- 1Department of Zoology, Faculty of Biological Sciences, Kohat University of Science and Technology, Kohat, Pakistan
- 2Department of Zoology, University of Peshawar, Peshawar, Pakistan
- 3Department of Zoology, Hazara University, Mansehra, Pakistan
- 4Department of Biosciences, COMSATS University Islamabad, Islamabad, Pakistan
- 5College of Veterinary Sciences and Animal Husbandry, Abdul Wali Khan University Garden Campus Mardan, Mardan, Pakistan
Background: Understanding the genetic diversity of Plasmodium species through polymorphic studies can assist in designing more effective control strategies of malaria like new drug formulation and development of a vaccine. Pakistan is moderate endemic for Plasmodium falciparum, but little is known about the genetic diversity of this parasite. This study aimed to investigate the molecular diversity of P. falciparum based on msp-1 and msp-2 genes in the malaria-endemic regions of Khyber Pakhtunkhwa, Pakistan.
Methods: A total of 199/723 blood samples, tested positive by microscopy for falciparum malaria, were collected from four districts (Dera Ismail Khan, Karak, Mardan, and Peshawar) of Khyber Pakhtunkhwa. Nested PCR amplification technique was employed to target block 2 of msp-1 and the central domain of msp-2 genes, including their respective allelic families K1, MAD20, RO33, FC27, and 3D7/IC, and to detect the extent of genetic diversity of P. falciparum clinical isolates.
Results: Among the 199 microscopy-positive P. falciparum samples, a total of 192 were confirmed using PCR. Ninety-seven amplicons were observed for msp-1 and 95 for msp-2. A total of 33 genotypes, 17 for msp-1 (eight K1, six MAD20, and three RO33) and 16 for msp-2 (nine FC27 and seven 3D7/IC), were identified. The specific allelic frequency of the K1 family was higher (44.3%) than that of MAD20 (33.0%) and RO33 (23.0%) for msp-1, while the FC27 allelic family was dominant (60.0%) compared with 3D7/IC (40.0%) for msp-2. No polyclonal infection was observed in msp-1 and msp-2. The expected heterozygosity was 0.98 and 0.97 for msp-1 and msp-2, respectively.
Conclusion: It was concluded that the P. falciparum populations are highly polymorphic, and diverse allelic variants of msp-1 and msp-2 are present in Khyber Pakhtunkhwa, Pakistan.
Introduction
Malaria causes 300–500 million cases worldwide and approximately 0.5–3 million deaths annually, the majority of which are caused by Plasmodium falciparum (Phillips, 2001; Ferreira et al., 2004). Pakistan is a moderate malaria-endemic region, and approximately 60% of its population is living in the endemic areas, whereas 177 million individuals are at risk of malaria (Qureshi et al., 2019). Annually, the estimated number of suspected and confirmed individuals is 3.5 million in Pakistan. The WHO included Pakistan as one of the six Eastern Mediterranean region countries with almost 100% population at risk of malaria (WHO, 2017; 2018). The endemicity of malaria varies in different provinces and even in different cities having variable climates. In 2017, about 30% of total malaria cases were reported from Khyber Pakhtunkhwa only, and the province has the highest reported cases of malaria (WHO, 2017).
The National Malaria Control Programmes (NMCP) has reported a record sixfold increase in P. falciparum during the last decade. The falciparum malaria has acknowledged sparse scientific attention, particularly concerning the molecular characterization of the local parasite population. The rise of P. falciparum in various districts of Pakistan may be attributable to the failed treatment of chloroquine resistance (Nizamani et al., 2006). Besides that, the heavy influx and continued presence of refugees from Afghanistan, where malaria is most prevalent, may contribute not only to the increasing number of cases of malaria but also to its genetic variations in Khyber Pakhtunkhwa province (Murtaza et al., 2004; Sheikh et al., 2005; Howard et al., 2011).
Analyses of the genotypes of Plasmodium spp. by PCR have remarkably improved our understanding of the biology of these parasites. In this regard, genetically distinct P. falciparum has been identified and extensively studied to further unveil its molecular epidemiology, parasite resistance, and potential vaccine candidates (Diggs et al., 1993; Greenhouse et al., 2006). The mainly used genetic markers of P. falciparum are the merozoite surface protein 1 (msp-1), merozoite surface protein 2 (msp-2), and glutamate-rich protein (glurp) (Smythe et al., 1991; Snounou and Beck, 1998). Allelic forms of these polymorphic markers have been reported in various parts of the world (Babiker et al., 1997; Jordan et al., 2001).
