alt. Med. Biol. 18 (3), 219–225. doi:10.1089/ham.2016.0147
Cheviron, Z. A., Natarajan, C., Projecto-Garcia, J., Eddy, D. K., Jones, J., Carling, M. D., et al. (2014). Integrating Evolutionary and Functional Tests of Adaptive Hypotheses: a Case Study of Altitudinal Differentiation in Hemoglobin Function in an Andean Sparrow, Zonotrichia capensis. Mol. Biol. Evol. 31 (11), 2948–2962. doi:10.1093/molbev/msu234
Chi, Q.-S., and Wang, D.-H. (2011). Thermal Physiology and Energetics in Male Desert Hamsters (Phodopus Roborovskii) during Cold Acclimation. J. Comp. Physiol. B 181 (1), 91–103. doi:10.1007/s00360-010-0506-6
Ding, B.-Y., Chi, Q.-S., Liu, W., Shi, Y.-L., and Wang, D.-H. (2018). Thermal Biology of Two Sympatric Gerbil Species: The Physiological Basis of Temporal Partitioning. J. Therm. Biol. 74, 241–248. doi:10.1016/j.jtherbio.2018.03.025
Elsen, M., Raschke, S., Tennagels, N., and Schwahn, U. (2014). BMP4 and BMP7 Induce the white-to-brown Transition of Primary Human Adipose Stem Cells. Am. J. Physiology-Cell Physiol. 306 (5), C431–C440. doi:10.1152/ajpcell.00290.2013
Finck, B. N., and Kelly, D. P. (2006). PGC-1 Coactivators: Inducible Regulators of Energy Metabolism in Health and Disease. J. Clin. Invest. 116 (3), 615–622. doi:10.1172/JCI27794
Fox, R. J., Donelson, J. M., Schunter, C., Ravasi, T., and Gaitán-Espitia, J. D. (2019). Beyond Buying Time: the Role of Plasticity in Phenotypic Adaptation to Rapid Environmental Change. Phil. Trans. R. Soc. B: Biol. Sci. 374 (1768), 20180174. doi:10.1098/rstb.2018.0174
Geiser, F. (2004). Metabolic Rate and Body Temperature Reduction during Hibernation and Daily Torpor. Annu. Rev. Physiol. 66, 239–274. doi:10.1146/annurev.physiol.66.032102.115105
Gordon, C. (2012). Thermal Physiology of Laboratory Mice: Defining Thermoneutrality. J. Therm. Biol. 37 (8), 654–685. doi:10.1016/j.jtherbio.2012.08.004
Han, H.-S., Kang, G., Kim, J. S., Choi, B. H., and Koo, S.-H. (2016). Regulation of Glucose Metabolism from a Liver-Centric Perspective. Exp. Mol. Med. 48 (3), e218. doi:10.1038/emm.2015.122
Handschin, C., Rhee, J., Lin, J., Tarr, P. T., and Spiegelman, B. M. (2003). An Autoregulatory Loop Controls Peroxisome Proliferator-Activated Receptor γ Coactivator 1α Expression in Muscle. Proc. Natl. Acad. Sci. 100 (12), 7111–7116. doi:10.1073/pnas.1232352100
Hanya, G., and Chapman, C. A. (2013). Linking Feeding Ecology and Population Abundance: a Review of Food Resource Limitation on Primates. Ecol. Res. 28 (2), 183–190. doi:10.1007/s11284-012-1012-y
Hao, Y., Xiong, Y., Cheng, Y., Song, G., Jia, C., Qu, Y., et al. (2019). Comparative Transcriptomics of 3 High-Altitude Passerine Birds and Their Low-Altitude Relatives. Proc. Natl. Acad. Sci. 116 (24), 11851–11856. doi:10.1073/pnas.1819657116
