- 1State Key Laboratory of Crop Genetics and Germplasm Enhancement, Centre of Pear Engineering Technology Research, Nanjing Agricultural University, Nanjing, China
- 2Department of Cell Biology and Molecular Genetics, University of Maryland, College Park, MD, United States
Type 2C protein phosphatase (PP2C) plays an essential role in abscisic acid (ABA) signaling transduction processes. In the current study, we identify 719 putative PP2C genes in eight Rosaceae species, including 118 in Chinese white pear, 110 in European pear, 73 in Japanese apricot, 128 in apple, 74 in peach, 65 in strawberry, 78 in sweet cherry, and 73 in black raspberry. Further, the phylogenetic analysis categorized PbrPP2C genes of Chinese white pear into twelve subgroups based on the phylogenic analysis. We observed that whole-genome duplication (WGD) and dispersed gene duplication (DSD) have expanded the Rosaceae PP2C family despite simultaneous purifying selection. Expression analysis finds that PbrPP2C genes have organ-specific functions. QRT-PCR validation of nine PbrPP2C genes of subgroup A indicates a role in ABA-mediated response to abiotic stress. Finally, we find that five PbrPP2C genes of subgroup A function in the nucleus. In summary, our research suggests that the PP2C family functions to modulate ABA signals and responds to abiotic stress.
Introduction
Protein phosphorylation is a fundamental signal that regulates cellular processes, including growth factor responses, hormone responses, metabolic control, and developmental processes, and as with all signals, removal is as important as induction (den Hertog 1999; Kerk et al., 2002; Schweighofer et al., 2007). Protein kinases (PKs) phosphorylate serine (Ser), threonine (Thr), and tyrosine (Tyr) residues, whereas protein phosphatases (PPs) can reverse this action by removing the phosphate group (Luan, 1998; Wei et al., 2014). Therefore, the PPs are classified into three groups based on substrate specificity: protein Tyr phosphatases (PTPs), Ser/Thr phosphatases (STPs), and dual-specificity phosphatases (DSPTPs) (Cohen, 1989; Kerk et al., 2008). STPs are further divided into three subgroups: the phosphor-protein phosphatase (PPP), Mg2+- or Mn2+-dependent protein phosphatase (PPM), and aspartate-based protein phosphatases (Cohen 1989; Kerk et al., 2008). The PPP family covers PP1, PP2A, PP4, PP5, PP6, PP7, and PP2B, whereas the PPM family includes type 2C protein phosphatases (PP2Cs) and also pyruvate dehydrogenase phosphatases (Cohen, 1989; Kerk et al., 2008).
PP2Cs modulate and regulate protein kinase signaling cascades in archaea, bacteria, fungi, plants, and animals (Cao et al., 2016). In higher plants, PP2C genes were demonstrated to negatively regulate signaling pathways by opposing specific protein kinases (Tähtiharju and Palva, 2001; Yoshida et al., 2006). In Arabidopsis, 76 PP2C genes have been identified and categorized into ten groups (A–J), with the remaining six being uncategorized. Several PP2C genes from subgroup A have been verified as factors in ABA signaling (Hirayama and Umezawa, 2010). AP2C1 of subgroup B interacts with MPK4 or MPK6 to suppress MAPK activates in response to wounding and pathogen stresses (Schweighofer et al., 2007). POL or PLL1 of PP2C of subgroup C interacts with the receptor kinase CLV1 to regulate flower development and maintain stem cell polarity (Song et al., 2008; Gagne and Clark, 2010). AtPP2C6-6 of PP2C of subgroup E interacts with histone acetyl transferase AtGCN5 to modulate stomatal signaling (Servet et al., 2008). WIN2 of PP2C of subgroup F interacts with the bacterial effector HopW1-1 to induce stress response (Lee et al., 2008). Likewise, KAPP of unclustered PP2Cs interacts with RLKs to regulate plant immunity responses and hormonal signaling (Gomez-Gomez et al., 2001).
PP2Cs regulate plant development in both biotic and abiotic stress conditions (Sugimoto et al., 2014; Singh et al., 2016). At the molecular level, the PP2C function reflects its role in modulating signals transmitted by abscisic acid (ABA) (Merlot et al., 2001; Yoshida et al., 2006). In the ABA signaling pathway, PP2Cs can inactivate SnRK2 via dephosphorylation, and this inactivation was inhibited by ABA receptors (PYR/PYL/RCRA) (Soon et al., 2012). In Arabidopsis, proteins encoded by PP2CA, ABI1, and ABI2 function in tolerance to exposure to salt, drought, and freezing (Strizhov et al., 1997; Merlot et al., 2001), and proteins encoded by HAB1, HAB2, and AHG1 negatively regulate SnRK2 kinases required for ABA signaling (Saez et al., 2004; Saez et al., 2008; Umezawa et al., 2009). In Fagus sylvatica, ectopic expression of FsPP2C1 in Arabidopsis resulted in ABA insensitivity during seed germination (Gonzalez-Garcia et al., 2003), but ectopic expression of FsPP2C2 in Arabidopsis resulted in enhanced ABA sensitivity and tolerance of abiotic stress in seeds (Reyes et al., 2006). This link between PP2C proteins and ABA signaling is ancient. In moss, PpABI1A and PpABI1B of subgroup A of PP2C function in drought tolerance via downregulation of ABA signaling (Komatsu et al., 2013), and in maize, ZmPP2C-A10 also regulates drought stress tolerance (Xiang et al., 2017). Taken together, PP2C genes in subgroup A have been demonstrated to play key roles in plant development and environmental stresses.
In this study, we identify 719 PP2C genes from eight Rosaceae species. At the genomic level, we analyze the expression and phylogeny of the 118 PP2C genes found in Chinese white pear and analyze its evolution. At the protein level, we describe protein features and functions, domains of expression, and subcellular localization. Our findings set a foundation to understand the function of PbrPP2C genes in mediating responses to various stress conditions in a commercially important family of higher plants.
Materials and Methods
Sequence Retrieval Resources and Identification of Type 2C Protein Phosphatase Genes
For identification of PP2C genes in pear and other Rosaceae species, the HMM (hidden Markov odel) files were constructed by downloading the seed file PP2C (PF00481) domains from Pfam (http://pfam.xfam.org/) and were searched against the local protein databases using HMMER3 (Finn et al., 2015). The protein sequences of candidate PP2C genes were validated by using Interproscan 63.0 (http://www.ebi.ac.uk/InterProScan/) and Pfam (http://pfam.xfam.org/). The methods of screening and identification were identical to a previous report (Qanmber et al., 2019a; Qanmber et al., 2019b). Arabidopsis PP2C genes were downloaded from TAIR (http://www.arabidopsis.org/). Chinese white pear (Pyrus bretschneideri) and Japanese apricot’s (Prunus mume) genome sequences were obtained from the Pear Genome Project (http://peargenome.njau.edu.cn/) and Prunus mume Genome Project (http://prunusmumegenome.bjfu.edu.cn/index.jsp), respectively. The genome sequences of European pear (Pyrus communis), apple (Malus domestica), peach (Prunus persica), strawberry (Fragaria vesca), black raspberry (Rubus occidentalis), and sweet cherry (Prunus avium) were collected from the Genome database for Rosaceae (GDR) (http://www.rosaceae.org). The obtained PP2C protein sequences were screened for the PP2C catalytic domain by using the Pfam website (https://pfam.xfam.org/).
Phylogenetic, Exon–Intron Structure, and Protein Motif Analysis
The full length protein sequences of PP2C were used to perform multiple sequence alignment, and the phylogenetic tree was performed using MEGA7.0 (Kumar et al., 2016) with the maximum likelihood method (ML), a bootstrap of 1,000 replications, and the Jones–Taylor–Thornton (JTT) model. The exon–intron organization of PbrPP2C genes was analyzed using CDSs and genomic DNA sequences using the Gene Structure Display Server (GSDS: http://gsds.cbi.pku.edu.cn/). Motif Elicitation (MEME: http://meme.sdsc.edu/meme/itro.html) was performed to identify conserved motifs of PbrPP2C proteins, with the maximum number of motifs = 20.
Cis-Element Predictions of PbrPP2C
All the PbrPP2C promoter sequences (selected as 2000 upstream bp) were downloaded from the Pear Genome Project (http://peargenome.njau.edu.cn/). The cis-regulatory elements of PbrPP2C were identified by the PlantCARE database (http://bioinformatics.psb.ugent.be/webtools/plantcare/html/).
Chromosomal Locations, Synteny, and Ka/Ks Analysis of Type 2C Protein Phosphatase Genes
The chromosomal location information of the PP2C family genes was obtained from the genome annotation files. The analysis of synteny among eight Rosaceae genomes was performed by PGDD (http://chibba.agtec.uga.edu/duplication/) (Lee et al., 2013). BLASTP was carried out to identify multiple alignments of protein sequences (e-value < 10–5, top 5 matches) in the eight Rosaceae species. Then, MCScanX was carried out to produce orthologous gene pairs of PP2C within each Rosaceae species (Wang et al., 2012). Segmental/whole-genome duplication (WGD), tandem duplication (TD), proximal duplication (PD), transposed duplication (TRD), and dispersed duplication (DSD) in the PP2C gene family were identified by using the tools in the MCScanX package (Qiao et al., 2019). Localization and duplicate gene pairs of the PP2C genes were visualized using TB tools software (Chen et al., 2020). The values of Ka (non-synonymous substitutions) and Ks (synonymous substitutions) were calculated using KaKs_Calculator 2.0 with default parameters, and the Ka/Ks ratio was based on a model-averaged method (Wang et al., 2010).