The msp-1 and msp-2 are antigenic proteins responsible for immunological responses in humans (Taylor et al., 1995; Aubouy et al., 2003). Block 2 of msp-1 has three polymorphic allelic families identified as MAD20, K1, and RO33 (Contamin et al., 1996). Similarly, the central domain of the msp-2 has two distinct families, i.e., 3D/IC and FC27 (Sallenave-Sales et al., 2000). These markers are unlinked and located on different chromosomes (Färnert et al., 2001). These features make them attractive candidates for studies where identification and enumeration of genetically distinct P. falciparum parasite subpopulations are of interest. As such, they have proven to be useful tools in molecular epidemiology studies in different epidemiological settings as well as to distinguish treatment failures from new infections in antimalarial drug trials (Cattamanchi et al., 2003; Collins et al., 2006).
The genetic diversity of P. falciparum population is an important indicator of the malaria transmission intensity in an area (Babiker et al., 1995; Paul et al., 1998). A high endemic area is generally characterized by extensive parasite diversity, and infected humans often carry multiple genotypes. Conversely, the parasite population in a low transmission area has a limited genetic diversity, and most infections are monoclonal (Babiker et al., 1997; Haddad et al., 1999; Peyerl-Hoffmann et al., 2001; Gómez et al., 2002). The P. falciparum field isolates have been characterized in Afghanistan, Iran, and India and previously from Sindh and Baluchistan in Pakistan, using the above-mentioned molecular markers. Therefore, we investigated the genetic diversity and polymorphic nature of P. falciparum isolates in selective districts of the malaria endemic province Khyber Pakhtunkhwa of Pakistan.
Materials and Methods
Ethics and Consent for Participation
The study protocol was approved by the Institutional Ethical Review Committee of Kohat University of Science and Technology (KUST), Kohat-26000, Pakistan. Signed and written informed consent was obtained from the participants/legal guardians before sample collection.
Sample Collection and Analysis
Blood samples were randomly collected from suspected individuals ≥1 year at the Malaria Control Laboratories of District Headquarters Hospitals (DHQs) in four districts of Khyber Pakhtunkhwa (Dera Ismail Khan, Karak, Peshawar, and Mardan). A total of 723 suspected individuals with fever or history of fever were screened. Finger-pricked blood was collected on a glass-slide to prepare thick and thin blood smears, air-dried, and stained with Giemsa’s stain (10%) for 15 min. The slides were examined under a microscope (Olympus CX31, Tokyo, Japan) by experienced laboratory technicians for Plasmodium species-specific identification. After careful examination, blood smears were considered negative when no parasite was detected and vice versa. Among the screened individuals, a total of 199 were confirmed positive for P. falciparum infection by microscopy.
Additionally, 200 μl of blood was collected into EDTA tubes, labelled, and transferred to the Molecular Parasitology and Virology Laboratory, Department of Zoology, KUST, Kohat-26000, Khyber Pakhtunkhwa, Pakistan, and stored at −80°C in a low deep freezer until genomic DNA extraction. Furthermore, a brief epidemiological/demographic history was also recorded using a structured questionnaire. The demographic data will be published elsewhere.
DNA Extraction and PCR Amplification
Genomic DNA was extracted from blood using the GF1 DNA extraction kit (Vivantis, Shah Alam, Selangor). Nested PCR was recruited to determine Plasmodium species with the help of 18S rRNA amplification using primers rPLUf: 5′-TTAAAATTGTTGCAGTTAAAACG-3′ and rPLUr: 5′-CCTGTTGTTGCCTTAAACTTC-3′ as previously described (Snounou et al., 1993; Singh et al., 1999). The amplicons were used as a template in the nested PCR; and species-specific primers rFALf: 5′-TTAAACTGGTTTGGGAAAACCAAATATATT-3′ and rFALr: 5′-ACACAATGAACTCAATCATGACTACCCGTC-3′ were employed for P. falciparum detection (Snounou et al., 1993). Deionized water and genomic DNA from laboratory strains were used as negative and positive control, respectively. Both primary and secondary PCRs were carried out in a final volume of 20 μl containing 5.0 μl of DNA, 0.4 μl of 10 mM of each dNTP (Fermentas, Hanover, MD, USA), 2.4 μl of 10× PCR buffer, 1.5 μl of 25 mM MgCl2, 0.5 μl of 5 U/μl Taq DNA polymerase (Fermentas, Hanover, MD, USA), paired primers of 1.0 μl each (20 μM), and 8.2 μl of sterile water. Thermocycler (Nyxtech, Boston, MA, USA) was used to complete all the reactions under the following conditions for primary (35 cycles) and secondary PCR (30 cycles): initial denaturation at 94°C for 1 min, extension at 94°C for 1 min, 58°C for 2 min and 72°C for 5 min, and final elongation at 72°C for 5 min.
The msp-1 and msp-2 genes were amplified using specific primers as per standard protocol previously described (Snounou et al., 1999). The primary reaction used a set of primers corresponding to the conserved regions of block 2 for msp-1 and block 3 for msp-2. The second reaction primer set targets specific allelic families of msp-1 (KI, MAD20, and RO33) or msp-2 (3D7/IC and FC27). The cycling conditions for both msp-1 and msp-2 as well as primers were previously described (Somé et al., 2018).
