- 1Co-Innovation Center for Sustainable Forestry in Southern China, Nanjing Forestry University, Nanjing, China
- 2International Center of Bamboo and Rattan, Beijing, China
The U-box gene encodes a ubiquitin ligase that contains a U-box domain. The plant U-box (PUB) protein plays an important role in the plant stress response; however, very few studies have investigated the role of these proteins in Moso bamboo (Phyllostachys edulis). Thus, more research on PUB proteins is necessary to understand the mechanisms of stress tolerance in P. edulis. In this study, we identified 121 members of the PUB family in P. edulis (PePUB), using bioinformatics based on the P. edulis V2 genome build. The U-box genes of P. edulis showed an uneven distribution among the chromosomes. Phylogenetic analysis of the U-box genes between P. edulis and Arabidopsis thaliana suggested that these genes can be classified into eight subgroups (Groups I–VIII) based on their structural and phylogenetic features. All U-box genes and the structure of their encoded proteins were identified in P. edulis. We further investigated the expression pattern of PePUB genes in different tissues, including the leaves, panicles, rhizomes, roots, and shoots. The qRT-PCR results showed that expression of three genes, PePUB15, PePUB92, and PePUB120, was upregulated at low temperatures compared to that at 25°C. The expression levels of two PePUBs, PePUB60 and PePUB120, were upregulated under drought stress. These results suggest that the PePUB genes play an important role in resistance to low temperatures and drought in P. edulis. This research provides new insight into the function, diversity, and characterization of PUB genes in P. edulis and provides a basis for understanding their biological roles and molecular mechanisms.
Introduction
The ubiquitin-proteasome pathway (UPP) is the most important and best characterized pathway for selective protein degradation in eukaryotic organisms. It is also an important mechanism for the regulation of cellular functions such as Arabidopsis thaliana (Vierstra, 2009). Previous studies have shown that the UPP is closely connected to plant signaling, development, biotic and abiotic stresses, and ubiquitination (Zhang and X., 2005; Stone et al., 2006; Zhang et al., 2007). Ubiquitin (Ub) is a 76 amino acid (aa) protein that is widely found in eukaryotic organisms, and is highly conserved in sequence (Azevedo et al., 2001). The sequence of Ub amino acids in various higher plants analyzed thus far is almost identical, differing from the human ubiquitin sequence by only three amino acids (Callis and Vierstra, 1989). UPP ubiquitination of the substrate is completed by the cascade reaction of three key enzymes: Ub-activating enzyme (E1), ubiquitin-conjugating enzyme (E2), and ubiquitin-protein ligase (E3). In this pathway, E3 is the most abundant enzyme in the ubiquitin connection cascade system (Parveen et al., 2021). The Arabidopsis thaliana genome is estimated to contain more than 1,300 genes encoding E3s, making up more than 5% of the A. thaliana proteome, and these are responsible for controlling the regulation of nearly 2,600 target proteins (Zeng et al., 2008; Schulman and Wade Harper, 2009; Ye and Rape, 2009). Each cascade reaction pathway can be used as a targeted method to identify specific degradation signals. E3s in plants can be divided into four types: Homology to the E6AP C-Terminus (HECT) (Metzger et al., 2012), real interesting new gene (Ring)/U-box (Cyr et al., 2002), Skp1-cullin-F-box (SCF) (Gagne et al., 2002), and the anaphase-promoting complex (Harper et al., 2002). The U-box type E3 ubiquitin ligase contains a U-box domain consisting of 75 aa, which is a variant of the RING-type ring finger structural domain. The main difference between the U-box type and the RING-type structural domains is that the U-box domain maintains its conformation throughout interactions with components such as hydrogen and salt bonds (Donna and Daphne, 2009; Dielen et al., 2010; Metzger et al., 2012). Analysis of their structure provides an in-depth understanding of the mechanism of action of these enzymes (Hellmann, 2002; Vierstra, 2009; Downes et al., 2010).
PUB genes are widely expressed in plants and have been reported in many higher plants, including A. thaliana (Jakob et al., 2008), rice (Zeng et al., 2008), cotton (Lu et al., 2020), soybean (Ning et al., 2016), Chinese cabbage (Wang et al., 2015), banana (Hu et al., 2018), and apples (Wang et al., 2020). Currently, 64 PUB genes, which perform multiple functions, have been identified in A. thaliana (Jakob et al., 2008). There are 125 PUB genes in soybeans, which are divided into eight subtypes based on their protein domains (Ning et al., 2016). A total of 101 PUB genes have been identified in Chinese cabbage, which can be divided into 10 subtypes according to their protein domains (Wang et al., 2015). In rice, 77 PUB genes have been identified, which can be divided into nine subtypes. Several studies have shown that PUB proteins are involved in the regulation of plant hormone signal transduction, stress tolerance, and insect resistance (Zeng et al., 2008). In the pepper plant, CaPUB1 acts as an E3 ubiquitin ligase to negatively regulate dehydration and high salt stress. CaPUB1 achieves this by promoting the degradation of the 26 S proteasome subunit through the ubiquitination pathway (Cho et al., 2008). Overexpression of CaPUB1 in transgenic tissues significantly upregulates the expression of the drought stress marker gene, RD29a (Cho et al., 2006). CaPUB1 homologs in A. thaliana, AtPUB22 and AtPUB23, can also interact with the 26 S proteasome subunit AtPRN12a. PUB22 and PUB23 double mutants display increased resistance to drought and salt stress, with AtPUB22 and AtPUB23 showing functional redundancy (Cho et al., 2008). Moreover, the A. thaliana PUB22/23/24 genes belonging to the Group II subtype, have been shown to play a role in plant resistance. The PUB22/23/24 triple mutant displays an enhancement in the pattern-triggered immunity (PTI) pathway activated by pathogen-associated molecular patterns that results in improved resistance to bacteria (Trujillo et al., 2008; Chen et al., 2014). Furthermore, AtPUB30 in A. thaliana is induced by salt stress, with AtPUB30 mutant tissues being more resistant to salt stress during seed germination (Hwang et al., 2015).
Mosa bamboo (Phyllostachys edulis) is a uniaxial scattered bamboo species belonging to the Gramineae family. P. edulis is native to China and is one of the most important bamboo species in the world, occupying the largest cultivation area and possessing the greatest economic value (Fu, 2001; Vorontsova et al., 2016). P. edulis was introduced to Japan in 1736 and has since become the second most economically valuable bamboo species in the country. P. edulis was later introduced to South Korea, the Philippines, Myanmar, Vietnam, Thailand, India, Europe, and America (Fu, 2001). This species is characterized by rapid growth, which is affected by various environmental factors (Banik, 2015). Further research on its growth and resistance to environmental stresses is important to improve cultivation and downstream applications. Sequencing of the P. edulis V2 genome was completed in 2018 (Zhao et al., 2018), and multiple growth (Wang et al., 2019; Yang et al., 2019; Lan et al., 2020; Wang et al., 2021) and stress-related genes (Hou et al., 2018; Cheng et al., 2019; Hou et al., 2020; Liu et al., 2020) have been extensively studied. However, research on the PUB gene family in P. edulis is lacking. In this study, we used the P. edulis V2 genome build (Zhao et al., 2018) to conduct a genome-wide identification of PUB genes and analyze of their gene structure and evolution. We also investigated the expression of PUBs in different tissues. This research provides a reference for future follow-up and in-depth studies of PUB gene function in P. edulis.
