- 1The Cancer Center of the Fifth Affiliated Hospital of Sun Yat-sen University, Zhuhai, China
- 2Guangdong Provincial Key Laboratory of Biomedical Imaging, The Fifth Affiliated Hospital, Sun Yat-sen University, Zhuhai, China
- 3Center of Infectious Diseases, The Fifth Affiliated Hospital, Sun Yat-Sen University, Zhuhai, China
Purpose: To investigate the prognostic significance of tumor mutational burden (TMB) combined with specific prognosis-related gene mutations in immunotherapy for recurrent and metastatic head and neck squamous cell carcinoma (r/m HNSCC).
Methods: One hundred thirty-two r/m HNSCC patients from the Morris and Allen cohorts had undergone immunotherapy. We constructed the immunotherapy-related gene prognostic index TP-PR combining TMB and PIK3CA, TP53, or ROS1 mutation. And we analyzed the differences in overall survival (OS) and immune cell infiltration between samples in different groups. The association of each signature’s single-sample gene set enrichment analysis scores with TP-PR was tested using Spearman’s correlation test.
Results: The median OS of the patients with high TMB (TMB ≥10 mut/Mb) who received immunotherapy for r/m HNSCC was 2.5 times as long as that of the patients with low TMB (25 vs. 10 months). More importantly, the high TP-PR (TP-PR >0) group had better median OS (25 vs. 8 months) than the low TP-PR (TP-PR ≤0) group. CD8+ T cells and activated memory CD4+ T cells in the tissues of the patients with high TP-PR were higher than those in the patients with low TP-PR. Results showed that TP-PR stratification had a higher area under the curve (AUC) value (0.77, 95% CI 0.86–0.68) compared with TMB stratification (0.56, 95% CI 0.68–0.44). The differential gene expression in the high and low TP-PR groups mainly influenced metabolism-related signaling pathways.
Conclusion: TP-PR was an effective predictor of immunotherapy outcome for r/m HNSCC, which might be better than TMB alone. Patients with high TP-PR had a better survival benefit than had the patients with low TP-PR.
Introduction
Head and neck squamous cell carcinoma (HNSCC) is a general term for squamous cell carcinoma originating in the head and neck, including the oral cavity, nasopharynx, oropharynx, hypopharynx, and larynx (Johnson et al., 2020). The incidence of HNSCC is the sixth highest in the world, with more than 830,000 people diagnosed each year and a fatality rate of approximately 50% (Bray et al., 2018). At present, the first-line treatment for HNSCC is mainly surgery, radiotherapy, and chemotherapy. Recurrent and metastatic (r/m) HNSCC has been treated with platinum-based chemotherapy in combination with an anti-epidermal growth factor receptor targeted drug cetuximab (Leemans et al., 2018). However, no matter what chemotherapy regimen was used in combination with cetuximab, the median overall survival (OS) of r/m HNSCC patients was approximately 10 months (Zhu et al., 2021). KEYNOTE-048 was a landmark clinical study performed for r/m HNSCC treatment, which demonstrated that the first-line treatment of r/m HNSCC population with positive programmed cell death ligand 1 (PD-L1) expression with pembrolizumab alone or combined with platinum-containing chemotherapy presented a greater survival benefit than traditional targeted first-line chemotherapy drugs (Burtness et al., 2019). The approval of pembrolizumab as a first-line therapy for r/m HNSCC marks the beginning of the era of immunotherapy for head and neck tumors.
However, one of the major limitations of immune checkpoint inhibitor (ICI) treatment is its low response rate. Therefore, we need to find more valuable biomarkers to predict the effect of immunotherapy on the prognosis of patients with r/m HNSCC. KEYNOTE-158 clinical trial was performed for advanced solid tumors including anal, biliary, and 10 other cancers (HNSCC was not included). It demonstrated significant improvement in objective response rate and progression-free survival after immunotherapy in patients with tumor mutational burden (TMB) ≥10 mut/Mb (Marabelle et al., 2020). As a result of this study, TMB became the second approved “pan-cancer” biomarker following microsatellite instability (Goodman et al., 2017; Marabelle et al., 2020; Valero, 2021).
The role of TMB in predicting the outcome of immunotherapy for advanced HNSCC remains unclear because of absence of definitive data. A previous study showed that TMB is a predictor of prognosis in patients with HNSCC, and high TMB (TMB-H) (top 25%) has a greater survival benefit than low TMB (TMB-L) (bottom 25%), whereas TMB ≥10 mut/Mb was not prognostic for them (Zhang et al., 2020a). But they did not investigate the prognosis of immunotherapy efficacy. Another study showed that microsatellite-stable solid tumors with TMB-H (TMB ≥10 mut/Mb) had higher response rates than those with TMB-L after immunotherapy (Valero, 2021). However, there were no data on survival prognosis.
To determine the significance of TMB in predicting immunotherapy outcome in patients with r/m HNSCC, and to find a valuable prognostic index, we retrospectively analyzed survival data, TMB expression, and gene mutations in patients with r/m HNSCC using The Cancer Genome Atlas (TCGA) and the cBioPortal databases. We further constructed the immunotherapy-related gene prognostic index TP-PR for r/m HNSCC to clarify the benefits of ICI therapy for some TP-PR-defined group.
Methods
Patient Selection
We used five cohorts, namely, the Morris cohort (Samstein et al., 2019), the Allen cohort (Miao et al., 2018), the Ho cohort (Morris et al., 2017), the Berger cohort (Zehir et al., 2017), and TCGA cohort. The inclusion criteria for r/m HNSCC is shown in Figure 1. The Morris cohort finally included 120 patients, and the Allen cohort included 12 patients (Supplemental Table S1). All patients were diagnosed with r/m HNSCC, not including nasopharyngeal carcinoma. Those 132 patients had undergone immunotherapy with anti-programmed cell death protein 1 (PD1)/PD-L1 agents alone or combined with CTLA-4 inhibitors. Only patients for whom whole-exome sequencing (WES) and survival information were available were analyzed for this study. The Ho cohort included 115 r/m HNSCC patients, and the Berger cohort included 49 r/m HNSCC patients who did not receive immunotherapy (Supplemental Table S2, S3). TCGA cohort included 488 patients with HNSCC with few r/m HNSCC patients included (Supplemental Table S4). No patient from TCGA cohort had received immunotherapy. All relevant information from the five cohorts was downloaded from cbioportal.org, including mutation landscape data, WES, and RNA sequencing (RNA-seq) data, and survival information.