Agarose gel (2%) stained with ethidium bromide was used to evaluate the PCR products under UV illumination. A 50 and 100-bp DNA ladders (Promega, Madison, WI, USA) were used; and alleles of msp-1 and msp-2 were categorized according to their molecular weights.
Statistical Analysis
Primarily, Microsoft Excel was used to manage the data. Statistical analyses were performed using SPSS (Version 20). The allelic frequencies for msp-1 and msp-2 were calculated and expressed in percentages. The proportion of alleles observed at each locus was compared using a chi-square test. The expected heterozygosity (He) was calculated using the following formula: He = [n/(n − 1)] [(1 − Σpi2)], where n is the number of isolates sampled and pi is the allele frequency at a given locus (Nei, 1978). The p-value (0.05) was assumed to be statistically significant.
Results
Out of 199 microscopy-positive samples, 192 were further confirmed for P. falciparum using PCR, whereas seven samples (3.2%) were excluded due to negative PCR outcome (Figure 1).
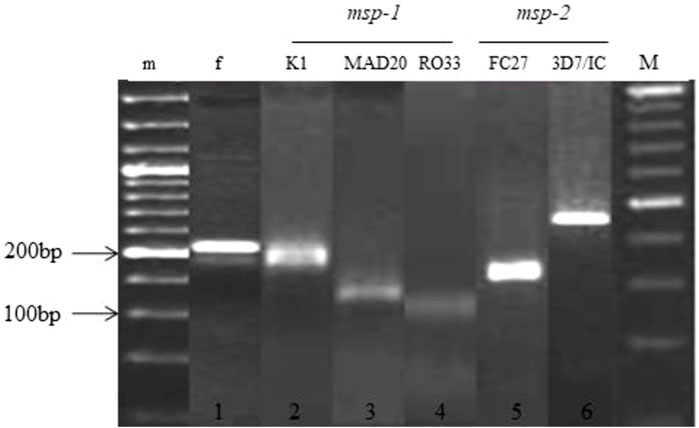
FIGURE 1. Allelic families of msp-1 and msp-2 genes of Plasmodium falciparum 1 show the positive infection of Plasmodium falciparum; 2, 3, and 4 represent the corresponding alleles of msp-1; 5 and 6 show the alleles of msp-2; m is a 50-bp DNA ladder marker, and M is a 100-bp DNA ladder marker.
Out of total, 97 amplicons were observed for msp-1 and 95 for msp-2. In msp-1, the highest frequency of K1, MAD20, and RO33 was 44.3% (43/97), 33.0% (32/97), and 23.0% (22/97), respectively (Table 1). In msp-2, the FC27 has the highest frequency of 60.0% (57/95), followed by 3D7/IC with 40.0% (38/95) (Table 2). However, no polyclonal infection was observed in msp-1 and msp-2.
Seventeen alleles were detected in msp-1 comprising eight alleles (180–350 bp) for K1, six alleles (110–250 bp) for MAD20, and three alleles (130–190 bp) for RO33. However, 16 alleles were attributed to msp-2, with nine alleles (400–580 bp) for FC27 and seven alleles (300–430 bp) for 3D7/IC. The district-wise details of allelic frequencies are presented in the supplementary materials (Supplementary Tables S1, S2). The expected heterozygosity was 0.98 and 0.97 for msp-1 and msp-2, respectively.
Discussion
Molecular studies provide an insight into the transmission intensity and genetic variation of parasite population within a region. The genetic diversity may be linked with the cross-border movement of populations living in the Frontier Regions of Pakistan (Ghanchi et al., 2010; Zakeri et al., 2010). Usually, areas with high malaria transmission are observed to have an extensive genetic diversity (Paul et al., 1998; Peyerl-Hoffmann et al., 2001). This study provides the basis to explore the genetic diversity of P. falciparum in the endemic regions of Khyber Pakhtunkhwa.
In the present study, the number of successfully genotyped samples for msp-1 was higher as compared with that for msp-2. This result is consistent with studies reported from Cote d’Ivoire and Gabon (Yavo et al., 2016) and Burkina Faso (Soulama et al., 2009; Somé et al., 2018). However, in contrast, Mohammad et al. (2019) from Ethiopia, Soe et al. (2017) from Myanmar, and A-Elbasit et al. (2007) from Sudan reported a relatively high frequency of msp-2 genotyped samples. Furthermore, of 192 positive samples for P. falciparum, less than half showed an amplicon for msp-1 (n = 97) or msp-2 (n = 95). In previous studies, relatively higher numbers of amplicons were observed for msp-1 or msp-2 (Somé et al., 2018; Mohammed et al., 2019; Eltayeb et al., 2020; Papa Mze et al., 2020). The nonspecific binding during P. falciparum identification by PCR may justify the smaller number of amplicons for msp-1 and msp-2 in the current study.
