Corrigendum: The 10-Repeat 3′-UTR VNTR Polymorphism in the SLC6A3 Gene May Confer Protection Against Parkinson’s Disease: A Meta-Analysis
- 1Maternal and Children’s Health Research Institute, Shunde Women and Children’s Hospital, Guangdong Medical University, Foshan, China
- 2Key Laboratory of Research in Maternal and Child Medicine and Birth Defects, Guangdong Medical University, Foshan, China
- 3Institute of Neurology, Affiliated Hospital of Guangdong Medical University, Zhanjiang, China
- 4Department of Clinical Laboratory, People’s Hospital of Haiyuan County, Zhongwei, China
- 5Department of Pediatrics, Shunde Women and Children’s Hospital, Guangdong Medical University, Foshan, China
- 6Institute of Respiratory, Shunde Women and Children’s Hospital, Guangdong Medical University, Foshan, China
The dopamine transporter (DAT) is encoded by the SLC6A3 gene and plays an important role in the regulation of the neurotransmitter dopamine. The SLC6A3 gene contains several repetition alleles (3–11 repeats) of a 40-base pair variable number of tandem repeats (VNTR) in the 3′-untranslated region (3′-UTR), which may affect DAT expression levels. The 10-repeat (10R) allele could play a protective role against PD. However, inconsistent findings have been reported.
Methods: A comprehensive meta-analysis was performed to accurately estimate the association between the 10R allele of the 3′-UTR VNTR in SLC6A3 and PD among four different genetic models.
Results: This meta-analysis included a total of 3,142 patients and 3,496 controls. We observed a significant difference between patients and controls for the allele model (10R vs. all others: OR = 0.860, 95% CI: 0.771–0.958, P = 0.006), pseudodominant model (10R/10R + 10R/9R vs. all others: OR = 0.781, 95% CI: 0.641–0.952, P = 0.014) and pseudorecessive model (10R/10R vs. all others: OR = 0.858, 95% CI: 0.760–0.969, P = 0.013) using a fixed effects model. No significant differences were observed under the pseudocodominant model (10R/9R vs. all others: OR = 1.079, 95% CI: 0.945–1.233, P = 0.262). By subgroup analysis, the 10R, 10R/10R and 10R/9R genotypes were found to be significantly different from PD in Asian populations.
Conclusion: Our findings suggest that the SLC6A3 10R may be a protective factor in susceptibility to PD.
Introduction
Parkinson’s disease (PD) is a very common neurodegenerative disorder. One of the critical neuropathologies of PD is the degeneration of dopamine-producing neurons in the substantia nigra, resulting in impairment of the dopaminergic pathway and the subsequent depletion of dopamine levels (Balestrino and Schapira 2020). Another pathologic hallmark is the presence of ubiquitinated protein deposits named Lewy bodies, which cause dopaminergic cell death (Balestrino and Schapira, 2020; Singleton et al., 2003). These pathologic changes result in depletion of dopamine levels that underlie the etiology of PD. Therefore, dopaminergic transmission and metabolism pathway genes have been investigated and are considered to be candidate genes for PD.
The dopamine transporter (DAT) plays an important role in dopaminergic neurotransmission. It is mainly present on the terminals of neurons in the substantia nigra and is responsible for controlling the duration and intensity of neurotransmission by rapid dopamine uptake into the presynaptic terminals; thus, DAT is critical in the temporal and spatial buffering of released dopamine and its recycling (Cheng and Bahar 2015; Uhl 2003; Bannon et al., 2001). In addition, DAT is considered a gateway for neurotoxicants because the nigrostriatal toxicant 1-methyl-4-phenylpyridinium (Mpp+) is taken up selectively by presynaptic DAT, and access to dopaminergic neurons leads to dopaminergic cell toxicity (Krontiris 1995; Uhl et al., 1994); DAT has also been shown to interact with alpha-synuclein (a kind of Lewy body) (Lee et al., 2001; Wersinger et al., 2003; Thomas and Beal 2007). These findings provide evidence for a role of DAT in PD and seem to explain why the density of DAT correlates with the extent of dopaminergic cell loss in PD brains.
DAT is coded by the SLC6A3 gene. The 3′-UTR of the SLC6A3 gene includes a 40 bp variable number tandem repeat (VNTR) polymorphism. Between 3 and 11 copies of the 40 bp VNTR have been identified in normal populations (Uhl 2003), and the 9 and 10 repeat alleles are most frequent in both PD patients and several populations (Uhl 2003; Bannon et al., 2001). The SLC6A3 VNTR itself seems to be a functional polymorphism. A recent study found that the seed region of miR-491 is located in the VNTR fragment of the DAT mRNA e 3′-UTR, and the effect of miR-491 on DAT expression is dependent on the VNTR copynumber (Jia et al., 2016). Thus, SLC6A3 polymorphic VNTRs may directly influence DAT expression (Fuke et al., 2001; Heinz et al., 2002; Mill et al., 2002; Lynch et al., 2003). Lin reported that the 10R alleles conferred protection against PD compared to other alleles (Lin et al., 2003), but other noteworthy studies showed different results. Given these controversial conclusions, we performed a meta-analysis to systematically, quantitatively, and objectively summarize the association between the 10R of the 3′-UTR VNTR in SLC6A3 and PD susceptibility.
Materials and Methods
Literature Search
The PubMed, Google Scholar, and Chinese National Knowledge Infrastructure databases were systematically searched for potentially qualified studies using a combination of the keywords “dopamine transporter,” “DAT,” “DAT1,” “SLC6A3,” “VNTR,” “3′-UTR,” “polymorphism,” “rs28363170” and “Parkinson.” with no language or date restrictions. All studies were evaluated on the basis of the title and abstract and we excluded studies that were clearly irrelevant. Then, potentially eligible studies were reviewed in full to determine the inclusion in the meta-analysis.