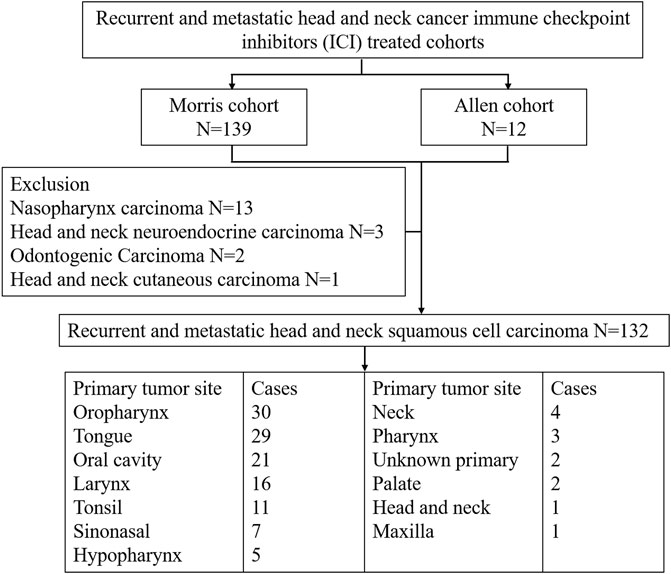
FIGURE 1. Patient selection of recurrent and metastatic head and neck cancer immune checkpoint inhibitor (ICI)-treated cohorts.
Tumor Mutational Burden Score
TMB was calculated as total variants divided by the total callable bases covered by the MSK-IMPACT or WES panel in megabases (Mb) for both the intronic and exonic sequences (total variants/Mb) or for exonic and splice site sequence (nonsynonymous mutations/Mb). TMB score of the Morris cohort, the Allen cohort, the Ho cohort, and the Berger cohort was downloaded from cbioportal.org. TMB score of TCGA cohort was downloaded from the literature (Thorsson et al., 2018). The mean coverage of the WES was 38 Mb. Variant allele frequencies cutoffs can vary from 0.5 to 10%, with lower thresholds increasing the risk of including false positives arising from sequencing artifacts such as C-to-T transitions introduced by formalin fixation (Chan et al., 2019). Thus, we selected variants with an allelic fraction of at least 10%.
Correlation Analysis of Tumor Immunogenicity and Immune Characteristics
We used the CIBERSORT web portal (https://cibersort.stanford.edu/) to evaluate the infiltration status of 22 immune cell types for TCGA cohort. Then, we analyzed the differences in immune cell infiltration between the samples in different groups.
RNA Sequencing Data Processing and Analysis
RNA-seq data were downloaded from UCSC Xena (https://xenabrowser.net/datapages). RNA-seq count data normalized to FPKM (fragments per kilobase per million) values were used as input for single-sample gene set enrichment analysis (ssGSEA). ssGSEA was performed for select signatures using the gene set variation analysis package with default settings. The association of each signature’s ssGSEA scores with TP-PR was tested using Spearman’s correlation test.
Construction of a Prognostic Model (TP-PR Score)
Univariate and multivariate Cox regression analyses were performed to analyze the gene mutations significantly associated with OS. TMB and the gene mutations significantly affecting OS were used to construct TP-PR score. The TP-PR score of each sample was calculated by multiplying the values of certain indexes by their weight in the Cox model and then adding them together. The prognostic index TP-PR (formula: TP-PR = TMB * 1.0803 + PIK3CA * 0.654 − TP53 * 0.5581 − ROS1 * 1.2342) was then constructed according to the expression of those three genes. It was scored 1 if TMB ≥10 mut/Mb or if the gene was mutated and was scored 0 if TMB <10 mut/Mb or if the gene was wide-type. The final score was calculated as the TP-PR score. Regression coefficients were generated in the process of multifactor Cox regression analysis with R software (version 4.1.1). The prognostic power of the TP-PR score was evaluated by Kaplan–Meier (K-M) survival curves with log-rank tests with the ICI cohort.
Statistics
The K-M estimator was used to describe the distribution of survival time. The log-rank Mantel–Cox test was used to compare survival between different strata. Statistical analyses were performed using GraphPad software, version 8.0 (GraphPad Software Inc., La Jolla, CA, USA). All data points and statistical analyses represent individual patients.
Results
Tumor Mutational Burden is a Prognostic Factor for the Overall Survival of Recurrent and Metastatic Head and Neck Squamous Cell Carcinoma Patients Undergoing Immunotherapy
We collected the data of 132 r/m HNSCC patients receiving immunotherapy from cBioPortal (https://www.cbioportal.org/study/summary?id=tmb_mskcc_2018; https://www.cbioportal.org/study/summary?id=mixed_allen_2018). The inclusion criteria for r/m HNSCC are shown in Figure 1. Detailed characteristics including age, gender, sample type, and administered immunotherapy drugs are shown in Table 1. We characterized patients as having TMB-H or TMB-L on the basis of the threshold of TMB ≥10 mut/Mb. Our analysis showed that the median OS of patients with TMB-H was 2.5 times as long as that of the patients with TMB-L (25 vs. 10 months), with an area under the curve (AUC) value of 0.56 (95% CI 0.68–0.44) (Figures 2A,B). In other words, immunotherapy was more effective in r/m HNSCC patients with TMB-H (TMB ≥10 mut/Mb). In addition, we collected data from two other cohorts that included r/m HNSCC patients receiving no immunotherapy (Figures 2C,D). Results showed that the difference in median OS between the patients receiving no immunotherapy with TMB-H and those with TMB-L was significant in the Ho cohort (Figure 2C, p = 0.0043) and not significant in the Berger cohort (Figure 2D, p = 0.55). It reminded us that the significance of TMB stratification in non-ICI cohorts of HNSCC was still uncertain. Thus, we need to look for a comprehensive prognostic index other than TMB alone.