It was observed that 17 allelic variants of msp-1 and 16 of msp-2 were present in the studied areas. This result is in comparison with a similar study from the south of Pakistan (Ghanchi et al., 2010), Iran, South Africa, Myanmar, Sudan, Senegal, and Thailand (Heidari et al., 2007; Soe et al., 2017; Somé et al., 2018; Ndiaye et al., 2019; Eltayeb et al., 2020). However, in two southern districts Bannu and Kohat of Khyber Pakhtunkhwa, less allelic variants of P. falciparum were reported (Khatoon et al., 2010; Khatoon et al., 2012). Similarly, a study from the hypo-endemic area of Colombia reported only one allele of msp-1 and three alleles of msp-2 (Montoya et al., 2003). This difference might be due to low malaria endemicity since higher allele frequencies have been reported with high malaria transmission (Konaté et al., 1999; Soulama et al., 2009), suggesting that malaria endemicity affects the circulating strain number. However, high genetic diversity was observed in the Kingdom of Eswatini, which is regarded as a low transmission area for P. falciparum (Roh et al., 2019). Therefore, the genetic diversity may also be attributed to the effects of several factors such as indiscriminate use of long-lasting insecticide-treated nets (LLINs), indoor insecticide spraying, and antimalarial pressure (Soulama et al., 2009).
The K1 and FC27 alleles of msp-1 and msp-2 were predominant, respectively. The K1 and FC27 allelic families were previously reported from Kohat district (Khatoon et al., 2012). Nevertheless, the present findings also showed slight discrepancy with the previous studies (Zakeri et al., 2005; Ghanchi et al., 2010). The current study and previous findings suggest that K1 and FC27 allelic families might have prominent roles in clinical malaria at least in southern Khyber Pakhtunkhwa. However, further in-depth investigation with larger dataset should be carried out to unveil the genetic diversity and prevailing genotypes.
Interestingly, no polyclonal infection was detected in the present study. Malaria treatment policies, geographic isolation, and transmission intensities may result in spatial heterogeneity of P. falciparum (Khatoon et al., 2010). Most importantly, the use of highly potent antiplasmodial drugs that kill the asexual blood stage parasites and gametocytes are more likely to decrease parasite transmission and clonal diversity (Targett et al., 2001; Greenwood et al., 2008). However, in the adjacent districts (Bannu and Kohat) of Khyber Pakhtunkhwa, polyclonal infections were reported previously (Khatoon et al., 2010; Khatoon et al., 2012). It is worth mentioning that these two districts (Bannu and Kohat) accommodated one million internally displaced persons (IDPs) from tribal areas of North Waziristan Agency (NWA) sharing its border with Afghanistan, which may have introduced new genetically distinct variants of P. falciparum. It shows that the huge influx and migration of people between the study area and the neighboring countries like Iran and especially Afghanistan may introduce different alleles of P. falciparum into Khyber Pakhtunkhwa province of Pakistan.
Malaria transmission in Pakistan is markedly seasonal and prone to outbreaks, in particular geographical areas, especially Khyber Pakhtunkhwa, Baluchistan, and Sindh province (Yasinzai and Kakarsulemankhel, 2009). Pakistan is considered to be endemic for malaria, but the precise data on the genetic diversity of malaria in Pakistan are still lacking (Khan et al., 2005). As limitations, a small number of samples were amplified for msp-1 and msp-2, and the use of nested PCR instead of DNA sequencing could possibly underestimate the genetic diversity. Therefore, studies with larger datasets and more robust techniques should be used to explore the genetic diversity in the future. Furthermore, only microscopy-positive samples for P. falciparum were further subjected to nested PCR, which is another limitation of the present study.
Conclusion
It was concluded that extensively diverse and polymorphic P. falciparum populations of merozoite surface protein 1 (msp-1) and 2 (msp-2) are present in Khyber Pakhtunkhwa, Pakistan.
Data Availability Statement
The datasets presented in this study can be found in online repositories. The names of the repository/repositories and accession number(s) can be found in the article/Supplementary Material.
Ethics Statement
The studies involving human participants were reviewed and approved by the recommendation of the Institutional Ethical Review Committee of Kohat University of Science and Technology (KUST), Kohat-26000, Pakistan. Written informed consent to participate in this study was provided by the participants’ legal guardian/next of kin.
Author Contributions
SNK, IA, and SA designed the research study. IA and SA supervised the study. SNK collected and analyzed the data. SNK and RA drafted the manuscript. MR and SN helped during literature search, text incorporation, and table and graph designing. SK, SZ, and RA provided critical comments for the improvement of the manuscript. Finally, all the authors have read and approved the final manuscript.