Inclusion and Exclusion Criteria
Studies included in the meta-analysis had to meet all the following inclusion criteria: 1) case-control or cohort studies that evaluated the associations between the SLC6A3 3′-UTR VNTR polymorphism and the risk of PD; 2) available data for estimating odds ratios (ORs) with corresponding 95% confidence intervals (CIs); and 3) studies in which all PD patients had been diagnosed according to the common diagnostic criteria (Jankovic 2008).
Studies were excluded from the current analysis with the following criteria: 1) not a case-control or cohort study; 2) irrelevant to PD or SLC6A3 3′-UTR VNTR; 3) genotype distribution of the control subjects is not in Hardy-Weinberg equilibrium (HWE); and 4) reports lacking detailed genotype data.
Data Extraction
The following data were independently extracted from the included studies and entered into a database to ensure the validity of the data: first author’s name, year of publication, ethnicity, number of patients and controls, allele distribution, and genotype distribution. Studies were excluded if they did not provide the above information.
Statistical Analysis
Four genetic models were used in the meta-analysis: the allele model (10R vs. all others), the pseudodominant model (10R/10R + 10R/9R vs. all others), the pseudorecessive model (10R/10R vs. all others), and the pseudocodominant model (10R/9R vs. all others). Genetic heterogeneity was evaluated using the Q-test and I2 test. I2 ranged from 0 to 100%. Significant heterogeneity was defined with p < 0.01 and I2 > 50% (He et al., 2015; Shen et al., 2015; Zhang et al., 2016). If there was no significant heterogeneity among the total of studies, ORs with corresponding 95% CIs were calculated by the fixed effect model (Mantel–Haenszel); otherwise, ORs were calculated by a random-effect model. Z test was used to determine the significance of OR. Additionally, publication bias was investigated with Egger’s test and Begg’s test (Li et al., 2016; Liu et al., 2017; Han et al., 2019). Statistical analyses were performed using STATA v.16.0 software (Stata Corporation, Texas, United States).
Results
Study Inclusion and Characteristics
A total of 175 potential studies were retrieved through the initial search. Thirty-two duplicates were excluded. Then, 143 studies were screened on title and abstract, 91 of which were excluded. The remaining 52 articles were evaluated by full-text reading, 34 of which were excluded because 10 were not case-control or cohort studies, 20 were not related to the SLC6A3 3′-UTR VNTR or PD, and 4 did not provide sufficient data. A flow chart of study selection in the meta-analysis is shown in Figure 1. There were 18 potentially relevant papers, including 14 in English and 4 in Chinese; among them, 15 studies provided allele model data (Chang et al., 2018; Lu et al., 2016; Benitez et al., 2010; Kelada et al., 2005; Zhao et al., 2004; Lin et al., 2003; Goudreau et al., 2002; Kimura et al., 2001; Kim et al., 2000; Zhang et al., 2000; Wang et al., 2000; Mercier et al., 1999; Leighton et al., 1997; Le Couteur et al., 1997; plante-Bordeneuve et al., 1997), and 15 studies had pseudodominant, pseudorecessive and pseudoadditive model data (Chang et al., 2018; Lu et al., 2016; Benitez et al., 2010; Ritz et al., 2009; Kelada et al., 2005; Zhao et al., 2004; Lin et al., 2003; Lynch et al., 2003; Kim et al., 2000; Zhang et al., 2000; Wang et al., 2000; Mercier et al., 1999; Nicholl et al., 1999; Leighton et al., 1997; Le Couteur et al., 1997). The characteristics of each study are shown in Table 1.
Heterogeneity Analysis
Cochran’s Q and I2 test results revealed low heterogeneity among studies in four models (10R vs. all others p = 0.772 I2 = 0.0%; 10R/10R + 10R/9R vs. all others: p = 0.986 I2 = 0.0%; 10R/10R vs. all others: p = 0.268 I2 = 16.5%; 10R/9R vs. all others p = 0.299 I2 = 13.8%, respectively) (Figure 2).
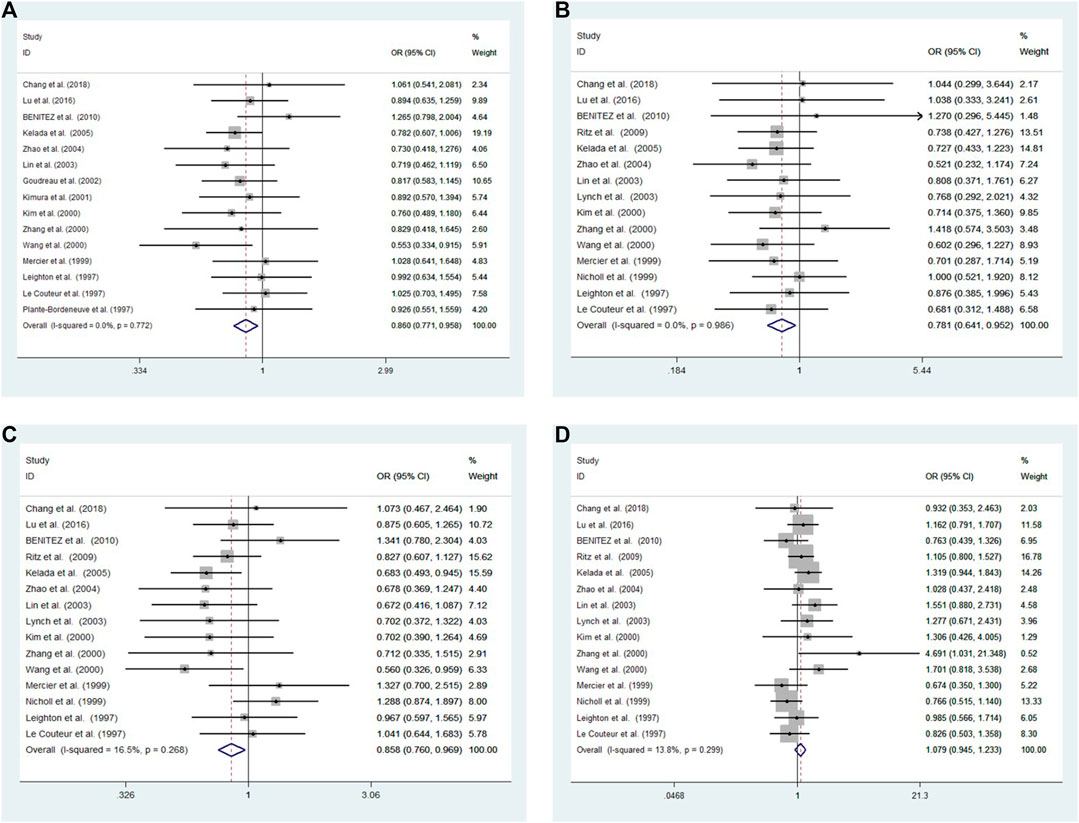
FIGURE 2. Meta-analysis with a fixed effects model for the association between the 3′-UTR VNTR in SLC6A3 and PD susceptibility. (A) Allele model, 10R vs. all others (B) Pseudodominant model, 10R/10R + 10R/9R vs. all others (C) Pseudorecessive model, 10R/10R vs. all others (D) Pseudocodominant model, 10R/9R vs. all others OR: odds ratio, CI: confidence interval, I-squared: measured to quantify the degree of heterogeneity in meta-analyses.