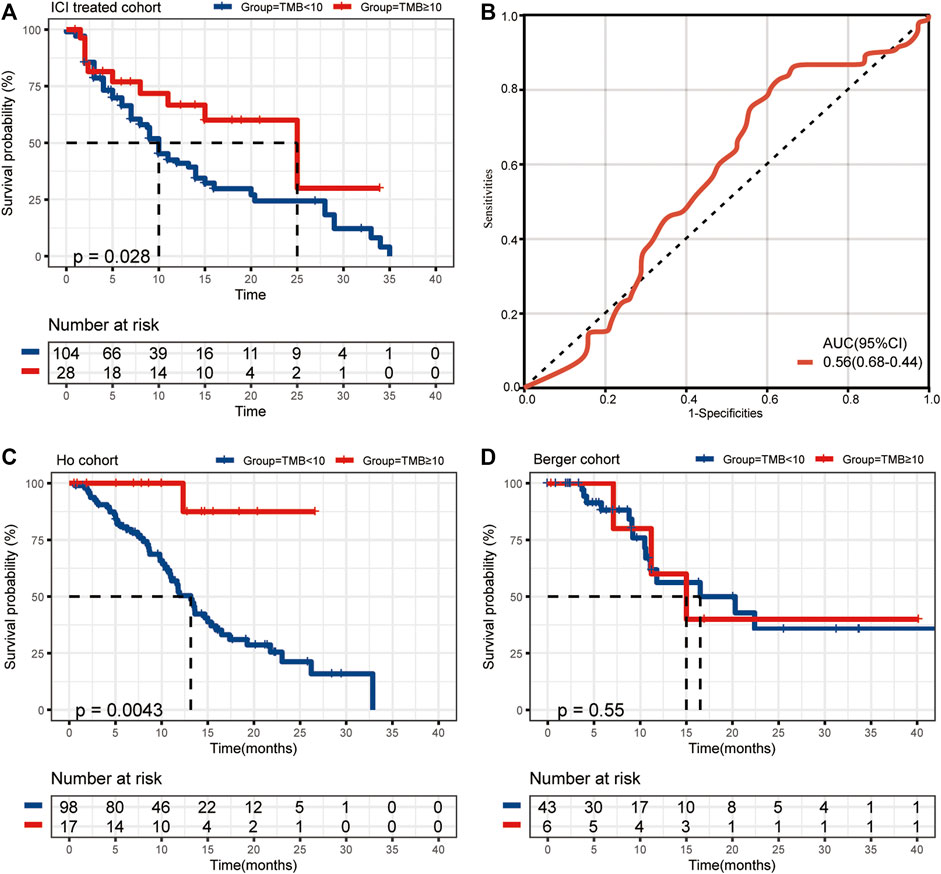
FIGURE 2. (A) The median OS of ICI-treated r/m HNSCC patients with high and low TMB (25 vs. 10 months, p = 0.028). (B) The ROC curve of TMB stratification. (C) The difference in median OS for non-ICI-treated HNSCC patients with high and low TMB (the Ho cohort, p = 0.0043). (D) The difference in median OS for non-ICI-treated HNSCC patients with high and low TMB (the Berger cohort, p = 0.55). OS, overall survival; TMB, tumor mutational burden; ICI, immune checkpoint inhibitor; r/m HNSCC, recurrent and metastatic head and neck squamous cell carcinoma; ROC, receiver operating characteristic.
Construction of TP-PR Model
The mutational landscape showed that the commonly mutated genes in r/m HNSCC patients from ICI-treated cohorts were TP53, TERT, PIK3CA, NOTCH1, FAT1, KMT2D, and CDKN2A (Supplementary Figure S1A). The flowchart of gene selection is shown in Supplementary Figure S1B. Twenty-seven genes with mutation frequency of no less than 5% were obtained from the results of mutational landscape. The correlation between those gene mutations and the OS was analyzed by K-M univariate analysis (Figures 3A–C; Supplementary Figure S2A–X). K-M univariate analysis showed that TP53, PIK3CA, ARID1A, ROS1, ATR, and ASXL1 might be related to the OS (p < 0.1) for r/m HNSCC patients who received immunotherapy. Then the six mutation genes and TMB were included for multivariate Cox regression analysis. Finally, the three genes, PIK3CA, TP53, and ROS1, were found to be associated with the OS (p < 0.05) (Figure 4). Among them, TMB-H or PIK3CA mutation was positively correlated with OS, while TP53 or ROS1 mutation was negatively correlated with OS. The prognostic index TP-PR (formula: TP-PR = TMB * 1.0803 + PIK3CA * 0.654 − TP53 * 0.5581 − ROS1 * 1.2342) was then constructed according to the expression of those three genes. It was scored 1 if TMB ≥10 mut/Mb or if the gene was mutated and was scored 0 if TMB <10 mut/Mb or if the gene was wide-type. The final score was calculated as the TP-PR score.
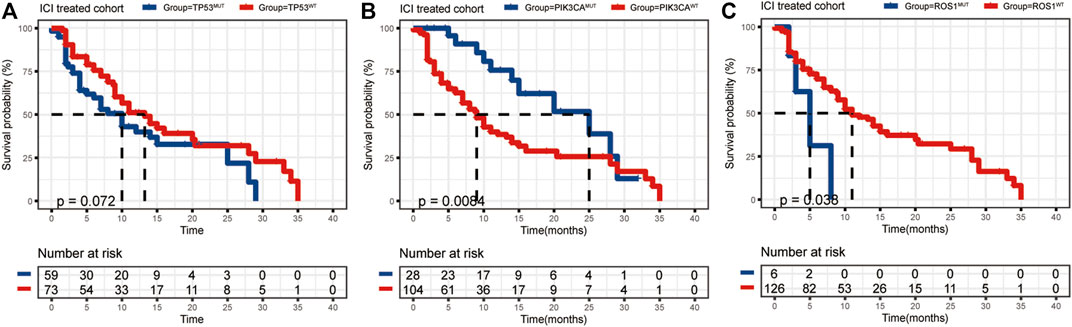
FIGURE 3. (A–C) K-M analysis of ICI-treated r/m HNSCC patients with TP53, PIK3CA, and ROS1 mutations. K-M, Kaplan–Meier; ICI, immune checkpoint inhibitor; r/m HNSCC, recurrent and metastatic head and neck squamous cell carcinoma.
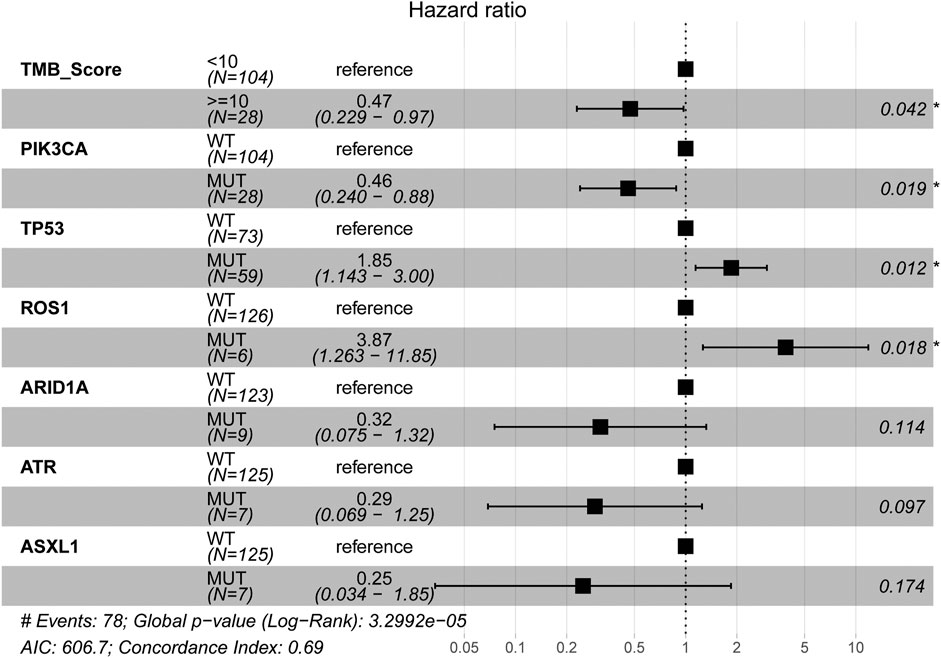
FIGURE 4. Multivariate Cox regression analysis of TMB and six genes, PIK3CA, TP53, ARID1A, ROS1, ATR, and ASXL1. TMB, tumor mutational burden.
