- 1Department of Medical Oncology, Rutgers Cancer Institute of New Jersey, New Brunswick, NJ, United States
- 2Department of Medicine, Rutgers Robert Wood Johnson Medical School, New Brunswick, NJ, United States
Immune checkpoint blockade leads to unprecedented responses in many cancer types. An alternative method of unleashing anti-tumor immune response is to target immunosuppressive metabolic pathways like the indoleamine 2,3-dioxygenase (IDO) pathway. Despite promising results in Phase I/II clinical trials, an IDO-1 inhibitor did not show clinical benefit in a Phase III clinical trial. Since, a treatment can be quite effective in a specific subset without being effective in the whole cancer type, it is important to identify the subsets of cancers that may benefit from IDO-1 inhibitors. In this study, we looked for the genomic and immunologic correlates of IDO pathway expression in cancer using the Cancer Genome Atlas (TCGA) dataset. Strong CD8+ T-cell infiltration, high mutation burden, and expression of exogenous viruses [Epstein-Barr virus (EBV), Human papilloma virus (HPV), and Hepatitis C virus (HCV)] or endogenous retrovirus (ERV3-2) were associated with over-expression of IDO-1 in most cancer types, IDO-2 in many cancer types, and TDO-2 in a few cancer types. High mutation burden in ER+ HER2− breast cancer, and ERV3-2 expression in ER− HER2− and HER2+ breast, colon, and endometrial cancers were associated with over-expression of all three genes. These results may have important implications for guiding development clinical trials of IDO-1 inhibitors.
Introduction
Unleashing anti-tumor immune response by targeting the immune checkpoint pathways (Pardoll, 2012; e.g., PD-1 blockade) leads to remarkable and durable responses in many cancer types (Kyi and Postow, 2016; Hargadon et al., 2018; Arora et al., 2019). An alternative method of unleashing anti-tumor immune response is to target the indoleamine 2,3-dioxygenase (IDO) pathway (Liu et al., 2010; Munn, 2012), a metabolic pathway that suppresses immune response (Frumento et al., 2002; Terness et al., 2002; Wang et al., 2014; Holmgaard et al., 2015). Despite pre-clinical evidence (Spranger et al., 2014; Triplett et al., 2018) and promising results in several Phase I/II clinical trials (Gibney et al., 2015; Gangadhar et al., 2017; Hamid et al., 2017; Daud et al., 2018; Mitchell et al., 2018; Zakharia et al., 2018), an IDO-1 inhibitor failed to show clinical benefit in a Phase III clinical trial (Long et al., 2019). A treatment can be quite effective in a specific subset without being effective in the whole cancer type, e.g., Herceptin is very effective in HER2+ breast cancer but not effective in breast cancer overall (Hudziak et al., 1989), so it is important to identify the subsets of cancers that may benefit from IDO-1 inhibitors. Since it is unknown whether high IDO-1 expression is a sufficient predictor of response to IDO-1 inhibitors or whether high expression of other genes in the IDO pathway (IDO-2 and TDO-2) is also necessary for response to IDO-1 inhibitors, in this study, we looked for the genomic and immunologic correlates of IDO pathway expression in cancer using the Cancer Genome Atlas (TCGA) dataset.
Materials and Methods
The mRNA expression data of tumors from TCGA was downloaded from the Broad Genome Data Analysis Center1 and the TCGA Data Portal.2 The data was median adjusted and log2 transformed as previously described (Panda et al., 2018b). The breast cancer samples were classified into clinical subtypes (ER+/HER2−, ER−/HER2−, and HER2+) using the focal copy number data of ERBB2 and the mRNA expression data of ESR1 obtained from the Broad Genome Data Analysis Center, and the three clinical subtypes were analyzed separately. Hyper-mutation status (Panda et al., 2017), Epstein-Barr virus (EBV) status (Cancer Genome Atlas Research Network, 2014), Hepatitis B and C virus (HBV, HCV) status (Cancer Genome Atlas Research Network, 2017), and ERV3-2 expression levels (Rooney et al., 2015) were obtained from recently published studies. Human papilloma virus (HPV) status was compiled from the auxiliary clinical files available in the TCGA Data Portal. Wilcoxon rank-sum test was used for all pairwise comparisons and Spearman Rho was used in all correlation analysis. All p-values are from two-sided tests, multiple hypothesis testing corrections were performed using the Benjamini-Hochberg method, and false discovery rate (FDR) < 0.05 was used as the threshold for statistical significance.