Conflict of Interest
The authors declare that the research was conducted in the absence of any commercial or financial relationships that could be construed as a potential conflict of interest.
Publisher’s Note
All claims expressed in this article are solely those of the authors and do not necessarily represent those of their affiliated organizations, or those of the publisher, the editors, and the reviewers. Any product that may be evaluated in this article, or claim that may be made by its manufacturer, is not guaranteed or endorsed by the publisher.
Supplementary Material
The Supplementary Material for this article can be found online at: https://www.frontiersin.org/articles/10.3389/fgene.2021.751552/full#supplementary-material
References
A-Elbasit, I. E., ElGhazali, G., A-Elgadir, T. M. E., Hamad, A. A., Babiker, H. A., Elbashir, M. I., et al. (2007). Allelic Polymorphism of MSP2 Gene in Severe P. Falciparum Malaria in an Area of Low and Seasonal Transmission. Parasitol. Res. 102 (1), 29–34. doi:10.1007/s00436-007-0716-3
Aubouy, A., Migot-Nabias, F., and Deloron, P. (2003). Polymorphism in Two Merozoite Surface Proteins of Plasmodium Falciparum Isolates from Gabon. Malar. J. 2, 12. doi:10.1186/1475-2875-2-12
Babiker, H. A., Charlwood, J. D., Smith, T., and Walliker, D. (1995). Gene Flow and Cross-Mating in Plasmodium Falciparum in Households in a Tanzanian Village. Parasitology 111 (Pt 4), 433–442. doi:10.1017/s0031182000065938
Babiker, H. A., Hill, W. G., Lines, J., and Walliker, D. (1997). Population Structure of Plasmodium Falciparum in Villages with Different Malaria Endemicity in East Africa. Am. J. Trop. Med. Hyg. 56 (2), 141–147. doi:10.4269/ajtmh.1997.56.141
Cattamanchi, A., Hubbard, A., Kyabayinze, D., Dorsey, G., and Rosenthal, P. J. (2003). Distinguishing Recrudescence from Reinfection in a Longitudinal Antimalarial Drug Efficacy Study: Comparison of Results Based on Genotyping of Msp-1, Msp-2, and Glurp. Am. J. Trop. Med. Hyg. 68 (2), 133–139. doi:10.4269/ajtmh.2003.68.133
Collins, W. J., Greenhouse, B., Rosenthal, P. J., and Dorsey, G. (2006). The Use of Genotyping in Antimalarial Clinical Trials: a Systematic Review of Published Studies from 1995-2005. Malar. J. 5, 122. doi:10.1186/1475-2875-5-122
Contamin, H., Konate, L., Trape, J.-F., Mercereau-Puijalon, O., Rogier, C., Fandeur, T., et al. (1996). Different Genetic Characteristics of Plasmodium Falciparum Isolates Collected during Successive Clinical Malaria Episodes in Senegalese Children. Am. J. Trop. Med. Hyg. 54 (6), 632–643. doi:10.4269/ajtmh.1996.54.632
Diggs, C. L., Ballou, W. R., and Miller, L. H. (1993). The Major Merozoite Surface Protein as a Malaria Vaccine Target. Parasitol. Today 9 (8), 300–302. doi:10.1016/0169-4758(93)90130-8
Eltayeb, L. B., Abdelghani, S., Madani, M., and Waggiallah, H. A. (2020). Molecular Characterization of Msp-1 and Msp-2 Among Plasmodium Falciparum-Infected Children: A Gene Polymorphisms Analysis. J. Biochem. Tech. 11 (2), 102–107.