In the subgroup analysis by ethnicity, the results also revealed low heterogeneity among studies in four models in the Asian populations (10R vs. all others p = 0.799 I2 = 0.0%; 10R/10R + 10R/9R vs. all others: p = 0.809 I2 = 0.0%; 10R/10R vs. all others: p = 0.792 I2 = 0.0%; 10R/9R vs. all others p = 0.589 I2 = 0.0%, respectively) and in Western populations (10R vs. all others p = 0.496 I2 = 0.0%; 10R/10R + 10R/9R vs. all others: p = 0.973 I2 = 0.0%; 10R/10R vs. all others: p = 0.100 I2 = 43.7%; 10R/9R vs. all others p = 0.228 I2 = 26.3%, respectively) (Figure 3).
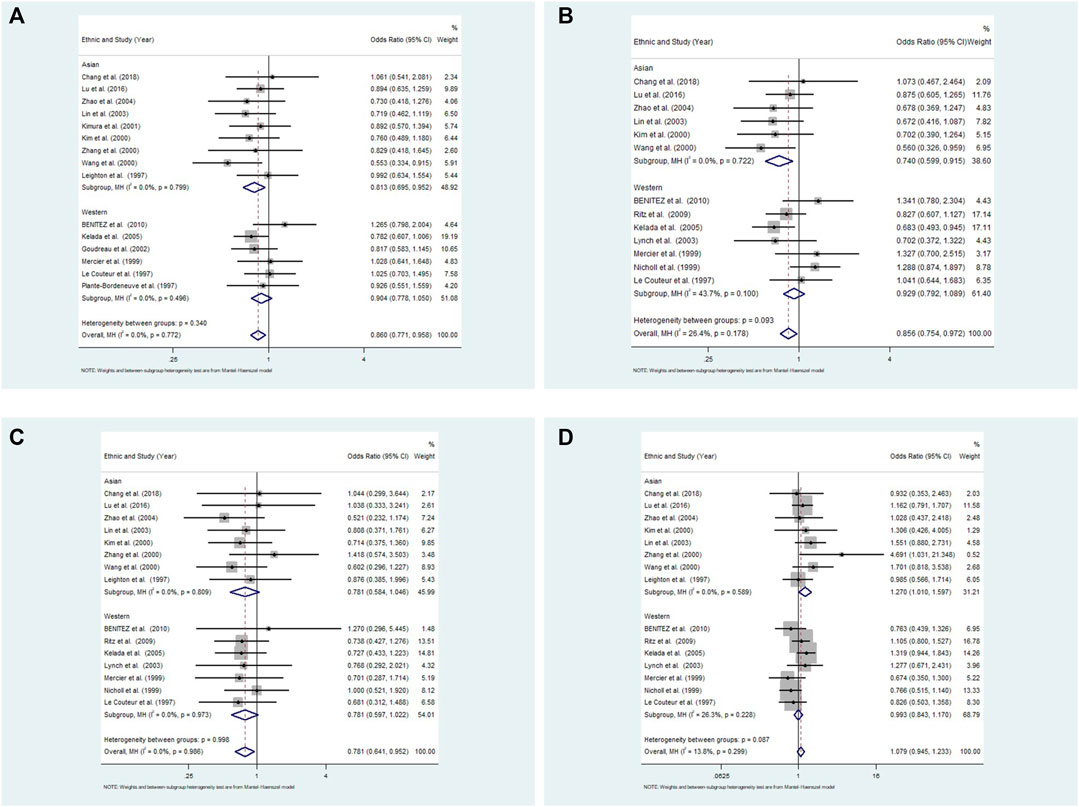
FIGURE 3. Meta-analysis with a fixed effects model for the association between the 3′-UTR VNTR in SLC6A3 and PD susceptibility in Asian and Western populations. (A) Allele model, 10R vs. all others; (B) Pseudodominant model, 10R/10R + 10R/9R vs. all others; (C) Pseudorecessive model, 10R/10R vs. all others; (D) Pseudocodominant model, 10R/9R vs. all others OR: odds ratio, CI: confidence interval, I-squared: measure to quantify the degree of heterogeneity in meta-analyses.