Transcriptome Expression Pattern Analysis in Different Tissues and in Different Ages of Pear Fruit
Previously published and unpublished dynamic RNA-seq data were used to analyze PP2C gene expression in different tissues of pear (Qiao et al., 2018; Li et al., 2019), including the pollen, seed, sepal, petal, ovary, bud, stem, leaf, and fruit. The raw RNA-seq reads were cleaned by removing low-quality reads (quality score <15), poly (A/T) tails, and adapter sequences. HISAT2 and feature counts were performed to align clean reads to the reference genome and estimate transcript abundance levels (Liao et al., 2014; Kim et al., 2015). Finally, the values of fragments per kilobase million (FPKM) were used to indicate the expression levels of PP2C genes. The heatmap of PP2C gene expression was visualized using TB tools software (Chen et al., 2020).
Plant Material and Treatment
“Cuiguan” pear (Pyrus pyrifolia Nakai) seeds were collected from the pear germplasm orchard of the Pear Engineering Technology Research Center of Nanjing Agricultural University in Nanjing, China. Seedlings were grown for 5 weeks in a growth chamber, with a photoperiod of 16/8 h and a temperature of 25 ± 1°C. The seedlings were irrigated with 200 mM NaCl and 20% PEG 6000 for salinity and drought abiotic stress, respectively. Seedling leaves were sprayed with 100 μM ABA for ABA treatment. Seedlings were subjected to temperatures of 4°C and 37°C for low and high temperature stress, respectively. The leaves of treated seedling were collected at 0, 6, 12 and 24 h, respectively. The collected samples were quickly frozen in liquid nitrogen and stored at −80°C until further use.
Quantitative Real-Time PCR Analysis
Total RNA was extracted using RNAprep Pure Plant Kit (Tiangen, Beijing, China). The extracted total RNAs were subjected to the first-strand cDNA using TransScript One-Step gDNA Removal and cDNA synthesis Supermix (TransGen, Beijing, China). The primers of 9 PP2C genes were designed using Primer Premier 6.0, and the tubulin gene of pear was used as the reference gene. All the primer sequences are listed in Supplementary Table S5. QRT-PCR was carried out in LightCycler 480 SYBRGREEN I Master (Roche, United States), and the reaction mixture and cycling program were identical to those of a previous report (Hao et al., 2018). All of the analyses were carried out with three independent biological replicates. The genes expression levels were calculated using the 2−ΔΔCt method (Livak and Schmittgen, 2001).
Subcellular Localization
For the subcellular localization analysis of PbrPP2Cs, the CDS sequences without the termination codon were amplified and cloned into pCAMBIA1300-35S: CDS-GFP vector. Primers used for cloning are listed in Supplementary Table S5. The recombinant plasmids and the control plasmid were transformed into 30-day-old tobacco (Nicotiana benthamiana) leaves according to the published protocol (Sparkes et al., 2006). Fluorescence was imaged using a confocal microscope LSM780 (Zeiss LSM 780, Germany).
Results
Identification, Characteristics, and Phylogenetic Relationship of Type 2C Protein Phosphatase Genes
A total of 719 putative PP2C genes were identified in eight Rosaceae species: 118 in Chinese white pear, 110 in European pear, 73 in Japanese apricot, 128 in apple, 74 in peach, 65 in strawberry (Haider et al., 2019), 78 in sweet cherry, and 73 in black raspberry (Supplementary Table S1). 118 putative PP2C genes of pear were arranged as PbrPP2C1 to PbrPP2C118 based on phylogenic analysis and the relative position of Arabidopsis orthologs. In addition, some members of the PbrPP2C gene family have two alternative splice variants, including Pbr012020, Pbr019958, Pbr019984, Pbr022419, and Pbr031084 (Supplementary Table S1). Splicing variants played a crucial role in the posttranscriptional regulatory mechanism that modulates transcriptome and proteome diversity, such as alternative splicing of PpDAM1 was important in the pear flower bud dormancy process (Li et al., 2021). The lengths of PP2C gene sequences ranged from 203 bp to 21,156 bp. Moreover, the protein molecular weights were 7.5–243 kDa, and the theoretical isoelectric point was from 3.9 to 10.49. The PP2C gene ID and the characteristics are shown in Supplementary Table S1.
To gain insights into the phylogenetic relationship of the PP2C genes in pear, a phylogenetic tree was constructed using MEGA7.0 by adopting the maximum likelihood method (ML) based on multiple sequence alignments of 80 Arabidopsis PP2C genes obtained from a previous study (Xue et al., 2008) and 118 pear PbrPP2C genes (Figure 1). This analysis divided 118 PbrPP2C genes into twelve subgroups: subgroups A–L and one unclassified subgroup U (Figure 1 and Supplementary Figure S1). Subgroups A, D, and G contain 18, 17, and 17 genes, respectively. Subgroup J, K, and L contain less.
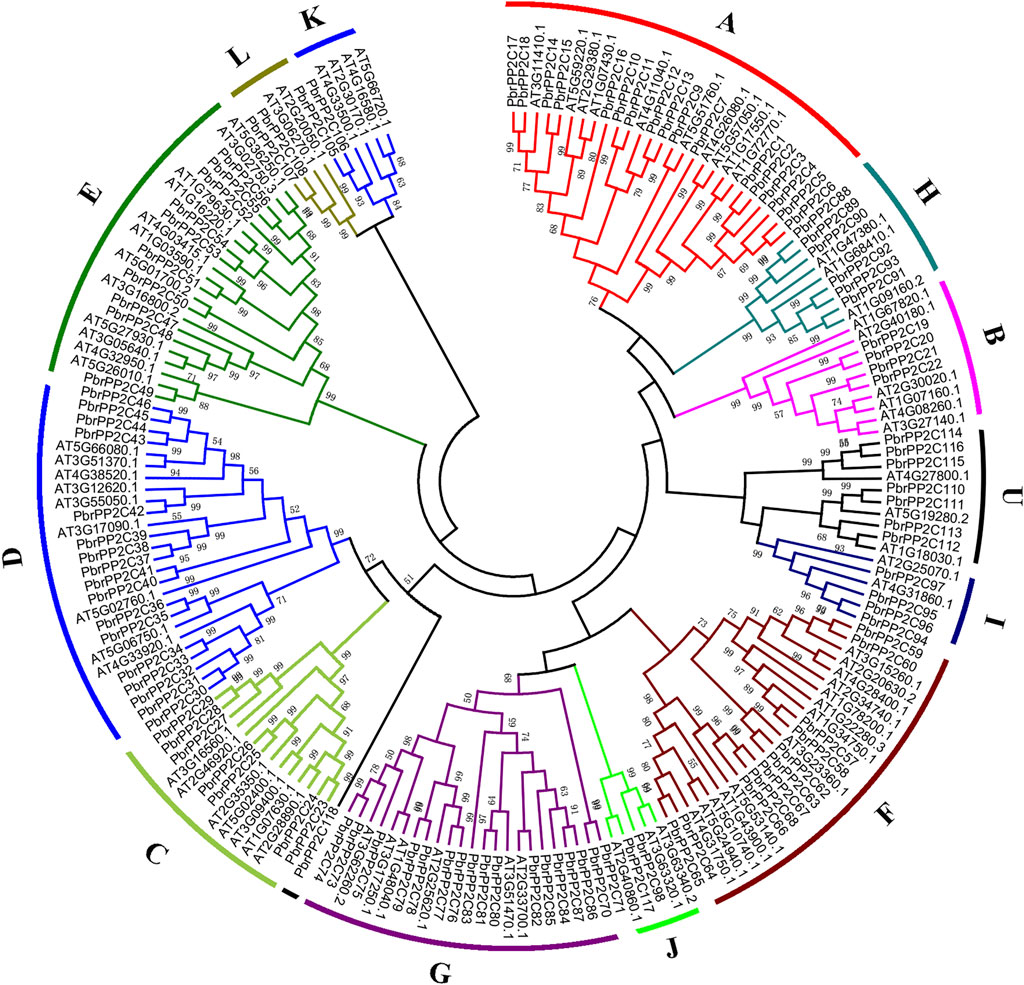
FIGURE 1. Phylogenetic analysis of the PbrPP2C family in both Chinese white pear and Arabidopsis. Phylogenetic tree was constructed using the maximum likelihood method.
In addition, the conserved motif analysis of PbrPP2C genes supported the phylogenetic analysis and classification (Supplementary Figure S1). A total of 20 motifs were identified in all the PbrPP2C family members. The PP2C proteins of each subgroup contained similar motifs. Motifs 1 and 5 were detected in almost all of the PbrPP2C genes, while Motif 4 was specific to subgroups C and D (Supplementary Figure S1). Most members of PbrPP2C subgroups contained more than 10 motifs, while a few members had 2–4 motifs, such as PbrPP2C16, PbrPP2C71, PbrPP2C85, PbrPP2C113, and PbrPP2C118. Different subgroups contain their own specific motifs that may lead to the functional divergence of each subgroup. To better understand the structures of PbrPP2C genes in pear, exon–intron organizations were compared among different subgroups (Supplementary Figure S1). The number of exons in the PbrPP2C family members varied from 1 to 21, and 37 genes were annotated in the 3′or 5′UTR region. Interestingly, PbrPP2C genes in the same subgroup show more or less similar exon–intron structures (Supplementary Figure S1).
