- 1Department of Pulmonary Medicine, Shanghai Chest Hospital, Shanghai Jiao Tong University, Shanghai, China
- 2Shanghai Institute of Thoracic Oncology, Shanghai Chest Hospital, Shanghai Jiao Tong University, Shanghai, China
- 3Translational Medical Research Platform for Thoracic Oncology, Shanghai Chest Hospital, Shanghai Jiao Tong University, Shanghai, China
- 4Clinical Research Center, Shanghai Chest Hospital, Shanghai Jiao Tong University, Shanghai, China
Atezolizumab, an immune checkpoint inhibitor, has been approved for use in clinical practice in non-small cell lung cancer (NSCLC) patients, but potential biomarkers for response stratification still need further screening. In the present study, a total of 399 patients with high-quality ctDNA profiling results were included. The mutation status of ubiquitin-like conjugation (UBL) biological process genes (including ABL1, APC, LRP6, FUBP1, KEAP1, and TOP2A) and clinical information were further integrated. The results suggested that the patients with the clinical characteristics of male or history of smoking had a higher frequency of UBL mutation positivity [UBL (+)]; the patients who were UBL (+) had shorter progression-free survival (PFS) (1.69 vs. 3.22 months, p = 0.0007) and overall survival (8.61 vs. 16.10 months, p < 0.0001) than those patients with UBL mutation negativity [UBL (–)]; and more promising predictive values were shown in the smoker subgroup and ≤ 3 metastasis subgroup. More interestingly, we found the predictor has more performance in TP53-negative cohorts [training in an independent POPLAR and OAK cohorts (n = 200), and validation in an independent MSKCC cohort (n = 127)]. Overall, this study provides a predictor, UBL biological process gene mutation status, not only for identifying NSCLC patients who may respond to atezolizumab therapy but also for screening out the potential NSCLC responders who received other immune checkpoint inhibitors.
Introduction
Non-small cell lung cancer (NSCLC) accounts for approximately 85% of all lung cancers (Lu et al., 2019b; Zhang et al., 2020; Lou et al., 2021). Standard therapeutic regimens for first-line therapy have been recommended for NSCLC patients according to the guidelines of the National Comprehensive Cancer Network (Ettinger et al., 2021). Patients harboring EGFR mutations are recommended to receive tyrosine kinase inhibitors (TKIs) such as osimertinib and gefitinib (Zhao et al., 2019; Ettinger et al., 2021); patients harboring ALK mutations are recommended to receive TKIs such as alectinib and crizotinib (Peters et al., 2017; Shaw et al., 2020). However, patients without driver gene mutations usually receive chemotherapy or immunotherapy (Gadgeel et al., 2020; Ettinger et al., 2021). Regarding second-line therapy for NSCLC patients, immunotherapy is potentially suitable for patients with PD-L1 expression (Fehrenbacher et al., 2016; Rittmeyer et al., 2017).
The POPLAR study and OAK studies demonstrated that NSCLC patients who received atezolizumab (one of immune checkpoint inhibitors) as second-line therapy had significantly prolonged overall survival (OS) compared with docetaxel patients, regardless of PD-L1 expression or histology (Fehrenbacher et al., 2016; Rittmeyer et al., 2017). After that, Gandara et al. (2018) found that ctDNA profiling was a potential technology to be used for atezolizumab response stratification in the above NSCLC patients. Furthermore, our previous study suggested that the ctDNA profiling potentially provides more information for immunotherapeutic stratification (Nie et al., 2020). Although an increasing number of studies have introduced that genetic profiling can be used for predicting the efficacy of immunotherapy (Chan et al., 2019; Fabrizio et al., 2021; McGrail et al., 2021), further screening of gene cluster-based biomarkers for immunotherapy is still an urgent problem that needs to be resolved.
Ubiquitin-like conjugation (UBL) biological processes play an important role in cancer development, progression, and therapy. However, the underlying role in immunotherapy is still unclear. In the present study, we found that UBL-enriched gene (ABL1, APC, LRP6, FUBP1, KEAP1, and TOP2A) mutation significantly affected atezolizumab efficacy as second-line therapy in NSCLC patients, and we sought to identify a biomarker for potential use in clinical practice in the future.
Materials and Methods
Patients
In the present study, all blood samples were collected from enrolled NSCLC patients from the POPLAR study (NCT01903993) and OAK study (NCT02008227). All clinical trials were performed according to Good Clinical Practice guidelines and those of the Declaration of Helsinki. The purpose of blood sample collection was completely explained to patients or their family members, and the signed informed consent was obtained. In the POPLAR study, 144 of 287 patients received atezolizumab therapy, and 425 of 850 patients in the OAK study received atezolizumab. Collectively, 569 patients were preliminarily selected in the present study.
ctDNA Profiling
The collected baseline plasma samples from all 569 atezolizumab-treated NSCLC patients underwent uniform procedures for ctDNA mutation calling. The methods for sample collection, storage conditions, cell-free DNA (cfDNA) extraction, library construction, sequencing, analysis, and mutation calling were performed according to a previously published article (Gandara et al., 2018). The clinical information for each patient was downloaded from the online database.1
Screening
In total, 569 NSCLC patients had complete clinical information and baseline blood samples. During library construction, 39 of 569 samples failed quality control (including the samples with cfDNA < 20 ng, and the samples failed with templated extension). After sequencing, 101 of 530 ctDNA profiles that did not achieve a minimum of 800× sequence coverage were excluded. Thirty of 429 patients without a definite efficacy evaluation were excluded. Therefore, in this study, 399 patients were selected for final analysis (Figure 1).