The Association Between the 10-Repeat of the 3′-UTR VNTR in SLC6A3 and PD
A fixed-effect model was used to analyze four models. The results showed a significant difference between patients and controls for the allele model (10R vs. all others: OR = 0.860, 95% CI: 0.771–0.958, p = 0.006), pseudodominant model (10R/10R + 10R/9R vs. all others: OR = 0.781, 95% CI: 0.641–0.952, p = 0.014) and pseudorecessive model (10R/10R vs. all others: OR = 0.858, 95% CI: 0.760–0.969, p = 0.013). No significant differences were observed under the pseudocodominant model (10R/9R vs. all others: OR = 1.079, 95% CI: 0.945–1.233, p = 0.262) (Figure 2).
In the subgroup analysis by ethnicity, the results showed a significant difference between patients and controls for the allele model (10R vs. all others: OR = 0.813, 95% CI: 0.695–0.952, p = 0.010), pseudorecessive model (10R/10R vs. all others: OR = 0.769, 95% CI: 0.637–0.928, p = 0.006) and pseudocodominant model (10R/9R vs. all others: OR = 1.270, 95% CI: 1.010–1.597, p = 0.041), but no significant differences were observed under the pseudodominant model (10R/10R + 10R/9R vs. all others: OR = 0.781, 95% CI: 0.584–1.046, p = 0.097) in Asian populations with a fixed-effect model. There was no significant difference between patients and controls for the four models in the Western populations (10R vs. all others: OR = 0.904, 95% CI: 0.778–1.050, p = 0.187; 10R/10R + 10R/9R vs. all others: OR = 0.781, 95% CI: 0.597–1.022, p = 0.071; 10R/10R vs. all others: OR = 0.929, 95% CI: 0.792–1.089, p = 0.361; 10R/9R vs. all others: OR = 0.993, 95% CI: 0.843–1.170, p = 0.930) (Figure 3).
Publication Bias
No significant publication bias was observed in any of the above genetic models via Begg’s funnel plot and Egger’s test (all p > 0.05, data not shown), and the funnel plot was symmetrical, with studies not coagulating into one quadrant of the funnel (Figures 4, 5).
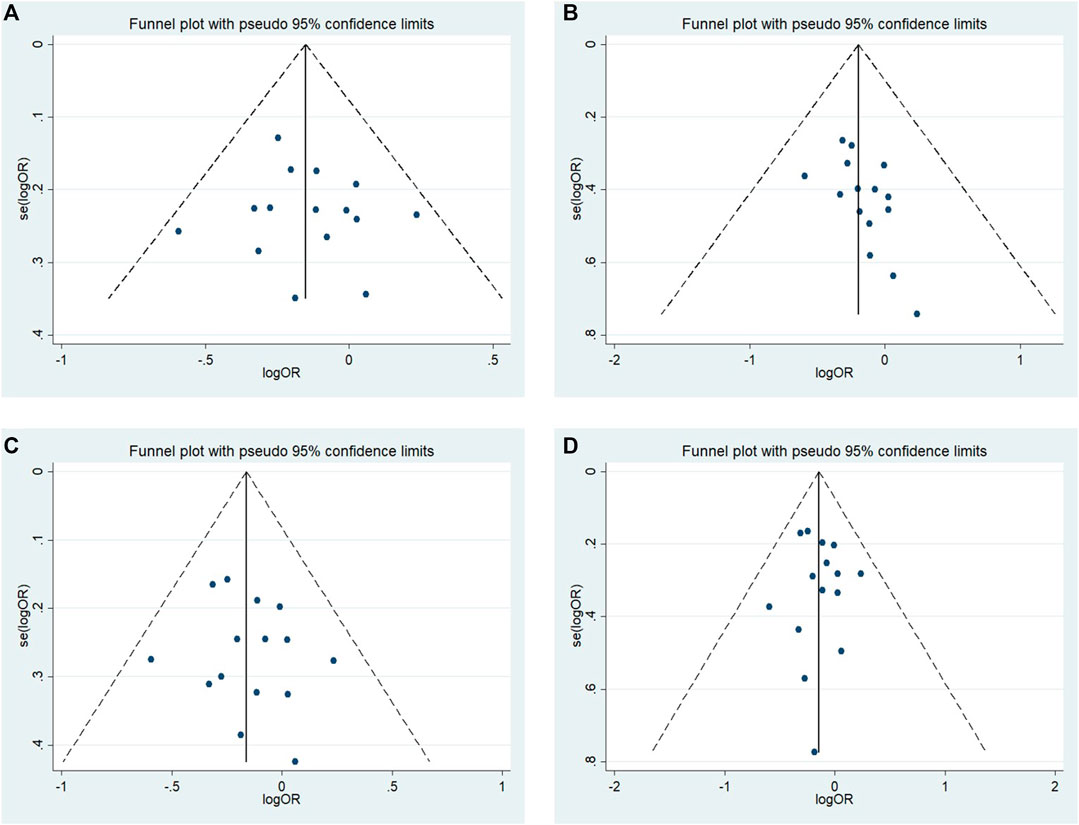
FIGURE 4. Funnel plot of the odds ratios in the meta-analysis. (A) Allele model, 10R vs. all others (B) Pseudodominant model, 10R/10R + 10R/9R vs. all others (C) Pseudorecessive model, 10R/10R vs. all others (D) Pseudocodominant model, 10R/9R vs. all others OR: odds ratio, CI: confidence interval, I-squared: measured to quantify the degree of heterogeneity in meta-analyses.