Chromosome Location and Collinearity Analysis of the Type 2C Protein Phosphatase Gene Family
To explore the contribution of different gene duplication modes to the expansion and evolution of PP2C genes in eight Rosaceae species, a comparative analysis of gene duplication was performed in each genome (Figure 2, Supplementary Table S2). 1,014 duplicated gene pairs were found in the PP2C family members and were assigned to five duplication modes of WGD, PD, TD, TRD, or DSD. WGD is responsible for 8.2–44.4% of PP2C gene pairs in the investigated species. Consistent with lineage-specific duplications, Chinese white pear (32.4%), European pear (44.4%), and apple (40.0%) exhibit a relatively high proportion of WGD-derived PP2C genes. DSD accounts for the highest number of derived genes (48.2–72.9%), but TD (0–2.4%) and PD (0–2.7%) were observed with low frequency. TRD (3.3–18.4%) of PP2C gene pairs shows a high frequency in each of the Rosaceae species, which contributed to the formation of the PP2C gene clusters observed. The results are consistent with the inference that different gene duplication modes of PP2C gene pairs may have led to the neofunctionalization of ancestral genes.
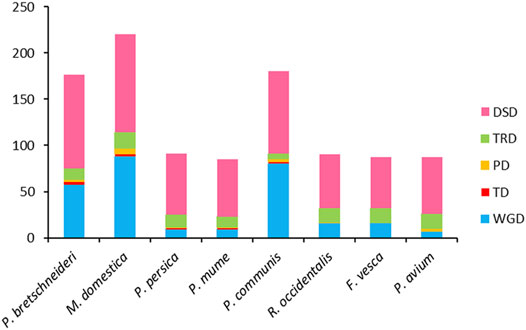
FIGURE 2. Number of PP2C gene pairs derived from different modes of gene duplication in pear and seven other Rosaceae species. X-axis represents the species. Y-axis represents the number of duplicated gene pairs. WGD: whole-genome duplication, TD: tandem duplication, PD: proximal duplication, TRD: transposed duplication, and DSD: dispersed duplication.
We located the 719 PP2C genes on the chromosomes of each species based on genome annotations. For Chinese white pear, 118 of PbrPP2C genes were anchored onto all the 17 chromosomes and scaffolds, with the maximum number of genes detected on Chr 15 (17), followed by 11 genes on Chr 5. However, there was no correlation between the number of genes and the length of chromosomes, and the genes were randomly distributed on each chromosome (Figure 3A, Supplementary Table S2). PP2C genes were also found to be randomly distributed in the other Rosaceae species’ genomes. We identified 274 collinearity gene pairs, including 59 pairs in Chinese white pear, 16 pairs in strawberry, 78 pairs in apple, 80 pairs in European pear, 10 pairs in Japanese apricot, 8 pairs in peach, 16 pairs in black raspberry, and 7 pairs in sweet cherry (Figure 3, Supplementary Table S2). A large number of collinearity gene pairs were identified in Chinese white pear, European pear, and apple species.
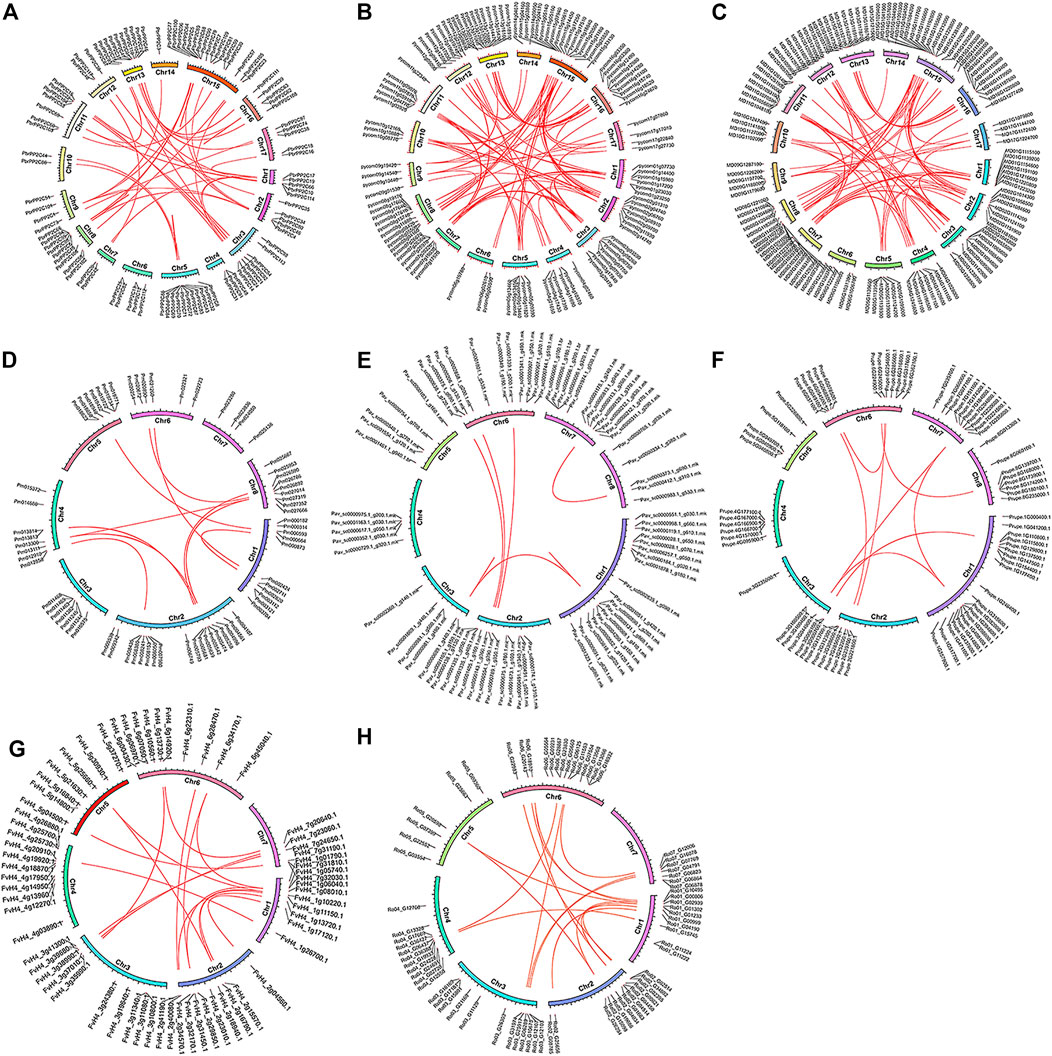
FIGURE 3. Gene location and collinearity analysis of the PP2C gene family. (A) Chinese white pear; (B) European pear; (C) apple; (D) Japanese apricot; (E) sweet cherry; (F) peach; (G) strawberry; (H) black raspberry. Genes were located on different chromosomes. Red lines represent the syntenic gene pairs.
Ka and Ks Substitutions per Site and Ka/Ks Analysis for Type 2C Protein Phosphatase Family Genes
The Ks value has been widely used to estimate the evolutionary stage of WGD events (Qiao et al., 2015). The mean Ks values of WGD-derived gene pairs in apple, European pear, Chinese white pear, Japanese apricot, black raspberry, sweet cherry, strawberry, and peach were 1.70, 1.90, 2.21, 2.40, 2.57, 2.907, 2.93, and 3.48, respectively (Supplementary Table S3). The lower Ks values of WGD-derived gene pairs in apple and European pear suggested that they were duplicated and retained from more recent WGD events, while sweet cherry, strawberry and peach were derived from more ancient WGD events. The Ka/Ks ratio is usually used to measure the magnitude and direction of selection pressure, and the Ka/Ks value refers to selection type: >1 indicate positive selection and <1 indicates purifying selection (Yang 2007). Purifying selection can remove deleterious mutations, and positive selection can induce favorable mutations (Starr et al., 2003). Here, the Ka/Ks values of PP2C orthologous gene pairs were calculated among eight Rosaceae species (Figure 4). The Ka/Ks values of duplicated gene pairs in European pear, Japanese apricot, black raspberry, and strawberry were less than 1, suggesting that PP2C genes evolved under strong purifying selection. Several gene pairs with higher Ka/Ks ratios were identified in apple, Chinese white pear, sweet cherry, and peach, suggesting that these genes may have a complicated evolutionary history. For Chinese white pear, the mean Ka/Ks ratios of WGD TD, PD, TRD, and DSD, were 0.17, 0.45, 0.98, 0.14, and 0.21, respectively (Figure 4). TD and PD had higher Ka/Ks ratios compared with other molds of duplicated gene pairs, suggesting that they evolved at a higher rate than the other gene pairs.