Materials and Methods
PUB Gene Identification
The hidden Markov model (HMM) file corresponding to the U-box domain (PF04564) was obtained from the Pfam database (http://pfam.xfam.org/) (Mistry et al., 2021). HMMER 3.0 was used to search the U-box domain from the Genome Database of Bamboos (http://www.bamboogdb.org/#/) (Zhao et al., 2014). To ensure accuracy, BLAST 2.9.0+ (http://www.bamboogdb.org/#/blast) (Priyann et al., 2019) was used for BLASTP (e > 1.0 e-10) screening of homologous genes from previously identified P. edulis PUB genes. The PFAM (Mistry et al., 2021) and SMART (Letunic et al., 2021) programs were used to verify the presence of the U-box core sequence. Candidate genes that potentially contain the U-box domain based on the HMMER results were further individually verified by manual inspection. The tools on the ExPasy website (Panu et al., 2012) were used to obtain the sequence length, molecular weight (MW), and isoelectric point (pI). The subcellular localization of PePUB proteins was predicted using the online tool Plant-mPLoc (http://www.csbio.sjtu.edu.cn/bioinf/plant-multi/) (Chou and Shen, 2010).
Phylogenetic Analysis and Classification of Moso Bamboo PUB Gene Family
A phylogenetic tree for the sequence alignment of PePUB genes was constructed using the maximum parsimony (MP) method in MEGA 7.0. The bootstrap replications were set to 1,000 to estimate the accuracy of the phylogenetic tree. EvolView (Subramanian et al., 2019) was used to visualize the unrooted phylogenetic tree. The PUB protein sequence of P. edulis was analyzed using the online program MEME (https://meme-suite.org/meme/tools/meme) (Bailey and Elkan, 1994) with parameters set to maximum motifs of 10, and the best group width set to between 6 and 50.
Chromosomal Distribution, Gene Duplication and Synteny Analysis
The BLAST module in TBtools software (Chen et al., 2020) was used for pairwise comparisons of the genomes of P. edulis against Oryza sativa (Ouyang et al., 2007) and P. edulis against A. thaliana (Cheng et al., 2017) The Multiple Collinearity Scan toolkit (MCScanX) (Wang et al., 2012), with default parameters, was used to obtain the chromosome distribution and interspecific covariance of the PUB gene family. The intraspecific and interspecific covariance results were visualized using Circos (http://circos.ca/) (Krzywinski et al., 2009) and multiple synteny plots (TBtools module) (Chen et al., 2020).
Cis-Regulatory Elements of PUB Genes in Phyllostachys edulis
The 2,000 bp sequence upstream of PePUB genes was extracted as the promoter sequence and used in the PlantCare tool (http://bioinformatics.psb.ugent.be/webtools/plantcare/html/) (Lescot et al., 2002) to identify cis-acting elements.
Plant Materials and Growth Conditions
The seeds of wild-type P. edulis were obtained from Guilin, Guangxi Province (110.38, 25.51) and planted in the White Horse Nursery of Nanjing Forestry University (119.18, 31.61). The seedlings were transplanted into an incubator, and the cycle conditions were 24°C with light for 16 h and 21°C without light for 8 h. Three-year-old P. edulis seedlings with an above ground height of approximately 80 cm were treated with 20% PEG 6000 and a temperature of 4°C to simulate drought and cold stress, respectively. Young leaves in a good growth state were collected at 0, 3, 6, and 12 h of treatment, snap frozen in liquid nitrogen, and stored at -80°C until RNA extraction.
Tissue Expression Patterns of PePUB Genes
To study the tissue expression patterns of the PePUB genes, transcriptome data from different tissues were obtained through the NCBI Short Read Archive database (https://www.ncbi.nlm.nih.gov/sra/) (accession number SRX2408,703) (Peng et al., 2013). The method of analysis was previously described by Peng et al., 2013.
Expression abundance is described as transcripts per million (TPM). TBtools Amazing Heatmap (Chen et al., 2020) was used to draw gene expression heat maps using a log2 (TPM + 1) scale.
Total RNA Extraction and cDNA Synthesis
Total RNA was extracted using the TRIzol reagent kit (Invitrogen Scientific, Inc., Carlsbad, CA, United States) according to the manufacturer’s protocol. RNA quality was assessed using an Agilent 2,100 Bioanalyzer (Agilent Technologies, Palo Alto, CA, United States) and RNase-free agarose gel electrophoresis. After total RNA was extracted, eukaryotic mRNA was enriched using Oligo (dT) beads, while prokaryotic mRNA was enriched by removing rRNA using the Ribo-ZeroTM Magnetic Kit (Epicenter, Madison, WI, United States). The enriched mRNA was then fragmented using fragmentation buffer, and reverse transcribed into cDNA using random primers. Second-strand cDNA was synthesized using DNA polymerase I, RNase H, and dNTPs. cDNA was stored at −20°C for until use.
Quantitative Real-Time PCR Analyses
Primer Express 5.0 (Singh and Pandey, 2015) was used to design gene-specific primers (listed in Schedule 1). Primer specificity was confirmed by a BLAST search using data downloaded from the BambooGDB and local CDS databases (http://www.bamboogdb.org/#/blast). The tonoplast intrinsic protein (TIP41) (Supplementary Table S6) was used as the reference gene (Fan et al., 2013). qRT-PCR was performed using SYBR Green Master Mix (Applied Biosystems) and samples were run in a CFX 96 Real-Time system (Applied Biosystems). The cycle conditions were as follows: denaturation for 5 s at 95°C, annealing at 55°C for 15 s, and extension at 65°C for 5 s. To ensure the accuracy of the results, three biological replicates and three technical replicates were performed for each sample. PCR products from each sample were verified by DNA sequencing. The fold change in RNA transcripts was calculated using the 2−ΔΔCt method.
Results
Identification of the PePUB Proteins in Moso Bamboo
HMMER 3.0 analysis identified 120 candidate genes corresponding to the Pfam PUB family, while BLASTp identified 100 candidate genes. P. edulis transcriptome data were also used to confirm candidate genes. Of the 220 identified candidate genes, 16 were found to have been incorrectly identified and 83 were redundant. The remaining 121 candidate genes were annotated as PePUB genes, based on the presence of a complete U-box domain. These genes were renamed PePUB01 to PePUB121 based on their chromosomal location (Supplementary Table S1).
We analyzed the gene characteristics, including the Gene ID, chromosomal location, length of the open reading frame, length of the protein sequence, protein MW, theoretical pI, and subcellular localization (Supplementary Table S1). Among the 121 PePUB genes, PePUB118 was identified as the smallest protein (188 aa), while PePUB117 was the largest (1,359 aa). The MW of the proteins ranged from 8.21 to 117.54 kDa, and the pI ranged from 4.65 (PePUB92) to 9.49 (PePUB51). The subcellular localization of all 121 PePUBs was predicted to be in the nuclear region, while six PePUBs were also located in the chloroplast.
Figure 3A shows the chromosomal location of the PePUB genes. Of the 121 PePUB genes, 117 could be mapped to the 20 chromosomes that make up the genome of P. edulis. However, four genes (PH02Gene24079.t1, PH02Gene49698.t1, PH02Gene50722.t1, and PH02Gene51283.t1) could not be localized to specific chromosomal locations using the P. edulis V2 genome. The largest number of PUB genes was found on chromosome 17, which contained 18 PePUB genes. Chromosomes 8 and 13 contained 11 PePUB genes. Nine PePUB genes were found on chromosome 3. Chromosomes 6, 23, and 24 each contained eight PePUB genes. Six PePUB genes were located on chromosome 20. Chromosomes 5 and 16 each contained four PePUB genes. Chromosomes 12 and 22 each contained three PePUB genes. Chromosomes 7, 9, 11, and 18 each contained two PePUB genes. Only one PePUB gene was located on chromosome 19.
Multiple Sequence Alignment, Phylogenetic Analysis, and Classification of PePUB Genes
A phylogenetic tree was constructed using the neighbor-joining method to investigate the relationships among the 121 PePUB genes. Phylogenetic analysis showed that these genes can be classified into eight subtypes (Groups I-VIII; Figure 1A). The protein sequence of all 121 PePUB genes are listed in Supplementary Table S1. Of the eight subgroups, Group II is the largest with 45 genes. Group VI was the smallest with only one gene.