Harms, M., and Seale, P. (2013). Brown and Beige Fat: Development, Function and Therapeutic Potential. Nat. Med. 19 (10), 1252–1263. doi:10.1038/nm.3361
Healy, K., McNally, L., Ruxton, G. D., Cooper, N., and Jackson, A. L. (2013). Metabolic Rate and Body Size Are Linked with Perception of Temporal Information. Anim. Behav. 86 (4), 685–696. doi:10.1016/j.anbehav.2013.06.018
Herzig, S., Long, F., Jhala, U. S., Hedrick, S., Quinn, R., Bauer, A., et al. (2001). CREB Regulates Hepatic Gluconeogenesis through the Coactivator PGC-1. Nature 413 (6852), 179–183. doi:10.1038/3509812310.1038/35093131
Hondares, E., Rosell, M., Díaz-Delfín, J., Olmos, Y., Monsalve, M., Iglesias, R., et al. (2011). Peroxisome Proliferator-Activated Receptor α (PPARα) Induces PPARγ Coactivator 1α (PGC-1α) Gene Expression and Contributes to Thermogenic Activation of Brown Fat: Involvement of PRDM16. J. Biol. Chem. 286 (50), 43112–43122. doi:10.1074/jbc.M111.252775
Hou, D. M., Jia, T., Tao, J. Q., Wang, Z. K., Guan, B.-R., and Zhu, W.-L. (2020). Browning Plasticity of white Adipose Tissue in Tree Shrew during Cold Acclimation and Rewarming. J. Vertebr. Biol. 70 (1), 20097–20091. doi:10.25225/jvb.20097
Humphries, M. M., Boutin, S., Thomas, D. W., Ryan, J. D., Selman, C., McAdam, A. G., et al. (2005). Expenditure Freeze: the Metabolic Response of Small Mammals to Cold Environments. Ecol. Lett. 8 (12), 1326–1333. doi:10.1111/j.1461-0248.2005.00839.x
Kabelik, D., Julien, A. R., Ramirez, D., and O'Connell, L. A. (2021). Social Boldness Correlates with Brain Gene Expression in Male green Anoles. Horm. Behav. 133, 105007. doi:10.1016/j.yhbeh.2021.105007
Ke, R., Xu, Q., Li, C., Luo, L., and Huang, D. (2018). Mechanisms of AMPK in the Maintenance of ATP Balance during Energy Metabolism. Cel Biol. Int. 42 (4), 384–392. doi:10.1002/cbin.10915
Killen, S. S., Glazier, D. S., Rezende, E. L., Clark, T. D., Atkinson, D., Willener, A. S., et al. (2016). Ecological Influences and Morphological Correlates of Resting and Maximal Metabolic Rates across Teleost Fish Species. The Am. Naturalist 187 (5), 592–606. doi:10.1086/685893
Li, J., Yang, Q., Bai, Z., Zhou, W., Semenza, G. L., and Ge, R.-L. (2018b). Chronic Cold Exposure Results in Subcutaneous Adipose Tissue browning and Altered Global Metabolism in Qinghai–Tibetan Plateau Pika (Ochotona Curzoniae). Biochem. biophysical Res. Commun. 500 (2), 117–123. doi:10.1016/j.bbrc.2018.03.147
Li, M., Hao, Z., Wanlong, Z., and Zhengkun, W. (2019). Seasonal Variations of Adipose Tissue in Tupaia Belangeri (Mammalia: Scandentia: Tupaiidae). Eur. Zoolog. J. 86 (1), 54–62. doi:10.1080/24750263.2019.1572798
Li, M., Tian, S., Jin, L., Zhou, G., Li, Y., Zhang, Y., et al. (2013). Genomic Analyses Identify Distinct Patterns of Selection in Domesticated Pigs and Tibetan Wild Boars. Nat. Genet. 45 (12), 1431–1438. doi:10.1038/ng.2811