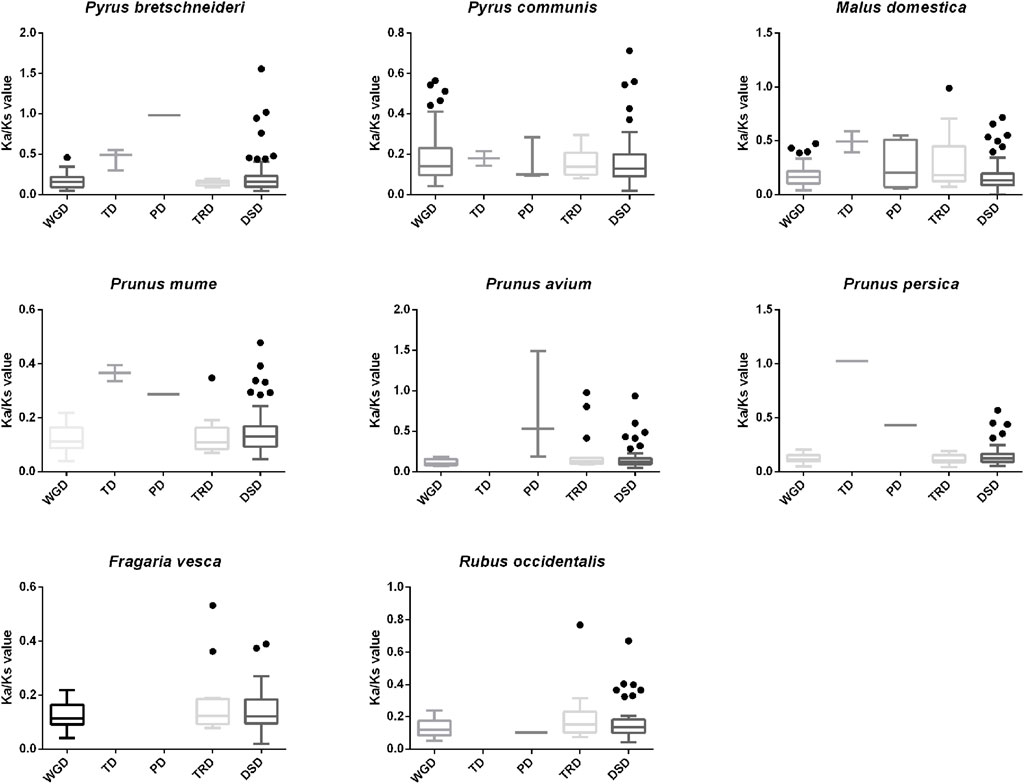
FIGURE 4. Ka/Ks distribution of eight Rosaceae species. Ka/Ks values were analyzed using coding sequences. X-axis represents five different duplication categories. Y-axis indicates the Ka/Ks ratio. T-boxplot was also constructed using prism 6.0.
Analysis of Putative Regulatory Cis-elements of the Type 2C Protein Phosphatase Gene Family
The cis-elements in promoter regions are closely related to gene transcription, and they play a critical role in plant signal transduction by interacting with their cognate transcription factor. Therefore, to better understand the function of PbrPP2C genes, 2.0 kb upstream promoter sequences of PbrPP2C genes were downloaded from the Pear Genome Database and analyzed by using PlantCARE. Some common cis-regulatory elements are briefly summarized and listed in Supplementary Table S6, such as ABRE was involved in ABA responsiveness, ERE was involved in ethylene responsiveness, MBS was the MYB binding site involved in drought-inducibility, and DRE was involved in adverse stress. The result showed that various cis-elements were related to plant hormones, light, abiotic stress, essential element, enhancer, circadian factors, and other regulatory stress responses (Supplementary Table S6). Consequently, various conserved cis-regulatory elements of PbrPP2C genes were crucial in mediating responses to various stress-related hormones or adverse biotic–abiotic stresses.
Expression Profiling of the Type 2C Protein Phosphatase Gene Family in Different Tissues of Pear
To investigate the expression patterns of PbrPP2C family genes in different pear tissues, a heatmap was constructed using previously published RNA-seq data including matured pollen, seed, petal, sepal, ovary, stem, bud, leaf, and fruit (Figure 5 and Supplementary Table S4). Most PbrPP2C genes displayed a very broad expression range, and several PbrPP2C genes showed expressional activation in at least three or more tissues. Five genes (PbrPP2C71, PbrPP2C72, PbrPP2C71, PbrPP2C102, PbrPP2C103, and PbrPP2C118) exhibited very low or no expression. Eighteen PbrPP2C genes were found to be highly expressed preferentially in leaves, and five were highly expressed in buds. We identified three genes with ovary-specific expression (PbrPP2C12, PbrPP2C16, and PbrPP2C49), three were pollen-specific (PbrPP2C9, PbrPP2C50, and PbrPP2C62), and one gene exhibited sepal-specific expression (PbrPP2C69) (Figure 5). This analysis has identified candidate PbrPP2C genes that may play specialized roles in different organs’ development.
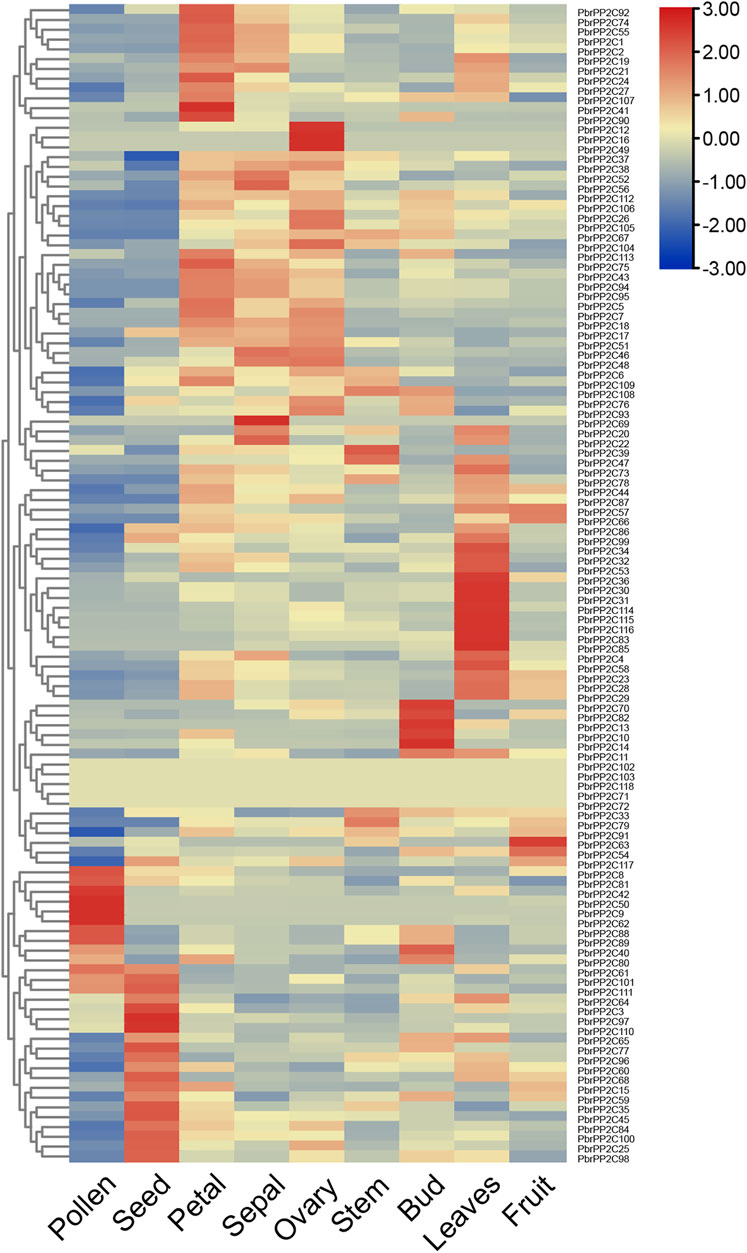
FIGURE 5. Heatmap of expression profiles (FPKM) for PP2C in the nine various tissues of pear (pollen, seed, petal, sepal, ovary, stem, bud, leaf, and fruit). Expression levels are indicated by the color bar.
To further verify the role of PP2C-mediated stress response, we analyzed the transcriptome of pear under biotic/abiotic stresses and pathogen treatments based on unpublished and published data of our laboratory (Li et al., 2016; Yang and Huang, 2018). The findings of the heatmaps showed that a large number of PbrPP2C genes responded to stress, and PbrPP2C genes showed variation in their expression pattern among different treatments (Supplementary Figure S2). In addition, most of PbrPP2Cs from subgroup A were also differentially upregulated by exposure to cold, drought, salt, and pathogen treatments, such as PbrPP2C1, PbrPP2C4, PbrPP2C7, PbrPP2C11, PbrPP2C17, and PbrPP2C18 (Supplementary Figure S2). The diversity in the expression profiling of PbrPP2C genes may suggest that these PbrPP2C genes were stress-responsive.
qRT-PCR Analysis of PbrPP2C
To explore PbrPP2C gene expression under different stress conditions and identify PbrPP2C genes important for improving tolerance, the seedlings were subjected to heat, cold, drought, NaCl, and ABA treatments. It has been verified that subgroup A PP2Cs in Arabidopsis and rice were transcriptionally upregulated under exogenous ABA treatment or abiotic stress that stimulates ABA biosynthesis (Xue et al., 2008; Singh et al., 2010). Nine PbrPP2C genes from subgroup A were selected to perform qRT-PCR at different time points after various treatments. QRT-PCR after exogenous ABA treatment indicated that four genes (PbrPP2C10, PbrPP2C11, PbrPP2C15, and PbrPP2C18) were upregulated at three different time points: 6, 12, and 24 h. Four more genes (PbrPP2C1/2, PbrPP2C4, PbrPP2C6, and PbrPP2C17) were upregulated more than 5-fold in 24 h after exogenous ABA treatment. In contrast, the expression of PbrPP2C6 and PbrPP2C7 decreased to less than half that of CK in 6 and 12 h, respectively (Figure 6). Nine PbrPP2C genes from subgroup A were upregulated in response to more than one treatment with abiotic stress (Figure 6). For example, the PbrPP2C6 expression level increased more than 3-fold under exposure to heat, drought, and salt treatment, but exposure to cold repressed its expression (Figure 6). All nine genes exhibited strongly increased expression levels (from 10-fold to 400-fold greater than CK) in response to NaCl treatment (Figure 6). Some results were consistently consistent with the analysis of heatmaps (Supplementary Figure S2). Taken together, the expression characteristics of subgroup A PbrPP2C genes indicate that these nine genes respond to exogenous ABA and abiotic stress.