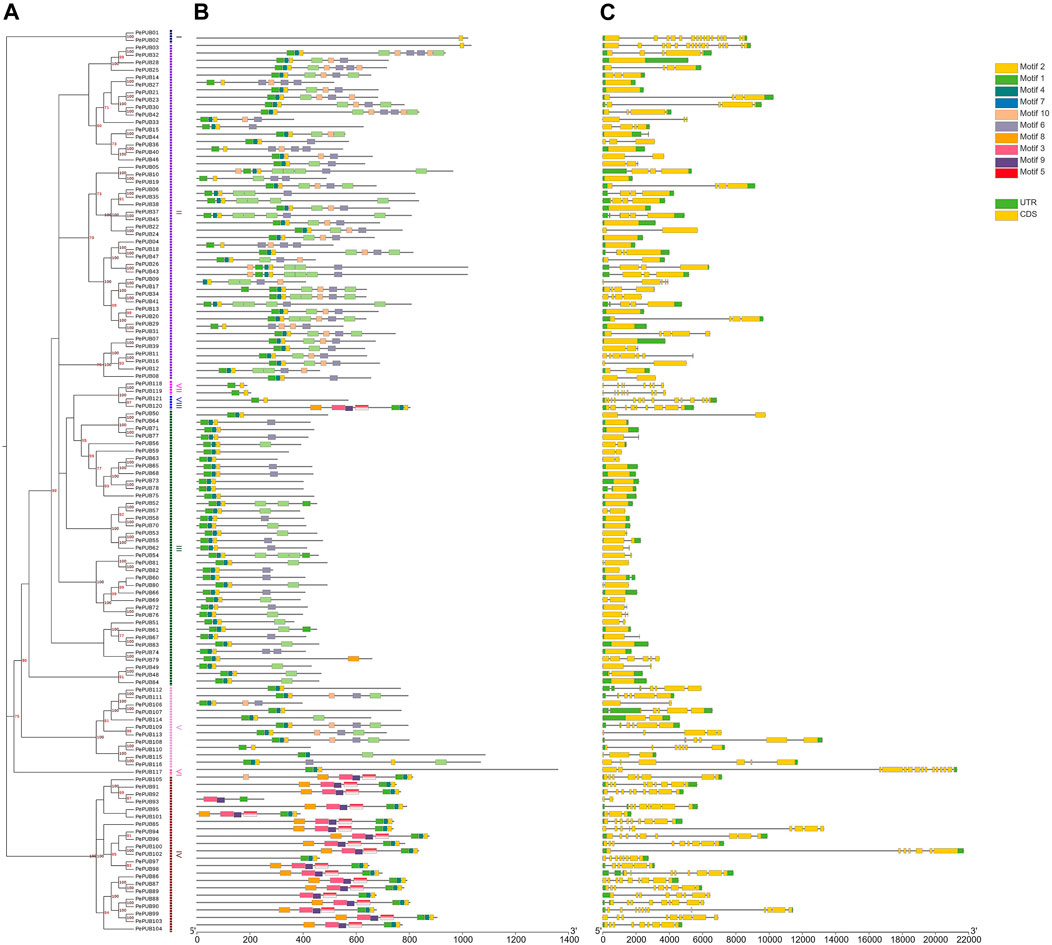
FIGURE 1. The unrooted phylogenetic tree, motif analyses, and the gene structure of PePUB genes. (A) The neighboring tree on the left consists of 121 PePUB proteins from Phyllostachys edulis. The right side of the phylogenetic tree lists the groups. (B) The 10 conserved groups are indicated by different colored boxes. (C) Yellow indicates the untranslated 5 and 3′ regions, and green indicates the exons.
To gain a more complete understanding of the composition and function of the PePUB genes, we investigated conserved sequences and exon/intron positions. Gene structure analysis indicated that the number of exons in PePUB genes varied from 0 to 15. Phylogenetic results based on the PUB gene family indicated that members of the same subgroup had similar exon/intron structures (Figure 1C). Most of the 37 genes in Group III contained one to three exons, while genes in Group I contained the most exons with all the genes in this group having 16 exons. In conclusion, the coding region structure of the PePUB genes are diverse, but there is some similarity among members of the same subgroup. This gene structure analysis corroborated the results of our phylogenetic analysis.
To further investigate the different structures of PUB genes, specific base sequences were evaluated using the MEME program. Ten conserved base sequences were identified and predicted for the 121 PePUB genes. The results showed that genes in the same subgroup exhibited a conserved base sequence with similar functions. At least one to four primary base sequences were present in all amino acid sequences (1, 2, 4, and 7; Figure 1B). Base sequences one and four are conserved U-box sequences, whereas the other sequences remained unpredictable.
To further investigate the evolution of the PUB family, 182 PUB proteins (121 from P. edulis and 61 from Arabidopsis thaliana) (Supplementary Table S2) were used to generate a phylogenetic tree (Figure 2A). We found that the PUB protein groupings were consistent with previous results and could be divided into eight subgroups. This further supports the accuracy of our results.
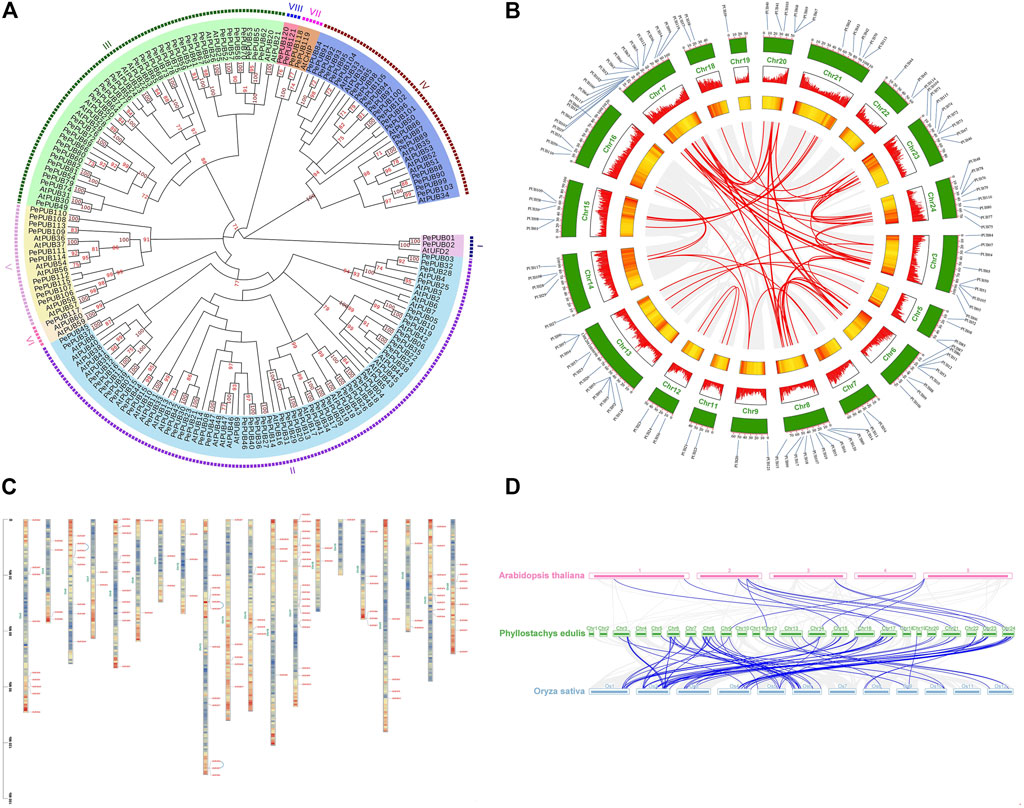
FIGURE 2. Localization and synteny of PePUB in Phyllostachys edulis genome. (A) Phylogenetic analysis of PUB genes from Phyllostachys edulis and Arabidopsis thaliana. (B) Localization of PUB on the chromosomes. Chromosome or scaffold number was indicated on the outer side. Yellow represented different chromosomes. The scale in the figure represents the chromosome length (Mb). The different colors represent the gene density of the chromosomes (increasing gene density from blue to red). The ligature between genes represents the tandem duplication event. The Whole genome duplications (WGD)/segmental duplication genes were connected by blue lines. (C) Distribution of gene duplications among PePUB genes. (D) Collinearity analysis between Phyllostachys edulis and Arabidopsis thaliana and Oryza sativa. The gray line represents the co-collinearity of all genes among the three species, and the blue line represents the collinearity among members of the PUB gene family.