Li, Q., Sun, R., Huang, C., Wang, Z., Liu, X., Hou, J., et al. (2001). Cold Adaptive Thermogenesis in Small Mammals from Different Geographical Zones of China. Comp. Biochem. Physiol. Part A: Mol. Integr. Physiol. 129 (4), 949–961. doi:10.1016/S1095-6433(01)00357-9
Li, T., Gao, D., Xie, L., Deng, C., Shi, P., Guan, M.-L., et al. (2018a). Comparative Genomic Investigation of High-Elevation Adaptation in Ectothermic Snakes. Proc. Natl. Acad. Sci. 115 (33), 8406–8411. doi:10.1073/pnas.1805348115
Liu, M., Qu, J., Yang, M., Wang, Z., Wang, Y., Zhang, Y., et al. (2012). Effects of Quinestrol and Levonorgestrel on Populations of Plateau Pikas, Ochotona Curzoniae, in the Qinghai–Tibetan Plateau. Pest Manag. Sci. 68 (4), 592–601. doi:10.1002/ps.2302
Liu, X., and Chen, B. (2000). Climatic Warming in the Tibetan Plateau during Recent Decades. Int. J. Climatology: A J. R. Meteorol. Soc. 20 (14), 1729–1742. doi:10.1002/1097-0088(20001130)20:14<172910.1002/1097-0088(20001130)20:14<1729:aid-joc556>3.0.co;2-y
Luo, Y., Gao, W., Gao, Y., Tang, S., Huang, Q., Tan, X., et al. (2008). Mitochondrial Genome Analysis of Ochotona Curzoniae and Implication of Cytochrome C Oxidase in Hypoxic Adaptation. Mitochondrion 8 (5-6), 352–357. doi:10.1016/j.mito.2008.07.005
Mannuthy, T. (2017). Behavioral Responses to Livestock Adaptation to Heat Stress Challenges. Asian J. Anim. Sci. 11 (1), 1–13. doi:10.3923/ajas.2017.1.13
Maurya, S. K., Bal, N. C., Sopariwala, D. H., Pant, M., Rowland, L. A., Shaikh, S. A., et al. (2015). Sarcolipin Is a Key Determinant of the Basal Metabolic Rate, and its Overexpression Enhances Energy Expenditure and Resistance against Diet-Induced Obesity. J. Biol. Chem. 290 (17), 10840–10849. doi:10.1074/jbc.M115.636878
Maurya, S. K., Herrera, J. L., Sahoo, S. K., Reis, F. C., Vega, R. B., Kelly, D. P., et al. (2018). Sarcolipin Signaling Promotes Mitochondrial Biogenesis and Oxidative Metabolism in Skeletal Muscle. Cel Rep. 24 (11), 2919–2931. doi:10.1016/j.celrep.2018.08.036
McKechnie, A. E., and Swanson, D. L. (2010). Sources and Significance of Variation in Basal, summit and Maximal Metabolic Rates in Birds. Curr. Zoolog. 56 (6), 741–758. doi:10.1093/czoolo/56.6.741
McKie, G. L., Medak, K. D., Knuth, C. M., Shamshoum, H., Townsend, L. K., Peppler, W. T., et al. (2019). Housing Temperature Affects the Acute and Chronic Metabolic Adaptations to Exercise in Mice. J. Physiol. 597 (17), 4581–4600. doi:10.1113/JP278221
McNab, B. K. (2009). Ecological Factors Affect the Level and Scaling of Avian BMR. Comp. Biochem. Physiol. Part A: Mol. Integr. Physiol. 152 (1), 22–45. doi:10.1016/j.cbpa.2008.08.021
Menzies, A. (2021). Warm Bodies in Cold Places: Thermoregulation, Activity, and Energy Expenditure of Boreal Homeotherms in winter. in press.