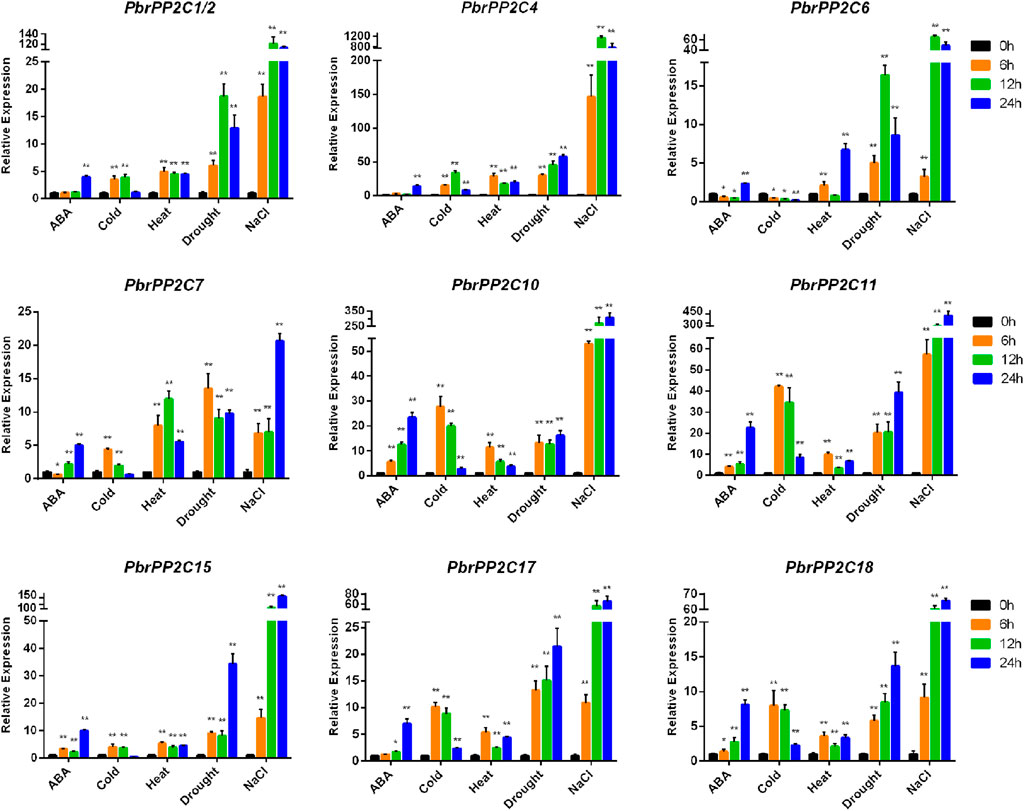
FIGURE 6. QRT-PCR analysis of subgroup A PbrPP2C genes under exposure to heat, cold, drought, NaCl, and ABA treatments in pear seedlings. Standard errors and ANOVA were calculated by applying Student’s t-test. Single and double stars stand for the levels of significant difference at p-value < 0.05 and <0.01, respectively.
Subcellular Localization of PbrPP2C Protein
Previous research has shown that PbrPP2C proteins localize in the nucleus (Haider et al., 2019; Kim et al., 2012). To determine the subcellular localizations of PbrPP2C proteins, five PbrPP2C genes (PbrPP2C1, PbrPP2C4, PbrPP2C7, PbrPP2C10, and PbrPP2C15) were selected from each branch from subfamily A of the PbrPP2C family. PbrPP2Cs-GFP recombinant plasmids were introduced into N. benthamiana leaves. The fluorescence confirms the localization of five PbrPP2C-GFP fusion proteins in the nucleus (Figure 7). ABA receptor proteins, namely PYR/PYL/RCRA, are localized in both the cytoplasm and nucleus, despite the fact that interacting PP2C proteins are localized exclusively in the nucleus (Santiago et al., 2009; Kim et al., 2012). This observation suggests that PbrPP2C may play a transcriptional regulatory role in the nucleus.
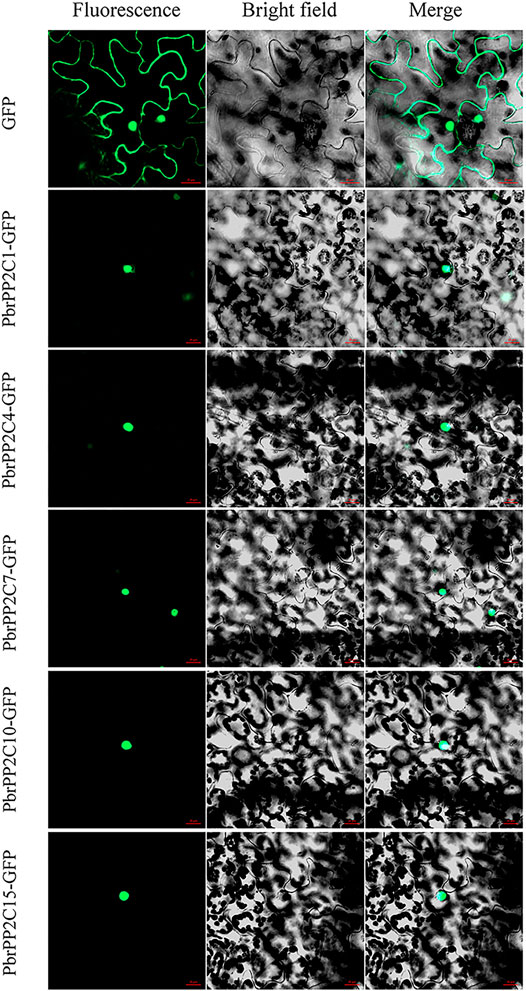
FIGURE 7. Subcellular localization of the fusion protein PbrPP2Cs-GFP in N. benthamiana leaves. Vector 35S-GFP was used as the control. Bar = 20 μm.
Discussion
The landscape of the PP2C genes family in the plant kingdom has been characterized in previous research studies, such as in Arabidopsis (Kerk et al., 2002), rice (Singh et al., 2010), maize (Wei and Pan 2014), banana (Hu et al., 2017), Brachypodium distachyon (Cao et al., 2016), Brassica rapa (Khan et al., 2019), and Gossypium hirsutum (Shazadee et al., 2019). However, the PP2C gene family has not been studied widely in the Rosaceae family. The current study provides a comprehensive analysis of the PP2C gene family in eight Rosaceae species, including gene identification, phylogenetic relationships, chromosomal localizations, and evolutionary analysis. 719 PP2Cs were identified, ranging from 65 genes in strawberry to 128 in apple. The genes exhibited widespread and uneven distribution across Rosaceae chromosomes (Figure 3). In Chinese white pear, 118 PbrPP2C genes were further categorized into twelve subgroups A–L and one unclassified subgroup according to phylogenetic and evolutionary analysis (Figure 1). This classification of PP2C genes was consistent with previous studies, such as those conducted on Arabidopsis (Kerk et al., 2002) and rice (Singh et al., 2010). PbrPP2C genes were found to be expressed in specific organs, providing strong evidence of specialized function. At least eight AtPP2C genes from subgroup A were key factors in the ABA signaling network (Hirayama and Umezawa, 2010). AP2C1 of subgroup B was involved in suppressing MAPK activates in response to wounding or pathogen stresses (Schweighofer et al., 2007). POL and PLL1 of subgroup C were involved in regulating flower development and maintain stem cell polarity (Song et al., 2008; Gagne and Clark, 2010). PP2C of subgroup E and AtPP2C6-6 were involved in modulating stomata signaling (Servet et al., 2008). WIN2 of subgroup F was involved in inducing the stress response (Lee et al., 2008). Overall, PP2C genes of the same subgroup have similar specialized biological functions, although the functions of many PP2C subgroups are still unclear.