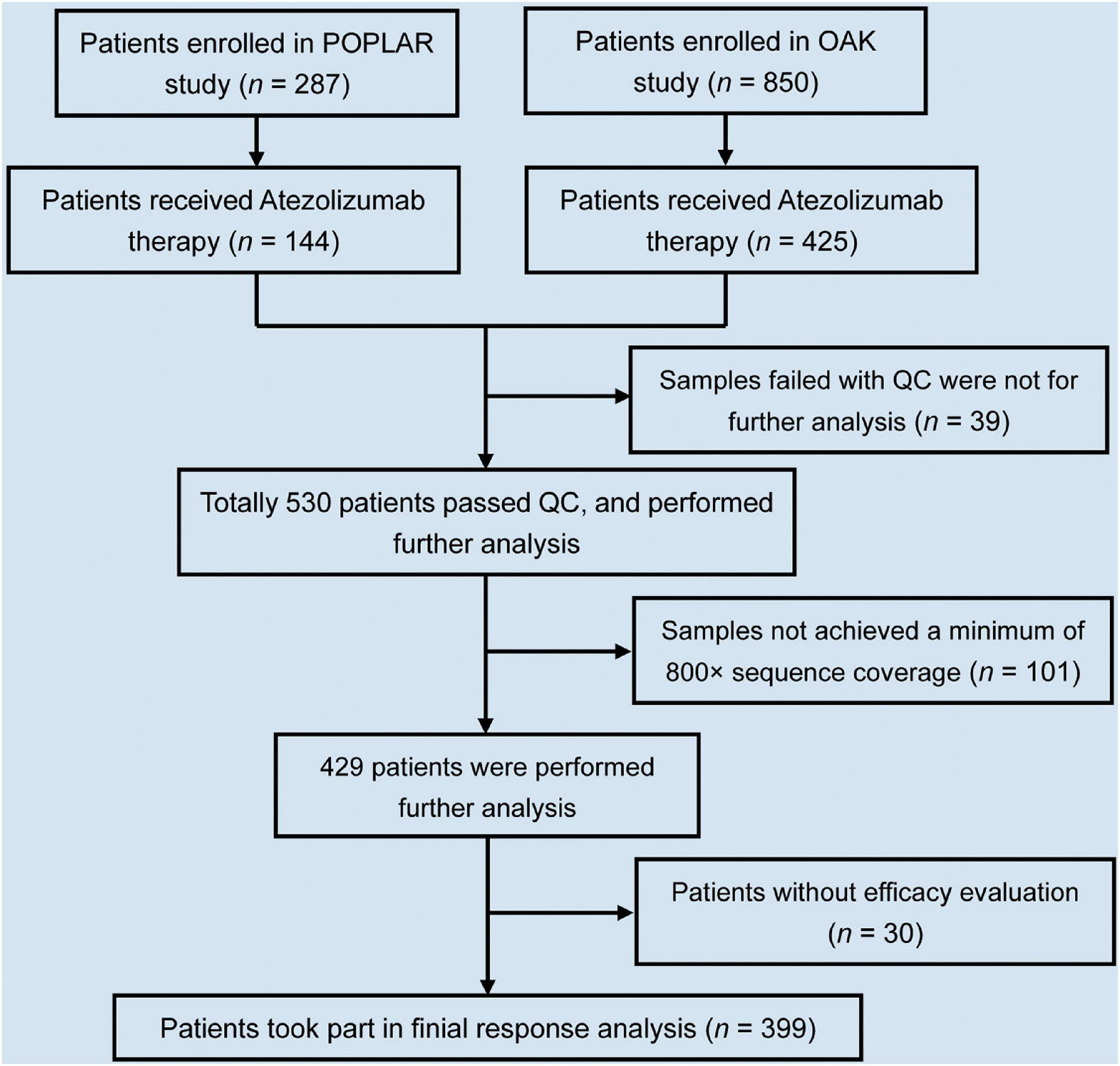
Figure 1. Flowchart of NSCLC patient selection in this study. In total, 1,137 NSCLC patients enrolled in POPLAR and OAK studies. Of 1,137 patients, 569 received atezolizumab therapy. Of 569, 399 patients took part in final analysis after selection.
UBL Biological Process
We found 394 cancer-related genes with mutations in all ctDNA samples from 399 patients. Then, the list of these genes was uploaded to the DAVID database for biological process enrichment analysis. UBL biological processes were significantly enriched and mainly included six genes (ABL1, APC, LRP6, FUBP1, KEAP1, and TOP2A).
Kaplan–Meier Curve Analysis
This method performed as our previous studies (Lu et al., 2019a,c; Chu et al., 2021). The 399 patients who harbored any mutation in UBL biological process genes (ABL1, APC, LRP6, FUBP1, KEAP1, and TOP2A) from ctDNA profiling were defined as UBL (+). A patient who harbored two or more gene mutations of the abovementioned six genes was defined as “UBL (+) harboring 2 or more gene mutations.” If ctDNA profiling detected no mutation in the above UBL biological process genes, the patient was defined as UBL (–). Based on the UBL prediction, the next procedure was stratifying progression-free survival (PFS) and OS by using GraphPad Prism 5 software.
Subgroup Analysis
According to the different clinical characteristics and driver gene mutation status, we classified the 399 patients into male and female, non-smoker and smoker, non-lung squamous carcinoma (non-LUSC) and LUSC, Asian and White, EGFR (+) and KRAS (+), Eastern Cooperative Oncology Group (ECOG) score = 0 and ECOG score = 1, and metastases ≤ 3 and metastases > 3. Kaplan–Meier curve analysis was performed to calculate the median PFS and median OS and the corresponding log-rank p-value. Hazard ratio (HR) was calculated by use of Cox proportional hazards model.
Validation Analysis
According to the TP53 mutation status, we classified the 399 patients into TP53 mutation-positive NSCLC patients and TP53 mutation-negative NSCLC patients. Similar UBL-based stratification analysis was performed on the NSCLC patients with TP53 mutation and the NSCLC patients without TP53 mutation, respectively. For the validation cohort, we selected the TP53 mutation-negative NSCLC patients who received at least one dose of immunotherapy (atezolizumab, avelumab, durvalumab, ipilimumab, nivolumab, pembrolizumab, or tremelimumab) from the Memorial Sloan Kettering Cancer Center (MSKCC). Different from the POPLAR and OAK cohorts, the mutation information of the NSCLC patients from the MSKCC cohort was derived from tumor tissue DNA (ttDNA). Furthermore, the patients undergo genomic profiling with the Integrated Mutation Profiling of Actionable Cancer Targets (MSK-IMPACT) panel. The patients from the MSKCC cohort just provided the OS information. Therefore, the predictive value of UBL for OS stratification was performed in the validation cohort.
Statistical Analysis
The Mann–Whitney U-test was performed to compare the age difference between the UBL (+) cohort and UBL (–) cohort. The chi-square test was performed to compare the differences of other clinical characters. The log-rank test was used to compare Kaplan–Meier curves during the stratification analysis. The HRs and exact 95% confidence intervals (CIs) are reported. Differences were considered significant at ∗p < 0.05, ∗∗p < 0.01, and ∗∗∗p < 0.001.
