- 1College of Life Science, North China University of Science and Technology, Tangshan, China
- 2School of Public Health, North China University of Science and Technology, Tangshan, China
- 3School of Clinical Medicine, North China University of Science and Technology, Tangshan, China
- 4Hebei Province Key Laboratory of Occupational Health and Safety for Coal Industry, School of Public Health, North China University of Science and Technology, Tangshan, China
WNT signaling pathway inhibitor Dickkopf-1 (DKK1) is related to cancer progression; however, its diagnostic and prognostic potential have not been investigated in a pan-cancer perspective. In this study, multiple bioinformatic analyses were conducted to evaluate therapeutic value of DKK1 in human cancers. The Cancer Genome Atlas (TCGA) and the Genotype-Tissue Expression (GTEx) project served as data resources. The Wilcoxon rank test was performed to evaluate the expression difference of DKK1 between cancer tissues and normal tissues. A Kaplan-Meier curve and Cox regression were used for prognosis evaluation. Single-sample gene set enrichment analysis (ssGSEA) was used to evaluate the association of DKK1 expression with the immune cell infiltration. The potential function of DKK1 was explored by STRING and clusterProfiler. We found that the expression level of DKK1 is significantly different in different cancer types. Importantly, we demonstrated that DKK1 is an independent risk factor in ESCA, LUAD, MESO, and STAD. Further analysis revealed that DKK1 had a large effect on the immune cell infiltration and markers of certain immune cells, such as Th1 and Th2 cells. PPI network analysis and further pathway enrichment analysis indicated that DKK1 was mainly involved in the WNT signaling pathway. Our findings suggested that DKK1 might serve as a marker of prognosis for certain cancers by affecting the WNT signaling pathway and tumor immune microenvironment.
Introduction
Worldwide, malignant tumors have jeopardized public health (Siegel et al., 2021). WNT signaling plays a critical role in the progress of multiple cancer types (Bian et al., 2020; Peng et al., 2021; Sun et al., 2021). Aberrant WNT signaling may subvert cancer immunosurveillance (Spranger and Gajewski, 2015; Augustin et al., 2016; Hong et al., 2016). Dickkopf-1 (DKK1), as a WNT signaling pathway inhibitor, is involved in the development of several types of cancers (Lu et al., 2017; Zhuang et al., 2017; Igbinigie et al., 2019). DKK1 had decreased expression in both gastric cancer (GC) and colorectal cancer (CRC), but increased expression in breast cancer (BRCA) and non-small cell lung cancer (NSCLC) (Aguilera et al., 2006; Sato et al., 2007; Li et al., 2013; Jia et al., 2016; Kasoha et al., 2018). In esophageal cancer (ESCA), DKK1 promoted cell proliferation through the cytoskeleton-associated protein 4 (CKAP4)-related pathway (Shinno et al., 2018). DKK1 was also involved in the invasion and metastasis of intrahepatic cholangiocarcinoma (ICC) cells and lymph node metastasis (Shi et al., 2013). Various studies also demonstrated the effect of DKK1 on the prognosis of certain cancers, such as head and neck squamous carcinoma (HNSC), NSCLC, and pancreatic adenocarcinoma (PAAD) (Yamabuki et al., 2007; Han et al., 2015; Gao et al., 2018). In liver hepatocellular carcinoma (LIHC), DKK1 could be induced by an active WNT/β-catenin signal and further contributed to patient’s poor prognosis (Yu et al., 2009). Some reports also presented that DKK1 might serve as a target for immunotherapy (Qian et al., 2012; Betella et al., 2020). DKK1 could affect the function of immune cells, such as T lymphocytes and bone marrow-derived suppressor cells (Katoh and Katoh, 2017). By activating CD4+ and CD8+ T lymphocytes, DKK1 could eliminate myeloma cells in mouse models (Qian et al., 2012). DKK1 also inhibited the secretion of IFN-γ in Th1 cells and induced the production of interleukin (IL)-4, IL-5, IL-10, and IL-13 in Th2 cells (Bais et al., 2005). The inflammation caused by tumor-specific Th1 cells could prevent cancer, but Th2 cells have the opposite function (Kennedy and Celis, 2008; Lefrancois et al., 2020). By inhibiting β-catenin to prevent clearance by natural killer (NK) cells, DKK1 helps to sustain the stem cell-like properties of cancer cells (Malladi et al., 2016). Based on these findings, it is necessary to evaluate the role of DKK1 in the cancer immune microenvironment.
For several years, numerous studies have been conducted to explore the role of DKK1 in various cancers and revealed the different roles of DKK1 in different cancer types. In this study, we evaluated the pan-cancer expression of DKK1 using The Cancer Genome Atlas (TCGA) dataset. Subsequently, we investigated the association of DKK1 expression with the survival time of patients with different cancers. Finally, we analyzed the effect of DKK1 expression on immune cell infiltration and immune cell markers. The overall process of this research is shown in Figure 1. Our findings deepened our understanding of the roles of DKK1 in cancer progression and prognosis.
Materials and Methods
Pan-Cancer DKK1 Expression Profile Analysis
The Genotype-Tissue Expression (GTEx) project and RNA-seq datasets from The Cancer Genome Atlas (TCGA) were downloaded from UCSC Xena (https://xena.ucsc.edu/) and used for pan-cancer analysis of DKK1. TOIL was used to reprocess the raw RNA-seq data from GTEx and TCGA databases to correct batch effects and allow for data merging across GTEx and TCGA datasets (Vivian et al., 2017). Expression differences of DKK1 were examined using the Wilcoxon rank test with the threshold of |log2 FC| >1 and p-value < 0.05.
Survival Analysis
Patients with different types of cancer were segregated into high and low expression groups by the median of the expression level of DKK1. Kaplan-Meier (KM) survival analysis was conducted by R survival and survMiner packages. Cox regression analysis was used to evaluate the relationship between DKK1 expression and overall survival (OS) based on TCGA data. Univariate Cox analysis was performed to select relevant variables, and a multivariate Cox model was used to evaluate the independent prognostic factors. Differences were considered significant when p-values were less than 0.05. All analyses were carried out using R language (version 3.6.3).
Correlations Between DKK1 Expression and Infiltration Immune Cells
Single-sample gene set enrichment analysis (ssGSEA) was used to assess the immune cell infiltration signatures of each individual with LUAD according to the expression level of DKK1 by using the R GSVA package (Hänzelmann et al., 2013). The gene set for immune cell markers was retrieved from the Laboratory of Integrative Cancer Immunology (LICI) (Bindea et al., 2013).
Correlation Between DKK1 Expression and Immune Cell Markers
The correlation module in TIMER (http://timer.cistrome.org/) was used to analyze the correlation between the expression of DKK1 and diverse immune cell markers. The list of different immune factors was obtained from the tumor immune system interaction database (Ru et al., 2019).
PPI Network Construction and Functional Enrichment Analysis
The protein-protein interaction network (PPI network) of DKK1 was constructed using the Search Tool for the Retrieval of Interacting Genes/Proteins (STRING, http://string-db.org) (von Mering et al., 2003) with a minimum required interaction score > 0.7. To identify the hub genes of DKK1 PPI, the maximal clique centrality (MCC) algorithm was analyzed by the Cytohubba (Chin et al., 2014) plugin based on Cytoscape (Shannon et al., 2003). To evaluate the biological functions that DKK1 is involved in, Gene Ontology (GO, http://geneontology.org/) enrichment and Kyoto Encyclopedia of Genes and Genomes (KEGG, http://www.kegg.jp/) pathway analyses were performed using the R package clusterProfiler (Yu et al., 2012).