Gene duplication is the predominant driving force for broad expansion of the gene family, which could obtain new functions and evolutionary processes (Qiao et al., 2019). The different types of gene duplications, including WGD, TD, PD, TRD, and DSD (Doerks et al., 2002; Moore and Purugganan, 2003), contribute differently to the expansion of gene families (Freeling, 2009). In the genome and genetic evolutionary system, WGD is the main driving force of new functions and features of eukaryotic genome evolution (Friedman and Hughes, 2001; Moore and Purugganan, 2003). For instance, the BES1 and GhERF subfamily B3 group gene families in cotton were expanded primarily though segmental or WGD duplication events (Liu et al., 2018; Lu et al., 2021). F-box and heat-shock transcription factor families in pear were expanded primarily through WGD and DSD (Qiao et al., 2015; Wang et al., 2016). In our study, we demonstrated WGD replication events in eight Rosaceae species (Figure 3). We also show that WGD and DSD were the driving forces for the expansion of the PP2C gene family in Chinese white pear, European pear, and apple (Figure 2). Finally, we found that most Ka/Ks ratios of PP2C gene pairs were less than one, suggesting that these genes have experienced strong purifying selection.
Surrounded by various stress factors, such as ABA, drought, salt, heat, cold, and phytohormonal stresses, are the major limiting factors of plant development and agricultural crop production, and the role of ABA signaling in stress adaptation and stress resistance mechanisms has been well documented (Sugimoto et al., 2014; Singh et al., 2016). Group A of PP2Cs comprises of negative regulators of ABA signaling by PYL intracellular receptors (Antoni et al., 2012). In Arabidopsis, at least six genes of group A PP2C (ABI1, ABI2, HAB1, HAB1, HAB2, PP2CA, and AHG1) resulted in increasing the ABA sensitivity under various stresses, indicating the diverse outcome in ABA signaling (Merlot et al., 2001; Tähtiharju and Palva, 2001; Umezawa et al., 2009). In Fagus sylvatica, two genes of group A PP2C (FsPP2C1 and FsPP2C2) resulted in influencing ABA sensitivity and tolerance of abiotic stress in seeds (Gonzalez-Garcia et al., 2003; Reyes et al., 2006). In maize, ZmPP2C-A10 of subgroup A of PP2C was confirmed for its negative regulation in drought stress (Xiang et al., 2017). Although PP2C genes in subgroup A have been demonstrated to play key roles in various stress conditions in some species, the role of the key components of ABA signaling against PP2C genes is mainly obscure in pear. In the present study, nine PbrPP2C genes from subgroup A exhibited substantial transcriptional variations when confronted by heat, cold, drought, and NaCl challenges and in response to ABA treatment, indicating their regulatory role in stress tolerance. Gene expression levels exhibited, especially, strong response to salt stress (Figure 6). Therefore, the study reveals potential functions of PP2C genes in a commercially important angiosperm family. However, validation of the individual gene product’s function at the molecular level remains an important step in understanding PP2C genes in the Rosacaea family in future.
5. Conclusion
In this study, a total of 719 PP2C gene family members were first identified in eight Rosaceae species. The PP2C gene pairs of Rosaceae species might evolve undergoing strong purifying selection. The 118 PbrPP2C genes of Chinese white pear were classified into twelve subgroups according to the phylogenetic relationship gene structure and protein motif pattern. Moreover, qRT-PCR revealed nine candidate genes from subgroup A which might have participated in the plant stress tolerance particularly to ABA, heat, cold, drought, and NaCl stress. Subcellular localization analysis proved the functionality of five PbrPP2C genes from each branch of subfamily A in the nucleus. Consequently, our findings provide a foundation for the potential function of PbrPP2C genes under various stress conditions.
Data Availability Statement
The original contributions presented in the study are included in the article/Supplementary Material; further inquiries can be directed to the corresponding authors.
Author Contributions
GW carried out the experiments and wrote the manuscript. CG and SZ designed the experiment and revised the manuscript. XQ contributed to collinearity analysis and the Perl script. XS, XG and ZG performed the experiments. DJ, LG and KQ directed and revised the manuscript. All authors have read and approved the final manuscript.
Funding
This work was financially supported by Jiangsu Agriculture Science and Technology Innovation Fund (CX (19)2028), the National Natural Science Foundation of China (31830081), the National Key Research and Development Program of China (2018YFD1000107), the Fundamental Research Funds for the Central Universities (JCQY201901), the Project Funded by the Priority Academic Program Development of Jiangsu Higher Education Institutions, the Earmarked Fund for China Agriculture Research System (CARS-28).
Conflict of Interest
The authors declare that the research was conducted in the absence of any commercial or financial relationships that could be construed as a potential conflict of interest.
Publisher’s Note
All claims expressed in this article are solely those of the authors and do not necessarily represent those of their affiliated organizations, or those of the publisher, the editors, and the reviewers. Any product that may be evaluated in this article, or claim that may be made by its manufacturer, is not guaranteed or endorsed by the publisher.
Acknowledgments
The authors thank the laboratory members for assistance. This work was supported by the high-performance computing platform of Bioinformatics Center, Nanjing Agricultural University.
Supplementary Material
The Supplementary Material for this article can be found online at: https://www.frontiersin.org/articles/10.3389/fgene.2021.770014/full#supplementary-material
Abbreviations
PK, protein kinase; PP, protein phosphatase; Ser, serine; Thr, threonine; Tyr, tyrosine; PTP, protein threonine phosphatase; STP, serine/threonine phosphatase; DSPTP, dual-specificity phosphatase; PPP, phosphor-protein phosphatase; PPM, the Mg2+- or Mn2+-dependent protein phosphatase; PP2C, type 2C protein phosphatase; HMM, hidden Markov mode; WGD, Whole-genome duplication; TD, tandem duplication; TRD, transposed duplication; PD, proximal duplication; DSD, dispersed duplication; ML, maximum likelihood; PGDD, llant genome duplication database; GDR, Genome Database for Rosaceae; RPKM, reads per kilobase of exon model per million mapped reads; Ka, non-synonymous substitution rates; Ks, synonymous substitution rates.
References
Antoni, R., Gonzalez-Guzman, M., Rodriguez, L., Rodrigues, A., Pizzio, G. A., and Rodriguez, P. L. (2012). Selective Inhibition of Clade A Phosphatases Type 2C by PYR/PYL/RCAR Abscisic Acid Receptors. Plant Physiol. 158, 970–980. doi:10.1104/pp.111.188623
Cao, J., Jiang, M., Li, P., and Chu, Z. (2016). Genome-Wide Identification and Evolutionary Analyses of the PP2C Gene Family with Their Expression Profiling in Response to Multiple Stresses in Brachypodium Distachyon. BMC Genomics 17, 175. doi:10.1186/s12864-016-2526-4
Chen, C., Chen, H., Zhang, Y., Thomas, H. R., Frank, M. H., He, Y., et al. (2020). TBtools: An Integrative Toolkit Developed for Interactive Analyses of Big Biological Data. Mol. Plant 13, 1194–1202. doi:10.1016/j.molp.2020.06.009
Cohen, P. (1989). The Structure and Regulation of Protein Phosphatases. Annu. Rev. Biochem. 58, 453–508. doi:10.1146/annurev.bi.58.070189.002321
den Hertog, J. (1999). Protein-Tyrosine Phosphatases in Development. Mech. Develop. 85, 3–14. doi:10.1016/s0925-4773(99)00089-1
Doerks, T., Copley, R. R., Schultz, J., Ponting, C. P., and Bork, P. (2002). Systematic Identification of Novel Protein Domain Families Associated with Nuclear Functions. Genome Res. 12, 47–56. doi:10.1101/gr.203201