TP-PR is Better Than Tumor Mutational Burden Alone to Screen Out More Recurrent and Metastatic Head and Neck Squamous Cell Carcinoma Patients Who Might Benefit From Immunotherapy
The data sets of r/m HNSCC patients who had received immunotherapy were divided into two groups: high TP-PR (TP-PR >0) and low TP-PR (TP-PR ≤0). We found that the high TP-PR group had better median OS (25 vs. 8 months) than the low TP-PR group, with a higher AUC value (0.77, 95% CI 0.86–0.68) than TMB stratification (Figures 5A,B). Moreover, the high TP-PR group screened out more people than the TMB-H group. In other words, TP-PR screened out more people who might benefit from immunotherapy than TMB. We further contrasted the mutational landscape of TP-PR high population and TMB-H population (Figure 6). We found that PIK3CA mutation rate was higher in the TP-PR high population, whereas TP53 and especially ROS1 (0 vs. 14%) mutation rates were higher in the TMB-H population. That was in accordance with our conclusion that PIK3CA mutation was positively correlated with OS, while TP53 or ROS1 mutation was negatively correlated with OS. And it might be the answer why TP-PR screened out more people who might benefit from immunotherapy than TMB alone. Gene mutation analysis was also performed between the high and low TP-PR groups. It was confirmed that the expressions of PIK3CA, TP53, and ROS1 were different in the high and low TP-PR groups; especially, those of PIK3CA and ROS1 were significantly different (data not shown). It supported that PIK3CA, TP53, and ROS1 were related to the prognosis of r/m HNSCC patients.
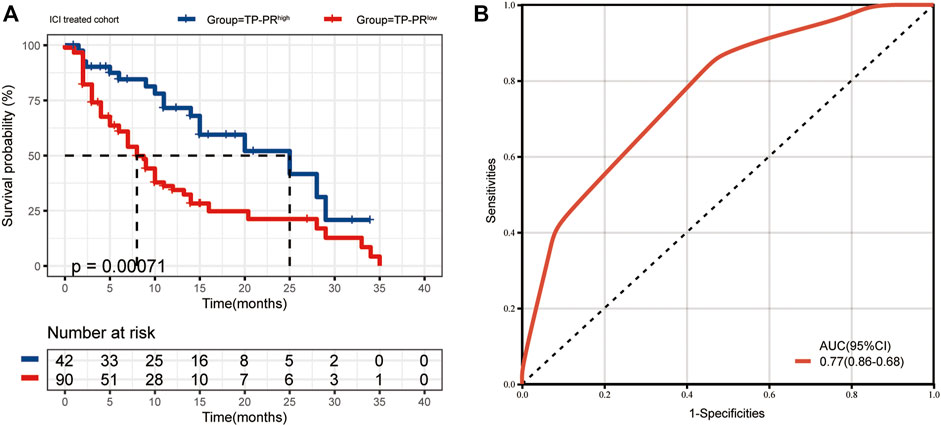
FIGURE 5. (A) The median OS of ICI-treated r/m HNSCC patients with high TP-PR and low TP-PR (25 vs. 8 months, p = 0.00071). (B) The ROC curve of TP-PR stratification. OS, overall survival; ICI, immune checkpoint inhibitor; r/m HNSCC, recurrent and metastatic head and neck squamous cell carcinoma; ROC, receiver operating characteristic.
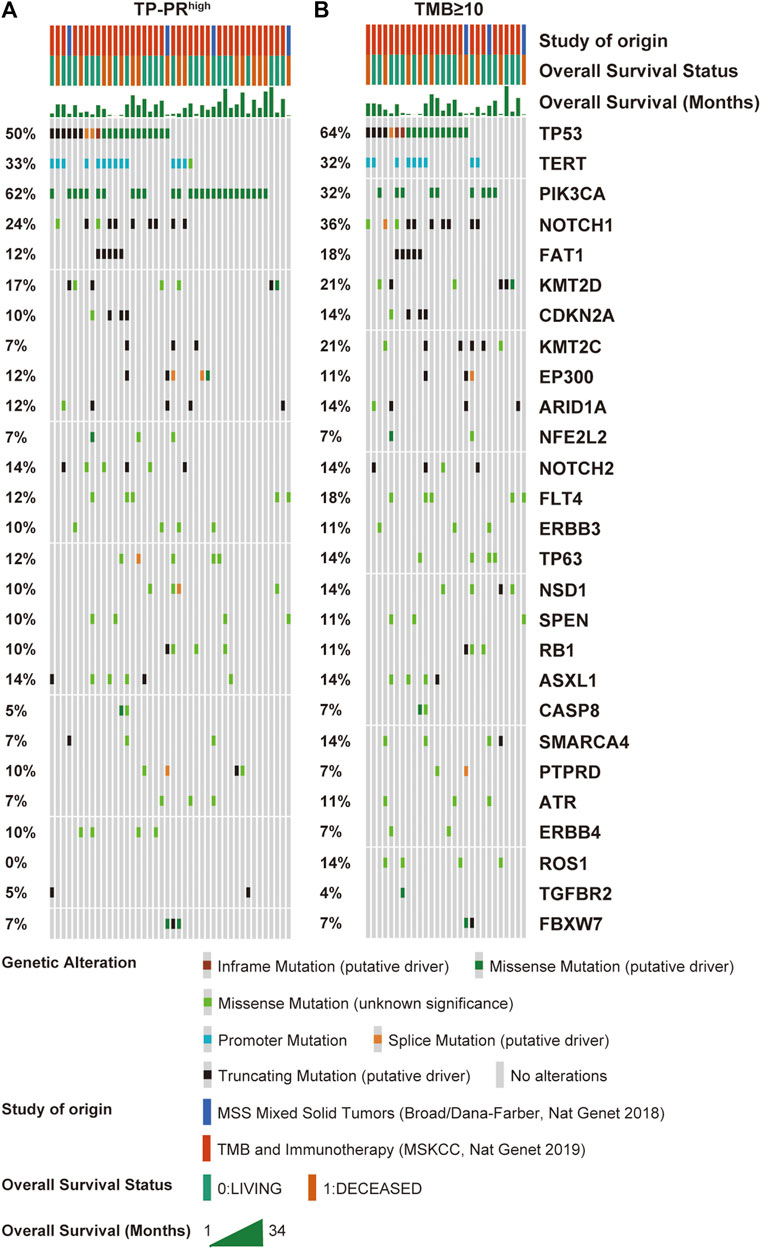
FIGURE 6. Mutational landscape of high TP-PR vs. high TMB in ICI-treated r/m HNSCC patients. Gene mutations (A) and their proportions (B) are shown. Specific mutation types are displayed in different colors and shown in the bottom panel. TMB, tumor mutational burden; ICI, immune checkpoint inhibitor; r/m HNSCC, recurrent and metastatic head and neck squamous cell carcinoma.