Results
DKK1 Expression Difference in Pan-Cancer
In this study, expression difference analyses of DKK1 were performed between cancer tissues and adjacent normal tissues. DKK1 mRNA expression in cancer tissues from the TCGA database was inconsistent with that in GTEx and TCGA normal tissues (Figures 2A,B). DKK1 expression was significantly higher in cancer tissues with cholangiocarcinoma (CHOL), ESCA, HNSC, LIHC, lung squamous cell carcinoma (LUSC), and STAD than that in their respective adjacent normal tissues. However, DKK1 expression was significantly decreased in bladder urothelial carcinoma (BLCA), kidney chromophobe (KICH), kidney renal papillary cell carcinoma (KIRP), and prostate adenocarcinoma (PRAD).
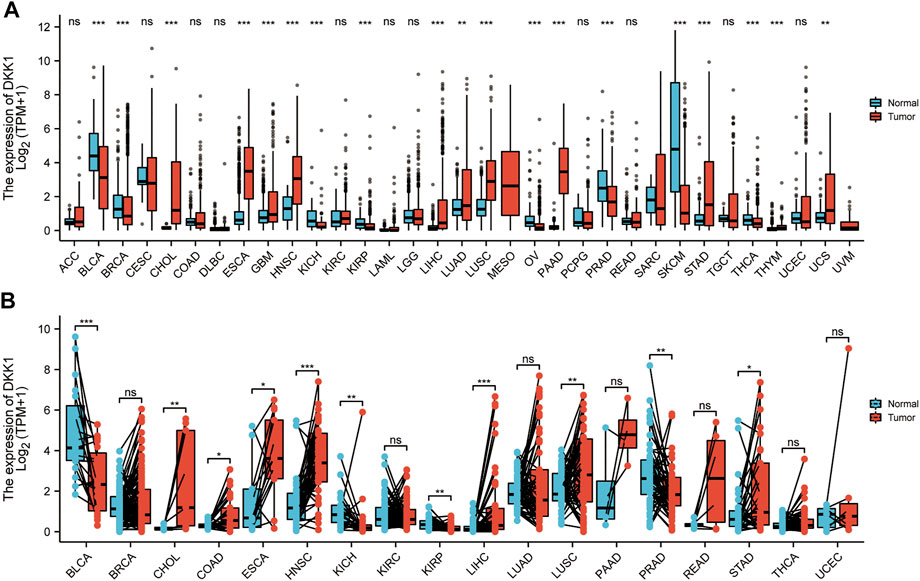
FIGURE 2. Pan-cancer analysis of differential expression of DKK1. The abundance is measured by log-normalized transcripts per million (TPM). (A) Differential expression analysis between unpaired cancer tissues and adjacent normal tissues. (B). Differential expression analysis between paired cancer tissues and adjacent normal tissues.
DKK1 Expression Correlates With Cancer Prognosis and Clinical Stages
To analyze the association of DKK1 expression with clinical outcomes across all TCGA cancer types. TCGA pan-cancer analyses showed that higher DKK1 level was significantly associated with the poor prognosis of adrenocortical carcinoma (ACC) (p < 0.01), HNSC (p < 0.01), LAML (p = 0.046), lung adenocarcinoma (LUAD) (p < 0.01), mesothelioma (MESO) (p < 0.01), PAAD (p < 0.01), and STAD (p < 0.01) (Figures 3A–G), and lower DKK1 expression was significantly associated with the poor prognosis of ESCA (p = 0.016) and kidney renal clear cell carcinoma (KIRC) (p = 0.024) (Figures 3H,I). In addition, the expression of DKK1 was closely related to the clinical stages of several cancer types, including ACC, KIRC, and PAAD (Figures 4A–C). These results indicated that DKK1 was a potential oncogene in many types of cancer.
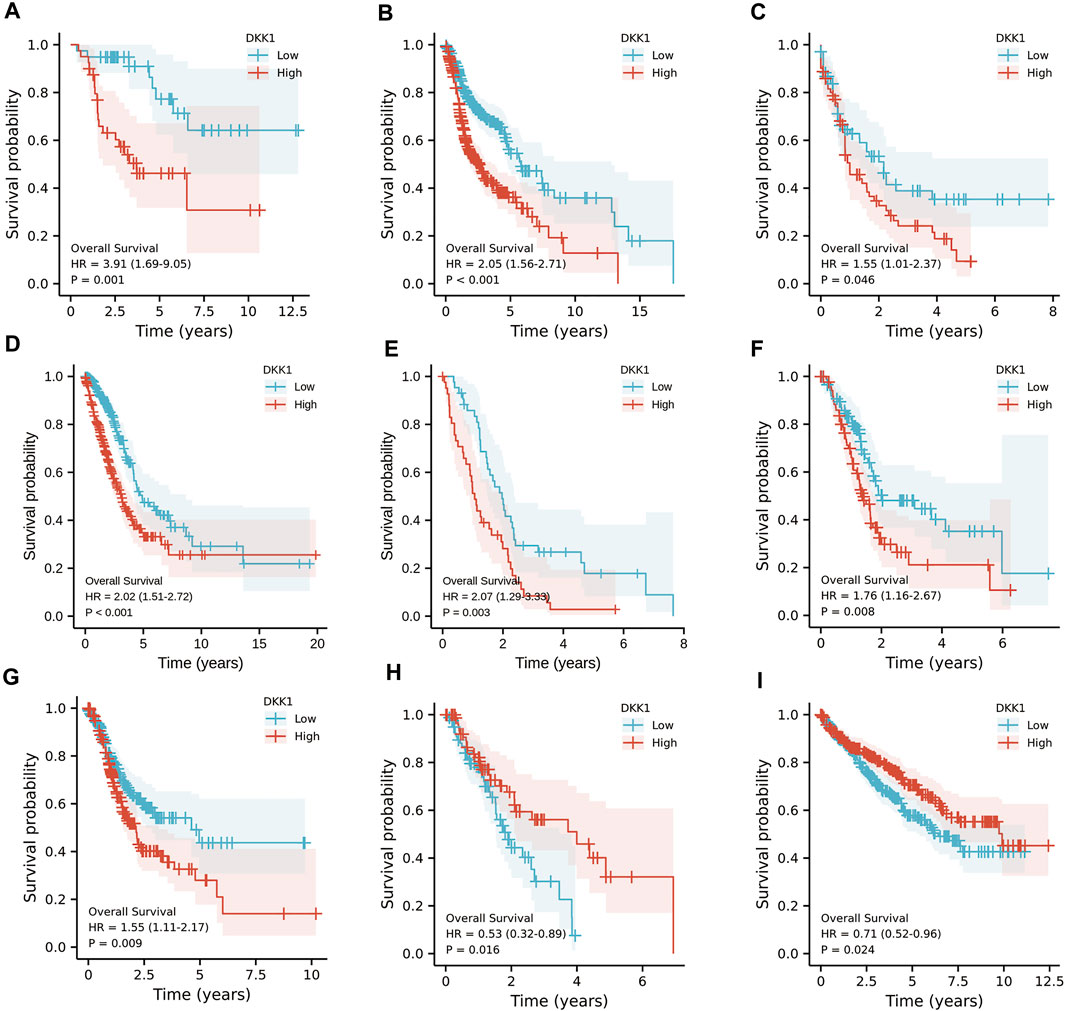
FIGURE 3. Kaplan-Meier analysis of DKK1 expression in different cancer types. (A–G) Higher DKK1 expression was correlated with the poor prognosis of ACC, HNSC, LAML, LUAD, MESO, PAAD, and STAD. (H–I) Lower DKK1 expression was correlated with the poor prognosis of ESCA and KIRC.