Färnert, A., Arez, A. P., Babiker, H. A., Beck, H. P., Benito, A., Björkman, A., et al. (2001). Genotyping of Plasmodium Falciparum Infections by PCR: a Comparative Multicentre Study. Trans. R. Soc. Trop. Med. Hyg. 95 (2), 225–232. doi:10.1016/s0035-9203(01)90175-0
Ferreira, M. U., da Silva Nunes, M., and Wunderlich, G. (2004). Antigenic Diversity and Immune Evasion by Malaria Parasites. Clin. Vaccin. Immunol 11 (6), 987–995. doi:10.1128/cdli.11.6.987-995.2004
Ghanchi, N. K., Mårtensson, A., Ursing, J., Jafri, S., Bereczky, S., Hussain, R., et al. (2010). Genetic Diversity Among Plasmodium Falciparum Field Isolates in Pakistan Measured with PCR Genotyping of the Merozoite Surface Protein 1 and 2. Malar. J. 9, 1. doi:10.1186/1475-2875-9-1
Gómez, D., Rojas, M. O., Rubiano, C., Chaparro, J., and Wasserman, M. (2002). Genetic Diversity of Plasmodium Falciparum Field Samples from an Isolated Colombian Village. Am. J. Trop. Med. Hyg. 67 (6), 611–616. doi:10.4269/ajtmh.2002.67.611
Greenhouse, B., Dorsey, G., Myrick, A., Carlson, E. J., Dokomajilar, C., Rosenthal, P. J., et al. (2006). Validation of Microsatellite Markers for Use in Genotyping Polyclonal Plasmodium Falciparum Infections. Am. J. Trop. Med. Hyg. 75 (5), 836–842. doi:10.4269/ajtmh.2006.75.836
Greenwood, B. M., Fidock, D. A., Kyle, D. E., Kappe, S. H. I., Alonso, P. L., Collins, F. H., et al. (2008). Malaria: Progress, Perils, and Prospects for Eradication. J. Clin. Invest. 118 (4), 1266–1276. doi:10.1172/jci33996
Haddad, D., Enamorado, I. G., Berzins, K., Snounou, G., Ståhl, S., Mattei, D., et al. (1999). Limited Genetic Diversity of Plasmodium Falciparum in Field Isolates from Honduras. Am. J. Trop. Med. Hyg. 60 (1), 30–34. doi:10.4269/ajtmh.1999.60.30
Heidari, A., Keshavarz, H., Rokni, M. B., and Jelinek, T. (2007). Genetic Diversity in Merozoite Surface Protein (MSP)-1 and MSP-2 Genes of Plasmodium Falciparum in a Major Endemic Region of Iran. Korean J. Parasitol. 45 (1), 59–63. doi:10.3347/kjp.2007.45.1.59
Howard, N., Durrani, N., Sanda, S., Beshir, K., Hallett, R., and Rowland, M. (2011). Clinical Trial of Extended-Dose Chloroquine for Treatment of Resistant Falciparum Malaria Among Afghan Refugees in Pakistan. Malar. J. 10, 171. doi:10.1186/1475-2875-10-171
Jordan, S., Jelinek, T., Aida, A. O., Peyerl-Hoffmann, G., Heuschkel, C., el Valy, A. O., et al. (2001). Population Structure of Plasmodium Falciparum Isolates during an Epidemic in Southern Mauritania. Trop. Med. Int. Health 6 (10), 761–766. doi:10.1046/j.1365-3156.2001.00802.x
Khan, M. A., Mekan, S. F., Abbas, Z., and Smego, R. A. (2005). Concurrent Malaria and Enteric Fever in Pakistan. Singapore Med. J. 46 (11), 635–638.
Khatoon, L., Baliraine, F. N., Bonizzoni, M., Malik, S. A., and Yan, G. (2010). Genetic Structure of Plasmodium Vivax and Plasmodium Falciparum in the Bannu District of Pakistan. Malar. J. 9 (1), 112. doi:10.1186/1475-2875-9-112
Khatoon, L., Khan, I. U., Shah, S. A., Jan, M. I., Ullah, F., and Malik, S. A. (2012). Genetic Diversity of Plasmodium Vivax and Plasmodium Falciparum in Kohat District, Pakistan. Braz. J. Infect. Dis. 16 (2), 184–187. doi:10.1590/s1413-86702012000200014
Konaté, L., Zwetyenga, J., Rogier, C., Bischoff, E., Fontenille, D., Tall, A., et al. (1999). 5. Variation of Plasmodium Falciparum Msp1 Block 2 and Msp2 Allele Prevalence and of Infection Complexity in Two Neighbouring Senegalese Villages with Different Transmission Conditions. Trans. R. Soc. Trop. Med. Hyg. 93 (Suppl. ment_1), 21–28. doi:10.1016/s0035-9203(99)90323-1
Mohammed, H., Hassen, K., Assefa, A., Mekete, K., Tadesse, G., Taye, G., et al. (2019). Genetic Diversity of Plasmodium Falciparum Isolates from Patients with Uncomplicated and Severe Malaria Based on Msp-1 and Msp-2 Genes in Gublak, North West Ethiopia. Malar. J. 18 (1), 413. doi:10.1186/s12936-019-3039-9