Analysis of Immune Infiltration and Pathway Enrichment in the Cancer Genome Atlas Cohort
TCGA cohort included 488 patients with HNSCC who had not received immunotherapy. RNA-seq analysis of TCGA cohort showed that CD8+ T cells and activated memory CD4+ T-cell infiltration in the tissues of the patients with high TP-PR were higher than those in the patients with low TP-PR, and the differences were statistically significant (Figure 7A). Infiltration of other immune cells was not significantly different between the two groups. It was suggested that high TP-PR might enhance CD8+ T cells and activated memory CD4+ T-cell infiltration, without affecting the mRNA expression of immunotherapy-related targets like LAG3, CTLA4, IDO1, and HAVCR2 (Figure 7B). But the PD-L1 (p = 0.047) and TIGIT (p = 0.026) mRNA expressions were higher in the high TP-PR group than in the low TP-PR group, indicating that high TP-PR might promote the mRNA expression of PD-L1 and TIGIT.
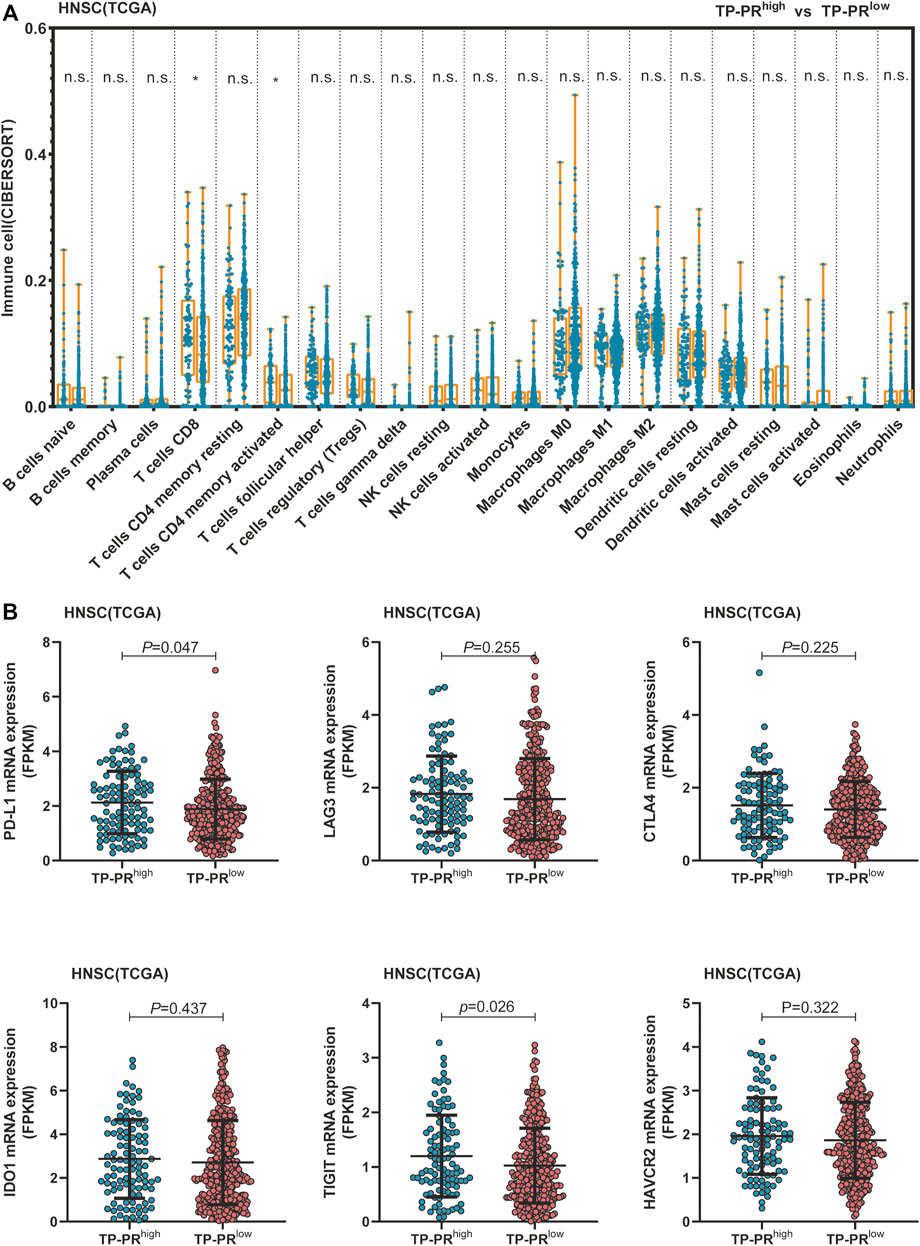
FIGURE 7. (A) Comparison of immune cell infiltration between the high and low TP-PR HNSCC patients in TCGA cohort. (B) mRNA expression of some immunotherapy-related targets in high TP-PR vs. low TP-PR HNSCC patients. HNSCC, head and neck squamous cell carcinoma; TCGA, The Cancer Genome Atlas.
Then we tried to find biological processes (BPs) and signaling pathways that might be relevant by gene ontology and Kyoto Encyclopedia of Genes and Genomes analyses. Figure 8 shows the 15 most relevant BPs or signaling pathways. It is suggested that the differential gene expression in the high and low TP-PR groups in TCGA cohort mainly influenced BP related to development and metabolism, and metabolism-related signaling pathways, such as the pathways for fatty acid metabolism, glycerolipid metabolism, and nitrogen metabolism.