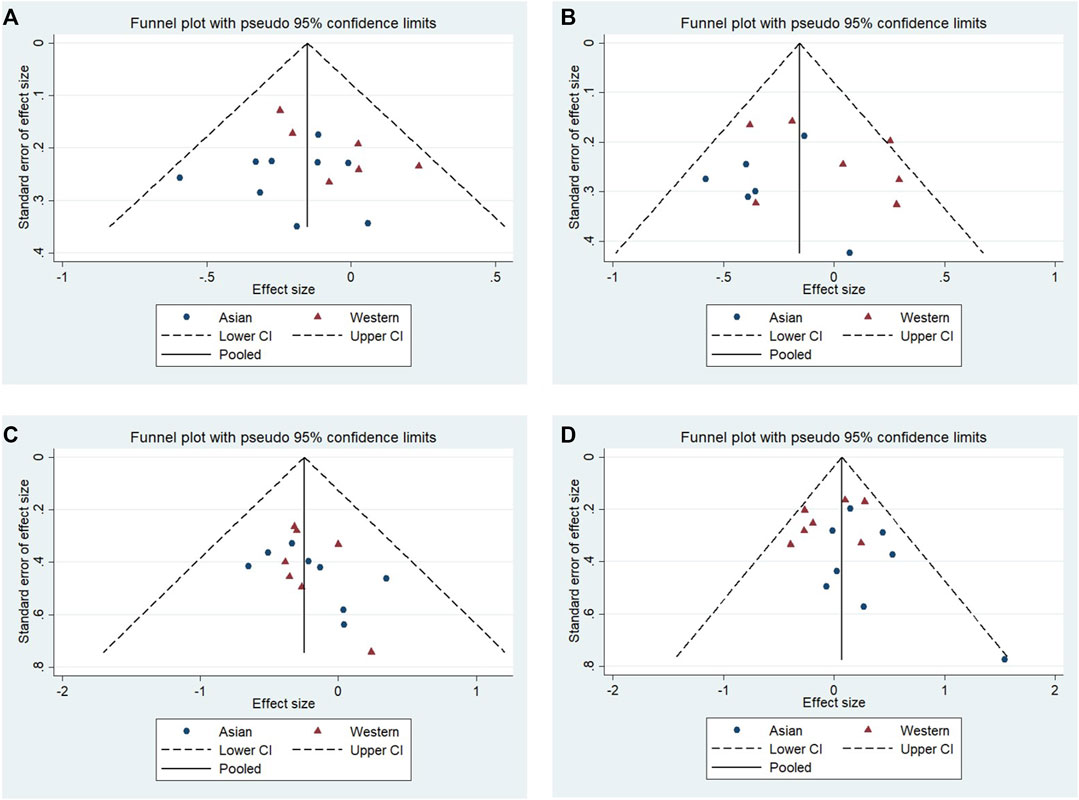
FIGURE 5. Funnel plot of the odds ratios in the subgroup: Asian populations and Western populations. (A) Allele model, 10R vs. all others. (B) Pseudodominant model, 10R/10R + 10R/9R vs. all others. (C) Pseudorecessive model, 10R/10R vs. all others. (D) Pseudocodominant model, 10R/9R vs. all others.
Discussion
This meta-analysis assessed the association between the 10R allele of the 3′-UTR VNTR in the SLC6A3 gene and PD, and it included a total of 18 published studies. In general, our findings suggested that the 10R alleles and 10R/10R and 10R/10R + 10R/9R genotypes of the VNTR polymorphism in the SLC6A3 gene confer protection against PD. The 10R alleles and 10R/10R genotype results were replicated in Asian populations, and the 10R/9R genotype was associated with an increased risk of PD in Asian populations. The current meta-analysis confirmed most of the previous findings showing that the 10R allele of the 3′-UTR VNTR in the SLC6A3 gene may be a protective factor in susceptibility to PD.
Previous studies have shown that the prevalence of PD in Asia is low, approximately half that of Caucasians (Zhang and Román 1993; Leighton et al., 1997). This may be related to the discrepancies in genetic polymorphisms among populations of different racial and ethnic groups. There was a difference in allelic frequency in the SLC6A3 VNTR polymorphism (Vandenbergh et al., 1992; Sano et al., 1993; Le Couteur et al., 1997; Leighton et al., 1997; Mercier et al., 1999; Kim et al., 2000) and the distribution was similar among the different Asian ethnic populations (Chinese, Korean and Japanese), but it was different from the Western populations. There are research findings that the frequencies of the 10 and 11 repeats of SLC6A3 in Asian populations were higher than those in Caucasians, but the 9R of SLC6A3 was much lower in normal Asian populations. The results of our meta-analysis indicate that the 10R may be a protective factor against susceptibility to PD in Asian populations, which may be one of the reasons for the low prevalence of PD in Asia.
Several studies indicate that the 9R allele demonstrates more enhanced transcription activity than the 10R allele of the SLC6A3 VNTR polymorphism (Purcaro et al., 2019; Miller and Madras, 2002; Michelhaugh et al., 2001). From a clinical point of view, increased DAT expression due to the 9R allele might exacerbate striatal neuronal damage over time by increasing the presynaptic uptake of potentially neurotoxic endogenous or exogenous substrates via DAT, such as 1-methyl-4-phenyl-1,2,3,6-tetrahydropyridine (MPTP) (Contin et al., 2004; Tipton and Singer, 1993). However, the 10R/10R genotype of the SLC6A3 gene may result in the most stable expression, which may confer nerve terminal protection against Mpp+-like compounds and prevent the toxicity of dopaminergic neurons (Lin et al., 2003). This may effectively reduce the incidence of PD. Moreover, interindividual genetic differences in DAT might also play a role in the therapeutic outcome of levodopa-treated PD patients (Contin et al., 2004). The DAT 9R allele has been suggested to be a predictor of dyskinesias or psychosis in PD patients (Kaiser et al., 2003). In general, research has shown that changes in the number of VNTR copies are closely related to PD, and our meta-analysis suggests that the 10R allele may be a protective factor in susceptibility to PD. We also conducted heterogeneity analysis, and we found low heterogeneity in our meta-analysis. In addition, our meta-analysis showed no publication bias.