Results
Correlation Between the IDO Pathway Expression and CD8A Expression
Analysis of the TCGA dataset showed a wide range of IDO-1 expression among different cancer types, with the highest median expression in diffuse large B-cell lymphoma and the lowest median expression in acute myeloid leukemia (Figure 1A). IDO-2 (Figure 1B) and TDO-2 (Figure 1C) expression showed a similar pattern, with high expression in diffuse large B-cell lymphoma and low expression in acute myeloid leukemia.
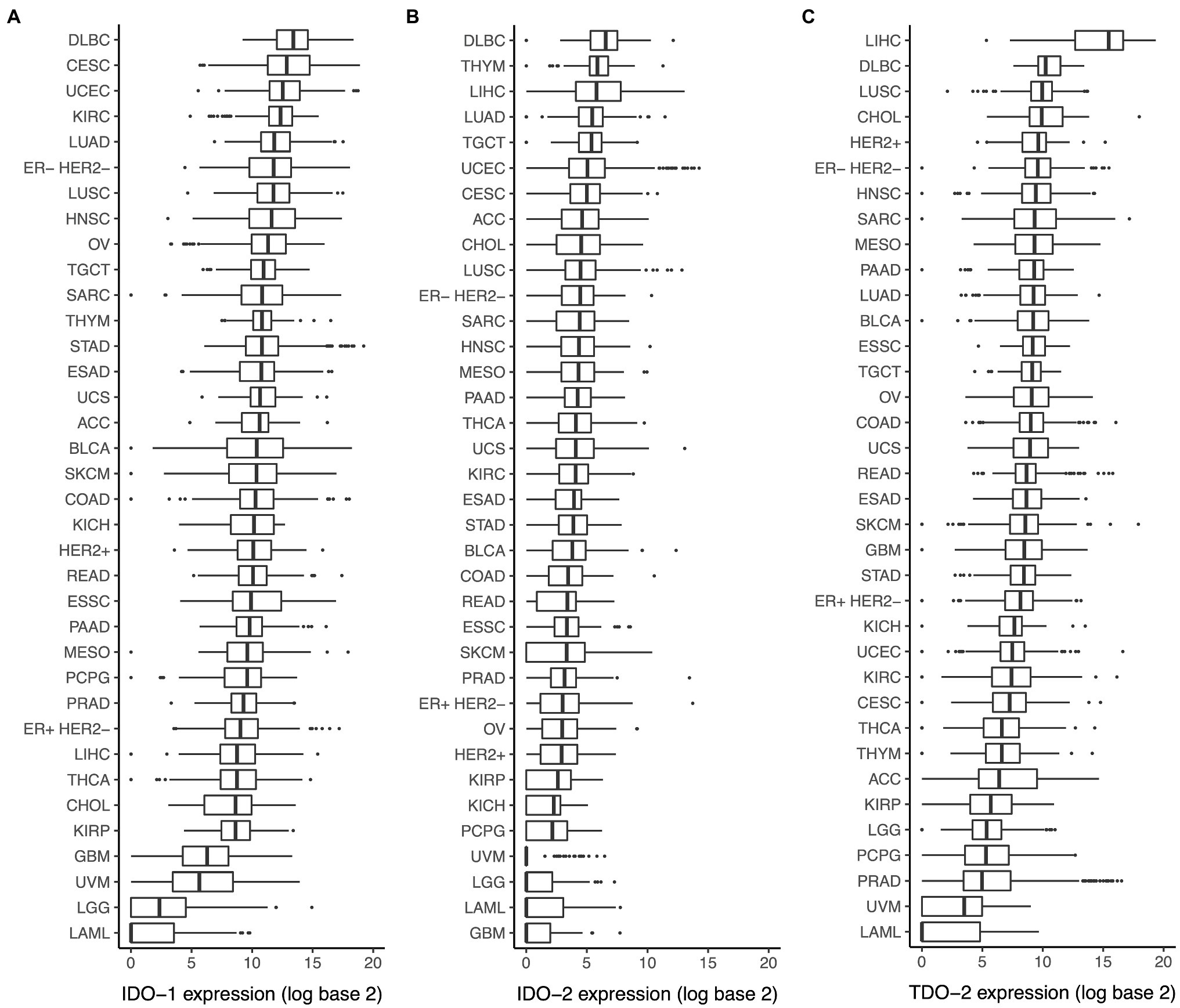
Figure 1. Expression of indoleamine 2,3-dioxygenase (IDO) pathway genes in various cancer types. Expression of (A) IDO-1, (B) IDO-2, and (C) TDO-2 in various cancer types. Cancer type acronyms are standard the Cancer Genome Atlas (TCGA) abbreviations (https://gdc.cancer.gov/resources-tcga-users/tcga-code-tables/tcga-study-abbreviations). ESAD, esophageal adenocarcinoma; ESSC, esophageal squamous cell carcinoma.