Results
In this study, we identified NSCLC patients harboring UBL biological process gene (ABL1, APC, LRP6, FUBP1, KEAP1, and TOP2) mutations, and this information could be used to stratify patients who might respond to the immune checkpoint inhibitor atezolizumab. Based on our rigorous screening procedure, 399 patients were finally included for screening the responsive biomarker (Figure 1). The analysis of clinical characteristics indicated that there was no association between UBL gene mutation status and age, pathological status, race, driver gene (EGFR, ALK, and EML4) status, metastasis site number, and ECOG score. However, there was a significant increase in UBL gene mutation frequency in the male patients and the patients with smoking history (Table 1). These results suggested that there is a bias in the mutation of UBL biological process genes in NSCLC patients. Whether this phenomenon can be used as a predictor of immunotherapy response is still unclear.
Here, we classified 399 atezolizumab-treated NSCLC patients into two cohorts: those who were UBL (+) and those who were UBL (–). Kaplan–Meier curve analysis suggested that the NSCLC patients who were UBL (+) had shorter PFS (UBL (+) vs. UBL (–) = 1.69 vs. 3.22 months, log-rank p-value = 0.0007) than the UBL (–) cohort (Figure 2A). Regarding OS analysis, the results showed a more significant difference: the patients in the UBL (–) cohort received more OS benefit from atezolizumab therapy (UBL (–) vs. UBL (+) = 16.10 vs. 8.41 months, log-rank p-value < 0.0001) than the patients in the UBL (+) cohort (Figure 2B). More interestingly, in the UBL (+) cohort, the patients who defined as UBL (+) harboring two or more gene mutations received shorter PFS and OS from atezolizumab therapy (PFS: 1.41 vs. 2.00 months, log-rank p-value = 0.1385; OS: 5.06 vs. 9.99 months, log-rank p-value = 0.0004) (Figures 2C,D). These results indicated that the mutation status of the UBL biological process genes could potentially be used as a predictor of response to atezolizumab as second-line therapy in NSCLC patients.
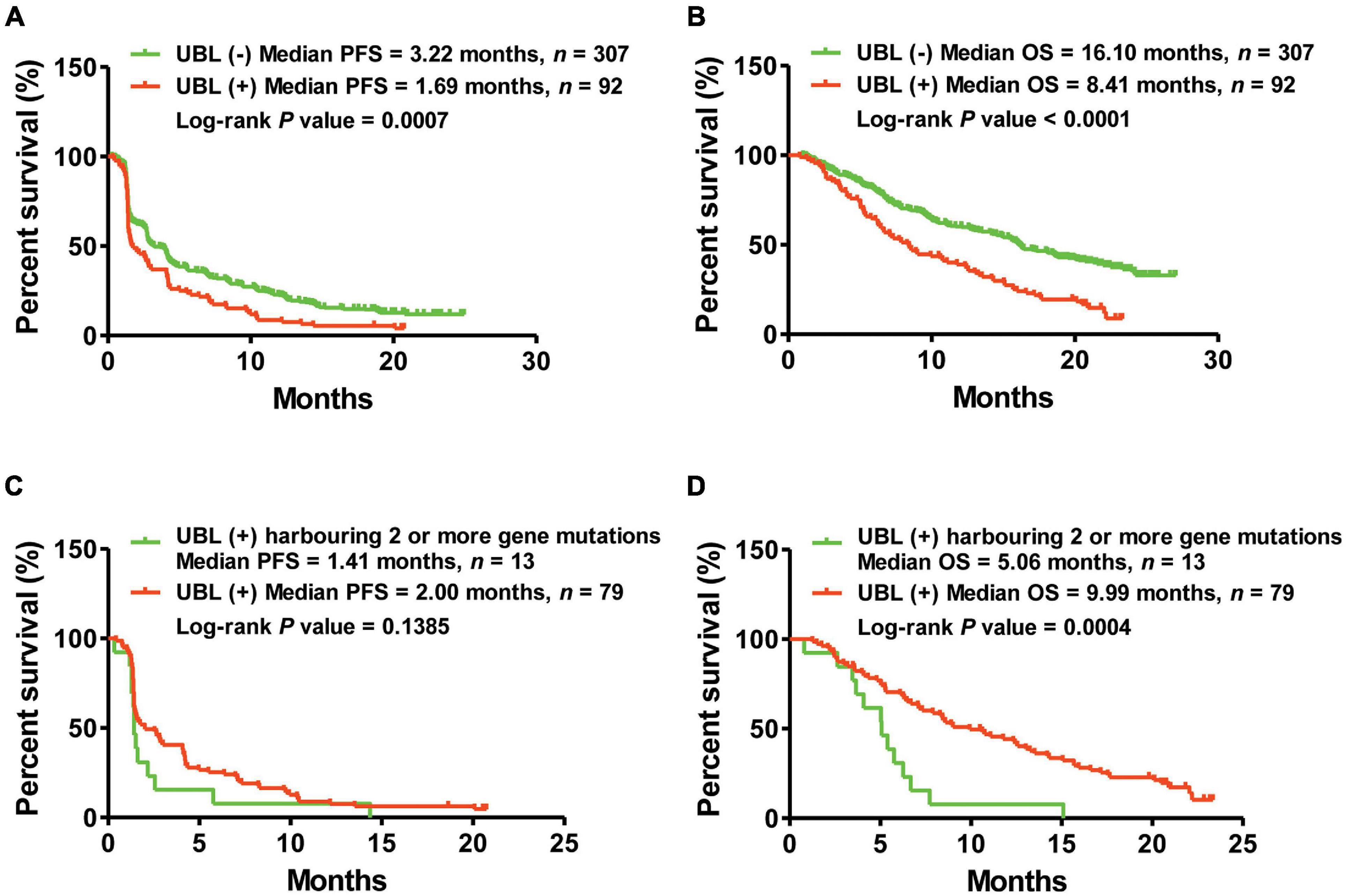
Figure 2. Kaplan–Meier curve analysis of PFS and OS via the predictor of UBL. (A) NSCLC patients harboring UBL (+) received median PFS of 1.69 months, while those patients harboring UBL (–) received median PFS of 3.22 months. (B) NSCLC patients harboring UBL (+) received median OS of 8.41 months, while those patients harboring UBL (–) received median OS of 16.10 months. (C) UBL (+) NSCLC patients harboring two or more gene mutations received median PFS of 1.41 months, while those UBL (+) patients harboring single gene mutation received median PFS of 2.00 months. (D) UBL (+) NSCLC patients harboring two or more gene mutations received median OS of 5.06 months, while those UBL (+) patients harboring single gene mutation received median OS of 9.99 months.