There are potential limitations to the current study. First, PD is a complex disorder that develops as a result of age, environmental, and genetic factors, but age and exposure to environmental agents were often not discussed in our included studies. Moreover, interactions between multiple genes might affect the risk of PD. Additionally, since some are a bit ambiguous from the current Ethnic column (e.g., Ritz et al., Lynch et al.). Ritz’s study inclued 13 Asian populations, and Lynch‘s sudy inclued 9 other populations. Although these quantities account for a relatively small proportion of the total, these were difficult to conduct more accurate analyses. Therefore, the findings should be interpreted with caution. Further studies are necessary to establish larger sample sizes and consider SNP-SNP, gene–gene and gene–environmental interactions before reaching robust conclusions.
Conclusion
Our findings suggest that the 10R of the 3′-UTR VNTR in SLC6A3 may be a protective factor in susceptibility to PD. This result was also confirmed in Asian populations.
Data Availability Statement
The original contributions presented in the study are included in the article, further inquiries can be directed to the corresponding authors.
Author Contributions
QZ, FN and SG were responsible for the statistical analysis, study design, and manuscript preparation. Qd Z, RC, and LP managed the literature searches and analyses. The study was supervised by DZ, GM, and YW.
Funding
Support for this work includes funding from the National Natural Science Foundation of China (81670252 and 81770034), Guangdong Basic and Applied Basic Foundation (2019A1515011306 and 2020A1515010240), Guangdong Medical University Research Foundation (GDMUM2020008 and GDMUM2020012); Medical Research Project of Foshan Health Bureau (20210188 and 20210289).
Conflict of Interest
The authors declare that the research was conducted in the absence of any commercial or financial relationships that could be construed as a potential conflict of interest.
Publisher’s Note
All claims expressed in this article are solely those of the authors and do not necessarily represent those of their affiliated organizations, or those of the publisher, the editors and the reviewers. Any product that may be evaluated in this article, or claim that may be made by its manufacturer, is not guaranteed or endorsed by the publisher.
References
Balestrino, R., and Schapira, A. H. V. (2020). Parkinson Disease. Eur. J. Neurol. 27 (1), 27–42. doi:10.1111/ene.14108
Bannon, M. J., Michelhaugh, S. K., Wang, J., and Sacchetti, P. (2001). The Human Dopamine Transporter Gene: Gene Organization, Transcriptional Regulation, and Potential Involvement in Neuropsychiatric Disorders. Eur. Neuropsychopharmacol. 11, 449–455. doi:10.1016/s0924-977x(01)00122-5
Benitez, B. A., Forero, D. A., Arboleda, G. H., Granados, L. A., Yunis, J. J., Fernandez, W., et al. (2010). Exploration of Genetic Susceptibility Factors for Parkinson's Disease in a South American Sample. J. Genet. 89, 229–232. doi:10.1007/s12041-010-0030-1
Chang, P., Zhao, P., Wang, X., Wang, C., and Bao, B. (2018). Relationship between Dopamine Transporter Gene Polymorphism and Parkinson's Disease Susceptibility and PET- CT Imagine. China Chin. J. Geriatr. 21, 5244–5246. [in Chinese].
Cheng, M. H., and Bahar, I. (2015). Molecular Mechanism of Dopamine Transport by Human Dopamine Transporter. Structure 23 (11), 2171–2181. doi:10.1016/j.str.2015.09.001
Contin, M., Martinelli, P., Mochi, M., Albani, F., Riva, R., Scaglione, C., et al. (2004). Dopamine Transporter Gene Polymorphism, Spect Imaging, and Levodopa Response in Patients with Parkinson Disease. Clin. Neuropharmacology 27 (3), 111–115. doi:10.1097/00002826-200405000-00004
Fuke, S., Suo, S., Takahashi, N., Koike, H., Sasagawa, N., and Ishiura, S. (2001). The VNTR Polymorphism of the Human Dopamine Transporter (DAT1) Gene Affects Gene Expression. Pharmacogenomics J. 1, 152–156. doi:10.1038/sj.tpj.6500026
Goudreau, J. L., Maraganore, D. M., Farrer, M. J., Lesnick, T. G., Singleton, A. B., Bower, J. H., et al. (2002). Case-control Study of Dopamine Transporter-1, Monoamine Oxidase-B, and Catechol-O-Methyl Transferase Polymorphisms in Parkinson's Disease. Mov Disord. 17, 1305–1311. doi:10.1002/mds.10268
Han, Z., Wang, T., Tian, R., Zhou, W., Wang, P., Ren, P., et al. (2019). BIN1 Rs744373 Variant Shows Different Association with Alzheimer's Disease in Caucasian and Asian Populations. BMC bioinformatics 20, 691. doi:10.1186/s12859-019-3264-9
He, D., Ma, L., Feng, R., Zhang, L., Jiang, Y., Zhang, Y., et al. (2015). Analyzing Large-Scale Samples Highlights Significant Association between Rs10411210 Polymorphism and Colorectal Cancer. Biomed. Pharmacother. 74, 164–168. doi:10.1016/j.biopha.2015.08.023