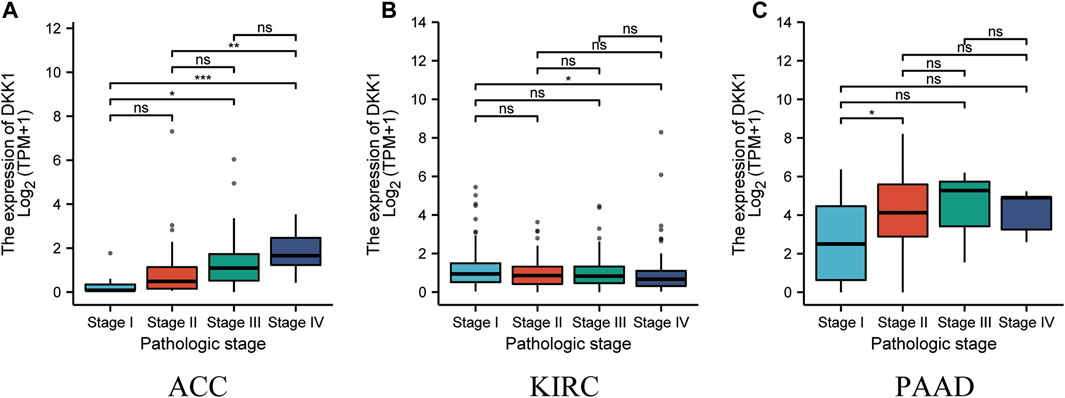
FIGURE 4. Association of DKK1 expression with tumor stages. (A–C) DKK1 expression in different stages of ACC, KIRC, and PAAD. Data shown as mean ± SD. *p < 0.05, **p < 0.01, ***p < 0.001.
DKK1 Expression as an Independent Prognostic Factor
Differential DKK1 expression was associated with poor overall survival (OS) in several types of cancer (p < 0.05; Figure 3). To explore possible correlations of DKK1 expression with clinical factors, several potential survival-related variables, including TNM stages, gender, age, smoking status, and DKK1 expression, were entered into a multivariate Cox model. The results suggested that DKK1 expression level was an independent protective factor for prognosis of ESCA patients [hazard ratio (HR) = 0.53, 95% confidence interval (CI) = 0.28–0.99, p < 0.05; Table 1), LUAD (HR = 1.95, 95% CI = 1.40–2.72, p < 0.01; Table 2), MESO (HR = 2.07, 95% CI = 1.29–3.33, p < 0.01; Table 3), and STAD (HR = 1.70, 95% CI = 1.19–2.44, p < 0.01; Table 4). Taken together, these results demonstrated that differential DKK1 expression had a non-directional effect on the progression and prognosis of certain cancers.
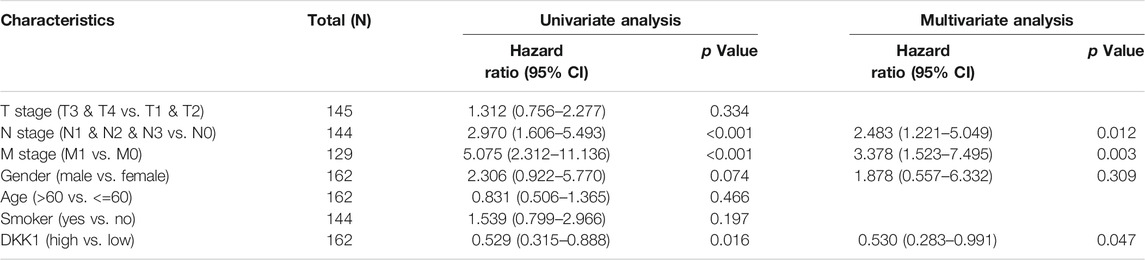
TABLE 1. Univariate and multivariate Cox analyses of DKK1 expression with overall survival (OS) among esophageal carcinoma (ESCA) patients.
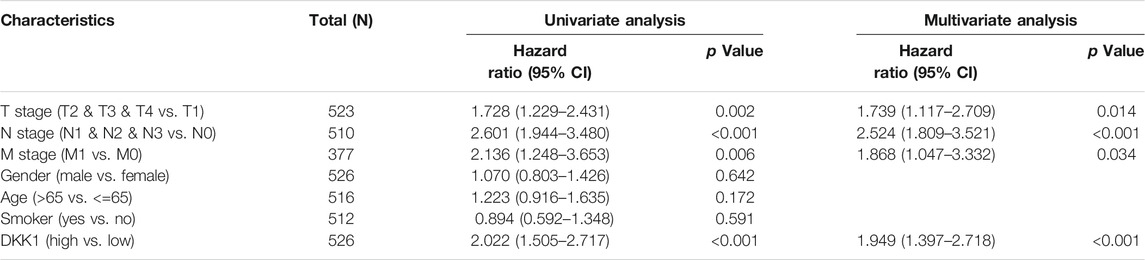
TABLE 2. Univariate and multivariate Cox analyses of DKK1 expression with overall survival (OS) among lung adenocarcinoma (LUAD) patients.
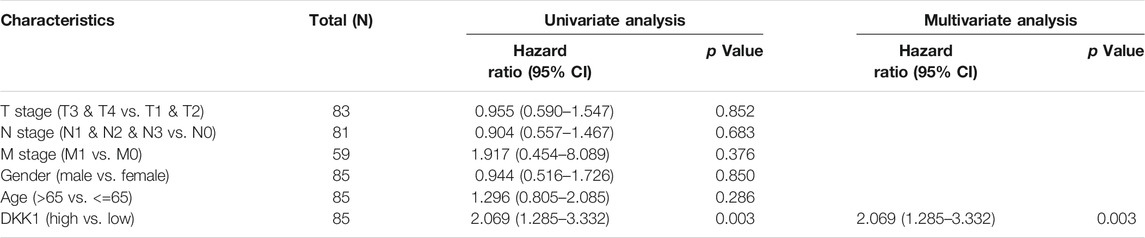
TABLE 3. Univariate and multivariate Cox analyses of DKK1 expression with overall survival (OS) among mesothelioma (MESO) patients.
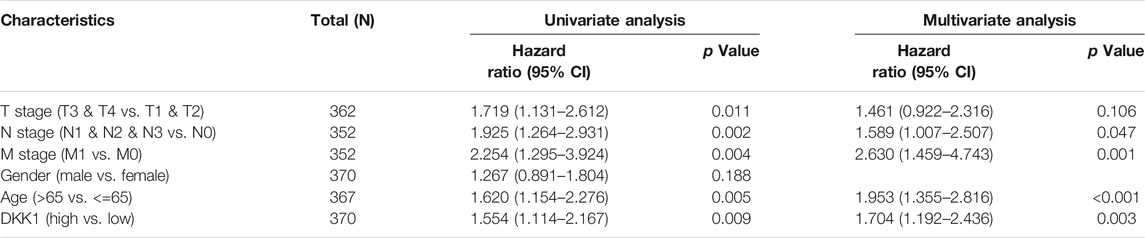
TABLE 4. Univariate and multivariate Cox analyses of DKK1 expression with overall survival (OS) among stomach adenocarcinoma (STAD) patients.