Meyer, C. W., Willershäuser, M., Jastroch, M., Rourke, B. C., Fromme, T., Oelkrug, R., et al. (2010). Adaptive Thermogenesis and thermal Conductance in Wild-type and UCP1-KO Mice. Am. J. Physiology-Regulatory, Integr. Comp. Physiol. 299 (5), R1396–R1406. doi:10.1152/ajpregu.00021.2009
Mineo, P. M., Cassell, E. A., Roberts, M. E., and Schaeffer, P. J. (2012). Chronic Cold Acclimation Increases Thermogenic Capacity, Non-shivering Thermogenesis and Muscle Citrate Synthase Activity in Both Wild-type and Brown Adipose Tissue Deficient Mice. Comp. Biochem. Physiol. Part A: Mol. Integr. Physiol. 161 (4), 395–400. doi:10.1016/j.cbpa.2011.12.012
Nedergaard, J., Bengtsson, T., and Cannon, B. (2007). Unexpected Evidence for Active Brown Adipose Tissue in Adult Humans. Am. J. Physiology-Endocrinology Metab. 293, E444–E452. doi:10.1152/ajpendo.00691.2006
Nespolo, R. F., Bacigalupe, L. D., Rezende, E. L., and Bozinovic, F. (2001). When Nonshivering Thermogenesis Equals Maximum Metabolic Rate: thermal Acclimation and Phenotypic Plasticity of Fossorial Spalacopus cyanus (Rodentia). Physiol. Biochem. Zool. 74 (3), 325–332. doi:10.1086/320420
Nowack, J., Giroud, S., Arnold, W., and Ruf, T. (2017). Muscle Non-shivering Thermogenesis and its Role in the Evolution of Endothermy. Front. Physiol. 8, 889. doi:10.3389/fphys.2017.00889
O'Brien, K. A., Simonson, T. S., and Murray, A. J. (2020). Metabolic Adaptation to High Altitude. Curr. Opin. Endocr. Metab. Res. 11, 33–41. doi:10.1016/j.coemr.2019.12.002
Oh, K.-J., Han, H.-S., Kim, M.-J., and Koo, S.-H. (2013). CREB and FoxO1: Two Transcription Factors for the Regulation of Hepatic Gluconeogenesis. BMB Rep. 46 (12), 567. doi:10.5483/BMBRep.2013.46.12.248
Olanrewaju, H., Purswell, J., Collier, S., and Branton, S. (2013). Interactive Effects of Photoperiod and Light Intensity on Blood Physiological and Biochemical Reactions of Broilers Grown to Heavy Weights. Poult. Sci. 92 (4), 1029–1039. doi:10.3382/ps.2012-02792
Oswald, K. N., Lee, A. T. K., and Smit, B. (2018). Seasonal Physiological Responses to Heat in an alpine Range-Restricted Bird: the Cape Rockjumper (Chaetops Frenatus). J. Ornithology 159 (4), 1063–1072. doi:10.1007/s10336-018-1582-8
Projecto-Garcia, J., Natarajan, C., Moriyama, H., Weber, R. E., Fago, A., Cheviron, Z. A., et al. (2013). Repeated Elevational Transitions in Hemoglobin Function during the Evolution of Andean Hummingbirds. Proc. Natl. Acad. Sci. 110 (51), 20669–20674. doi:10.1073/pnas.1315456110
Qu, J., Li, W., Yang, M., Ji, W., and Zhang, Y. (2013). Life History of the Plateau Pika (Ochotona Curzoniae) in alpine Meadows of the Tibetan Plateau. Mamm. Biol. 78 (1), 68–72. doi:10.1016/j.mambio.2012.09.005
Qu, J., Réale, D., Fletcher, Q. E., and Zhang, Y. (2019). Among-population Divergence in Personality Is Linked to Altitude in Plateau Pikas (Ochotona Curzoniae). Front. Zoolog. 16 (1), 1–7. doi:10.1186/s12983-019-0329-6