Heinz, A., Goldman, D., Jones, D. W., Palmour, R., Hommer, D., Gorey, J. G., et al. (2002). Genotype Influences In Vivo Dopamine Transporter Availability in Human Striatum. Neuropsychopharmacology 22, 133–139. doi:10.1016/S0893-133X(99)00099-8
Jankovic, J. (2008). Parkinson's Disease: Clinical Features and Diagnosis. J. Neurol. Neurosurg. Psychiatry 79, 368–376. doi:10.1136/jnnp.2007.131045
Jia, X., Wang, F., Han, Y., Geng, X., Li, M., Shi, Y., et al. (2016). miR-137 and miR-491 Negatively Regulate Dopamine Transporter Expression and Function in Neural Cells. Neurosci. Bull. 32 (6), 512–522. doi:10.1007/s12264-016-0061-6
Kaiser, R., Hofer, A., Grapengiesser, A., Gasser, T., Kupsch, A., Roots, I., et al. (2003). L -Dopa-Induced Adverse Effects in PD and Dopamine Transporter Gene Polymorphism. Neurology 60 (11), 1750–1755. doi:10.1212/01.wnl.0000068009.32067.a1
Kelada, S. N., Costa-Mallen, P., Checkoway, H., Carlson, C. S., Weller, T.-S., Swanson, P. D., et al. (2005). Dopamine Transporter (SLC6A3) 5′ Region Haplotypes Significantly Affect Transcriptional Activity In Vitro but Are Not Associated with Parkinson's Disease. Pharmacogenetics and genomics 15, 659–668. doi:10.1097/01.fpc.0000170917.04275.d6
Kim, J. W., Kim, D. H., Kim, S. H., and Cha, J. K. (2000). Association of the Dopamine Transporter Gene with Parkinson's Disease in Korean Patients. J. Korean Med. Sci. 15, 449–451. doi:10.3346/jkms.2000.15.4.449
Kimura, M., Matsushita, S., Arai, H., Takeda, A., and Higuchi, S. (2001). No Evidence of Association between a Dopamine Transporter Gene Polymorphism (1215A/G) and Parkinson's Disease. Ann. Neurol. 49, 276–277. doi:10.1002/1531-8249(20010201)49:2<276:aid-ana54>3.0.co;2-2
Krontiris, T. (1995). Minisatellites and Human Disease. Science 269, 1682–1683. doi:10.1126/science.7569893
Le Couteur, D. G., Leighton, P. W., McCann, S. J., and Pond, S. M. (1997). Association of a Polymorphism in the Dopamine-Transporter Gene with Parkinson's Disease. Mov Disord. 12, 760–763. doi:10.1002/mds.870120523
Lee, F. J. S., Liu, F., Pristupa, Z. B., and Niznik, H. B. (2001). Direct Binding and Functional Coupling of α‐synuclein to the Dopamine Transporters Accelerate Dopamine‐induced Apoptosis. FASEB j. 15, 916–926. doi:10.1096/fj.00-0334com10.1096/fsb2fj000334com
Leighton, P. W., Le Couteur, D. G., Pang, C. C. P., McCann, S. J., Chan, D., Law, L. K., et al. (1997). The Dopamine Transporter Gene and Parkinson's Disease in a Chinese Population. Neurology 49, 1577–1579. doi:10.1212/wnl.49.6.1577
Li, Y., Song, D., Jiang, Y., Wang, J., Feng, R., Zhang, L., et al. (2016). CR1 Rs3818361 Polymorphism Contributes to Alzheimer's Disease Susceptibility in Chinese Population. Mol. Neurobiol. 53, 4054–4059. doi:10.1007/s12035-015-9343-7
Lin, J. J., Yueh, K. C., Chang, D. C., Chang, C. Y., Yeh, Y. H., and Lin, S. Z. (2003). The Homozygote 10-Copy Genotype of Variable Number Tandem Repeat Dopamine Transporter Gene May Confer Protection Against Parkinson's Disease for Male, But Not to Female Patients. J. Neurol. Sci. 209, 87–92. doi:10.1016/s0022-510x(03)00002-9
Liu, G., Xu, Y., Jiang, Y., Zhang, L., Feng, R., and Jiang, Q. (2017). PICALM Rs3851179 Variant Confers Susceptibility to Alzheimer's Disease in Chinese Population. Mol. Neurobiol. 54, 3131–3136. doi:10.1007/s12035-016-9886-2
Lu, Q., Song, Z., Deng, X., Xiong, W., Xu, H., Zhang, Z., et al. (2016). SLC6A3 Rs28363170 and Rs3836790 Variants in Han Chinese Patients with Sporadic Parkinson's Disease. Neurosci. Lett. 629, 48–51. doi:10.1016/j.neulet.2016.06.053
Lynch, D. R., Mozley, P. D., Sokol, S., Maas, N. M. C., Balcer, L. J., and Siderowf, A. D. (2003). Lack of Effect of Polymorphisms in Dopamine Metabolism Related Genes on Imaging of TRODAT-1 in Striatum of Asymptomatic Volunteers and Patients with Parkinson's Disease. Mov Disord. 18, 804–812. doi:10.1002/mds.10430
Mercier, G., Turpin, J. C., and Lucotte, G. (1999). Variable Number Tandem Repeat Dopamine Transporter Gene Polymorphism and Parkinson's Disease: No Association Found. J. Neurol. 246, 45–47. doi:10.1007/s004150050304
Michelhaugh, S. K., Fiskerstrand, C., Lovejoy, E., Bannon, M. J., and Quinn, J. P. (2001). The Dopamine Transporter Gene (SLC6A3) Variable Number of Tandem Repeats Domain Enhances Transcription in Dopamine Neurons. J. Neurochem. 79 (5), 1033–1038. doi:10.1046/j.1471-4159.2001.00647.x
Mill, J., Asherson, P., Browes, C., D'Souza, U., and Craig, I. (2002). Expression of the Dopamine Transporter Gene Is Regulated by the 3? UTR VNTR: Evidence from Brain and Lymphocytes Using Quantitative RT-PCR. Am. J. Med. Genet. 114, 975–979. doi:10.1002/ajmg.b.10948
Miller, G. M., and Madras, B. K. (2002). Polymorphisms in the 3′-untranslated Region of Human and Monkey Dopamine Transporter Genes Affect Reporter Gene Expression. Mol. Psychiatry 7 (1), 44–55. doi:10.1038/sj.mp.4000921
Nicholl, D. J., Bennett, P., Hiller, L., Bonifati, V., Vanacore, N., Fabbrini, G., et al. (1999). A Study of Five Candidate Genes in Parkinson's Disease and Related Neurodegenerative Disorders. Neurology 53, 1415. doi:10.1212/wnl.53.7.1415