Chromosomal Distribution and Gene Duplication of Moso Bamboo PUB Gene Family
The chromosome distribution of the PePUB gene family is shown in Figure 2B. A total of 121 genes were distributed among the 20 chromosomes and four scaffolds. Gene distribution was uneven between chromosomes. For example, 18 PePUB genes were located on chromosome 17, while only one PePUB gene was found on chromosome 19. Gene duplication also occurred, with three pairs of genes being duplicated in parallel. As shown in Supplementary Tables S3, S4; Figure 2C, 94 genes were derived from whole genome duplication (WGD) and segmental duplication; 20 genes were assigned to dispersed duplication blocks while four genes were assigned to tandem duplication blocks. Only 2.48% were derived from proximal duplication. These results suggest that WGD and segmental duplication events can cause the expansion of the PUB gene family. The relationship between P. edulis, A. thaliana, and rice is shown in Figure 2D. When compared with PUB genes in P. edulis, only 13 PUB homologs were found in the A. thaliana genome, while 93 PUB homologs were found in the rice genome. This shows that P. edulis and rice are genetically closely related. In addition, 13 PUB genes in rice had two homologous copies in P. edulis, while some had three copies. There were also 28 PUB genes in rice that had no corresponding copies in P. edulis. This may have resulted from a whole genome polyploidy event during the evolution of the PUB gene family.
Cis-Regulatory Element Analyses of PePUB Genes
We analyzed specific promoter regions of the 121 PePUB genes (Figure 3 and Supplementary Table S5) and examined the response of the cis-elements to biotic and abiotic stress. In addition to basic cis-elements, such as the CAAT-box and TATA-box, cis-elements are divided into four groups: hormone-responsive, light-responsive, plant growth and development-related, and stress-related. The results showed that 114 genes had hormone-responsive cis-elements, 117 genes had light-responsive cis-elements, 98 genes had plant growth and development-related cis-elements, and 116 genes had hormone-responsive cis-elements. Hormone-responsive cis-elements included ABRE, AuxRR-core, CGTCA-motif, ERE, GARE-motif, P-box, TATC-box, TCA-element, TGA-box, TGACG-motif, and TGA-element cis-elements. Light-responsive cis-elements included Box 4, G-box, GT1-motif, GATA-motif, MRE, AE-box, TCCC-motif, I-box, chs-CMA, Sp1, TCT-motif, ACE, and ATCT-motif. Development-related cis-elements included A-box, AT-box, AT-box, TCCC-motif, I-box, chs-CMA, Sp1, TCT-motif, ACE, and ATCT-motif rich element. Stress-related cis-elements included ARE, GC-motif, GCN4-motif, HD-Zip 1, MBSI, MSA-like, NON-box, O2-site, plant AP-2-like, and RY-element. LTR, MBS, TC-rich repeats, and WUN-motif are closely related to anaerobic induction, drought induction, low-temperature responsiveness, defense, stress responsiveness, wound-responsive elements, and hypoxia-specific induction.
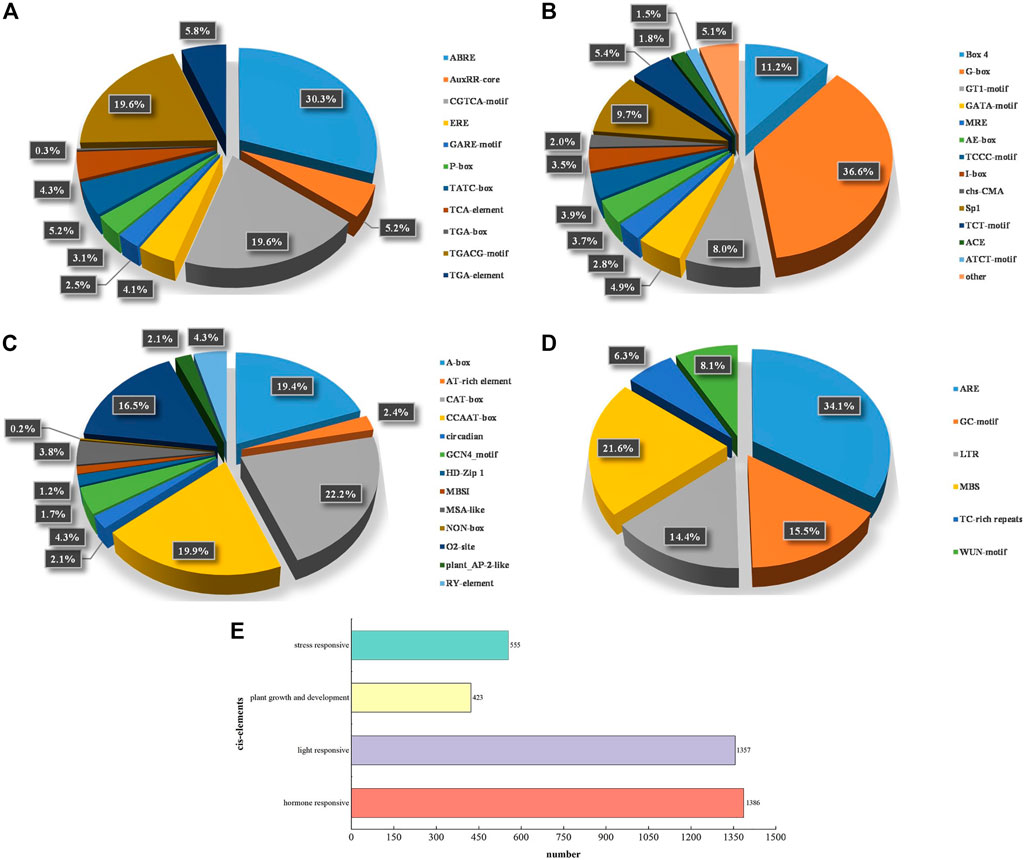
FIGURE 3. The analyses of cis-acting elements found in PePUB genes. (A) hormone-responsive, (B) light-responsive, (C) plant growth and development, (D) stress-responsive. (E) The sum of cis-elements in the four categories. Different colors indicate different cis-acting elements and the ratio of cis-elements present in PePUB genes.
Expression Analyses of PePUB Genes in Different Tissues of Moso Bamboo Based on RNA-Seq Dataset
Expression heat maps of transcriptome data were created (Figure 4A) to explore the potential functions of the PePUB genes. We investigated the expression of PePUB genes in the leaves, panicles, rhizomes, roots, and shoots of P. edulis. We used fragments per kilobase of exon model per million mapped fragments (FPKM) to obtain PePUB gene expression abundance TPM and analyzed the expression profile of PUB gene by log2 (TPM + 1) values for the gene expression heat map. The results showed that the following fractions of TPM values were greater than 1: 72% (87/121) of the panicle, 65% (79/121) of the leaf, 55% (67/121) of the root, 51% (62/121) of the rhizome, and 43% (53/121) of the shoots.