Rotter, D., Peiris, H., Grinsfelder, D. B., Martin, A. M., Burchfield, J., Parra, V., et al. (2018). Regulator of Calcineurin 1 Helps Coordinate Whole‐body Metabolism and Thermogenesis. EMBO Rep. 19 (12), e44706. doi:10.15252/embr.201744706
Rui, L. (2014). Energy Metabolism in the Liver. Compr. Physiol. 4 (1), 177. doi:10.1002/cphy.c130024
Ryder, J. W., Bassel-Duby, R., Olson, E. N., and Zierath, J. R. (2003). Skeletal Muscle Reprogramming by Activation of Calcineurin Improves Insulin Action on Metabolic Pathways. J. Biol. Chem. 278 (45), 44298–44304. doi:10.1074/jbc.M304510200
Sahoo, S. K., Shaikh, S. A., Sopariwala, D. H., Bal, N. C., and Periasamy, M. (2013). Sarcolipin Protein Interaction with Sarco (Endo) Plasmic Reticulum Ca2+ ATPase (SERCA) Is Distinct from Phospholamban Protein, and Only Sarcolipin Can Promote Uncoupling of the SERCA Pump. J. Biol. Chem. 288 (10), 6881–6889. doi:10.1074/jbc.M112.436915
Scantlebury, M., Bennett, N. C., Speakman, J. R., Pillay, N., and Schradin, C. (2006). Huddling in Groups Leads to Daily Energy Savings in Free-Living African Four-Striped Grass Mice, Rhabdomys pumilio. Funct. Ecol. 20 (1), 166–173. doi:10.1111/j.1365-2435.2006.01074.x
Schaeffer, P. J., Wende, A. R., Magee, C. J., Neilson, J. R., Leone, T. C., Chen, F., et al. (2004). Calcineurin and Calcium/calmodulin-dependent Protein Kinase Activate Distinct Metabolic Gene Regulatory Programs in Cardiac Muscle. J. Biol. Chem. 279 (38), 39593–39603. doi:10.1074/jbc.M403649200
Seale, P., Conroe, H. M., Estall, J., Kajimura, S., Frontini, A., Ishibashi, Jeff., et al. (2011). Prdm16 Determines the Thermogenic Program of Subcutaneous white Adipose Tissue in Mice. J. Clin. Invest. 121 (1), 96–105. doi:10.1172/JCI44271
Selman, C., McLaren, J. S., Collins, A. R., Duthie, G. G., and Speakman, J. R. (2013). Deleterious Consequences of Antioxidant Supplementation on Lifespan in a Wild-Derived Mammal. Biol. Lett. 9 (4), 20130432. doi:10.1098/rsbl.2013.0432
Shaikh, S. A., Sahoo, S. K., and Periasamy, M. (2016). Phospholamban and Sarcolipin: Are They Functionally Redundant or Distinct Regulators of the Sarco (Endo) Plasmic Reticulum Calcium ATPase? J. Mol. Cell. Cardiol. 91, 81–91. doi:10.1016/j.yjmcc.2015.12.030
Smith, A. T., and Foggin, J. M. (1999). The Plateau Pika (Ochotona Curzoniae) Is a keystone Species for Biodiversity on the Tibetan Plateau. Anim. Conservation 2 (4), 235–240. doi:10.1111/j.1469-1795.1999.tb00069.x
Speakman, J. R., Chi, Q., Ołdakowski, Ł., Fu, H., Fletcher, Q. E., Hambly, C., et al. (2021). Surviving winter on the Qinghai–Tibetan Plateau: Pikas Suppress Energy Demands and Exploit Yak Feces to Survive winter. Proc. Natl. Acad. Sci. 118 (30), 1–11. doi:10.1073/pnas.2100707118
Storz, J. F., and Scott, G. R. (2019). Life Ascending: Mechanism and Process in Physiological Adaptation to High-Altitude Hypoxia. Annu. Rev. Ecol. Evol. Syst. 50 (1), 503–526. doi:10.1146/annurev-ecolsys-110218-025014