Association Between DKK1 Expression and Immune Responses in Cancer
To further validate the role of DKK1 as a potential immune influencer, the relationship between DKK1 expression and immune cell infiltration was estimated. It turned out that DKK1 was strongly correlated with the immune cell infiltration in many types of cancer (|r| > 0.4, p < 0.05). The infiltration level of Th2 cells was positively correlated with the expression of DKK1 in ACC, KICH, MESO, and PAAD (Figures 5A–D). DKK1 level was also correlated with immune cell infiltration of macrophages (GBM), neutrophils (PRAD), Th1 cells (SARC), NK cells (TGCT), Th1 cells (THYM), and Tgd (UVM) (Supplementary Figures S1A–F).
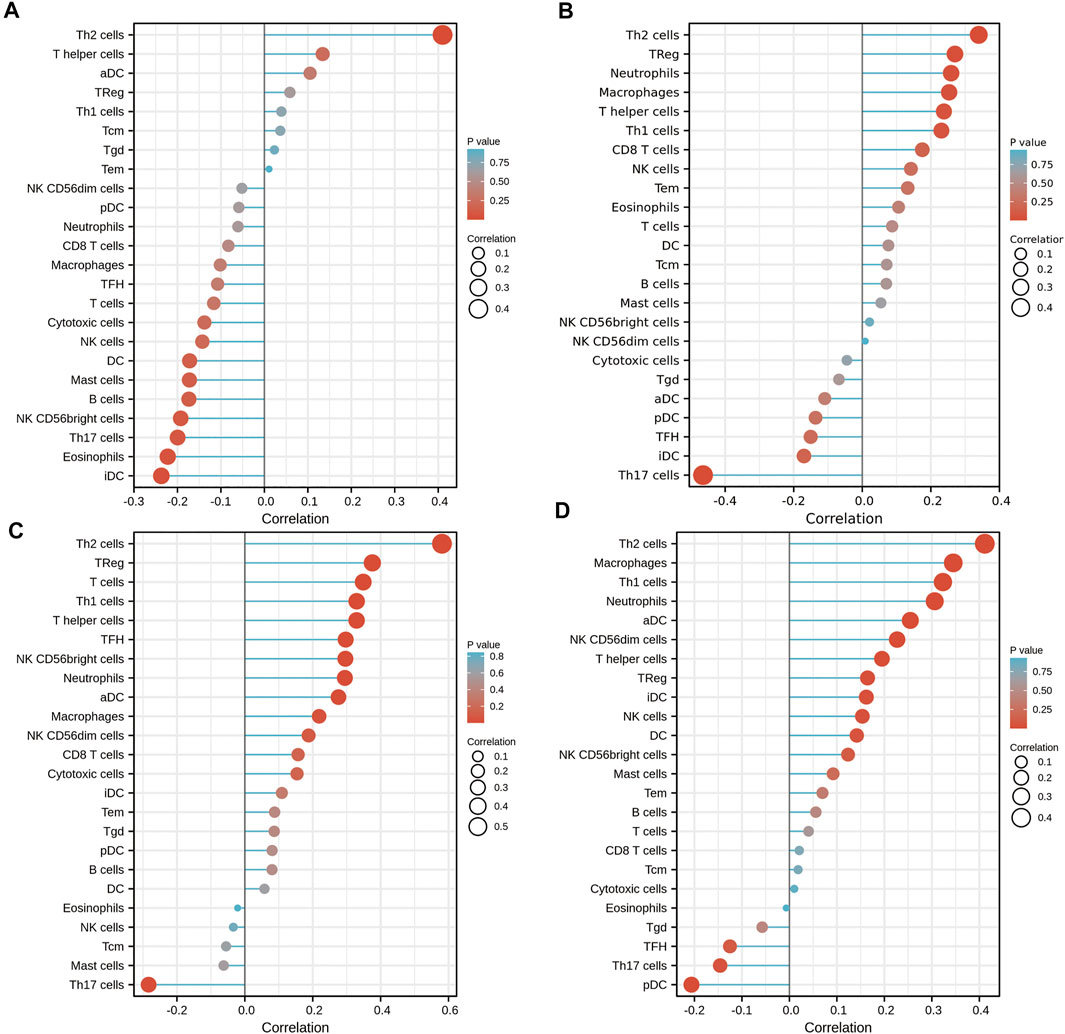
FIGURE 5. Effects of DKK1 expression on immune cell infiltration status. (A) ACC; (B) KICH; (C) MESO; and (D) PAAD.
Using the data from the TIMER database, we evaluated the correlation between DKK1 and immune infiltrating cells. Recognizable immune cell markers included B cells, T cells (general), CD8+ T cells, T cells with different functions, M1 and M2 macrophages, TAMs, monocytes, NK cells, neutrophils, and dendritic cells. More directly, our findings provided evidence that the expression of DKK1 was correlated with the level of Th1 markers (STAT1 and IFNG) and Th2 markers (GATA3 and STAT6) in various cancers, such as ACC, KICH, MESO, and PAAD (Figure 6; Supplementary Table S1). In addition, DKK1 was also correlated with the level of other immune cell markers, such as GBM (macrophage markers), PRAD (neutrophils markers), and TGCT (NK cell markers) (Supplementary Table S2). These results suggested that DKK1 might have a large impact on the tumor immune microenvironment.
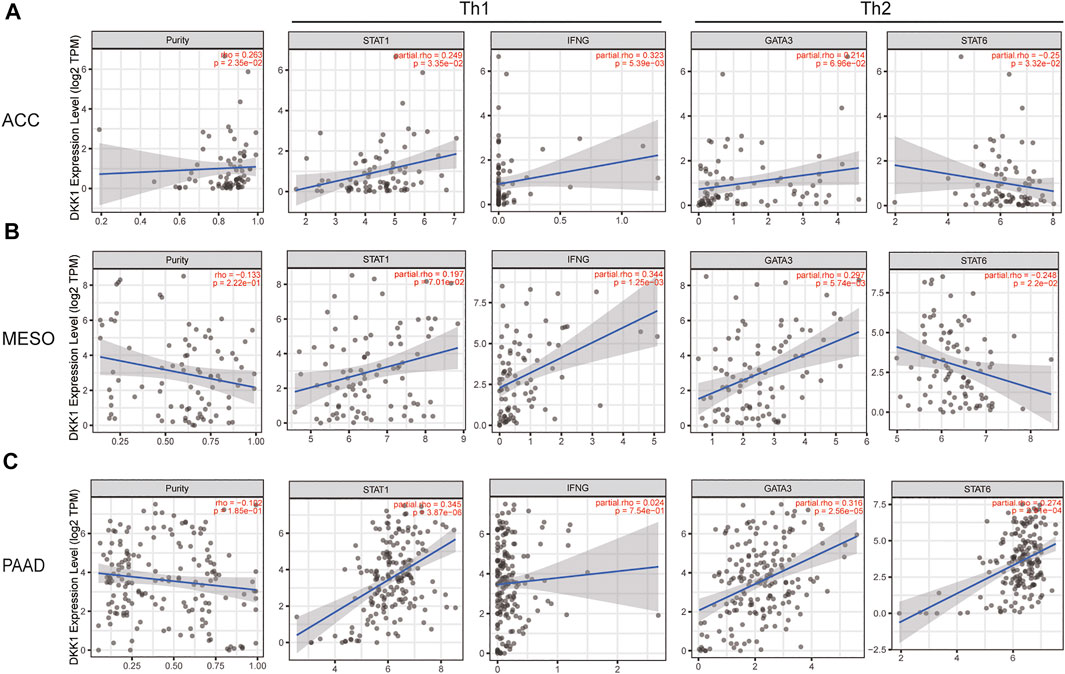
FIGURE 6. The integrated network of DKK1. (A) Protein-protein interaction network of DKK1; (B) gene-concept network for KEGG pathway analysis; and (C) gene-concept network for GO terms analysis.