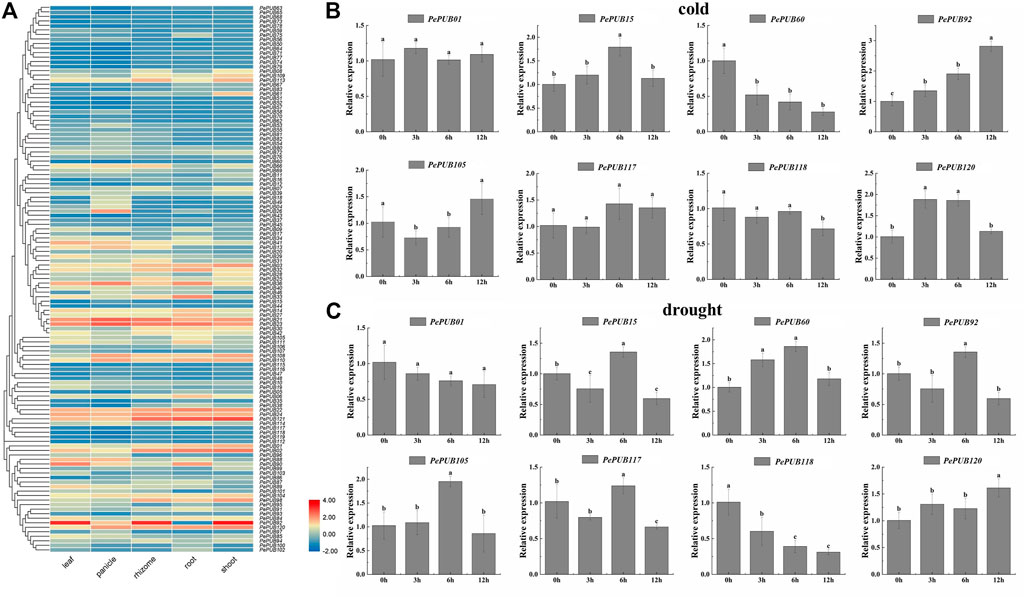
FIGURE 4. (A) Tissue-specific gene expression patterns of 121 PePUB genes. The expression patterns of 121 PePUB genes in leaf, panicle, rhizome, root, and shoot. The color scale illustrates the log2 (TPM + 1), red and blue colors reveal the high or low transcript abundance, respectively. (B) Expression profiles of eight candidate PePUB genes under low temperature treatment. (C) Expression profiles of eight candidate PePUB genes under drought stress. The values on X axis and Y axis represent the time point (hour) and the relative gene expression levels, respectively. The error strip represents three independent standard deviations (SD). Biological repetition. Different lowercase letters showed significant differences, which were determined by one-way analysis of variance, and then Duncan’s multi-range test (p < 0.05).
qRT-PCR Verification of Selected PePUB Genes in Different Stress
Low temperature and drought are the most important factors affecting the survival of P. edulis. Therefore, it is important to analyze whether PePUB genes are responsive to low-temperature and drought-stress treatments.
To test the role of PePUB genes under low temperature stress, eight PePUB genes from eight different subgroups (PePUB01, PePUB15, PePUB60, PePUB92, PePUB105, PePUB117, PePUB118, and PePUB120) were randomly selected for qRT-PCR analysis (Figure 4B). The expression levels of PePUB15, PePUB92, PePUB118, and PePUB120 were upregulated at all treatment time points in low temperature conditions. However, PePUB60 expression was downregulated throughout the treatment. The expression levels of both PePUB92 and PePUB105 were highest at 12 h PePUB01 and PePUB117 showed no significant difference in expression levels between the control and treatment groups.
To assess the response of PePUB genes to drought conditions, eight PePUB genes from different subgroups, including PePUB01, PePUB15, PePUB60, PePUB92, PePUB105, PePUB117, PePUB118, and PePUB120, were selected for qRT-PCR analysis (Figure 4C). The expression levels of three PePUB genes (PePUB60, PePUB105, and PePUB117) reached their highest levels at 6 h. Their expression patterns were different; PePUB118 showed a decreasing pattern compared to that in the control group, and four PePUB genes (PePUB01, PePUB15, PePUB92, and PePUB120) did not significantly differ from those in the control group.
Discussion
During growth and development, P. edulis is affected by various environmental and abiotic stresses, such as seasonal drought or flooding, and this can seriously affect the yield and quality of bamboo timber and bamboo shoots. Temperature and moisture are particularly important factors that limit the growth of P. edulis. In particular, soil salinization in China is another important limiting factor (Shi et al., 2020). The release of the first- and second-generation genome builds of P. edulis has significantly increased the identification of transcription factors and protein families involved in the regulation of P. edulis yield, quality, and stress tolerance (Zhang et al., 2005; Stone et al., 2006; Zhang et al., 2007). However, little is known about the PUB gene family in P. edulis. The PUB gene family has been shown to play important roles in several physiological activities in many plants, including phytohormones and light signaling. These genes are also involved in the regulation of cell cycle, transcription, and self-recognition in response to biotic and abiotic stresses. The properties and functions of the PUB gene family have been studied in a variety of plants. In this study, bioinformatics analysis of the PUB genes in the P. edulis genome identified 121 PePUB genes. These genes were found to be unevenly distributed among chromosomes. PePUB genes in the same subgroup were found to have similar gene structures, while gene structure differed among subgroups. The diversity of the gene structures further suggests that PePUB proteins may have diverse biological functions.
The Function of Different Subgroups of PePUB Genes
The conserved U-box domain of the PePUB genes is often found in combination with other domains, such as ARM repeats, WD40 repeats, and TRR structures. The PePUB genes can be divided into eight subgroups based on phylogenetic analysis of the PUB domains. Group I in P. edulis consists of two genes, whereas in A. thaliana, there is only one gene (AtUFD2). AtUFD2 contains a conserved domain similar to that of the yeast UFD2 protein and can interact with the AAA-type ATPase CDC48 to participate in the regulation of cell cycle, death, organelle formation, and other physiological activities. Group II contains the largest number of genes, with 47 genes in P. edulis, and 30 genes in A. thaliana. In addition to the U-box domain, Group II genes contain an ARM repeat at the C-terminus, which allows protein–protein interactions to regulate physiological activities in the cell. Group III contains 34 genes all of which have an isomerase domain. Group IV in P. edulis contains 21 genes, while in A. thaliana, there are eight genes. Group IV genes contain a kinase domain at the N-terminus of the U-box domain, indicating that these proteins are involved in signal transduction via phosphorylation, in addition to being modified by ubiquitination. Group V in P. edulis contains 12 genes, and in A. Thaliana, there are seven genes. Group V genes only have U-box domains, while WD40 repeats are involved in signaling, transcriptional regulation, and regulation of various cellular functions through protein interactions. Group VII in P. edulis consists of two genes. PUBs in group VII contain the TRR domain, a 34 aa protein repeating sequence that often exhibits scaffold-like structures and is involved in protein–protein interactions. Group VIII in P. edulis consists of two genes, while there are none in A. thaliana.
In the structural analysis of the PePUB genes, we systematically analyzed highly conserved sequences (as indicated by phylogenetic analysis) and found that PePUB proteins in the same subgroup share a remarkably similar sequence. We also found many cis-elements that were commonly associated with various developmental, adversity-response, and stress-response factors. Ubiquitin ligase regulates the ABA signaling pathway and ABA synthesis (Pankaj et al., 2021), resulting in the inhibition of leaf senescence (Lim et al., 2017). There were 103 PePUB genes that contained methyl jasmonate response (MeJA) cis-acting elements (TGACG and CGTCA). Both local and systemic responses are rapid and co-regulated by mechanical damage and herbivory. A previous study showed that ethylene and growth hormones can limit metabolism in tomato fruits through a light-signaling cascade (Aline et al., 2018). The CAT-box (GCCACT) and CGTCC were associated with meristematic expression and were found in 61 and 45 PePUB genes, respectively. TGA-elements (AACGAC) (Kumar et al., 2018) and the growth hormone suppressor core sequence (GGTCCAT) were found in 52 and 18 growth hormone-responsive PePUB genes, respectively. The HD-ZIP promoter found in seven PePUB genes provides protein-binding sites and is closely involved in leaf sarcomere differentiation and trichome development (Wang et al., 2013). Of the PePUB genes, 70 were found to contain the MBS motif (TAACTG), 32 genes were found with TC-rich repeats (GTTTTCTTAC), 61 genes with anaerobic induction-related cis-elements (GCCCCGG and TGGTTT), 91 genes with low-temperature and drought-response cis-regulation (GGCCGACAT), and 43 genes with other adversity-response elements. These elements were shown to rapidly regulate gene expression in response to changes in external stimuli. These findings provide a considerable advancement to our understanding of the molecular mechanisms of PePUB gene functions.