Su, C., Xie, T., Wang, Y., Si, C., Li, L., Ma, J., et al. (2020). Miocene Diversification and High-Altitude Adaptation of Parnassius Butterflies (Lepidoptera: Papilionidae) in Qinghai–Tibet Plateau Revealed by Large-Scale Transcriptomic Data. Insects 11 (11), 754. doi:10.3390/insects11110754
Sukhchuluun, G., Zhang, X.-Y., Chi, Q.-S., and Wang, D.-H. (2018). Huddling Conserves Energy, Decreases Core Body Temperature, but Increases Activity in Brandt's Voles (Lasiopodomys Brandtii). Front. Physiol. 9, 563. doi:10.3389/fphys.2018.00563
Sun, H., Niu, Y., Chen, Y.-S., Song, B., Liu, C.-Q., Peng, D.-L., et al. (2014). Survival and Reproduction of Plant Species in the Qinghai–Tibet Plateau. J. Syst. Evol. 52 (3), 378–396. doi:10.1111/jse.12092
Swanson, D. L., McKechnie, A. E., and Vézina, F. (2017). How Low Can You Go? an Adaptive Energetic Framework for Interpreting Basal Metabolic Rate Variation in Endotherms. J. Comp. Physiol. B 187 (8), 1039–1056. doi:10.1007/s00360-017-1096-3
Tan, C., Yu, Y., Jiang, Z., Zhong, L., Zhang, Y., and Qu, J. (2020). Differences in Exploration and Resting Metabolic Rates of Plateau Pikas (Ochotona Curzoniae) at Different Altitudes. Acta Theriologica Sinica 40 (1), 27–36. doi:10.16829/j.slxb.150288
Tattersall, G. J., Sinclair, B. J., Withers, P. C., Fields, P. A., Seebacher, F., Cooper, C. E., et al. (2012). Coping with thermal Challenges: Physiological Adaptations to Environmental Temperatures. Compr. Physiol. 2 (3), 2151–2202. doi:10.1002/cphy.c110055
Tickle, P. G., Hutchinson, J. R., and Codd, J. R. (2018). Energy Allocation and Behaviour in the Growing Broiler Chicken. Scientific Rep. 8 (1), 1–13. doi:10.1038/s41598-018-22604-2
Tószögyová, A. (2020). Bird Diversity and Life-History Patterns along Gradients of Productivity and its Variation. [dissertation/doctor’s thesis]. [(Czech Republic) (CZ)]. Prague: Charles University.
Van Beest, F. M., Van Moorter, B., and Milner, J. M. (2012). Temperature-mediated Habitat Use and Selection by a Heat-Sensitive Northern Ungulate. Anim. Behav. 84 (3), 723–735. doi:10.1016/j.anbehav.2012.06.032
Van Sant, M. J., and Hammond, K. A. (2008). Contribution of Shivering and Nonshivering Thermogenesis to Thermogenic Capacity for the Deer Mouse (Peromyscus maniculatus). Physiol. Biochem. Zool. 81 (5), 605–611. doi:10.1086/588175
Vicenzi, N., Bacigalupe, L. D., Laspiur, A., Ibargüengoytía, N., and Sassi, P. L. (2021). Could Plasticity Mediate highlands Lizards’ Resilience to Climate Change? A Case Study of the Leopard iguana (Diplolaemus Leopardinus) in central andes of Argentina. J. Exp. Biol. 224 (14), jeb242647. doi:10.1242/jeb.242647
Wang, B., Zhang, Y.-B., Zhang, F., Lin, H., Wang, X., Wan, N., et al. (2011). On the Origin of Tibetans and Their Genetic Basis in Adapting High-Altitude Environments. PLoS ONE 6 (2), e17002. doi:10.1371/journal.pone.0017002
Wang, D., and Wang, Z. (1990). Survival Strategies of Small Mammals in Alpine Environment II: Seasonal Variation of Nonshivering Thermogenesis (NST) of Pika Pika and Vole microtus. Acta Theriologica Sinica 10 (1), 40–53. doi:10.16829/j.slxb.1990.01.007