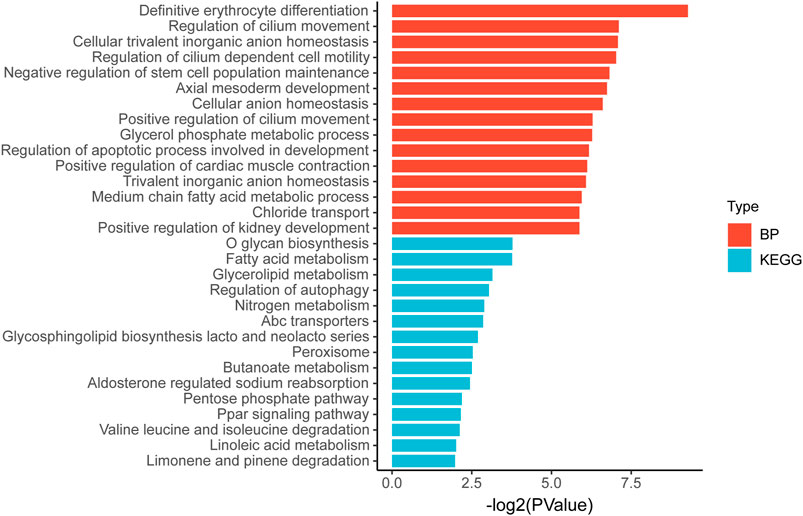
FIGURE 8. Gene ontology and Kyoto Encyclopedia of Genes and Genomes pathway enrichment analysis of differential gene expression in high and low TP-PR groups.
RNA-seq analysis also showed that CD8+ T cells and resting memory CD4+ T-cell infiltration in patients with TMB-H were higher than those in patients with TMB-L, with a statistically significant difference (Supplementary Figure S3A). But there was no difference in the mRNA expression of PD-L1, TIGIT, LAG3, CTLA4, IDO1, and HAVCR2 between the TMB-H and TMB-L groups (Supplementary Figure S3B). Compared with analysis stratified by TMB alone, patients with TP-PR stratification showed a difference in the mRNA expression of PD-L1 and TIGIT, which might influence the immune microenvironment. The differential gene expression in TMB-H and TMB-L groups in TCGA cohort also mainly influenced metabolism-related signaling pathways (Supplementary Figure S4).
Discussion
With immunotherapy now being included in the first-line treatment regimen for r/m HNSCC, finding appropriate biomarkers for predicting the efficacy of immunotherapy has become an urgent problem that needs to be solved. Positive expression of PD-L1 [combined positive score (CPS) ≥1] has become a concomitant indication for the use of pembrolizumab in HNSCC. The role of TMB in predicting the immunotherapy efficacy for r/m HNSCC has also not been well determined. Our analysis showed that immunotherapy was more effective in r/m HNSCC patients with TMB-H (TMB ≥ 10 mut/Mb), and the median OS of these patients was 2.5 times as long as that of the patients with TMB-L (25 vs. 10 months). However, the significance of TMB stratification in non-ICI cohorts of HNSCC was still uncertain. Although current evidence suggests that TMB is associated with increased efficacy of ICIs (Chen et al., 2020; Sha et al., 2020), the underlying mechanism linking TMB to immunotherapy benefits is not fully understood (Dos Santos et al., 2021). In addition, some studies have suggested that TMB is only useful for predicting the clinical response of some cancer patients (those with endometrial cancer, colorectal cancer, melanoma, lung adenocarcinoma, etc.) to ICIs, and some solid tumors with TMB-H failed to achieve the expected clinical effect (McGrail et al., 2018; Jiang et al., 2021; McGrail, 2021). Thus, we are looking for a comprehensive prognostic index involving genetic mutations that can predict the efficacy of immunotherapy in r/m HNSCC.
In our study, we screened out three genes PIK3CA, TP53, and ROS1, and we constructed an ICI-related gene prognostic index TP-PR, together with TMB, for r/m HNSCC. The median OS of the high TP-PR group (TP-PR >0) was three times that of the low TP-PR group (TP-PR ≤0) (25 vs. 8 months). That means that patients with high TP-PR may benefit from ICI treatment than patients with low TP-PR. What is more, the high TP-PR group screened out more people who might benefit from immunotherapy than the TMB-H group. And TP-PR stratification had a higher AUC value than TMB stratification. Thus, we believe that TP-PR is a better predictor of the prognosis of r/m HNSCC immunotherapy than TMB alone. Our analysis is directly based on the cohort of r/m HNSCC patients who received immunotherapy. Unfortunately, we currently have no other cohort to verify this, which is our limitation.
Among the mutated genes contained in TP-PR, PIK3CA and TP53 were common in patients with r/m HNSCC, while ROS1 was relatively rare. But the mutation rate of ROS1 in HNSCC in TCGA cohort is 5% (data not shown). Also, the ROS1 mutation rate in r/m HNSCC in Morris and Allen’s cohorts is 5%. Thus, we believe ROS1 is related to HNSCC and r/m HNSCC and might provide a direction for targeted drug selection in the future. Previously known HNSCC genes are TP53, CDKN2A, PTEN, PIK3CA, HRAS, NOTCH1, IRF6, TP63, etc. (Stransky et al., 2011). For r/m HNSCC, the known common mutated genes are TP53, TERT, CDKN2A, PIK3CA, FAT1, NOTCH1, etc. (Morris et al., 2017). Our study also showed that CDKN2A and NOTCH1 had high mutation rate in r/m HNSCC cohorts. However, CDKN2A or NOTCH1 mutation was not related to the prognosis of r/m HNSCC patients receiving immunotherapy. Thus, they were eliminated for further analysis.
Recent literature has shown that the combination of TMB and CNA or gene signature is more accurate than TMB alone in predicting ICI response. Patients with TMB-H and ADOBEC signature in breast cancer had sustained response to ICIs (Chumsri et al., 2020). Combining TMB and CNA can predict response and prognosis to immunotherapy in metastatic cancers or lung cancer (Liu et al., 2019; Xiang et al., 2020). In our study, PIK3CA, TP53, and ROS1 were found to be associated with the OS in the ICI-treated cohort. The prognostic index that combined TMB with those gene mutations is supposed to better screen out groups who may benefit from immunotherapy.
Our study suggested that the differential gene expression in the high and low TP-PR groups mainly influenced BP related to development and metabolism, and metabolism-related signaling pathways. However, the pathway enrichment was analyzed in non-ICI cohorts, instead of ICI cohorts. Due to lack of relevant data, we did not have information on gene expression in the ICI cohorts and therefore could not obtain the results of pathway enrichment in the ICI cohorts. That is a limitation of our study. At present, there are only a few studies on this topic (Zhang et al., 2020b; Feng and Hess, 2021), and we hope that our study can act as a reference for follow-up research for identifying the underlying pathways and for seeking a possible link between enriched metabolic pathways and immunotherapy efficacy.
In fact, there may be some correlation between TMB and the expression of PD-L1, but this correlation is not present consistently (Luchini et al., 2019; Yarchoan et al., 2019). TMB is a more complex biomarker, which is related to tumor neoantigens and represents the whole immune microenvironment. Therefore, TMB has increasingly become a sensitive prognostic factor in immunotherapy. At present, TMB is mainly detected by second-generation sequencing (Klempner et al., 2020). Adjustments and improvements to standardize genetic testing offered by different manufacturer are needed, so that patients can get an accurate prediction of immunotherapy efficacy irrespective of the manufacturer.