Protein–Protein Interaction Network, Gene Ontology, and KEGG Pathway Analyses
A PPI network of DKK1 included 46 nodes and 506 edges. The hub genes were screened out using the Cytoscape app cytoHubba plugin. The top five hub genes were DKK1, WNT1, WNT2, WNT3A, and WNT5A (Figure 7A), which indicated that DKK1 might be involved in the functional regulation of WNT family genes. To further clarify the biological functions of DKK1, KEGG pathway and GO terms analyses were performed. KEGG pathway analysis showed that genes in the DKK1 PPI were mainly enriched in the WNT signaling pathway, basal cell carcinoma, breast cancer, gastric cancer, and hepatocellular carcinoma pathways (Figure 7B). GO terms were mainly concentrated in regulation of the WNT signaling pathway (biological process, BP), WNT signalosome (cellular component, CC), and frizzled binding (molecular function, MF) (Figure 7C). These results implied that genes in the DKK1 PPI might work together to participate in cancer progression by the WNT signaling pathway.
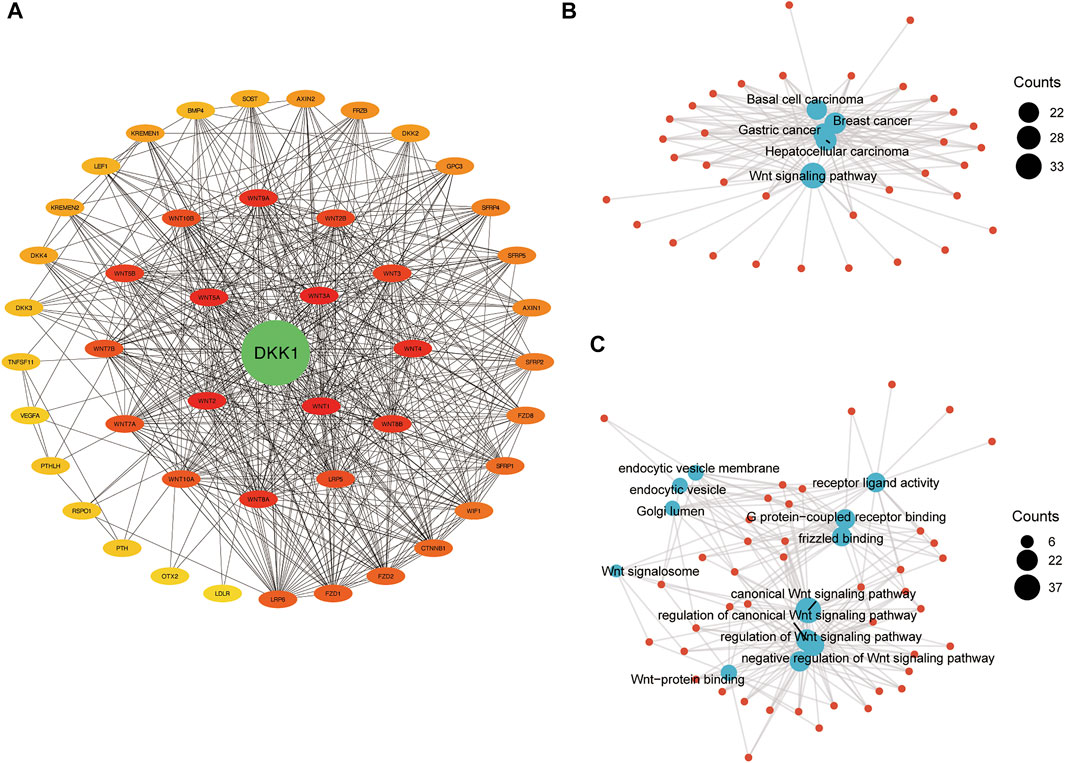
FIGURE 7. DKK1 expression is related to the changes in Th1 and Th2 cells of ACC, LICH, MESO, and PAAD. The markers include STAT1 and IFNG of Th1 cells; GATA3 and STAT6 of Th2. Scatter plot of DKK1 expression in ACC (A) (n = 79), KICH (B) (n = 66), MESO (C) (n = 87), and PAAD (D) (n = 179) correlated with Th1 and Th2 cell gene markers.
Discussion
DKK1 is a well-established crucial participant in the WNT signal pathway and also acts as a major target in drug design. DKN-01, an anti-DKK1 mAb, could block the immunosuppressive effects of DKK1 in the tumor microenvironment (TME) (Haas et al., 2021) and also perform potential antiangiogenic and immunomodulatory activity in combination therapy with gemcitabine/cisplatin in advanced biliary tract cancer (Goyal et al., 2020). Other clinical trials using anti-DKK1 mAb, such as BHQ880 (Fulciniti et al., 2009) and PF-04840082 (Betts et al., 2010) were also carried out to treat certain cancers.
The differential expression of DKK1 has been reported in many different cancers; however, there is a lack of comprehensive pan-cancer analysis of DKK1. In the current study, after analyzing the expression level of DKK1 in cancer and normal tissues of 33 cancer types, we found that DKK1 was upregulated in CHOL, ESCA, HNSC, LIHC, LUSC, and STAD, but downregulated in BLCA, KICH, KIRP, and PRAD. Similarly, several studies demonstrated that DKK1 was differentially expressed in a variety of cancers and affected cancer progression by changing cancer proliferation and invasion capabilities (Shi et al., 2013; Zhuang et al., 2017; Fezza et al., 2019). Our prognostic analysis showed that in most cancers (ACC, HNSC, LAML, LUAD, MESO, PAAD, and STAD), the upregulated expression of DKK1 was associated with poor prognosis. This further supported the results from previous studies which also confirmed the relationship between the overexpression of DKK1 and the lower overall survival in HNSC (Gao et al., 2018), NSCLC (Yamabuki et al., 2007), and PAAD (Han et al., 2015). However, in ESCA and KIRC, our data showed that a lower DKK1 level contributed to poor prognosis. Other pan-cancer analysis also provided evidence that the same gene could result in a controversial consequence in different kinds of cancer. For example, a pan-cancer study showed that there was a significant different expression of NLRP3 in 15 different cancers, and it was used as an independent posterior factor of SKCM (Ju et al., 2021). Similarly, when comparing the expression level of Fam20C in cancer tissues with that in neighboring normal tissues, we found a large variation across different kinds of cancers which indicated the different roles in different cancers (Liu et al., 2021).