CD8+ T-cell infiltration in tumor is associated with local immune activation and response to immune checkpoint therapy (Tumeh et al., 2014), and PD-L1 expression is a marker of response to PD-1 blockade (Khunger et al., 2017). In this study, we found that CD8A expression, a known marker of CD8+ T-cell infiltration, had a much stronger correlation with IDO-1 expression than PD-L1 expression, a comparable correlation with IDO-2 expression and PD-L1 expression, and a much weaker correlation with TDO-2 expression than PD-L1 expression (Figure 2). This suggests that the presence of CD8+ T-cell infiltration in tumor is very strongly correlated with IDO-1 expression, strongly correlated with IDO-2 expression, but not so strongly correlated with TDO-2 expression. CD8A expression was correlated with all three genes in 22 cancer types, and with IDO-1 and IDO-2 (but not TDO-2) in nine more cancer types, so CD8+ T-cell infiltration is likely a widespread predictor of IDO pathway over-expression. These results are consistent with and substantially extend the finding of a recent study (Feng et al., 2020) that observed a strong correlation between IDO-1 expression and T-cell infiltration in breast and gynecologic cancers.
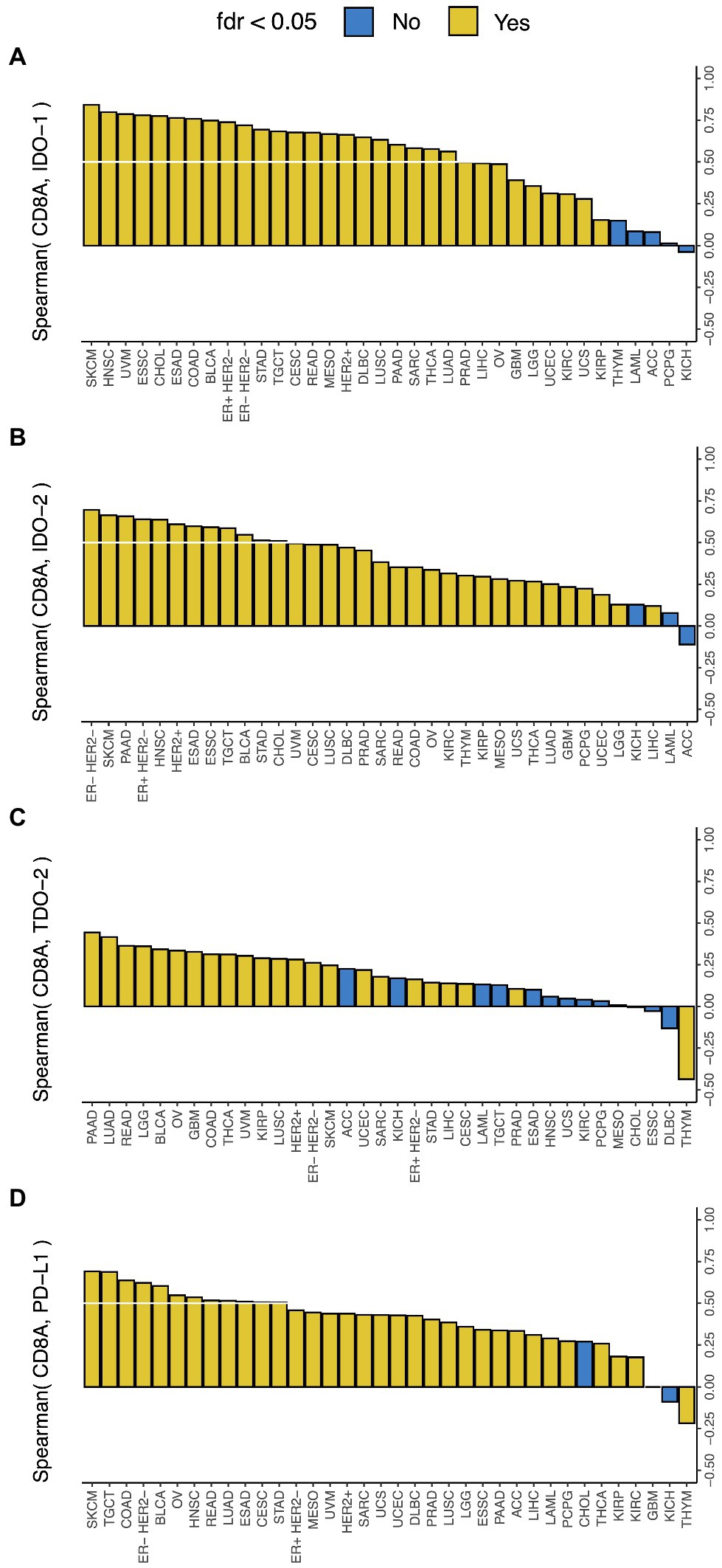
Figure 2. Correlation between IDO pathway expression and CD8A expression. Correlation between expressions of CD8A and (A) IDO-1, (B) IDO-2, (C) TDO-2, and (D) PD-L1 (the white lines mark Spearman Rho = 0.5). Cancer type acronyms are standard TCGA abbreviations (https://gdc.cancer.gov/resources-tcga-users/tcga-code-tables/tcga-study-abbreviations). FDR, false discovery rate. ESAD, esophageal adenocarcinoma; ESSC, esophageal squamous cell carcinoma.