Montoya, L., Maestre, A., Carmona, J., Lopes, D., Do Rosario, V., and Blair, S. (2003). Plasmodium Falciparum: Diversity Studies of Isolates from Two Colombian Regions with Different Endemicity. Exp. Parasitol. 104 (1-2), 14–19. doi:10.1016/s0014-4894(03)00112-7
Murtaza, G., Memon, I. A., and Noorani, A. K. (2004). Academic Works and Political Activities. Med. Channel 10 (2), 41–95. doi:10.4324/9780203335239-7
Ndiaye, T., Sy, M., Gaye, A., and Ndiaye, D. (2019). Genetic Polymorphism of Merozoite Surface Protein 1 (Msp1) and 2 (Msp2) Genes and Multiplicity of Plasmodium Falciparum Infection across Various Endemic Areas in Senegal. Afr. H. Sci. 19 (3), 2446–2456. doi:10.4314/ahs.v19i3.19
Nei, M. (1978). Estimation of Average Heterozygosity and Genetic Distance from a Small Number of Individuals. Genetics 89 (3), 583–590. doi:10.1093/genetics/89.3.583
Nizamani, A., Kalar, N., and Khushk, I. (2006). Burden of Malaria in Sindh,Pakistan: A Two Years Surveillance Report. J. Liaquat Uni Med. Health Sci. 05, 76–83. doi:10.22442/jlumhs.06520092
Papa Mze, N., Bogreau, H., Diedhiou, C. K., Herdell, V., Rahamatou, S., Bei, A. K., et al. (2020). Genetic Diversity of Plasmodium Falciparum in Grande Comore Island. Malar. J. 19 (320). doi:10.1186/s12936-020-03384-5
Paul, R. E., Nosten, F., White, N. J., Luxemburger, C., Price, R., Hackford, I., et al. (1998). Transmission Intensity and Plasmodium Falciparum Diversity on the Northwestern Border of Thailand. Am. J. Trop. Med. Hyg. 58 (2), 195–203. doi:10.4269/ajtmh.1998.58.195
Peyerl-Hoffmann, G., Jelinek, T., Kilian, A., Kabagambe, G., Metzger, W. G., and von Sonnenburg, F. (2001). Genetic Diversity of Plasmodium Falciparum and its Relationship to Parasite Density in an Area with Different Malaria Endemicities in West Uganda. Trop. Med. Int. Health 6 (8), 607–613. doi:10.1046/j.1365-3156.2001.00761.x
Phillips, R. S. (2001). Current Status of Malaria and Potential for Control. Clin. Microbiol. Rev. 14 (1), 208–226. doi:10.1128/cmr.14.1.208-226.2001
Qureshi, N. A., Fatima, H., Afzal, M., Khattak, A. A., and Nawaz, M. A. (2019). Occurrence and Seasonal Variation of Human Plasmodium Infection in Punjab Province, Pakistan. BMC Infect. Dis. 19 (1), 935. doi:10.1186/s12879-019-4590-2
Roh, M. E., Tessema, S. K., Murphy, M., Nhlabathi, N., Mkhonta, N., Vilakati, S., et al. (2019). High Genetic Diversity of Plasmodium Falciparum in the Low-Transmission Setting of the Kingdom of Eswatini. J. Infect. Dis. 220 (8), 1346–1354. doi:10.1093/infdis/jiz305
Sallenave-Sales, S., Daubersies, P., Mercereau-Puijalon, O., Rahimalala, L., Contamin, H., Druilhe, P., et al. (2000). Plasmodium Falciparum: a Comparative Analysis of the Genetic Diversity in Malaria-Mesoendemic Areas of Brazil and Madagascar. Parasitol. Res. 86 (8), 692–698. doi:10.1007/pl00008554
Sheikh, A. S., Sheikh, A. A., Sheikh, N. S., and Paracha, S. M. (2005). Endemicity of Malaria in Quetta. Pak J. Med. Res. 44 (1), 41–50.
Singh, B., Snounou, G., Abdullah, M. S., Rahman, H. A., Bobogare, A., and Cox-Singh, J. (1999). A Genus- and Species-specific Nested Polymerase Chain Reaction Malaria Detection Assay for Epidemiologic Studies. Am. J. Trop. Med. Hyg. 60 (4), 687–692. doi:10.4269/ajtmh.1999.60.687
Smythe, J. A., Coppel, R. L., Day, K. P., Martin, R. K., Oduola, A. M., Kemp, D. J., et al. (1991). Structural Diversity in the Plasmodium Falciparum Merozoite Surface Antigen 2. Proc. Natl. Acad. Sci. 88 (5), 1751–1755. doi:10.1073/pnas.88.5.1751
Snounou, G., and Beck, H.-P. (1998). The Use of PCR Genotyping in the Assessment of Recrudescence or Reinfection after Antimalarial Drug Treatment. Parasitol. Today 14 (11), 462–467. doi:10.1016/s0169-4758(98)01340-4
Snounou, G., Viriyakosol, S., Xin Ping Zhu, Z. P., Jarra, W., Pinheiro, L., do Rosario, V. E., et al. (1993). High Sensitivity of Detection of Human Malaria Parasites by the Use of Nested Polymerase Chain Reaction. Mol. Biochem. Parasitol. 61, 315–320. doi:10.1016/0166-6851(93)90077-b