Finn, R. D., Clements, J., Arndt, W., Miller, B. L., Wheeler, T. J., Schreiber, F., et al. (2015). HMMER Web Server: 2015 Update. Nucleic Acids Res. 43, W30–W38. doi:10.1093/nar/gkv397
Freeling, M. (2009). Bias in Plant Gene Content Following Different Sorts of Duplication: Tandem, Whole-Genome, Segmental, or by Transposition. Annu. Rev. Plant Biol. 60, 433–453. doi:10.1146/annurev.arplant.043008.092122
Friedman, R., and Hughes, A. L. (2001). Pattern and Timing of Gene Duplication in Animal Genomes. Genome Res. 11, 1842–1847. doi:10.1101/gr.200601
Gagne, J. M., and Clark, S. E. (2010). The Arabidopsis Stem Cell Factor Poltergeist Is Membrane Localized and Phospholipid Stimulated. Plant Cell. 22, 729–743. doi:10.1105/tpc.109.068734
Gomez-Gomez, L., Bauer, Z., and Boller, T. (2001). Both the Extracellular Leucine-Rich Repeat Domain and the Kinase Activity of FLS2 Are Required for Flagellin Binding and Signaling in Arabidopsis. The Plant Cell 13, 1155–1163. doi:10.2307/3871370
González-García, M. P., Rodríguez, D., Nicolás, C., Rodríguez, P. L., Nicolás, G., and Lorenzo, O. (2003). Negative Regulation of Abscisic Acid Signaling by the Fagus Sylvatica FsPP2C1 Plays a Role in Seed Dormancy Regulation and Promotion of Seed Germination. Plant Physiol. 133, 135–144. doi:10.1104/pp.103.025569
Haider, M. S., Khan, N., Pervaiz, T., Zhongjie, L., Nasim, M., Jogaiah, S., et al. (2019). Genome-Wide Identification, Evolution, and Molecular Characterization of the PP2C Gene Family in woodland Strawberry. Gene 702, 27–35. doi:10.1016/j.gene.2019.03.025
Hao, P.-P., Wang, G.-M., Cheng, H.-Y., Ke, Y.-Q., Qi, K.-J., Gu, C., et al. (2018). Transcriptome Analysis Unravels an Ethylene Response Factor Involved in Regulating Fruit Ripening in Pear. Physiol. Plantarum 163, 124–135. doi:10.1111/ppl.12671
Hirayama, T., and Umezawa, T. (2010). The PP2C-SnRK2 Complex. Plant Signaling Behav. 5, 160–163. doi:10.4161/psb.5.2.10460
Hu, W., Yan, Y., Shi, H., Liu, J., Miao, H., Tie, W., et al. (2017). The Core Regulatory Network of the Abscisic Acid Pathway in Banana: Genome-Wide Identification and Expression Analyses during Development, Ripening, and Abiotic Stress. BMC Plant Biol. 17, 145. doi:10.1186/s12870-017-1093-4
Kerk, D., Templeton, G., and Moorhead, G. B. (2008). Evolutionary Radiation Pattern of Novel Protein Phosphatases Revealed by Analysis of Protein Data from the Completely Sequenced Genomes of Humans, Green Algae, and Higher Plants. Plant Physiol. 146, 351–367. doi:10.1104/pp.107.111393
Kerk, D., Bulgrien, J., Smith, D. W., Barsam, B., Veretnik, S., and Gribskov, M. (2002). The Complement of Protein Phosphatase Catalytic Subunits Encoded in the Genome of Arabidopsis. Plant Physiol. 129, 908–925. doi:10.1104/pp.004002
Khan, N., Ke, H., Hu, C. M., Naseri, E., Haider, M. S., Ayaz, A., et al. (2019). Genome-Wide Identification, Evolution, and Transcriptional Profiling of PP2C Gene Family in Brassica Rapa. Biomed. Res. Int. 2019, 15. doi:10.1155/2019/2965035
Kim, D., Langmead, B., and Salzberg, S. L. (2015). HISAT: A Fast Spliced Aligner with Low Memory Requirements. Nat. Methods 12, 357–360. doi:10.1038/nmeth.3317
Kim, H., Hwang, H., Hong, J.-W., Lee, Y.-N., Ahn, I. P., Yoon, I. S., et al. (2012). A rice Orthologue of the ABA Receptor, OsPYL/RCAR5, Is a Positive Regulator of the ABA Signal Transduction Pathway in Seed Germination and Early Seedling Growth. J. Exp. Bot. 63, 1013–1024. doi:10.1093/jxb/err338
Komatsu, K., Suzuki, N., Kuwamura, M., Nishikawa, Y., Nakatani, M., Ohtawa, H., et al. (2013). Group A PP2Cs Evolved in Land Plants as Key Regulators of Intrinsic Desiccation Tolerance. Nat. Commun. 4, 2219. doi:10.1038/ncomms3219
Kumar, S., Stecher, G., and Tamura, K. (2016). MEGA7: Molecular Evolutionary Genetics Analysis Version 7.0 for Bigger Datasets. Mol. Biol. Evol. 33, 1870–1874. doi:10.1093/molbev/msw054
Lee, M. W., Jelenska, J., and Greenberg, J. T. (2008). Arabidopsis Proteins Important for Modulating Defense Responses to Pseudomonas syringae that Secrete HopW1-1. Plant J. 54, 452–465. doi:10.1111/j.1365-313x.2008.03439.x
Lee, T. H., Tang, H., Wang, X., and Paterson, A. H. (2013). PGDD: A Database of Gene and Genome Duplication in Plants. Nucleic Acids Res. 41, D1152–D1158. doi:10.1093/nar/gks1104
Li, J., Yan, X., Ahmad, M., Yu, W., Song, Z., Ni, J., et al. (2021). Alternative Splicing of the Dormancy-Associated MADS-Box Transcription Factor Gene PpDAM1 Is Associated with Flower Bud Dormancy in 'Dangshansu' Pear (Pyrus Pyrifolia white Pear Group). Plant Physiol. Biochem. 166, 1096–1108. doi:10.1016/j.plaphy.2021.07.017
Li, K. Q., Xu, X. Y., and Huang, X. S. (2016). Identification of Differentially Expressed Genes Related to Dehydration Resistance in a Highly Drought-Tolerant Pear, Pyrus Betulaefolia, as through RNA-Seq. PloS one 11, e0149352. doi:10.1371/journal.pone.0149352
Li, Q., Qiao, X., Yin, H., Zhou, Y., Dong, H., Qi, K., et al. (2019). Unbiased Subgenome Evolution Following a Recent Whole-Genome Duplication in Pear (Pyrus Bretschneideri Rehd.). Hortic. Res. 6, 34. doi:10.1038/s41438-018-0110-6
Liao, Y., Smyth, G. K., and Shi, W. (2014). featureCounts: An Efficient General Purpose Program for Assigning Sequence Reads to Genomic Features. Bioinformatics 30, 923–930. doi:10.1093/bioinformatics/btt656
Liu, Z., Qanmber, G., Lu, L., Qin, W., Liu, J., Li, J., et al. (2018). Genome-Wide Analysis of BES1 Genes in Gossypium Revealed Their Evolutionary Conserved Roles in Brassinosteroid Signaling. Sci. China Life Sci. 61, 1566–1582. doi:10.1007/s11427-018-9412-x
Livak, K. J., and Schmittgen, T. D. (2001). Analysis of Relative Gene Expression Data Using Real-Time Quantitative PCR and the 2−ΔΔCT Method. Methods 25, 402–408. doi:10.1006/meth.2001.1262
Lu, L., Qanmber, G., Li, J., Pu, M., Chen, G., Li, S., et al. (2021). Identification and Characterization of the ERF Subfamily B3 Group Revealed GhERF13.12 Improves Salt Tolerance in Upland Cotton. Front. Plant Sci. 12, 705883. doi:10.3389/fpls.2021.705883
Luan, S. (1998). Protein Phosphatases and Signaling Cascades in Higher Plants. Trends Plant Sci. 3, 271–275. doi:10.1016/s1360-1385(98)01258-8
Merlot, S., Gosti, F., Guerrier, D., Vavasseur, A., and Giraudat, J. (2001). The ABI1 and ABI2 Protein Phosphatases 2C Act in a Negative Feedback Regulatory Loop of the Abscisic Acid Signalling Pathway. Plant J. 25, 295–303. doi:10.1046/j.1365-313x.2001.00965.x
Moore, R. C., and Purugganan, M. D. (2003). The Early Stages of Duplicate Gene Evolution. Proc. Natl. Acad. Sci. 100, 15682–15687. doi:10.1073/pnas.2535513100
Qanmber, G., Liu, J., Yu, D., Liu, Z., Lu, L., Mo, H., et al. (2019a). Genome-Wide Identification and Characterization of the PERK Gene Family in Gossypium Hirsutum Reveals Gene Duplication and Functional Divergence. Int. J. Mol. Sci. 20, 1750. doi:10.3390/ijms20071750
Qanmber, G., Lu, L., Liu, Z., Yu, D., Zhou, K., Huo, P., et al. (2019b). Genome-Wide Identification of GhAAI Genes Reveals that GhAAI66 Triggers a Phase Transition to Induce Early Flowering. J. Exp. Bot. 70, 4721–4736. doi:10.1093/jxb/erz239
Qiao, X., Li, M., Li, L., Yin, H., Wu, J., and Zhang, S. (2015). Genome-Wide Identification and Comparative Analysis of the Heat Shock Transcription Factor Family in Chinese white Pear (Pyrus Bretschneideri) and Five Other Rosaceae Species. BMC Plant Biol. 15, 12. doi:10.1186/s12870-014-0401-5
Qiao, X., Li, Q., Yin, H., Qi, K., Li, L., Wang, R., et al. (2019). Gene Duplication and Evolution in Recurring Polyploidization-Diploidization Cycles in Plants. Genome Biol. 20, 38–23. doi:10.1186/s13059-019-1650-2
Qiao, X., Yin, H., Li, L., Wang, R., Wu, J., Wu, J., et al. (2018). Different Modes of Gene Duplication Show Divergent Evolutionary Patterns and Contribute Differently to the Expansion of Gene Families Involved in Important Fruit Traits in Pear (Pyrus Bretschneideri). Front. Plant Sci. 9, 161. doi:10.3389/fpls.2018.00161