Association Between the IDO Pathway Expression and Tumor Mutational Burden
We recently showed that in eight solid cancer types, the tumors above a non-synonymous mutation burden threshold (iCAM+) show RNA-seq based evidence of immune activation and immune checkpoint pathway upregulation in the TCGA dataset (Panda et al., 2017, 2020), and are more sensitive to immune checkpoint blockade in publicly available datasets of multiple cancer types (Panda et al., 2017) compared with the tumors below this threshold (iCAM−).
In this study, we found that compared to the tumors with low mutation burden (iCAM−), the tumors with high mutation burden (iCAM+) had significantly higher IDO-1 expression in most (6/8) cancer types (Figure 3A), but significantly higher IDO-2 expression in only a minority (3/8) of cancer types (Figure 3B), and significantly higher TDO-2 expression in very few (2/8) cancer types (Figure 3C). This suggests that high mutation burden is often associated with IDO-1 over-expression, but only rarely associated with IDO-2 over-expression, and very rarely associated with TDO-2 over-expression. High mutation burden was associated with over-expression of all three genes in ER+ HER2− breast cancer, and over-expression of IDO-1 and IDO-2 (but not TDO-2) in skin melanoma. These results are consistent with and substantially extend the finding of a recent study (Feng et al., 2020) that observed a significant correlation between tumor mutational burden and IDO-1 expression in breast and cervical cancer.