To further understand the performance of UBL status in subgroups, we first analyzed the sex-induced response difference for NSCLC patients who received atezolizumab. Male NSCLC patients who were UBL (+) had significantly shorter PFS and OS than male patients who were UBL (–), while there was no significant difference between female patients who were UBL (+) and female patients who were UBL (–). UBL (+) patients with a history of smoking had significantly shorter PFS and OS than UBL (–) patients with a history of smoking. For those patients without a smoking history, there was no significant difference between UBL (+) patients and UBL (–) patients. Regarding the non-LUSC subgroup, patients who were UBL (+) had significantly shorter PFS and OS than male patients who were UBL (–) (Table 2).
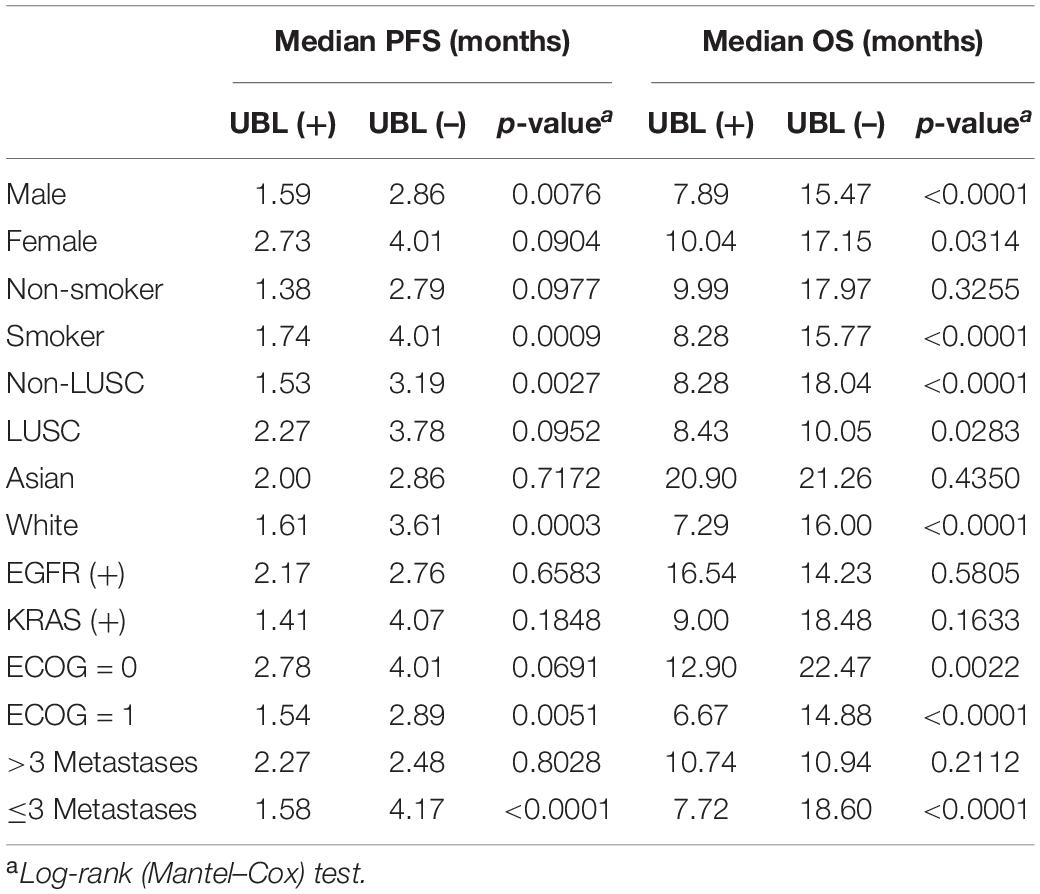
Table 2. Subgroup response analysis using the biomarkers of UBL in atezoluzimab-treated patients from OAK and POPLAR cohorts.
For LUSC patients, UBL (+) patients had a shorter OS than UBL (–) patients, while there was no difference when comparing the PFS between these two cohorts. Neither UBL (+) nor UBL (–) labeled in Asian patients, and there was no significant PFS or OS outcome difference when patients received atezolizumab therapy. Patients harboring EGFR mutations or KRAS mutations combined with UBL biological process gene mutations who received atezolizumab therapy had PFS and OS outcomes that were similar to those of patients without UBL biological process gene mutations. Regarding the ECOG score = 1 subgroup, patients who were UBL (+) had significantly shorter PFS and OS than those without UBL (–). For the ECOG score = 0 subgroup, UBL (+) patients had a shorter OS than UBL (–) patients, while there was no difference when comparing the PFS between the two cohorts. Interestingly, UBL significantly stratified responders from non-responders in the ≤ 3 metastasis subgroup (Table 2). These results suggested that UBL biological process gene mutation status could potentially be used as a predictor of response to atezolizumab therapy in NSCLC patients, especially in the male, smoker, non-LUSC, White, ECOG score = 1, and ≤ 3 metastasis subgroups. In addition, our analysis focused on HR indicated that the UBL status predictor remarkably distinguished patients with an atezolizumab response and reduced risk of death in the White subgroup and the ≤ 3 metastasis subgroup (Figure 3).