Reyes, D., Rodríguez, D., González-García, M. P., Lorenzo, O., Nicolás, G., García-Martínez, J. L., et al. (2006). Overexpression of a Protein Phosphatase 2C from Beech Seeds in Arabidopsis Shows Phenotypes Related to Abscisic Acid Responses and Gibberellin Biosynthesis. Plant Physiol. 141, 1414–1424. doi:10.1104/pp.106.084681
Saez, A., Apostolova, N., Gonzalez‐Guzman, M., Gonzalez‐Garcia, M. P., Nicolas, C., Lorenzo, O., et al. (2004). Gain‐of‐Function and Loss‐of‐Function Phenotypes of the Protein Phosphatase 2C HAB1 Reveal its Role as a Negative Regulator of Abscisic Acid Signalling. Plant J. 37, 354–369. doi:10.1046/j.1365-313x.2003.01966.x
Saez, A., Rodrigues, A., Santiago, J., Rubio, S., and Rodriguez, P. L. (2008). HAB1-SWI3B Interaction Reveals a Link between Abscisic Acid Signaling and Putative SWI/SNF Chromatin-Remodeling Complexes in Arabidopsis. Plant Cel. 20, 2972–2988. doi:10.1105/tpc.107.056705
Santiago, J., Rodrigues, A., Saez, A., Rubio, S., Antoni, R., Dupeux, F., et al. (2009). Modulation of Drought Resistance by the Abscisic Acid Receptor PYL5 through Inhibition of Clade A PP2Cs. Plant J. 60, 575–588. doi:10.1111/j.1365-313x.2009.03981.x
Schweighofer, A., Kazanaviciute, V., Scheikl, E., Teige, M., Doczi, R., Hirt, H., et al. (2007). The PP2C-Type Phosphatase AP2C1, Which Negatively Regulates MPK4 and MPK6, Modulates Innate Immunity, Jasmonic Acid, and Ethylene Levels in Arabidopsis. Plant Cel. 19, 2213–2224. doi:10.1105/tpc.106.049585
Servet, C., Benhamed, M., Latrasse, D., Kim, W., Delarue, M., and Zhou, D.-X. (2008). Characterization of a Phosphatase 2C Protein as an Interacting Partner of the Histone Acetyltransferase GCN5 in Arabidopsis. Biochim. Biophys. Acta (Bba) - Gene Regul. Mech. 1779, 376–382. doi:10.1016/j.bbagrm.2008.04.007
Shazadee, H., Khan, N., Wang, J., Wang, C., Zeng, J., Huang, Z., et al. (2019). Identification and Expression Profiling of Protein Phosphatases (PP2C) Gene Family in Gossypium Hirsutum L. Int. J. Mol. Sci. 20, 1395. doi:10.3390/ijms20061395
Singh, A., Giri, J., Kapoor, S., Tyagi, A. K., and Pandey, G. K. (2010). Protein Phosphatase Complement in rice: Genome-Wide Identification and Transcriptional Analysis under Abiotic Stress Conditions and Reproductive Development. BMC Genomics 11, 435. doi:10.1186/1471-2164-11-435
Singh, A., Pandey, A., Srivastava, A. K., Tran, L.-S. P., and Pandey, G. K. (2016). Plant Protein Phosphatases 2C: From Genomic Diversity to Functional Multiplicity and Importance in Stress Management. Crit. Rev. Biotechnol. 36, 1023–1035. doi:10.3109/07388551.2015.1083941
Song, S.-K., Hofhuis, H., Lee, M. M., and Clark, S. E. (2008). Key Divisions in the Early Arabidopsis Embryo Require POL and PLL1 Phosphatases to Establish the Root Stem Cell Organizer and Vascular axis. Develop. Cel 15, 98–109. doi:10.1016/j.devcel.2008.05.008
Soon, F. F., Ng, L. M., Zhou, X. E., West, G. M., Kovach, A., Tan, M. H., et al. (2012). Molecular Mimicry Regulates ABA Signaling by SnRK2 Kinases and PP2C Phosphatases. Science 335, 85–88. doi:10.1126/science.1215106
Sparkes, I. A., Runions, J., Kearns, A., and Hawes, C. (2006). Rapid, Transient Expression of Fluorescent Fusion Proteins in Tobacco Plants and Generation of Stably Transformed Plants. Nat. Protoc. 1, 2019–2025. doi:10.1038/nprot.2006.286
Starr, T. K., Jameson, S. C., and Hogquist, K. A. (2003). Positive Andnegativeselection Oft Cells. Annu. Rev. Immunol. 21, 139–176. doi:10.1146/annurev.immunol.21.120601.141107
Strizhov, N., Ábrahám, E., Ökrész, L., Blickling, S., Zilberstein, A., Schell, J., et al. (1997). Differential Expression of Two P5CS Genes Controlling Proline Accumulation during Salt‐Stress Requires ABA and Is Regulated by ABA1, ABI1 and AXR2 in Arabidopsis. Plant J. 12, 557–569. doi:10.1111/j.0960-7412.1997.00557.x
Sugimoto, H., Kondo, S., Tanaka, T., Imamura, C., Muramoto, N., Hattori, E., et al. (2014). Overexpression of a Novel Arabidopsis PP2C Isoform, AtPP2CF1, Enhances Plant Biomass Production by Increasing Inflorescence Stem Growth. J. Exp. Bot. 65, 5385–5400. doi:10.1093/jxb/eru297
Tähtiharju, S., and Palva, T. (2001). Antisense Inhibition of Protein Phosphatase 2C Accelerates Cold Acclimation in Arabidopsis thaliana. Plant J. 26, 461–470. doi:10.1046/j.1365-313x.2001.2641048.x
Umezawa, T., Sugiyama, N., Mizoguchi, M., Hayashi, S., Myouga, F., Yamaguchi-Shinozaki, K., et al. (2009). Type 2C Protein Phosphatases Directly Regulate Abscisic Acid-Activated Protein Kinases in Arabidopsis. Proc. Natl. Acad. Sci. 106, 17588–17593. doi:10.1073/pnas.0907095106
Wang, D., Zhang, Y., Zhang, Z., Zhu, J., and Yu, J. (2010). KaKs_Calculator 2.0: A Toolkit Incorporating Gamma-Series Methods and Sliding Window Strategies. Genomics, Proteomics & Bioinformatics 8, 77–80. doi:10.1016/s1672-0229(10)60008-3
Wang, G.-M., Yin, H., Qiao, X., Tan, X., Gu, C., Wang, B.-H., et al. (2016). F-box Genes: Genome-Wide Expansion, Evolution and Their Contribution to Pollen Growth in Pear (Pyrus Bretschneideri). Plant Sci. 253, 164–175. doi:10.1016/j.plantsci.2016.09.009
Wang, Y., Tang, H., DeBarry, J. D., Tan, X., Li, J., Wang, X., et al. (2012). MCScanX: A Toolkit for Detection and Evolutionary Analysis of Gene Synteny and Collinearity. Nucleic Acids Res. 40, e49. doi:10.1093/nar/gkr1293
Wei, K., and Pan, S. (2014). Maize Protein Phosphatase Gene Family: Identification and Molecular Characterization. BMC Genomics 15, 773. doi:10.1186/1471-2164-15-773
Wei, K., Wang, Y., and Xie, D. (2014). Identification and Expression Profile Analysis of the Protein Kinase Gene Superfamily in maize Development. Mol. Breed. 33, 155–172. doi:10.1007/s11032-013-9941-x
Xiang, Y., Sun, X., Gao, S., Qin, F., and Dai, M. (2017). Deletion of an Endoplasmic Reticulum Stress Response Element in a ZmPP2C-A Gene Facilitates Drought Tolerance of Maize Seedlings. Mol. Plant 10, 456–469. doi:10.1016/j.molp.2016.10.003
Xue, T., Wang, D., Zhang, S., Ehlting, J., Ni, F., Jakab, S., et al. (2008). Genome-Wide and Expression Analysis of Protein Phosphatase 2C in Rice and Arabidopsis. BMC Genomics 9, 550. doi:10.1186/1471-2164-9-550
Yang, T., and Huang, X.-S. (2018). Deep Sequencing-Based Characterization of Transcriptome of Pyrus Ussuriensis in Response to Cold Stress. Gene 661, 109–118. doi:10.1016/j.gene.2018.03.067
Yang, Z. (2007). PAML 4: Phylogenetic Analysis by Maximum Likelihood. Mol. Biol. Evol. 24, 1586–1591. doi:10.1093/molbev/msm088
Yoshida, T., Nishimura, N., Kitahata, N., Kuromori, T., Ito, T., Asami, T., et al. (2006). ABA-Hypersensitive Germination3 Encodes a Protein Phosphatase 2C (AtPP2CA) that Strongly Regulates Abscisic Acid Signaling during Germination Among Arabidopsis Protein Phosphatase 2Cs. Plant Physiol. 140, 115–126. doi:10.1104/pp.105.070128
Keywords: type 2C protein phosphatase, abscisic acid, pear (Pyrus bretschneideri), Rosaceae, abiotic stress
Citation: Wang G, Sun X, Guo Z, Joldersma D, Guo L, Qiao X, Qi K, Gu C and Zhang S (2021) Genome-wide Identification and Evolution of the PP2C Gene Family in Eight Rosaceae Species and Expression Analysis Under Stress in Pyrus bretschneideri. Front. Genet. 12:770014. doi: 10.3389/fgene.2021.770014
Received: 03 September 2021; Accepted: 11 October 2021;
Published: 11 November 2021.
Edited by:
Suxu Tan, Michigan State University, United StatesReviewed by:
Mehanathan Muthamilarasan, University of Hyderabad, IndiaGhulam Qanmber, Cotton Research Institute (CAAS), China
Copyright © 2021 Wang, Sun, Guo, Joldersma, Guo, Qiao, Qi, Gu and Zhang. This is an open-access article distributed under the terms of the Creative Commons Attribution License (CC BY). The use, distribution or reproduction in other forums is permitted, provided the original author(s) and the copyright owner(s) are credited and that the original publication in this journal is cited, in accordance with accepted academic practice. No use, distribution or reproduction is permitted which does not comply with these terms.
*Correspondence: Chao Gu, Z3VjaGFvQG5qYXUuZWR1LmNu; Shaoling Zhang, c2x6aGFuZ0BuamF1LmVkdS5jbg==
†These authors have contributed equally to this work