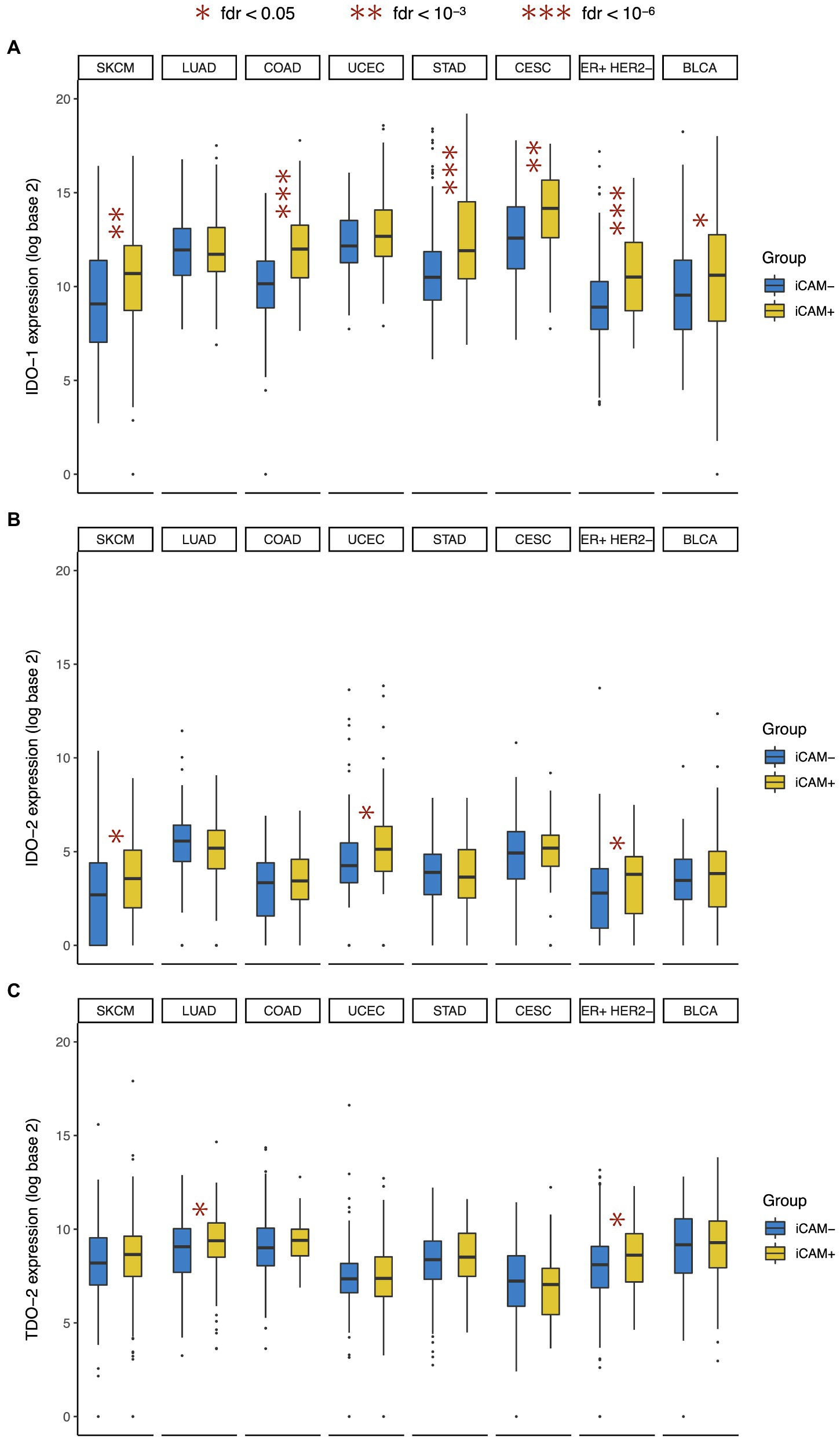
Figure 3. Association between IDO pathway expression and tumor mutational burden. Expression of (A) IDO-1, (B) IDO-2, and (C) TDO-2 in high mutation burden (iCAM+) and low mutation burden (iCAM−) tumors. Cancer type acronyms are standard TCGA abbreviations (https://gdc.cancer.gov/resources-tcga-users/tcga-code-tables/tcga-study-abbreviations). FDR, false discovery rate. *fdr < 0.05, **fdr < 10-3, and ***fdr < 10-6.