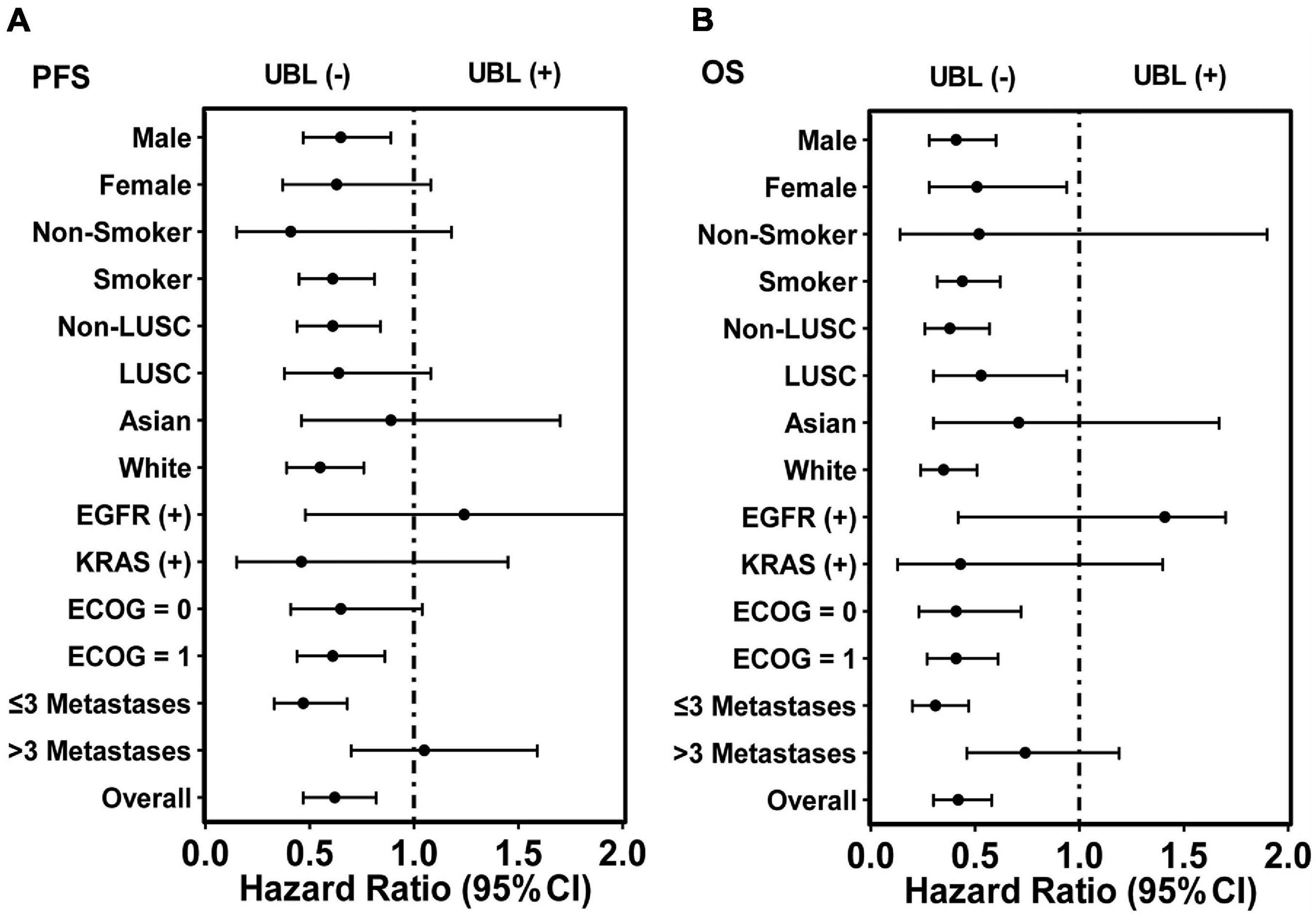
Figure 3. HR analysis of overall 399 patients and corresponding subgroups via the predictor UBL-based stratification. (A) The predictor UBL potentially decreased HR in nearly all subgroups except the subgroups of EGFR (+) and > 3 metastases when PFS analysis was performed. (B) The predictor UBL potentially decreased HR in all subgroups except the subgroup of EGFR (+) when OS analysis was performed.
The frequency of TP53 mutation accounts for the highest grade (about 50%) in NSCLC. Whether the predictor of UBL plays a differential role between TP53-positive NSCLC and TP53-negative NSCLC is still unclear. To further understand the performance of UBL status in TP53 mutation-based subgroups, here we analyzed the TP53 mutation-induced response difference for NSCLC patients who received atezolizumab. As shown in Figure 4A, there is no significant distinguishing values of the predictor UBL among the NSCLC patients harboring TP53 mutation. Interestingly, the predictor UBL showed more predictive values in the TP53 negative NSCLC patients than that in overall NSCLC patients (Figures 3, 4B). To validate whether the predictor UBL can be validated in an independent cohort, we used the NSCLC patients who received immunotherapy from MSKCC center as the validation cohort. Results demonstrated that the TP53 mutation-negative NSCLC patients with benefited OS outcome can be screened out significantly via the predictor UBL-based stratification (Figure 4C).
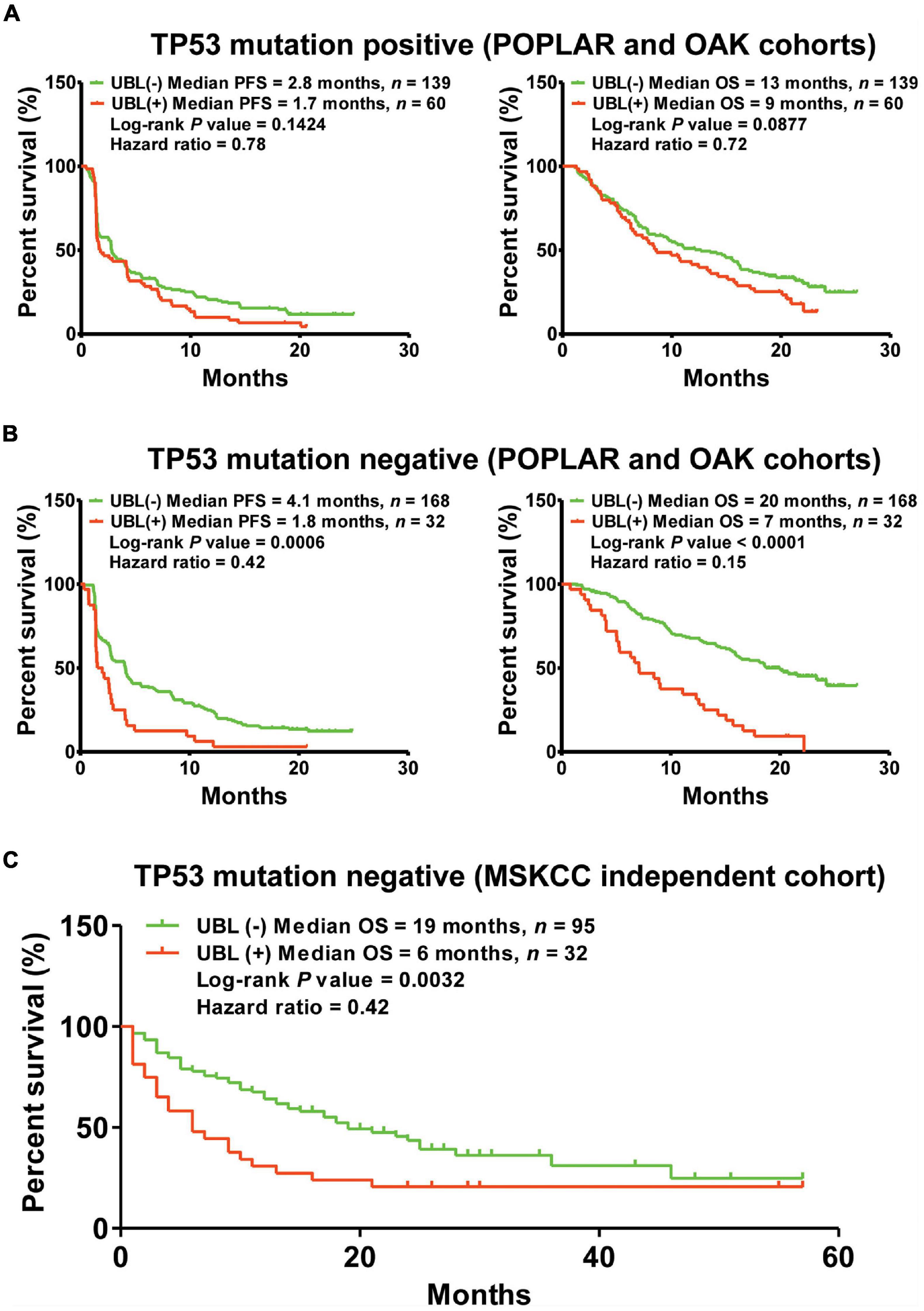
Figure 4. The predictor of UBL for immunotherapeutic stratification in TP53 mutation-based subgroups. (A) The predictive values of UBL in the NSCLC patients harboring TP53 mutation. (B) The predictive values of UBL in the NSCLC patients without harboring TP53 mutation. (C) An independent MSKCC cohort was used to validate the predictive value of UBL in the TP53-negative NSCLC patients who received immunotherapy.