The effect of DKK1 on various cancers may be the result of abnormal activation of WNT signaling (Niida et al., 2004). Our PPI analysis and the pathway enrichment analysis showed that the genes interacting with DKK1 were mainly involved in the WNT signaling pathway, basal cell carcinoma, breast cancer, gastric cancer, and liver cancer. DKK1 has been reported to inhibit the interaction of LRP 5/6 with a WNT signal and the formation of the Fzd-WNT-LRP5/6 complex (Wirths et al., 2003; Ahn et al., 2011). By interfering with DKK1, the activated WNT3a/β-catenin signal had a great influence on cell proliferation, cell cycle acceleration, invasion, and migration (Ren et al., 2021). These results suggested that DKK1 may participate in cancer progression through the WNT signaling pathway.
To unravel the potential mechanism of the predictive value of DKK1 alterations for the tumor immune microenvironment, types of infiltrating immune cells were surveyed. Chronic inflammation is a well-acknowledged risk factor of cancers, we hypothesized that DKK1 influenced cancer prognosis through immune cell infiltration. After conducting immune infiltration analysis, we found that the expression DKK1 was correlated with certain immune cell markers on Th1 and Th2 cells. Wang’s study showed that Th2 cells were identified as prognostic immune cells in gastric cancer (Wang et al., 2020). Th2 cells also demonstrated therapeutic potential for adoptive cell therapy (ACT) (Lorvik et al., 2016). Recent studies demonstrated that Th2 responses played a critical role in the pathogenesis of cancers, such as luminal breast cancer (Zhang et al., 2015), prostate and advanced melanoma cancer (Dulos et al., 2012), and myeloma (Tian et al., 2019). The deep understanding of the relationship between DKK1 and Th2 responses will help us comprehend the mechanism of local antitumor response. In recent years, with the development of immune checkpoint inhibitors, infiltrating immune cell markers can not only be used as prognostic markers, but also have received extensive attention as a new type of treatment (Ladanyi, 2015).
Taken together, our study annotated DKK1 expression in a pan-cancer manner and identified that DKK1 could be used as an independent prognosis factor. DKK1 was significantly expressed in various cancers, and it might also be a biomarker for tumor immunity or even targeted therapy. This study also provided evidence of the effect of DKK1 on immune cell infiltration. However, this study has its limitations. Since all analyses were based on online datasets, experimental confirmation from a laboratory is still needed.
Data Availability Statement
Publicly available datasets were analyzed in this study. This data can be found here: https://xena.ucsc.edu/
Author Contributions
HZ conceived and designed the research. SG analyzed the data. SG and YJ wrote the original draft. HZ edited and formed the final version. All authors have read and approved the final version of the article.
Funding
This research was funded by the Basic Scientific Research Funds for Provincial Universities of North China University of Science and Technology (JQN2019013) and Postgraduate Innovation Funding Project of Hebei Province (CXZZBS2021105).
Conflict of Interest
The authors declare that the research was conducted in the absence of any commercial or financial relationships that could be construed as a potential conflict of interest.
Publisher’s Note
All claims expressed in this article are solely those of the authors and do not necessarily represent those of their affiliated organizations, or those of the publisher, the editors, and the reviewers. Any product that may be evaluated in this article, or claim that may be made by its manufacturer, is not guaranteed or endorsed by the publisher.
Supplementary Material
The Supplementary Material for this article can be found online at: https://www.frontiersin.org/articles/10.3389/fgene.2021.757897/full#supplementary-material
References
Aguilera, O., Fraga, M. F., Ballestar, E., Paz, M. F., Herranz, M., Espada, J., et al. (2006). Epigenetic Inactivation of the Wnt Antagonist DICKKOPF-1 (DKK-1) Gene in Human Colorectal Cancer. Oncogene 25 (29), 4116–4121. doi:10.1038/sj.onc.1209439
Ahn, V. E., Chu, M. L.-H., Choi, H.-J., Tran, D., Abo, A., and Weis, W. I. (2011). Structural Basis of Wnt Signaling Inhibition by Dickkopf Binding to LRP5/6. Developmental Cel 21 (5), 862–873. doi:10.1016/j.devcel.2011.09.003
Augustin, I., Dewi, D. L., Hundshammer, J., Rempel, E., Brunk, F., and Boutros, M. (2016). Immune Cell Recruitment in Teratomas Is Impaired by Increased Wnt Secretion. Stem Cel Res. 17 (3), 607–615. doi:10.1016/j.scr.2016.10.010
Bais, A. G., Beckmann, I., Lindemans, J., Ewing, P. C., Meijer, C. J., Snijders, P. J., et al. (2005). A Shift to a Peripheral Th2-type Cytokine Pattern during the Carcinogenesis of Cervical Cancer Becomes Manifest in CIN III Lesions. J. Clin. Pathol. 58 (10), 1096–1100. doi:10.1136/jcp.2004.025072
Betella, I., Turbitt, W. J., Szul, T., Wu, B., Martinez, A., Katre, A., et al. (2020). Wnt Signaling Modulator DKK1 as an Immunotherapeutic Target in Ovarian Cancer. Gynecol. Oncol. 157 (3), 765–774. doi:10.1016/j.ygyno.2020.03.010
Betts, A. M., Clark, T. H., Yang, J., Treadway, J. L., Li, M., Giovanelli, M. A., et al. (2010). The Application of Target Information and Preclinical Pharmacokinetic/pharmacodynamic Modeling in Predicting Clinical Doses of a Dickkopf-1 Antibody for Osteoporosis. J. Pharmacol. Exp. Ther. 333 (1), 2–13. doi:10.1124/jpet.109.164129