Potential Functions and Expression Analyses of PePUB Genes
As previously mentioned, P. edulis is an important temperate woody bamboo species. Persistent and extremely low temperatures, annual effective cumulative temperature, and rainfall from March to April (the shooting period) and September (the rhizome growth period) are the main factors that limit the spread of the species (Shi et al., 2020). Previous studies have shown that PUB genes play an important role in the regulation of plant growth, development, and stress response (Azevedo et al., 2001). In this study, cis-element analysis of the PePUB promoters revealed the presence of elements associated with plant growth, development, and stress response. We also investigated the expression pattern of PePUBs in response to low temperatures and drought stress using qRT-PCR. Many studies have shown that PUB genes play an important role in the plant stress response. For example, overexpression of AtCHIP leads to increased sensitivity to temperature stress in A. thaliana (Yan et al., 2003). In this study, the P. edulis homolog, PePUB120, was identified with an identical protein class and subcellular localization. Significant gene expression changes in response to cold stress indicated that the homologous genes were functionally similar. We also found that the PePUB15 and PePUB92 genes responded positively to cold stress, and no homologs were found in A. thaliana or rice. qRT-PCR analysis showed high expression of PePUB60 and PePUB120 was in drought conditions. This suggests that PePUB genes play an important role in the response to adverse stress. In conclusion, we propose that PePUB15, PePUB60, PePUB92, and PePUB120 are important candidates for improving P. edulis growth and quality.
Conclusion
In this study, we investigated the PUB gene family in P. edulis by analyzing the genome and transcriptome, and identified 121 PePUB genes. To better understand the structure and function of PePUB genes, we analyzed their gene structure, protein motifs, and cis-acting elements in the promoter. WGD and segmental duplication were the main causes of the expansion of the PUB gene family. The expression pattern of PePUB genes was altered under different stress conditions. In addition, we found that PePUB15, PePUB60, PePUB92, and PePUB120 responded positively to abiotic stress. This study provides a basis for a comprehensive understanding of the PUB genes in P. edulis and lays the foundation for future studies.
Data Availability Statement
The RNA-seq data were deposited in the Sequence Read Archive (SRA) of the National Center for Biotechnology Information (https://www.ncbi.nlm.nih.gov/sra) under the accession number SRX2408703.
Author Contributions
JZ and QG conceived the experimental design. YH, ZY and JL performed the experiments. JZ and YH analysed the data. JZ and QG wrote the manuscript with contributions from all authors.
Funding
This research was partially supported by the National Natural Science Foundation of China: 31971648 and the Talent Introduction Project of Nanjing Forestry University: GXL2018001.
Conflict of Interest
The authors declare that the research was conducted in the absence of any commercial or financial relationships that could be construed as a potential conflict of interest.
Publisher’s Note
All claims expressed in this article are solely those of the authors and do not necessarily represent those of their affiliated organizations, or those of the publisher, the editors and the reviewers. Any product that may be evaluated in this article, or claim that may be made by its manufacturer, is not guaranteed or endorsed by the publisher.
Acknowledgments
The authors would like to thank Co-Innovation Center for Sustainable Forestry in Southern China for helpful discussions on topics related to this work.
Supplementary Material
The Supplementary Material for this article can be found online at: https://www.frontiersin.org/articles/10.3389/fgene.2021.710113/full#supplementary-material
References
Aline, B. C., Ricardo, E. B., Frederico, R. R. A., Eduardo, P., Lazaro, E. P. P., Magdalena, R., et al. (2018). Light, Ethylene and Auxin Signaling Interaction Regulates Carotenoid Biosynthesis during Tomato Fruit Ripening. Front. Plant Sci. 9, 1370. doi:10.3389/fpls.2018.01370
Azevedo, C., Santos-Rosa, M. J., and Shirasu, K. (2001). The U-Box Protein Family in Plants. Trends Plant Sci. 6, 354–358. doi:10.1016/S1360-1385(01)01960-4
Bailey, T. L., and Elkan, C. (1994). Fitting a Mixture Model by Expectation Maximization to Discover Motifs in Biopolymers. Proc. Int. Conf. Intell. Syst. Mol. Biol. 2, 28–36.
Callis, J., and Vierstra, R. D. (1989). Ubiquitin and Ubiquitin Genes in Higher Plants. Plant Mol. Cel Biol 6, 1–30.
Chen, C. J., Chen, H., Zhang, Y., Thomas, H. R., Frank, M. H., He, Y., et al. (2020). TBtools: An Integrative Toolkit Developed for Interactive Analyses of Big Biological Data. Mol. Plant 13, 1194–1202. doi:10.1016/j.molp.2020.06.009
Chen, Y. C., Wong, C. L., Muzzi, F., Vlaardingerbroek, I., Kidd, B. N., and Schenk, P. M. (2014). Root Defense Analysis against Fusarium Oxysporum Reveals New Regulators to Confer Resistance. Sci. Rep. 4, 1–10. doi:10.1038/srep05584
Cheng, C.-Y., Krishnakumar, V., Chan, A. P., Thibaud-Nissen, F., Schobel, S., and Town, C. D. (2017). Araport11: a Complete Reannotation of the Arabidopsis thaliana Reference Genome. Plant J. 89, 789–804. doi:10.1111/tpj.13415
Cheng, X. R., Xiong, R., Yan, H. W., Gao, Y. M., Liu, H. L., Wu, M., et al. (2019). The Trihelix Family of Transcription Factors: Functional and Evolutionary Analysis in Moso Bamboo (Phyllostachys Edulis). BMC Plant Biol. 19, 1–20. doi:10.1186/s12870-019-1744-8
Cho, S. K., Chung, H. S., Ryu, M. Y., Park, M. J., Lee, M. M., Bahk, Y.-Y., et al. (2006). Heterologous Expression and Molecular and Cellular Characterization of CaPUB1 Encoding a Hot Pepper U-Box E3 Ubiquitin Ligase Homolog. Plant Physiol. 142, 1664–1682. doi:10.1104/pp.106.087965
Cho, S. K., Ryu, M. Y., Song, C., Kwak, J. M., and Kim, W. T. (2008). Arabidopsis PUB22 and PUB23 Are Homologous U-Box E3 Ubiquitin Ligases that Play Combinatory Roles in Response to Drought Stress. Plant Cell 20, 1899–1914. doi:10.1105/tpc.108.060699
Chou, K.-C., and Shen, H.-B. (2010). Plant-mPLoc: A Top-Down Strategy to Augment the Power for Predicting Plant Protein Subcellular Localization. PLoS One 5, e11335. doi:10.1371/journal.pone.0011335
Cyr, D. M., Hohfeld, J., and Patterson, C. (2002). Protein Quality Control: U-Box-Containing E3 Ubiquitin Ligases Join the Fold. Trends Biochem. Sci. 27, 368–375. doi:10.1016/s0968-0004(02)02125-4
Dielen, A. S., Saloua, B., Thierry, C., and Sylvie, G. R. (2010). The ubiquitin/26S Proteasome System in Plant–Pathogen Interactions: a Never‐ending Hide‐and‐seek Game. Mol. Plant Pathol. 11, 293–308. doi:10.1111/j.1364-3703.2009.00596.x
Donna, Y., and Daphne, R. G. (2009). The Diversity of Plant U-Box E3 Ubiquitin Ligases: from Upstream Activators to Downstream Target Substrates. J. Exp. Bot. 60, 1109–1121. doi:10.1093/jxb/ern369
Downes, B. P., Stupar, R. M., Gingerich, D. J., and Vierstra, R. D. (2010). The HECT Ubiquitin-Protein Ligase (UPL) Family in Arabidopsis: UPL3 Has a Specific Role in Trichome Development. Plant J. 35, 729–742. doi:10.1046/j.1365-313X.2003.01844.x
Fan, C., Ma, J., Guo, Q., Li, X., Wang, H., and Lu, M. (2013). Selection of Reference Genes for Quantitative Real-Time PCR in Bamboo (Phyllostachys Edulis). PLoS One 8, e56573. doi:10.1371/journal.pone.0056573