IDO Pathway Expression in Virally Mediated Tumors
Virally mediated tumors, like EBV+ gastric cancer (Grogg et al., 2003; Chiaravalli et al., 2006; Panda et al., 2018b) and HPV+ cervical cancer (Varn et al., 2018) and head and neck squamous cell cancer (Varn et al., 2018), are associated with immune activation and immune checkpoint pathway upregulation (Panda et al., 2020). EBV+ tumors also respond to PD-1 blockade in gastric cancer (Panda et al., 2018b), NK/T-cell lymphoma (Kwong et al., 2017), and Hodgkin’s lymphoma (Carbone et al., 2018).
In this study, we found that EBV+ gastric cancer, HPV+ cervical and head-neck squamous cell cancer, and HCV+ liver cancer (but not HBV+ liver cancer) showed over-expression of IDO-1 (Figure 4A), but only EBV+ gastric cancer and HPV+ head and neck squamous cell cancer showed over-expression of IDO-2 (Figure 4B). Virally mediated tumors did not show over-expression of TDO-2, in fact HPV+ head and neck squamous cell cancer showed under-expression of TDO-2 (Figure 4C). This suggests that exogenous viral infection in tumor (e.g., EBV, HPV, and HCV) is associated with the over-expression of IDO-1 and sometimes IDO-2 but not TDO-2. These results are consistent with and extend the findings of previous studies that reported IDO-1 over-expression in EBV+ nasopharyngeal cancer (Liu et al., 2014), HPV+ cervical (Kobayashi et al., 2008) and head-neck squamous cell cancer (Sailer et al., 2019), and chronic HCV infected liver (Larrea et al., 2007).
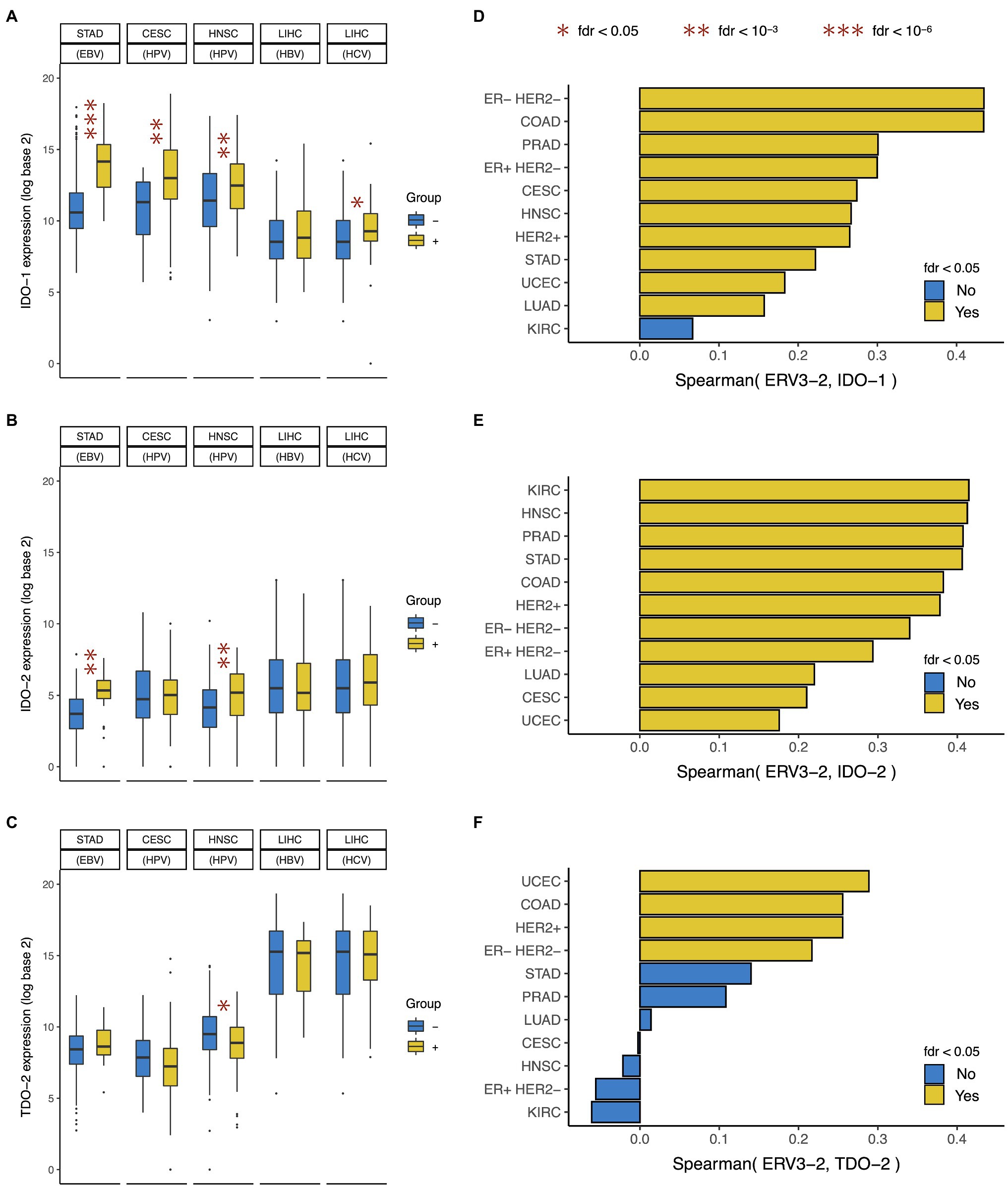
Figure 4. Association between IDO pathway expression and viral expression in tumor. Expression of (A) IDO-1, (B) IDO-2, and (C) TDO-2 in tumors positive and negative for Epstein-Barr virus (EBV), Human papilloma virus (HPV), Hepatitis B virus (HBV), and Hepatitis C virus (HCV). Correlation between expressions of ERV3-2 and (D) IDO-1, (E) IDO-2, and (F) TDO-2. Cancer type acronyms are standard TCGA abbreviations (https://gdc.cancer.gov/resources-tcga-users/tcga-code-tables/tcga-study-abbreviations). In liver cancer, the negative group only includes tumors negative for both HBV and HCV (i.e., HBV− HCV−) and does not include tumors that are HBV+ HCV− or HBV− HCV+. FDR, false discovery rate. *fdr < 0.05, **fdr < 10-3, and ***fdr < 10-6.