Discussion
Immunotherapy has changed clinical practice in NSCLC (Eguren-Santamaria et al., 2020; Pinheiro et al., 2020; Ettinger et al., 2021). With the clinical application of immune checkpoint inhibitors in NSCLC, an increasing number of clinical problems have surfaced (de Miguel and Calvo, 2020; Haanen et al., 2020; Ramos-Casals et al., 2020; Shankar et al., 2020). Among these emerging clinical problems, how to screen out responders using a predictor is always confusing for clinical physicians and scientists (Darvin et al., 2018; de Miguel and Calvo, 2020). Therefore, in the present study, we sought to identify a ctDNA profiling-based predictor that might be used to stratify responders to the immune checkpoint inhibitor atezolizumab as second-line therapy among NSCLC patients.
Previous studies have demonstrated that genetic profiling can potentially be used as a biomarker for response to immune checkpoint inhibitors (Gandara et al., 2018; Samstein et al., 2019; Alborelli et al., 2020). However, an increasing number of scientists have provided different viewpoints about the usage of genetic profiling for predicting therapeutic efficacy in immunotherapy (Fabrizio et al., 2021; McGrail et al., 2021). With the development of technology, blood-based next-generation sequencing has opened a new field of view for biomarkers predicting response to immune checkpoint inhibitors (Gandara et al., 2018). Our previous study showed that the ctDNA profiling potentially provides more information for immunotherapeutic stratification (Nie et al., 2020). Samstein et al. (2019) and McGrail et al. (2021) demonstrated that genetic profiling plays different roles in different cancer types. Furthermore, the biomarkers including PD-L1 and microsatellite instability also have some questions that need to be resolved (Chang et al., 2018; Gandara et al., 2018). Therefore, predictor discovery for immunotherapy has just started, and there is much unknown information that needs to be explored.
In this study, we provided evidence that UBL could be used as a predictor to screen out responders from non-responders among NSCLC patients who received atezolizumab as second-line therapy. Our results indicated that NSCLC patients who were male and had a smoking history had a higher frequency of being UBL (+). This is a very interesting phenomenon. Although there is not enough evidence to confirm the association of the above characteristics, we still have a reason to speculate that smoking potentially contributes to UBL biological process gene mutations according to previously reported relationships between smoking and genetic variation (Nagahashi et al., 2018). Further analysis demonstrated that NSCLC patients who were UBL (+) had shorter PFS and OS than patients who were UBL (–). Either PFS analysis or OS analysis showed very promising results for UBL biological process gene mutation status to be able to significantly screen out responders from non-responders.
The roles of UBL biological process gene mutation status in screening out responders in subgroups contributed an important composition in the present study. According to our results, 70.4% of patients were White, and 69.4% of patients had ≤ 3 metastases among all 399 NSCLC patients. Some of the bright points in the subgroup analysis are that UBL biological process gene mutation status can significantly distinguish responders and non-responders when used in the above subgroups. However, why this phenomenon occurred still requires further study.
Based on existing evidence, there may be great differences in tumor biology between patients with NSCLC harboring TP53 mutations and those without TP53 mutations (Mogi and Kuwano, 2011; Jamal-Hanjani et al., 2017; Bailey et al., 2018; Birkbak and McGranahan, 2020). Interestingly, we found the UBL biological process gene mutation status has a very promising predictive value for screening out responders from non-responders both in the POPLAR and OAK cohorts, as well as the MSKCC cohort.
Collectively, this study provided a predictor, UBL biological process gene mutation status, that could be used to distinguish potential responders from non-responders to atezolizumab as second-line therapy among NSCLC patients, with a more promising predictive value in TP53 mutation-negative subgroups. Furthermore, the predictor UBL biological process also potentially screen the responders from non-responders for the TP53 mutation-negative NSCLC patients who received other immune checkpoint inhibitors.
Data Availability Statement
The datasets presented in this study can be found in online repositories. The names of the repository/repositories and accession number(s) can be found in the article/supplementary material.
Ethics Statement
The studies involving human participants were reviewed and approved by the Shanghai Chest Hospital. The patients/participants provided their written informed consent to participate in this study.
Author Contributions
BH, WZ, HW, and JL conceived and designed the experiments. JL, YZ, YL, BY, BZ, MH, YW, YC, ZY, and WZ performed the clinical analysis, bioinformatics analysis, and statistical analysis. JL, YZ, and YL generated the figures and tables. JL wrote the manuscript. BH revised the manuscript. All authors contributed to the article and approved the submitted version.