Gagne, J. M., Downes, B. P., Shiu, S.-H., Durski, A. M., and Vierstra, R. D. (2002). The F-Box Subunit of the SCF E3 Complex Is Encoded by a Diverse Superfamily of Genes in Arabidopsis. Proc. Natl. Acad. Sci. 99, 11519–11524. doi:10.1073/pnas.162339999
Harper, J. W., Burton, J. L., and Solomon, M. J. (2002). The Anaphase-Promoting Complex: It's Not Just for Mitosis Any More. Genes Dev. 16, 2179–2206. doi:10.1101/gad.1013102
Hellmann, H. (2002). Plant Development: Regulation by Protein Degradation. Science 297, 793–797. doi:10.1126/science.1072831
Hou, D., Cheng, Z. C., Xie, L. H., Li, X. Y., Li, J., Mu, S. H., et al. (2018). The R2R3MYB Gene Family in Phyllostachys Edulis: Genome-wide Analysis and Identification of Stress or Development-Related R2R3MYBs. Front. Plant Sci. 9, 738. doi:10.3389/fpls.2018.00738
Hou, D., Zhao, Z. Y., Hu, Q. T., Li, L., Vasupalli, N., Zhuo, J., et al. (2020). PeSNAC-1 a NAC Transcription Factor from Moso Bamboo (Phyllostachys Edulis) Confers Tolerance to Salinity and Drought Stress in Transgenic rice. Tree Physiol. 40, 1792–1806. doi:10.1093/treephys/tpaa099
Hu, H. G., Dong, C., Sun, D. Q., Hu, Y., and Xie, J. H. (2018). Genome-wide Identification and Analysis of U-Box E3 Ubiquitin-Protein Ligase Gene Family in Banana. INT. J. MOL. SCI. 19, 3874. doi:10.3390/ijms19123874
Hwang, J. H., Seo, D. H., Kang, B. G., Kwak, J. M., and Kim, W. T. (2015). Suppression of Arabidopsis AtPUB30 Resulted in Increased Tolerance to Salt Stress during Germination. Plant Cel Rep 34, 277–289. doi:10.1007/s00299-014-1706-4
Jakob, W., Charlotte, O. S., and Karen, S. (2008). Biochemical Function of Typical and Variant Arabidopsis thaliana U-Box E3 Ubiquitin-Protein Ligases. Biochem. J. 413, 447–457. doi:10.1042/BJ20071568
Krzywinski, M., Schein, J., Birol, I., Connors, J., Gascoyne, R., Horsman, D., et al. (2009). Circos: An Information Aesthetic for Comparative Genomics. Genome Res. 19, 1639–1645. doi:10.1101/gr.092759.109
Kumar, P., Mishra, A., Sharma, H., Sharma, D., Rahim, M. S., Sharma, M., et al. (2018). Pivotal Role of bZIPs in Amylose Biosynthesis by Genome Survey and Transcriptome Analysis in Wheat (Triticum aestivum L.) Mutants. Scientific Rep. 8, 17240. doi:10.1038/s41598-018-35366-8
Lan, Y. G., Wu, L., Wu, M., Liu, H. L., Gao, Y. M., Zhang, K. M., et al. (2020). Transcriptome Analysis Reveals Key Genes Regulating Signaling and Metabolic Pathways during the Growth of Moso Bamboo (Phyllostachys Edulis) Shoots. Physiol. Plant. doi:10.1111/ppl.1329610.1111/ppl.13296
Lescot, M., Dehais, P., Thijs, G., Marchal, K., Moreau, Y., Van de Peer, Y., et al. (2002). PlantCARE, a Database of Plant Cis-Acting Regulatory Elements and a portal to Tools for In Silico Analysis of Promoter Sequences. Nucleic Acids Res. 30, 325–327. doi:10.1093/nar/30.1.325
Letunic, I., Khedkar, S., and Bork, P. (2021). SMART: Recent Updates, New Developments and Status in 2020. Nucleic Acids Res. 49, D458–D460. doi:10.1093/nar/gkaa937
Lim, C. W., Park, C., Kim, J.-H., Joo, H., Hong, E., and Lee, S. C. (2017). Pepper CaREL1, a Ubiquitin E3 Ligase, Regulates Drought Tolerance via the ABA-Signalling Pathway. Sci. Rep. 7, 1–12. doi:10.1038/s41598-017-00490-4
Liu, H., Gao, Y., Wu, M., Shi, Y., Wang, H., Wu, L., et al. (2020). TCP10, a TCP Transcription Factor in Moso Bamboo (Phyllostachys Edulis), Confers Drought Tolerance to Transgenic Plants. Environ. Exp. Bot. 172, 104002. doi:10.1016/j.envexpbot.2020.104002
Lu, X., Shu, N., Wang, D., Wang, J., and Ye, W. (2020). Genome-wide Identification and Expression Analysis of PUB Genes in Cotton. BMC Genomics 21, 213–224. doi:10.1186/s12864-020-6638-5
Metzger, M. B., Hristova, V. A., and Weissman, A. M. (2012). HECT and RING finger Families of E3 Ubiquitin Ligases at a Glance. J. Cel Sci 125, 531–537. doi:10.1242/jcs.091777
Mistry, J., Chuguransky, S., Williams, L., Qureshi, M., Salazar, G. A., Sonnhammer, E. L. L., et al. (2021). Pfam: The Protein Families Database in 2021. Nucleic Acids Res. 49, D412–D419. doi:10.1093/nar/gkaa913
Ning, W., Liu, Y., Cong, Y., Wang, T., Zhong, X., Yang, S., et al. (2016). Genome-Wide Identification of Soybean U-Box E3 Ubiquitin Ligases and Roles of GmPUB8 in Negative Regulation of Drought Stress Response in Arabidopsis. Plant Cel Physiol 57, 1189–1209. doi:10.1093/pcp/pcw068
Ouyang, S., Zhu, W., Hamilton, J., Lin, H., Campbell, M., Childs, K., et al. (2007). The TIGR Rice Genome Annotation Resource: Improvements and New Features. Nucleic Acids Res. 35, D883–D887. doi:10.1093/nar/gkl976
Pankaj, K., Afsana, P., Himanshu, S., Mohammed, S. R., Ankita, M., Prashant, K., et al. (2021). Understanding the Regulatory Relationship of Abscisic Acid and bZIP Transcription Factors towards Amylose Biosynthesis in Wheat. Mol. Biol. Rep. 48, 2473–2483. doi:10.1007/s11033-021-06282-4
Panu, A., Manohar, J., Konstantin, A., Delphine, B., Gabor, C., Edouard, D. C., et al. (2012). ExPASy: SIB Bioinformatics Resource portal. Nucleic Acids Res. 40, W597–W603. doi:10.1093/nar/gks400
Parveen, A., Rahim, M. S., Sharma, A., Mishra, A., and Roy, J. (2021). Genome-wide Analysis of RING-type E3 Ligase Family Identifies Potential Candidates Regulating High Amylose Starch Biosynthesis in Wheat (Triticum aestivum L). Scientific Rep. 11, 11461. doi:10.1038/s41598-021-90685-7
Peng, Z. H., Lu, Y., Li, L. B., Zhao, Q., Feng, Q., Gao, Z. M., et al. (2013). The Draft Genome of the Fast-Growing Non-timber forest Species Moso Bamboo (Phyllostachys Heterocycla). Nat. Genet. 45, 456–461. doi:10.1038/ng.2569
Peng, Z. H., Zhang, C. L., Zhang, Y., Hu, T., Mu, S. H., Li, X. P., et al. (2013). Transcriptome Sequencing and Analysis of the Fast Growing Shoots of Moso Bamboo (Phyllostachys Edulis). PLoS One 8, e78944. doi:10.1371/journal.pone.0078944
Priyann, A., Woodcroft, B. J., Rai, V., Moghul, I., Munagala, A., Ter, F., et al. (2019). Sequenceserver: A Modern Graphical User Interface for Custom BLAST Databases. Mol. Biol. Evol. 36, 2922–2924. doi:10.1093/molbev/msz185
Schulman, B. A., and Wade Harper, J. (2009). Ubiquitin-like Protein Activation by E1 Enzymes: the apex for Downstream Signalling Pathways. Nat. Rev. Mol. Cel Biol 10, 319–331. doi:10.1038/nrm2673
Shi, P., Preisler, H. K., Quinn, B. K., Zhao, J., Huang, W., Roell, A., et al. (2020). Precipitation Is the Most Crucial Factor Determining the Distribution of Moso Bamboo in Mainland China. GLOB. ECOL. CONSERV 22, e00924. doi:10.1016/j.gecco.2020.e00924
Singh, A., and Pandey, G. K. (2015). Primer Design Using Primer Express for SYBR Green-based Quantitative PCR. Methods Mol. Biol. 1275, 153–164. doi:10.1007/978-1-4939-2365-6_11
Stone, S. L., Williams, L. A., Farmer, L. M., Vierstra, R. D., and Callis, J. (2006). KEEP on GOING, a RING E3 Ligase Essential for Arabidopsis Growth and Development, Is Involved in Abscisic Acid Signaling. Plant Cell 18, 3415–3428. doi:10.1105/tpc.106.046532
Subramanian, B., Gao, S., Lercher, M. J., Hu, S., and Chen, W.-H. (2019). Evolview V3: a Webserver for Visualization, Annotation, and Management of Phylogenetic Trees. Nucleic Acids Res. 47, W270–W275. doi:10.1093/nar/gkz357
Trujillo, M., Ichimura, K., Casais, C., and Shirasu, K. (2008). Negative Regulation of PAMP-Triggered Immunity by an E3 Ubiquitin Ligase Triplet in Arabidopsis. Curr. Biol. 18, 1396–1401. doi:10.1016/j.cub.2008.07.085
Vierstra, R. D. (2009). The ubiquitin-26S Proteasome System at the Nexus of Plant Biology. Nat. Rev. Mol. Cel Biol 10, 385–397. doi:10.1038/nrm2688
Vorontsova, M. S., Clark, L. G., Dransfield, J., Govaerts, R., and Baker, W. J. (2016). World Checklist of Bamboos and Rattans: In Celebration of INBAR's 20th Anniversary. Beijing, 13–15. https://www.researchgate.net/publication/316620295.