Bian, J., Dannappel, M., Wan, C., and Firestein, R. (2020). Transcriptional Regulation of Wnt/β-Catenin Pathway in Colorectal Cancer. Cells 9 (9), 2125. doi:10.3390/cells9092125
Bindea, G., Mlecnik, B., Tosolini, M., Kirilovsky, A., Waldner, M., Obenauf, A. C., et al. (2013). Spatiotemporal Dynamics of Intratumoral Immune Cells Reveal the Immune Landscape in Human Cancer. Immunity 39 (4), 782–795. doi:10.1016/j.immuni.2013.10.003
Chin, C.-H., Chen, S.-H., Wu, H.-H., Ho, C.-W., Ko, M.-T., and Lin, C.-Y. (2014). cytoHubba: Identifying Hub Objects and Sub-networks from Complex Interactome. BMC Syst. Biol. 8 (Suppl. 4), S11. doi:10.1186/1752-0509-8-s4-s11
Dulos, J., Carven, G. J., van Boxtel, S. J., Evers, S., Driessen-Engels, L. J. A., Hobo, W., et al. (2012). PD-1 Blockade Augments Th1 and Th17 and Suppresses Th2 Responses in Peripheral Blood from Patients with Prostate and Advanced Melanoma Cancer. J. Immunother. 35 (2), 169–178. doi:10.1097/CJI.0b013e318247a4e7
Fezza, M., Moussa, M., Aoun, R., Haber, R., and Hilal, G. (2019). DKK1 Promotes Hepatocellular Carcinoma Inflammation, Migration and Invasion: Implication of TGF-Β1. PLoS One 14 (9), e0223252. doi:10.1371/journal.pone.0223252
Fulciniti, M., Tassone, P., Hideshima, T., Vallet, S., Nanjappa, P., Ettenberg, S. A., et al. (2009). Anti-DKK1 mAb (BHQ880) as a Potential Therapeutic Agent for Multiple Myeloma. Blood 114 (2), 371–379. doi:10.1182/blood-2008-11-191577
Gao, H., Li, L., Xiao, M., Guo, Y., Shen, Y., Cheng, L., et al. (2018). Elevated DKK1 Expression Is an Independent Unfavorable Prognostic Indicator of Survival in Head and Neck Squamous Cell Carcinoma. Cmar Vol. 10, 5083–5089. doi:10.2147/CMAR.S177043
Goyal, L., Sirard, C., Schrag, M., Kagey, M. H., Eads, J. R., Stein, S., et al. (2020). Phase I and Biomarker Study of the Wnt Pathway Modulator DKN-01 in Combination with Gemcitabine/Cisplatin in Advanced Biliary Tract Cancer. Clin. Cancer Res. 26 (23), 6158–6167. doi:10.1158/1078-0432.Ccr-20-1310
Haas, M. S., Kagey, M. H., Heath, H., Schuerpf, F., Rottman, J. B., and Newman, W. (2021). mDKN-01, a Novel Anti-DKK1 mAb, Enhances Innate Immune Responses in the Tumor Microenvironment. Mol. Cancer Res. 19 (4), 717–725. doi:10.1158/1541-7786.Mcr-20-0799
Han, S.-x., Zhou, X., Sui, X., He, C.-c., Cai, M.-j., Ma, J.-l., et al. (2015). Serum Dickkopf-1 Is a Novel Serological Biomarker for the Diagnosis and Prognosis of Pancreatic Cancer. Oncotarget 6 (23), 19907–19917. doi:10.18632/oncotarget.4529
Hänzelmann, S., Castelo, R., and Guinney, J. (2013). GSVA: Gene Set Variation Analysis for Microarray and RNA-Seq Data. BMC Bioinformatics 14, 7. doi:10.1186/1471-2105-14-7
Hong, Y., Manoharan, I., Suryawanshi, A., Shanmugam, A., Swafford, D., Ahmad, S., et al. (2016). Deletion of LRP5 and LRP6 in Dendritic Cells Enhances Antitumor Immunity. Oncoimmunology 5 (4), e1115941. doi:10.1080/2162402x.2015.1115941
Igbinigie, E., Guo, F., Jiang, S.-W., Kelley, C., and Li, J. (2019). Dkk1 Involvement and its Potential as a Biomarker in Pancreatic Ductal Adenocarcinoma. Clinica Chim. Acta 488, 226–234. doi:10.1016/j.cca.2018.11.023
Jia, X., Li, N., Peng, C., Deng, Y., Wang, J., Deng, M., et al. (2016). miR-493 Mediated DKK1 Down-Regulation Confers Proliferation, Invasion and Chemo-Resistance in Gastric Cancer Cells. Oncotarget 7 (6), 7044–7054. doi:10.18632/oncotarget.6951
Ju, M., Bi, J., Wei, Q., Jiang, L., Guan, Q., Zhang, M., et al. (2021). Pan-cancer Analysis of NLRP3 Inflammasome with Potential Implications in Prognosis and Immunotherapy in Human Cancer. Brief Bioinform 22 (4). doi:10.1093/bib/bbaa345
Kasoha, M., Bohle, R. M., Seibold, A., Gerlinger, C., Juhasz-Böss, I., and Solomayer, E.-F. (2018). Dickkopf-1 (Dkk1) Protein Expression in Breast Cancer with Special Reference to Bone Metastases. Clin. Exp. Metastasis 35 (8), 763–775. doi:10.1007/s10585-018-9937-3
Katoh, M., and Katoh, M. (2017). Molecular Genetics and Targeted Therapy of WNT-Related Human Diseases (Review). Int. J. Mol. Med. 40 (3), 587–606. doi:10.3892/ijmm.2017.3071
Kennedy, R., and Celis, E. (2008). Multiple Roles for CD4+T Cells in Anti-tumor Immune Responses. Immunol. Rev. 222, 129–144. doi:10.1111/j.1600-065X.2008.00616.x
Ladányi, A. (2015). Prognostic and Predictive Significance of Immune Cells Infiltrating Cutaneous Melanoma. Pigment Cel Melanoma Res 28 (5), 490–500. doi:10.1111/pcmr.12371
Lefrançois, P., Xie, P., Gunn, S., Gantchev, J., Villarreal, A. M., Sasseville, D., et al. (2020). In Silico analyses of the Tumor Microenvironment Highlight Tumoral Inflammation, a Th2 Cytokine Shift and a Mesenchymal Stem Cell-like Phenotype in Advanced in Basal Cell Carcinomas. J. Cel Commun. Signal. 14 (2), 245–254. doi:10.1007/s12079-020-00563-6
Li, S., Qin, X., Guo, X., Cui, A., He, Y., Wei, S., et al. (2013). Dickkopf-1 Is Oncogenic and Involved in Invasive Growth in Non Small Cell Lung Cancer. PLoS One 8 (12), e84944. doi:10.1371/journal.pone.0084944
Liu, X., Zhan, Y., Xu, W., Liu, X., Geng, Y., Liu, L., et al. (2021). Prognostic and Immunological Role of Fam20C in Pan-Cancer. Biosci. Rep. 41 (1). doi:10.1042/BSR20201920
Lorvik, K. B., Hammarström, C., Fauskanger, M., Haabeth, O. A. W., Zangani, M., Haraldsen, G., et al. (2016). Adoptive Transfer of Tumor-specific Th2 Cells Eradicates Tumors by Triggering an In Situ Inflammatory Immune Response. Cancer Res. 76 (23), 6864–6876. doi:10.1158/0008-5472.Can-16-1219
Lu, Z., Zhou, C., Hu, J., Xiong, L., Cong, Z., and Shen, Y. (2017). DKK1 Maintained Cancer Stem-like Properties of Esophageal Carcinoma Cells via ALDH1A1/SOX2 axis. Int. J. Clin. Exp. Pathol. 10 (9), 9489–9495.