Funding
This work was supported by the foundation of Shanghai Chest Hospital (project nos. 2019YNJCM11 and YJXT20190102), the Shanghai Leading Talents Program (2013), the Shanghai Jiao Tong University (project nos. 15ZH4009 and YG2021QN121), the Key Program of Translational Medicine from Shanghai Jiao Tong University School of Medicine (project no. 15ZH1008), the Foundation of Chinese Society of Clinical Oncology (project nos. Y-2019AZZD-0355 and Y-QL2019-0125), and the National Natural Science Foundation of China grants (project no. 31801118).
Conflict of Interest
The authors declare that the research was conducted in the absence of any commercial or financial relationships that could be construed as a potential conflict of interest.
Publisher’s Note
All claims expressed in this article are solely those of the authors and do not necessarily represent those of their affiliated organizations, or those of the publisher, the editors and the reviewers. Any product that may be evaluated in this article, or claim that may be made by its manufacturer, is not guaranteed or endorsed by the publisher.
Acknowledgments
We thank the patients for their participation in the OAK and POPLAR clinical trials and MSKCC clinical trials, and the investigators for releasing the sequencing data and clinical data.
Footnotes
References
Alborelli, I., Leonards, K., Rothschild, S. I., Leuenberger, L. P., Savic Prince, S., Mertz, K. D., et al. (2020). Tumor mutational burden assessed by targeted NGS predicts clinical benefit from immune checkpoint inhibitors in non-small cell lung cancer. J. Pathol. 250, 19–29. doi: 10.1002/path.5344
Bailey, M. H., Tokheim, C., Porta-Pardo, E., Sengupta, S., Bertrand, D., Weerasinghe, A., et al. (2018). Comprehensive characterization of cancer driver genes and mutations. Cell 173, 371–385. doi: 10.1016/j.cell.2018.02.060
Birkbak, N. J., and McGranahan, N. (2020). Cancer genome evolutionary trajectories in metastasis. Cancer Cell 37, 8–19. doi: 10.1016/j.ccell.2019.12.004
Chan, T. A., Yarchoan, M., Jaffee, E., Swanton, C., Quezada, S. A., Stenzinger, A., et al. (2019). Development of tumor mutation burden as an immunotherapy biomarker: utility for the oncology clinic. Ann. Oncol. 30, 44–56. doi: 10.1093/annonc/mdy495
Chang, L., Chang, M., Chang, H. M., and Chang, F. (2018). Microsatellite instability: a predictive biomarker for cancer immunotherapy. Appl. Immunohistochem. Mol. Morphol. 26, e15–e21. doi: 10.1097/PAI.0000000000000575
Chu, T., Lu, J., Bi, M., Zhang, H., Zhuang, W., Yu, Y., et al. (2021). Equivalent efficacy study of QL1101 and bevacizumab on untreated advanced non-squamous non-small cell lung cancer patients: a phase 3 randomized, double-blind clinical trial. Cancer Biol. Med. 18, 816–824. doi: 10.20892/j.issn.2095-3941.2020.0212
Darvin, P., Toor, S. M., Sasidharan Nair, V., and Elkord, E. (2018). Immune checkpoint inhibitors: recent progress and potential biomarkers. Exp. Mol. Med. 50, 1–11. doi: 10.1038/s12276-018-0191-1
de Miguel, M., and Calvo, E. (2020). Clinical challenges of immune checkpoint inhibitors. Cancer Cell 38, 326–333. doi: 10.1016/j.ccell.2020.07.004
Eguren-Santamaria, I., Sanmamed, M. F., Goldberg, S. B., Kluger, H. M., Idoate, M. A., Lu, B. Y., et al. (2020). PD-1/PD-L1 blockers in NSCLC brain metastases: challenging paradigms and clinical practice. Clin. Cancer Res. 26, 4186–4197. doi: 10.1158/1078-0432.CCR-20-0798
Ettinger, D. S., Wood, D. E., Aisner, D. L., Akerley, W., Bauman, J. R., Bharat, A., et al. (2021). NCCN guidelines insights: non-small cell lung cancer, version 2.2021. J. Natl. Compr. Canc. Netw. 19, 254–266. doi: 10.6004/jnccn.2021.0013
Fabrizio, D., Cristescu, R., Albacker, L., Snyder, A., Ward, A., Lunceford, J., et al. (2021). Real-world prevalence across 159,872 patients with cancer supports the clinical utility of TMB-H to define metastatic solid tumors for treatment with pembrolizumab. Ann. Oncol. 32, 1193–1194. doi: 10.1016/j.annonc.2021.05.805
Fehrenbacher, L., Spira, A., Ballinger, M., Kowanetz, M., Vansteenkiste, J., Mazieres, J., et al. (2016). Atezolizumab versus docetaxel for patients with previously treated non-small-cell lung cancer (POPLAR): a multicentre, open-label, phase 2 randomised controlled trial. Lancet 387, 1837–1846. doi: 10.1016/S0140-6736(16)00587-0
Gadgeel, S., Rodriguez-Abreu, D., Speranza, G., Esteban, E., Felip, E., Domine, M., et al. (2020). Updated analysis from KEYNOTE-189: pembrolizumab or placebo plus pemetrexed and platinum for previously untreated metastatic nonsquamous non-small-cell lung cancer. J. Clin. Oncol. 38, 1505–1517. doi: 10.1200/JCO.19.03136
Gandara, D. R., Paul, S. M., Kowanetz, M., Schleifman, E., Zou, W., Li, Y., et al. (2018). Blood-based tumor mutational burden as a predictor of clinical benefit in non-small-cell lung cancer patients treated with atezolizumab. Nat. Med. 24, 1441–1448. doi: 10.1038/s41591-018-0134-3
Haanen, J., Ernstoff, M., Wang, Y., Menzies, A., Puzanov, I., Grivas, P., et al. (2020). Rechallenge patients with immune checkpoint inhibitors following severe immune-related adverse events: review of the literature and suggested prophylactic strategy. J. Immunother. Cancer 8:e000604.