Wang, J.-M., Zhang, Y.-M., and Wang, D.-H. (2006). Seasonal Thermogenesis and Body Mass Regulation in Plateau Pikas (Ochotona Curzoniae). Oecologia 149 (3), 373–382. doi:10.1007/s00442-006-0469-1
Wang, Y., Shan, S., Zhang, H., Dong, B., Zheng, W., and Liu, J. (2019). Physiological and Biochemical Thermoregulatory Responses in Male Chinese Hwameis to Seasonal Acclimatization: Phenotypic Flexibility in a Small Passerine. Zoolog. Stud. 58, e6. doi:10.6620/ZS.2019.58-06
Wei, D., Wei, L., Li, X., Wang, Y., and Wei, L. (2016). Effect of Hypoxia on Ldh-C Expression in Somatic Cells of Plateau Pika. Int. J. Environ. Res. Public Health 13 (8), 773. doi:10.3390/ijerph13080773
Wiersma, P., Muñoz-Garcia, A., Walker, A., and Williams, J. B. (2007). Tropical Birds Have a Slow Pace of Life. Proc. Natl. Acad. Sci. 104 (22), 9340–9345. doi:10.1073/pnas.0702212104
Wilson, M. C., and Smith, A. T. (2015). The Pika and the Watershed: The Impact of Small Mammal Poisoning on the Ecohydrology of the Qinghai-Tibetan Plateau. AMBIO 44 (1), 16–22. doi:10.1007/s13280-014-0568-x
Xie, L., Zhang, X., Qi, D., Guo, X., Pang, B., Du, Y., et al. (2014). Inhibition of Inducible Nitric Oxide Synthase Expression and Nitric Oxide Production in Plateau Pika (Ochotona Curzoniae) at High Altitude on Qinghai-Tibet Plateau. Nitric Oxide 38, 38–44. doi:10.1016/j.niox.2014.02.009
Yang, J., Zhao, X., Guo, S., Li, H., Qi, D., Wang, D., et al. (2006). Leptin cDNA Cloning and its mRNA Expression in Plateau Pikas (Ochotona Curzoniae) from Different Altitudes on Qinghai-Tibet Plateau. Biochem. biophysical Res. Commun. 345 (4), 1405–1413. doi:10.1016/j.bbrc.2006.05.052
Yang, Y., Wang, L., Han, J., Tang, X., Ma, M., Wang, K., et al. (2015). Comparative Transcriptomic Analysis Revealed Adaptation Mechanism of Phrynocephalus Erythrurus, the Highest Altitude Lizard Living in the Qinghai-Tibet Plateau. BMC Evol. Biol. 15 (1), 1–14. doi:10.1186/s12862-015-0371-8
Yaskin, V. (2011). Seasonal Changes in hippocampus Size and Spatial Behavior in Mammals and Birds. Biol. Bull. Rev. 1 (3), 279–288. doi:10.1134/S2079086411030108
Yu, F., Li, S., Kilpatrick, W. C., McGuire, P. M., He, K., and Wei, W. (2012). Biogeographical Study of Plateau Pikas Ochotona Curzoniae (Lagomorpha, Ochotonidae). Zoolog. Sci. 29 (8), 518–526. doi:10.2108/zsj.29.518
Yu, L., Wang, G.-D., Ruan, J., Chen, Y.-B., Yang, C.-P., Cao, X., et al. (2016). Genomic Analysis of Snub-Nosed Monkeys (Rhinopithecus) Identifies Genes and Processes Related to High-Altitude Adaptation. Nat. Genet. 48 (8), 947–952. doi:10.1038/ng.3615
Yu, Y., Zhong, L., Zhu, H., Tan, C., and Qu, J. (2021). Effects of Warble Flies (Oestromyia Leporine) Parasite on the Physiological Traits and Personality of Plateau Pika (Ochotona Curzoniae). Grassland and Turf 41 (2), 1–10. doi:10.13817/j.cnki.cyycp.2021.02.001
Zhang, L., Yang, F., Wang, Z.-k., and Zhu, W.-l. (2017). Role of thermal Physiology and Bioenergetics on Adaptation in Tree Shrew (Tupaia Belangeri): the experiment Test. Scientific Rep. 7, 41352. doi:10.1038/srep41352