Wang, C., Duan, W., Riquicho, A. R., Jing, Z. G., Liu, T. K., Hou, X. L., et al. (2015). Genome-wide Survey and Expression Analysis of the PUB Family in Chinese Cabbage (Brassica Rapa Ssp. Pekinesis). Mol. Genet. Genomics 290, 2241–2260. doi:10.1007/s00438-015-1075-x
Wang, H., Li, G.-B., Zhang, D.-Y., Lin, J., Sheng, B.-L., Han, J.-L., et al. (2013). Biological Functions of HD-Zip Transcription Factors. Hereditas 35, 1179–1188. doi:10.3724/SP.J.1005.2013.01179
Wang, K. L., Yang, Q. Y., Lanhuang, B., Lin, H. T., Shi, Y., Dhanasekaran, S., et al. (2020). Genome-wide Investigation and Analysis of U-Box Ubiquitin–Protein Ligase Gene Family in Apple: Expression Profiles during Penicillium expansum Infection Process. Physiol. Mol. Plant P 111, 101487. doi:10.1016/j.pmpp.2020.101487
Wang, T. T., Yang, Y., Lou, S. T., Wei, W., Zhao, Z. X., Lin, C. T., et al. (2019). Genome-wide Analysis of GATA Factors in Moso Bamboo (Phyllostachys Edulis) Unveils that PeGATAs Regulate Shoot Rapid-Growth and Rhizome Development. bioRxiv 21, 744003. doi:10.1101/74400310.1101/744003
Wang, X. Q., Yan, X. Y., Li, S. B., Jing, Y., Gu, L. F., Zou, S. Q., et al. (2021). Genome-wide Identification, Evolution and Expression Analysis of the Aspartic Protease Gene Family during Rapid Growth of Moso Bamboo (Phyllostachys Edulis) Shoots. BMC Genomics 22, 1–20. doi:10.1186/s12864-020-07290-7
Wang, Y., Tang, H., DeBarry, J. D., Tan, X., Li, J., Wang, X., et al. (2012). MCScanX: a Toolkit for Detection and Evolutionary Analysis of Gene Synteny and Collinearity. Nucleic Acids Res. 40, e49. doi:10.1093/nar/gkr1293
Yan, J., Wang, J., Li, Q., Hwang, J. R., Patterson, C., and Zhang, H. (2003). AtCHIP, a U-Box-Containing E3 Ubiquitin Ligase, Plays a Critical Role in Temperature Stress Tolerance in Arabidopsis. Plant Physiol. 132, 861–869. doi:10.1104/pp.103.020800
Yang, K., Li, Y., Wang, S., Xu, X., Sun, H., Zhao, H., et al. (2019). Genome-wide Identification and Expression Analysis of the MYB Transcription Factor in Moso Bamboo (Phyllostachys Edulis). PeerJ 6, e6242. doi:10.7717/peerj.6242
Ye, Y., and Rape, M. (2009). Building Ubiquitin Chains: E2 Enzymes at Work. Nat. Rev. Mol. Cel Biol 10, 755–764. doi:10.1038/nrm2780
Zeng, L. R., ParkChan, H., Venu, R. C., Gough, J., and Wang, G. L. (2008). Classification, Expression Pattern, and E3 Ligase Activity Assay of Rice U-Box-Containing Proteins. Mol. Plant Cel Physiol 1, 800–815. doi:10.1093/mp/ssn044
Zhang, X. R., Garreton, V., and Chua, N. H. (2005). The AIP2 E3 Ligase Acts as a Novel Negative Regulator of ABA Signaling by Promoting ABI3 Degradation. Genes Dev. 19, 1532–1543. doi:10.1101/gad.1318705
Zhang, Y., Yang, C., Li, Y., Zheng, N., Chen, H., Zhao, Q., et al. (2007). SDIR1 Is a RING finger E3 Ligase that Positively Regulates Stress-Responsive Abscisic Acid Signaling in Arabidopsis. Plant Cell 19, 1912–1929. doi:10.1105/tpc.106.048488
Zhao, H. S., Gao, Z. M., Wang, L., Wang, J. L., Wang, S. B., Fei, B. H., et al. (2018). Chromosome-level Reference Genome and Alternative Splicing Atlas of Moso Bamboo (Phyllostachys Edulis). Gigascience 7, giy115. doi:10.1093/gigascience/giy115
Keywords: U-box domain, PUB gene family, Phyllostachys edulis, evolution, collinearity, abiotic stresses
Citation: Zhou J, Hu Y, Li J, Yu Z and Guo Q (2021) Genome-Wide Identification and Expression Analysis of the Plant U-Box Protein Gene Family in Phyllostachys edulis. Front. Genet. 12:710113. doi: 10.3389/fgene.2021.710113
Received: 15 May 2021; Accepted: 15 November 2021;
Published: 30 November 2021.
Edited by:
Abdelfattah Badr, Helwan University, EgyptReviewed by:
Himanshu Sharma, National Agri-Food Biotechnology Institute, IndiaCheng Qin, Zunyi Vocational and Technical College, China
Qi Yang, Zhejiang Agriculture and Forestry University, China
Mohammad Arif, University of Hawaii at Manoa, United States
Copyright © 2021 Zhou, Hu, Li, Yu and Guo. This is an open-access article distributed under the terms of the Creative Commons Attribution License (CC BY). The use, distribution or reproduction in other forums is permitted, provided the original author(s) and the copyright owner(s) are credited and that the original publication in this journal is cited, in accordance with accepted academic practice. No use, distribution or reproduction is permitted which does not comply with these terms.
*Correspondence: Qirong Guo, cXJndW9AbmpmdS5lZHUuY24=