Jamal-Hanjani, M., Wilson, G. A., McGranahan, N., Birkbak, N. J., Watkins, T. B. K., Veeriah, S., et al. (2017). Tracking the evolution of non-small-cell lung cancer. N. Engl. J. Med. 376, 2109–2121. doi: 10.1056/NEJMoa1616288
Lou, Y., Xu, J., Zhang, Y., Zhang, W., Zhang, X., Gu, P., et al. (2021). Akt kinase LANCL2 functions as a key driver in EGFR-mutant lung adenocarcinoma tumorigenesis. Cell Death Dis. 12:170. doi: 10.1038/s41419-021-03439-8
Lu, J., Shi, Q., Zhang, L., Wu, J., Lou, Y., Qian, J., et al. (2019a). Integrated transcriptome analysis reveals KLK5 and L1CAM predict response to anlotinib in NSCLC at 3rd line. Front. Oncol. 9:886. doi: 10.3389/fonc.2019.00886
Lu, J., Zhong, H., Chu, T., Zhang, X., Li, R., Sun, J., et al. (2019b). Role of anlotinib-induced CCL2 decrease in anti-angiogenesis and response prediction for nonsmall cell lung cancer therapy. Eur. Respir. J. 53:1801562. doi: 10.1183/13993003.01562-2018
Lu, J., Zhong, H., Wu, J., Chu, T., Zhang, L., Li, H., et al. (2019c). Circulating DNA-based sequencing guided anlotinib therapy in non-small cell lung cancer. Adv. Sci. 6:1900721. doi: 10.1002/advs.201900721
McGrail, D. J., Pilie, P. G., Rashid, N. U., Voorwerk, L., Slagter, M., Kok, M., et al. (2021). High tumor mutation burden fails to predict immune checkpoint blockade response across all cancer types. Ann. Oncol. 32, 661–672. doi: 10.1016/j.annonc.2021.02.006
Mogi, A., and Kuwano, H. (2011). TP53 mutations in nonsmall cell lung cancer. J. Biomed. Biotechnol. 2011:583929. doi: 10.1155/2011/583929
Nagahashi, M., Sato, S., Yuza, K., Shimada, Y., Ichikawa, H., Watanabe, S., et al. (2018). Common driver mutations and smoking history affect tumor mutation burden in lung adenocarcinoma. J. Surg. Res. 230, 181–185. doi: 10.1016/j.jss.2018.07.007
Nie, W., Qian, J., Xu, M. D., Gu, K., Qian, F. F., Hu, M. J., et al. (2020). A non-linear association between blood tumor mutation burden and prognosis in NSCLC patients receiving atezolizumab. Oncoimmunology 9:1731072. doi: 10.1080/2162402X.2020.1731072
Peters, S., Camidge, D. R., Shaw, A. T., Gadgeel, S., Ahn, J. S., Kim, D. W., et al. (2017). Alectinib versus crizotinib in untreated ALK-positive non-small-cell lung cancer. N. Engl. J. Med. 377, 829–838. doi: 10.1056/NEJMoa1704795
Pinheiro, F. D., Teixeira, A. F., de Brito, B. B., da Silva, F. A. F., Santos, M. L. C., and de Melo, F. F. (2020). Immunotherapy–new perspective in lung cancer. World J. Clin. Oncol. 11, 250–259. doi: 10.5306/wjco.v11.i5.250
Ramos-Casals, M., Brahmer, J. R., Callahan, M. K., Flores-Chavez, A., Keegan, N., Khamashta, M. A., et al. (2020). Immune-related adverse events of checkpoint inhibitors. Nat. Rev. Dis. Primers 6:38. doi: 10.1038/s41572-020-0160-6
Rittmeyer, A., Barlesi, F., Waterkamp, D., Park, K., Ciardiello, F., von Pawel, J., et al. (2017). Atezolizumab versus docetaxel in patients with previously treated non-small-cell lung cancer (OAK): a phase 3, open-label, multicentre randomised controlled trial. Lancet 389, 255–265. doi: 10.1016/S0140-6736(16)32517-X
Samstein, R. M., Lee, C. H., Shoushtari, A. N., Hellmann, M. D., Shen, R., Janjigian, Y. Y., et al. (2019). Tumor mutational load predicts survival after immunotherapy across multiple cancer types. Nat. Genet. 51, 202–206. doi: 10.1038/s41588-018-0312-8
Shankar, B., Zhang, J., Naqash, A. R., Forde, P. M., Feliciano, J. L., Marrone, K. A., et al. (2020). Multisystem immune-related adverse events associated with immune checkpoint inhibitors for treatment of non-small cell lung cancer. JAMA Oncol. 6, 1952–1956. doi: 10.1001/jamaoncol.2020.5012
Shaw, A. T., Bauer, T. M., de Marinis, F., Felip, E., Goto, Y., Liu, G., et al. (2020). First-line lorlatinib or crizotinib in advanced alk-positive lung cancer. N. Engl. J. Med. 383, 2018–2029. doi: 10.1056/NEJMoa2027187
Zhang, L. L., Lu, J., Liu, R. Q., Hu, M. J., Zhao, Y. M., Tan, S., et al. (2020). Chromatin accessibility analysis reveals that TFAP2A promotes angiogenesis in acquired resistance to anlotinib in lung cancer cells. Acta Pharmacol. Sin. 41, 1357–1365. doi: 10.1038/s41401-020-0421-7
Keywords: UBL, biomarker, immune checkpoint inhibitors, atezolizumab, NSCLC
Citation: Lu J, Zhang Y, Lou Y, Yan B, Zou B, Hu M, Wang Y, Chen Y, Yang Z, Wang H, Zhang W and Han B (2021) ctDNA-Profiling-Based UBL Biological Process Mutation Status as a Predictor of Atezolizumab Response Among TP53-Negative NSCLC Patients. Front. Genet. 12:723670. doi: 10.3389/fgene.2021.723670
Received: 11 June 2021; Accepted: 09 August 2021;
Published: 07 September 2021.
Edited by:
Guoshuai Cai, University of South Carolina, United StatesCopyright © 2021 Lu, Zhang, Lou, Yan, Zou, Hu, Wang, Chen, Yang, Wang, Zhang and Han. This is an open-access article distributed under the terms of the Creative Commons Attribution License (CC BY). The use, distribution or reproduction in other forums is permitted, provided the original author(s) and the copyright owner(s) are credited and that the original publication in this journal is cited, in accordance with accepted academic practice. No use, distribution or reproduction is permitted which does not comply with these terms.
*Correspondence: Jun Lu, bHVqdW41MTJAeWFob28uY29t; Huimin Wang, Y2hlc3R3aG1AMTI2LmNvbQ==; Wei Zhang, emh3ZWkyMDAyQGhvdG1haWwuY29t; Baohui Han, MTg5MzA4NTgyMTZAMTYzLmNvbQ==
†These authors have contributed equally to this work