Zhang, X., Liu, X., and Wang, D. (2012). Seasonal Changes in Body Mass and Energy Balance in Wild Small Mammals. Springer Berlin Heidelberg, 207–216. doi:10.1007/978-3-642-29056-5_13
Zheng, W.-H., Li, M., Liu, J.-S., Shao, S.-L., and Xu, X.-J. (2014a). Seasonal Variation of Metabolic Thermogenesis in Eurasian Tree Sparrows (Passer Montanus) over a Latitudinal Gradient. Physiol. Biochem. Zool. 87 (5), 704–718. doi:10.1086/676832
Zheng, W.-H., Liu, J.-S., and Swanson, D. L. (2014b). Seasonal Phenotypic Flexibility of Body Mass, Organ Masses, and Tissue Oxidative Capacity and Their Relationship to Resting Metabolic Rate in Chinese Bulbuls. Physiol. Biochem. Zool. 87 (3), 432–444. doi:10.1086/675439
Zhou, S.-S., Cao, L.-L., Xu, W.-D., Cao, J., and Zhao, Z.-J. (2015). Effect of Temperature on Oxidative Stress, Antioxidant Levels and Uncoupling Protein Expression in Striped Hamsters. Comp. Biochem. Physiol. Part A: Mol. Integr. Physiol. 189, 84–90. doi:10.1016/j.cbpa.2015.07.017
Zhu, K., Ge, D., Wen, Z., Xia, L., and Yang, Q. (2018). Evolutionary Genetics of Hypoxia and Cold Tolerance in Mammals. J. Mol. Evol. 86 (9), 618–634. doi:10.1007/s00239-018-9870-8
Zhu, W., Hou, D., Sun, S., and Wang, Z. (2017). White Adipose Tissue Undergoes ‘browning’in Tree Shrews (Tupaia Belangeri) during Cold Acclimation. Mammal Study 42 (4), 1–8. doi:10.3106/041.042.0405
Zhu, W. L., Zhang, D., Hou, D. M., and G, Y. (2017). Roles of Hypothalamic Neuropeptide Gene Expression in Body Mass Regulation in Eothenomys Miletus (Mammalia: Rodentia: Cricetidae). Eur. Zoolog. J. 84 (1), 322–333. doi:10.1080/24750263.2017.1334840
Zhu, W. L., Zhang, H., and Wang, Z.-k. (2012). Seasonal Changes in Body Mass and Thermogenesis in Tree Shrews (Tupaia Belangeri): the Roles of Photoperiod and Cold. J. Therm. Biol. 37 (7), 479–484. doi:10.1016/j.jtherbio.2012.04.007
Keywords: plateau pika, thermogenic capacity, energy metabolism, gene expression, altitude
Citation: Zhu H, Zhong L, Li J, Wang S and Qu J (2022) Differential Expression of Metabolism-Related Genes in Plateau Pika (Ochotona curzoniae) at Different Altitudes on the Qinghai–Tibet Plateau. Front. Genet. 12:784811. doi: 10.3389/fgene.2021.784811
Received: 28 September 2021; Accepted: 28 December 2021;
Published: 20 January 2022.
Edited by:
Chao Tong, University of Pennsylvania, United StatesCopyright © 2022 Zhu, Zhong, Li, Wang and Qu. This is an open-access article distributed under the terms of the Creative Commons Attribution License (CC BY). The use, distribution or reproduction in other forums is permitted, provided the original author(s) and the copyright owner(s) are credited and that the original publication in this journal is cited, in accordance with accepted academic practice. No use, distribution or reproduction is permitted which does not comply with these terms.
*Correspondence: Jiapeng Qu, jpqu@nwipb.cas.cn