Another limitation of our study is that we found no related data for human papillomavirus (HPV)-16 or other data for HPV status in the cohorts. But our findings are important for stratification of r/m HNSCC patients receiving immunotherapy. Future studies of HPV status and the efficacy of immunotherapy in r/m HNSCC patients will be interesting.
In conclusion, our study showed that TP-PR could act as a predictor of immunotherapy efficacy in r/m HNSCC, and patients with high TP-PR (TP-PR >0) had a better survival benefit than the patients with low TP-PR (TP-PR ≤0). We hope that our study will help in selecting the patient population for which immunotherapy is effective in order to better implement individualized treatment strategy with precision.
Data Availability Statement
The original contributions presented in the study are included in the article/Supplementary Material. Further inquiries can be directed to the corresponding author.
Author Contributions
Conceptualization and methodology: Z-GL. Formal analysis, investigation, and data curation: RW, Y-PP, and Q-DL. Visualization and resources: X-WX, WW, X-MP, and X-TH. Writing—original draft: Y-PP. Writing—review and editing, supervision, and funding acquisition: Z-GL.
Funding
The study was funded by the Zhuhai City Medical and Health Technology Plan (Grant No. ZH2202200013HJL, awarded to Z-GL) and The Fundamental Research Funds for the Central Universities (Grant No. 19ykzd07, awarded to Z-GL).
Conflict of Interest
The authors declare that the research was conducted in the absence of any commercial or financial relationships that could be construed as a potential conflict of interest.
Publisher’s Note
All claims expressed in this article are solely those of the authors and do not necessarily represent those of their affiliated organizations, or those of the publisher, the editors, and the reviewers. Any product that may be evaluated in this article, or claim that may be made by its manufacturer, is not guaranteed or endorsed by the publisher.
Supplementary Material
The Supplementary Material for this article can be found online at: https://www.frontiersin.org/articles/10.3389/fgene.2021.756506/full#supplementary-material
References
Bray, F., Ferlay, J., Soerjomataram, I., Siegel, R. L., Torre, L. A., and Jemal, A. (2018). Global Cancer Statistics 2018: GLOBOCAN Estimates of Incidence and Mortality Worldwide for 36 Cancers in 185 Countries. CA: A Cancer J. Clinicians 68 (6), 394–424. doi:10.3322/caac.21492
Burtness, B., Harrington, K. J., Greil, R., Soulières, D., Tahara, M., de Castro, G., et al. (2019). Pembrolizumab Alone or with Chemotherapy versus Cetuximab with Chemotherapy for Recurrent or Metastatic Squamous Cell Carcinoma of the Head and Neck (KEYNOTE-048): a Randomised, Open-Label, Phase 3 Study. Lancet 394 (10212), 1915–1928. doi:10.1016/S0140-6736(19)32591-7
Chan, T. A., Yarchoan, M., Jaffee, E., Swanton, C., Quezada, S. A., Stenzinger, A., et al. (2019). Development of Tumor Mutation burden as an Immunotherapy Biomarker: Utility for the Oncology Clinic. Ann. Oncol. 30 (1), 44–56. doi:10.1093/annonc/mdy495
Chen, Y., Wang, Y., Luo, H., Meng, X., Zhu, W., Wang, D., et al. (2020). The Frequency and Inter-relationship of PD-L1 Expression and Tumour Mutational burden across Multiple Types of Advanced Solid Tumours in China. Exp. Hematol. Oncol. 9, 17. doi:10.1186/s40164-020-00173-3
Chumsri, S., Sokol, E. S., Soyano-Muller, A. E., Parrondo, R. D., Reynolds, G. A., Nassar, A., et al. (2020). Durable Complete Response with Immune Checkpoint Inhibitor in Breast Cancer with High Tumor Mutational Burden and APOBEC Signature. J. Natl. Compr. Canc Netw. 18 (5), 517–521. doi:10.6004/jnccn.2020.7543
Dos Santos, L. V., Abrahão, C. M., and William, W. N. (2021). Overcoming Resistance to Immune Checkpoint Inhibitors in Head and Neck Squamous Cell Carcinomas. Front. Oncol. 11, 596290. doi:10.3389/fonc.2021.596290
Feng, B., and Hess, J. (2021). Immune-Related Mutational Landscape and Gene Signatures: Prognostic Value and Therapeutic Impact for Head and Neck Cancer. Cancers (Basel) 13 (5), 1162. doi:10.3390/cancers13051162
Goodman, A. M., Kato, S., Bazhenova, L., Patel, S. P., Frampton, G. M., Miller, V., et al. (2017). Tumor Mutational Burden as an Independent Predictor of Response to Immunotherapy in Diverse Cancers. Mol. Cancer Ther. 16 (11), 2598–2608. doi:10.1158/1535-7163.mct-17-0386
Jiang, A.-M., Ren, M.-D., Liu, N., Gao, H., Wang, J.-J., Zheng, X.-Q., et al. (2021). Tumor Mutation Burden, Immune Cell Infiltration, and Construction of Immune-Related Genes Prognostic Model in Head and Neck Cancer. Int. J. Med. Sci. 18 (1), 226–238. doi:10.7150/ijms.51064
Johnson, D. E., Burtness, B., Leemans, C. R., Lui, V. W. Y., Bauman, J. E., and Grandis, J. R. (2020). Head and Neck Squamous Cell Carcinoma. Nat. Rev. Dis. Primers 6 (1), 92. doi:10.1038/s41572-020-00224-3
Klempner, S. J., Fabrizio, D., Bane, S., Reinhart, M., Peoples, T., Ali, S. M., et al. (2020). Tumor Mutational Burden as a Predictive Biomarker for Response to Immune Checkpoint Inhibitors: A Review of Current Evidence. Oncologist 25 (1), e147–e159. doi:10.1634/theoncologist.2019-0244
Leemans, C. R., Snijders, P. J. F., and Brakenhoff, R. H. (2018). The Molecular Landscape of Head and Neck Cancer. Nat. Rev. Cancer 18 (5), 269–282. doi:10.1038/nrc.2018.11
Liu, L., Bai, X., Wang, J., Tang, X.-R., Wu, D.-H., Du, S.-S., et al. (2019). Combination of TMB and CNA Stratifies Prognostic and Predictive Responses to Immunotherapy across Metastatic Cancer. Clin. Cancer Res. 25 (24), 7413–7423. doi:10.1158/1078-0432.ccr-19-0558