Malladi, S., Macalinao, D. G., Jin, X., He, L., Basnet, H., Zou, Y., et al. (2016). Metastatic Latency and Immune Evasion through Autocrine Inhibition of WNT. Cell 165 (1), 45–60. doi:10.1016/j.cell.2016.02.025
Mering, C. v., Huynen, M., Jaeggi, D., Schmidt, S., Bork, P., and Snel, B. (2003). STRING: a Database of Predicted Functional Associations between Proteins. Nucleic Acids Res. 31 (1), 258–261. doi:10.1093/nar/gkg034
Niida, A., Hiroko, T., Kasai, M., Furukawa, Y., Nakamura, Y., Suzuki, Y., et al. (2004). DKK1, a Negative Regulator of Wnt Signaling, Is a Target of the β-catenin/TCF Pathway. Oncogene 23 (52), 8520–8526. doi:10.1038/sj.onc.1207892
Peng, Y., Zhang, X., Lin, H., Deng, S., Qin, Y., He, J., et al. (2021). Dual Activation of Hedgehog and Wnt/β-Catenin Signaling Pathway Caused by Downregulation of SUFU Targeted by miRNA-150 in Human Gastric Cancer. Aging 13 (7), 10749–10769. doi:10.18632/aging.202895
Qian, J., Zheng, Y., Zheng, C., Wang, L., Qin, H., Hong, S., et al. (2012). Active Vaccination with Dickkopf-1 Induces Protective and Therapeutic Antitumor Immunity in Murine Multiple Myeloma. Blood 119 (1), 161–169. doi:10.1182/blood-2011-07-368472
Ren, Y., Guo, T., Xu, J., Liu, Y., and Huang, J. (2021). The Novel Target of Esophageal Squamous Cell Carcinoma: lncRNA GASL1 Regulates Cell Migration, Invasion and Cell Cycle Stagnation by Inactivating the Wnt3a/β-Catenin Signaling. Pathol. - Res. Pract. 217, 153289. doi:10.1016/j.prp.2020.153289
Ru, B., Wong, C. N., Tong, Y., Zhong, J. Y., Zhong, S. S. W., Wu, W. C., et al. (2019). TISIDB: an Integrated Repository portal for Tumor-Immune System Interactions. Bioinformatics 35 (20), 4200–4202. doi:10.1093/bioinformatics/btz210
Sato, H., Suzuki, H., Toyota, M., Nojima, M., Maruyama, R., Sasaki, S., et al. (2007). Frequent Epigenetic Inactivation of DICKKOPF Family Genes in Human Gastrointestinal Tumors. Carcinogenesis 28 (12), 2459–2466. doi:10.1093/carcin/bgm178
Shannon, P., Markiel, A., Ozier, O., Baliga, N. S., Wang, J. T., Ramage, D., et al. (2003). Cytoscape: a Software Environment for Integrated Models of Biomolecular Interaction Networks. Genome Res. 13 (11), 2498–2504. doi:10.1101/gr.1239303
Shi, R.-Y., Yang, X.-R., Shen, Q.-J., Yang, L.-X., Xu, Y., Qiu, S.-J., et al. (2013). High Expression of Dickkopf-Related Protein 1 Is Related to Lymphatic Metastasis and Indicates Poor Prognosis in Intrahepatic Cholangiocarcinoma Patients after Surgery. Cancer 119 (5), 993–1003. doi:10.1002/cncr.27788
Shinno, N., Kimura, H., Sada, R., Takiguchi, S., Mori, M., Fumoto, K., et al. (2018). Activation of the Dickkopf1-CKAP4 Pathway Is Associated with Poor Prognosis of Esophageal Cancer and Anti-CKAP4 Antibody May Be a New Therapeutic Drug. Oncogene 37 (26), 3471–3484. doi:10.1038/s41388-018-0179-2
Siegel, R. L., Miller, K. D., Fuchs, H. E., and Jemal, A. (2021). Cancer Statistics, 2021. CA A. Cancer J. Clin. 71 (1), 7–33. doi:10.3322/caac.21654
Spranger, S., and Gajewski, T. F. (2015). A New Paradigm for Tumor Immune Escape: β-catenin-driven Immune Exclusion. J. Immunotherapy Cancer 3, 43. doi:10.1186/s40425-015-0089-6
Sun, C.-X., Zhu, F., and Qi, L. (2021). Demethylated miR-216a Regulates High Mobility Group Box 3 Promoting Growth of Esophageal Cancer Cells through Wnt/β-Catenin Pathway. Front. Oncol. 11, 622073. doi:10.3389/fonc.2021.622073
Tian, F., Lu, B., Chen, Z., Liu, J., Ji, D., Li, J., et al. (2019). Microbial Antigens-Loaded Myeloma Cells Enhance Th2 Cell Proliferation and Myeloma Clonogenicity via Th2-Myeloma Cell Interaction. BMC Cancer 19 (1), 1246. doi:10.1186/s12885-019-6469-4
Vivian, J., Rao, A. A., Nothaft, F. A., Ketchum, C., Armstrong, J., Novak, A., et al. (2017). Toil Enables Reproducible, Open Source, Big Biomedical Data Analyses. Nat. Biotechnol. 35 (4), 314–316. doi:10.1038/nbt.3772
Wang, M., Li, Z., Peng, Y., Fang, J., Fang, T., Wu, J., et al. (2020). Identification of Immune Cells and mRNA Associated with Prognosis of Gastric Cancer. BMC Cancer 20 (1), 206. doi:10.1186/s12885-020-6702-1
Wirths, O., Waha, A., Weggen, S., Schirmacher, P., Kühne, T., Goodyer, C. G., et al. (2003). Overexpression of Human Dickkopf-1, an Antagonist of Wingless/WNT Signaling, in Human Hepatoblastomas and Wilms' Tumors. Lab. Invest. 83 (3), 429–434. doi:10.1097/01.lab.0000059926.66359.bd
Yamabuki, T., Takano, A., Hayama, S., Ishikawa, N., Kato, T., Miyamoto, M., et al. (2007). Dikkopf-1 as a Novel Serologic and Prognostic Biomarker for Lung and Esophageal Carcinomas. Cancer Res. 67 (6), 2517–2525. doi:10.1158/0008-5472.CAN-06-3369
Yu, B., Yang, X., Xu, Y., Yao, G., Shu, H., Lin, B., et al. (2009). Elevated Expression of DKK1 Is Associated with Cytoplasmic/nuclear β-catenin Accumulation and Poor Prognosis in Hepatocellular Carcinomas. J. Hepatol. 50 (5), 948–957. doi:10.1016/j.jhep.2008.11.020
Yu, G., Wang, L.-G., Han, Y., and He, Q.-Y. (2012). clusterProfiler: an R Package for Comparing Biological Themes Among Gene Clusters. OMICS: A J. Integr. Biol. 16 (5), 284–287. doi:10.1089/omi.2011.0118
Zhang, Q., Qin, J., Zhong, L., Gong, L., Zhang, B., Zhang, Y., et al. (2015). CCL5-Mediated Th2 Immune Polarization Promotes Metastasis in Luminal Breast Cancer. Cancer Res. 75 (20), 4312–4321. doi:10.1158/0008-5472.Can-14-3590
Keywords: DKK1, pan-cancer, survival analysis, immune infiltration, biomarker
Citation: Gao S, Jin Y and Zhang H (2021) Pan-Cancer Analyses Reveal Oncogenic and Immunological Role of Dickkopf-1 (DKK1). Front. Genet. 12:757897. doi: 10.3389/fgene.2021.757897
Received: 16 August 2021; Accepted: 05 November 2021;
Published: 24 November 2021.
Edited by:
Alfredo Pulvirenti, University of Catania, ItalyReviewed by:
Hongde Liu, Southeast University, ChinaQi Zhao, University of Science and Technology Liaoning, China
Copyright © 2021 Gao, Jin and Zhang. This is an open-access article distributed under the terms of the Creative Commons Attribution License (CC BY). The use, distribution or reproduction in other forums is permitted, provided the original author(s) and the copyright owner(s) are credited and that the original publication in this journal is cited, in accordance with accepted academic practice. No use, distribution or reproduction is permitted which does not comply with these terms.
*Correspondence: Hongmei Zhang, emhhbmdobUBuY3N0LmVkdS5jbg==