Planté-Bordeneuve, V., Taussig, D., Thomas, F., Said, G., Wood, N. W., Marsden, C. D., et al. (1997). Evaluation of Four Candidate Genes Encoding Proteins of the Dopamine Pathway in Familial and Sporadic Parkinson's Disease. Neurology 48, 1589–1593. doi:10.1212/wnl.48.6.1589
Purcaro, C., Vanacore, N., Moret, F., Di Battista, M. E., Rubino, A., Pierandrei, S., et al. (2019). DAT Gene Polymorphisms (Rs28363170, Rs393795) and Levodopa-Induced Dyskinesias in Parkinson's Disease. Neurosci. Lett. 690, 83–88. doi:10.1016/j.neulet.2018.10.021
Ritz, B. R., Manthripragada, A. D., Costello, S., Lincoln, S. J., Farrer, M. J., Cockburn, M., et al. (2009). Dopamine Transporter Genetic Variants and Pesticides in Parkinson's Disease. Environ. Health Perspect. 117, 964–969. doi:10.1289/ehp.0800277
Sano, A., Kondoh, K., Kakimoto, Y., and Kondo, I. (1993). A 40-nucleotide Repeat Polymorphism in the Human Dopamine Transporter Gene. Hum. Genet. 91, 405–406. doi:10.1007/BF00217369
Shen, N., Chen, B., Jiang, Y., Feng, R., Liao, M., Zhang, L., et al. (2015). An Updated Analysis with 85,939 Samples Confirms the Association between CR1 Rs6656401 Polymorphism and Alzheimer's Disease. Mol. Neurobiol. 51, 1017–1023. doi:10.1007/s12035-014-8761-2
Singleton, A. B., Farrer, M., Johnson, J., Singleton, A., Hague, S., Kachergus, J., et al. (2003). -Synuclein Locus Triplication Causes Parkinson's Disease. Science 302, 841. doi:10.1126/science.1090278
Thomas, B., and Beal, M. F. (2007). Parkinson's Disease. Hum. Mol. Genet. 16, R183–R194. doi:10.1093/hmg/ddm159
Tipton, K. F., and Singer, T. P. (1993). Advances in Our Understanding of the Mechanisms of the Neurotoxicity of MPTP and Related Compounds. J. Neurochem. 61 (4), 1191–1206. doi:10.1111/j.1471-4159.1993.tb13610.x
Uhl, G. R. (2003). Dopamine Transporter: Basic Science and Human Variation of a Key Molecule for Dopaminergic Function, Locomotion, and Parkinsonism. Mov Disord. 18, S71–S80. doi:10.1002/mds.10578
Uhl, G. R., Walther, D., Mash, D., Faucheux, B., and Javoy-Agid, F. (1994). Dopamine Transporter Messenger RNA in Parkinson's Disease and Control Substantia Nigra Neurons. Ann. Neurol. 35, 494–498. doi:10.1002/ana.410350421
Vandenbergh, D. J., Persico, A. M., Hawkins, A. L., Griffin, C. A., Li, X., Jabs, E. W., et al. (1992). Human Dopamine Transporter Gene (DAT1) Maps to Chromosome 5p15.3 and Displays a VNTR. Genomics 14, 1104–1106. doi:10.1016/s0888-7543(05)80138-7
Wang, J., Liu, Z., Chen, B., Li, J., and Chen, L. (2000). Association between Genetic Polymorphism of Dopamine Transporter Gene and Susceptibility to Parkinson's Disease. Zhonghua Yi Xue Za Zhi 80, 346–348. [in Chinese].
Wersinger, C., Prou, D., Vernier, P., and Sidhu., A. (2003). Modulation of Dopamine Transporter Function by α‐synuclein Is Altered by Impairment of Cell Adhesion and by Induction of Oxidative Stress. FASEB j. 17, 1–30. doi:10.1096/fj.03-0152fje
Zhang, L., Shao, M., Xu, Q., Dong, X., Yang, J., and Li, Y. (2000). Association between Dopamine Transporter Gene Polymorphism and Parkinson's Disease. Zhonghua Yi Xue Yi Chuan Xue Za Zhi 80, 431–434. [in Chinese].
Zhang, S., Li, X., Ma, G., Jiang, Y., Liao, M., Feng, R., et al. (2016). CLU Rs9331888 Polymorphism Contributes to Alzheimer's Disease Susceptibility in Caucasian but Not East Asian Populations. Mol. Neurobiol. 53, 1446–1451. doi:10.1007/s12035-015-9098-1
Zhang, Z.-X., and Román, G. C. (1993). Worldwide Occurrence of Parkinson's Disease: an Updated Review. Neuroepidemiology 12, 195–208. doi:10.1159/000110318
Keywords: Parkinson’s disease, Slc6a3, dopamine transporter, variable number of tandem repeats, meta-analysis
Citation: Zeng Q, Ning F, Gu S, Zeng Q, Chen R, Peng L, Zou D, Ma G and Wang Y (2021) The 10-Repeat 3′-UTR VNTR Polymorphism in the SLC6A3 Gene May Confer Protection Against Parkinson’s Disease: A Meta-analysis. Front. Genet. 12:757601. doi: 10.3389/fgene.2021.757601
Received: 12 August 2021; Accepted: 14 September 2021;
Published: 27 September 2021.
Edited by:
Guiyou Liu, Tianjin Institute of Industrial Biotechnology (CAS), ChinaReviewed by:
Nicholas B. Larson, Mayo Clinic, United StatesZhijie Han, Chongqing Medical University, China
Copyright © 2021 Zeng, Ning, Gu, Zeng, Chen, Peng, Zou, Ma and Wang. This is an open-access article distributed under the terms of the Creative Commons Attribution License (CC BY). The use, distribution or reproduction in other forums is permitted, provided the original author(s) and the copyright owner(s) are credited and that the original publication in this journal is cited, in accordance with accepted academic practice. No use, distribution or reproduction is permitted which does not comply with these terms.
*Correspondence: Dehua Zou, 757384895@qq.com; Guoda Ma, sihan1107@126.com; Yajun Wang, wangyajuny1977@aliyun.com
†These authors have contributed equally to this work