Luchini, C., Bibeau, F., Ligtenberg, M. J. L., Singh, N., Nottegar, A., Bosse, T., et al. (2019). ESMO Recommendations on Microsatellite Instability Testing for Immunotherapy in Cancer, and its Relationship with PD-1/pd-L1 Expression and Tumour Mutational burden: a Systematic Review-Based Approach. Ann. Oncol. 30 (8), 1232–1243. doi:10.1093/annonc/mdz116
Marabelle, A., Fakih, M., Lopez, J., Shah, M., Shapira-Frommer, R., Nakagawa, K., et al. (2020). Association of Tumour Mutational burden with Outcomes in Patients with Advanced Solid Tumours Treated with Pembrolizumab: Prospective Biomarker Analysis of the Multicohort, Open-Label, Phase 2 KEYNOTE-158 Study. Lancet Oncol. 21 (10), 1353–1365. doi:10.1016/s1470-2045(20)30445-9
McGrail, D. J., Federico, L., Li, Y., Dai, H., Lu, Y., Mills, G. B., et al. (2018). Multi-omics Analysis Reveals Neoantigen-independent Immune Cell Infiltration in Copy-Number Driven Cancers. Nat. Commun. 9 (1), 1317. doi:10.1038/s41467-018-03730-x
McGrail, D. J. (2021). High Tumor Mutation burden Fails to Predict Immune Checkpoint Blockade Response across All Cancer Types. Ann. Oncol. 32, 661. doi:10.1016/j.annonc.2021.02.006
Miao, D., Margolis, C. A., Vokes, N. I., Liu, D., Taylor-Weiner, A., Wankowicz, S. M., et al. (2018). Genomic Correlates of Response to Immune Checkpoint Blockade in Microsatellite-Stable Solid Tumors. Nat. Genet. 50 (9), 1271–1281. doi:10.1038/s41588-018-0200-2
Morris, L. G. T., Chandramohan, R., West, L., Zehir, A., Chakravarty, D., Pfister, D. G., et al. (2017). The Molecular Landscape of Recurrent and Metastatic Head and Neck Cancers. JAMA Oncol. 3 (2), 244–255. doi:10.1001/jamaoncol.2016.1790
Samstein, R. M., Lee, C.-H., Shoushtari, A. N., Hellmann, M. D., Shen, R., Janjigian, Y. Y., et al. (2019). Tumor Mutational Load Predicts Survival after Immunotherapy across Multiple Cancer Types. Nat. Genet. 51 (2), 202–206. doi:10.1038/s41588-018-0312-8
Sha, D., Jin, Z., Budczies, J., Kluck, K., Stenzinger, A., and Sinicrope, F. A. (2020). Tumor Mutational Burden as a Predictive Biomarker in Solid Tumors. Cancer Discov. 10 (12), 1808–1825. doi:10.1158/2159-8290.cd-20-0522
Stransky, N., Egloff, A. M., Tward, A. D., Kostic, A. D., Cibulskis, K., Sivachenko, A., et al. (2011). The Mutational Landscape of Head and Neck Squamous Cell Carcinoma. Science 333 (6046), 1157–1160. doi:10.1126/science.1208130
Thorsson, V., Gibbs, D. L., Brown, S. D., Wolf, D., Bortone, D. S., Ou Yang, T. H., et al. (2018). The Immune Landscape of Cancer. Immunity 48 (4), 812. doi:10.1016/j.immuni.2018.03.023
Valero, C. (2021). Response Rates to Anti-PD-1 Immunotherapy in Microsatellite-Stable Solid Tumors with 10 or More Mutations Per Megabase. JAMA Oncol. 7, 739. doi:10.1001/jamaoncol.2020.7684
Xiang, L., Fu, X., Wang, X., Li, W., Zheng, X., Nan, K., et al. (2020). A Potential Biomarker of Combination of Tumor Mutation Burden and Copy Number Alteration for Efficacy of Immunotherapy in KRAS-Mutant Advanced Lung Adenocarcinoma. Front. Oncol. 10, 559896. doi:10.3389/fonc.2020.559896
Yarchoan, M., Albacker, L. A., Hopkins, A. C., Montesion, M., Murugesan, K., Vithayathil, T. T., et al. (2019). PD-L1 Expression and Tumor Mutational burden Are Independent Biomarkers in Most Cancers. JCI Insight 4 (6), 908. doi:10.1172/jci.insight.126908
Zehir, A., Benayed, R., Shah, R. H., Syed, A., Middha, S., Kim, H. R., et al. (2017). Erratum: Mutational Landscape of Metastatic Cancer Revealed from Prospective Clinical Sequencing of 10,000 Patients. Nat. Med. 23 (8), 1004. doi:10.1038/nm0817-1004c
Zhang, L., Li, B., Peng, Y., Wu, F., Li, Q., Lin, Z., et al. (2020). The Prognostic Value of TMB and the Relationship between TMB and Immune Infiltration in Head and Neck Squamous Cell Carcinoma: A Gene Expression-Based Study. Oral Oncol. 110, 104943. doi:10.1016/j.oraloncology.2020.104943
Zhang, Y., Lin, A., Li, Y., Ding, W., Meng, H., Luo, P., et al. (2020). Age and Mutations as Predictors of the Response to Immunotherapy in Head and Neck Squamous Cell Cancer. Front. Cel. Dev. Biol. 8, 608969. doi:10.3389/fcell.2020.608969
Keywords: tumor mutational burden, gene mutation, immunotherapy, survival prognosis, recurrent and metastatic head and neck squamous cell carcinoma
Citation: Peng Y-P, Wang R, Liu Q-D, Xu X-W, Wei W, Huang X-T, Peng X-M and Liu Z-G (2021) Combination of Tumor Mutational Burden and Specific Gene Mutations Stratifies Outcome to Immunotherapy Across Recurrent and Metastatic Head and Neck Squamous Cell Carcinoma. Front. Genet. 12:756506. doi: 10.3389/fgene.2021.756506
Received: 11 August 2021; Accepted: 14 October 2021;
Published: 16 November 2021.
Edited by:
Hauke Busch, University of Lübeck, GermanyReviewed by:
Michael Poidinger, Murdoch Childrens Research Institute, AustraliaRifat Hamoudi, University of Sharjah, United Arab Emirates
Copyright © 2021 Peng, Wang, Liu, Xu, Wei, Huang, Peng and Liu. This is an open-access article distributed under the terms of the Creative Commons Attribution License (CC BY). The use, distribution or reproduction in other forums is permitted, provided the original author(s) and the copyright owner(s) are credited and that the original publication in this journal is cited, in accordance with accepted academic practice. No use, distribution or reproduction is permitted which does not comply with these terms.
*Correspondence: Zhi-Gang Liu, liuzhg9@mail.sysu.edu.cn, zhigangliu1983@hotmail.com
†These authors have contributed equally to this work