Snounou, G., Zhu, X., Siripoon, N., Jarra, W., Thaithong, S., Brown, K. N., et al. (1999). Biased Distribution of Msp1 and Msp2 Allelic Variants in Plasmodium Falciparum Populations in Thailand. Trans. R. Soc. Trop. Med. Hyg. 93 (4), 369–374. doi:10.1016/s0035-9203(99)90120-7
Soe, T. N., Wu, Y., Tun, M. W., Xu, X., Hu, Y., Ruan, Y., et al. (2017). Genetic Diversity of Plasmodium Falciparum Populations in Southeast and Western Myanmar. Parasites Vectors 10 (1), 322. doi:10.1186/s13071-017-2254-x
Somé, A. F., Bazié, T., Zongo, I., Yerbanga, R. S., Nikiéma, F., Neya, C., et al. (2018). Plasmodium Falciparum Msp1 and Msp2 Genetic Diversity and Allele Frequencies in Parasites Isolated from Symptomatic Malaria Patients in Bobo-Dioulasso, Burkina Faso. Parasites Vectors 11 (1), 323. doi:10.1186/s13071-018-2895-4
Soulama, I., Nébié, I., Ouédraogo, A., Gansane, A., Diarra, A., Tiono, A. B., et al. (2009). Plasmodium Falciparum Genotypes Diversity in Symptomatic Malaria of Children Living in an Urban and a Rural Setting in Burkina Faso. Malar. J. 8, 135. doi:10.1186/1475-2875-8-135
Targett, G., Drakeley, C., Jawara, M., von Seidlein, L., Coleman, R., Deen, J., et al. (2001). Artesunate Reduces but Does Not Prevent Posttreatment Transmission of Plasmodium Falciparum to Anopheles Gambiae. J. Infect. Dis. 183 (8), 1254–1259. doi:10.1086/319689
Taylor, R. R., Smith, D. B., Robinson, V. J., McBride, J. S., and Riley, E. M. (1995). Human Antibody Response to Plasmodium Falciparum Merozoite Surface Protein 2 Is Serogroup Specific and Predominantly of the Immunoglobulin G3 Subclass. Infect. Immun. 63 (11), 4382–4388. doi:10.1128/iai.63.11.4382-4388.1995
Who (2017). World malaria report. World Health Organization, Available at: https://www.who.int/publications-detail-redirect/9789241565523.
Who (2018). World malaria report. World Health Organization, Available at: https://apps.who.int/iris/handle/10665/275867.
Yasinzai, M. I., and Kakarsulemankhel, J. K. (2009). Prevalence of Human Malaria Infection in Bordering Areas of East Balochistan, Adjoining with Punjab: Loralai and Musakhel. J. Pak Med. Assoc. 59 (3), 132–135.
Yavo, W., Konaté, A., Mawili-Mboumba, D. P., Kassi, F. K., Tshibola Mbuyi, M. L., Angora, E. K., et al. (2016). Genetic Polymorphism of msp1 and msp2 in Plasmodium falciparum isolates from Côte d'Ivoire versus Gabon. J. Parasitol. Res. 2016, 1–7. doi:10.1155/2016/3074803
Zakeri, S., Bereczky, S., Naimi, P., Pedro Gil, J., Djadid, N. D., Färnert, A., et al. (2005). Multiple Genotypes of the Merozoite Surface Proteins 1 and 2 in Plasmodium Falciparum Infections in a Hypoendemic Area in Iran. Trop. Med. Int. Health 10 (10), 1060–1064. doi:10.1111/j.1365-3156.2005.01477.x
Keywords: Plasmodium falciparum, genetic diversity, polymorphism, msp-1 and msp-2 genes, nested PCR, Khyber Pakhtunkhwa, Pakistan
Citation: Khan SN, Ali R, Khan S, Rooman M, Norin S, Zareen S, Ali I and Ayaz S (2021) Genetic Diversity of Polymorphic Marker Merozoite Surface Protein 1 (Msp-1) and 2 (Msp-2) Genes of Plasmodium falciparum Isolates From Malaria Endemic Region of Pakistan. Front. Genet. 12:751552. doi: 10.3389/fgene.2021.751552
Received: 01 August 2021; Accepted: 04 October 2021;
Published: 17 November 2021.
Edited by:
Moses Okpeku, University of KwaZulu-Natal, South AfricaReviewed by:
Diego Garzón-Ospina, Universidad Pedagógica y Tecnológica de Colombia, ColombiaOlusola Ojurongbe, Ladoke Akintola University of Technology, Nigeria
Copyright © 2021 Khan, Ali, Khan, Rooman, Norin, Zareen, Ali and Ayaz. This is an open-access article distributed under the terms of the Creative Commons Attribution License (CC BY). The use, distribution or reproduction in other forums is permitted, provided the original author(s) and the copyright owner(s) are credited and that the original publication in this journal is cited, in accordance with accepted academic practice. No use, distribution or reproduction is permitted which does not comply with these terms.
*Correspondence: Shahid Niaz Khan, c2hhaGlkQGt1c3QuZWR1LnBr; Rehman Ali, cmVobWFuYWxpNzY4MEBnbWFpbC5jb20=