Correlation Between the IDO Pathway Expression and Expression of Potentially Immunogenic ERV
Expression of endogenous retrovirus (ERV) is a potential mechanism of immune activation (Hurst and Magiorkinis, 2015), and we recently showed that the expression of ERV3-2 in tumor is correlated with immune activation and immune checkpoint pathway upregulation in 11 solid cancer types (Panda et al., 2018a) and response to PD-1 blockade in clear-cell renal cancer (Panda et al., 2018a).
In this study, we found that ERV3-2 expression was correlated with IDO-1 expression in most (10/11) of these cancer types (Figure 4D), IDO-2 expression in all (11/11) of these cancer types (Figure 4E), and TDO-2 expression in only a few (4/11) of these cancer types (Figure 4F). Interestingly, ERV3-2 expression was often more strongly correlated with IDO-2 expression than IDO-1 expression. These results suggest that the expression of potentially immunogenic ERV is associated with the over-expression of both IDO-1 and IDO-2, but rarely TDO-2. ERV3-2 expression was correlated with all three genes in ER− HER2− and HER2+ breast, colon, and endometrial cancers; and IDO-1 and IDO-2 (but not TDO-2) in ER+ HER2− breast, gastric, lung, prostate, cervical, and head-neck squamous cell cancers.
Association Between the IDO Pathway Expression and Patient Survival
Survival analysis using UALCAN (Chandrashekar et al., 2017) shows that high expression of IDO-1 was often associated with worse prognosis (Supplementary Figure 1), such as in low-grade glioma, papillary renal cancer, pancreatic cancer, endometrial cancer, and uveal melanoma. However, skin melanoma was an exception to this, where high expression of IDO-1 was associated with better prognosis (Supplementary Figure 1). Like IDO-1, high expression of TDO-2 was also associated with worse prognosis (Supplementary Figure 2), such as in low-grade glioma, clear cell renal cancer, papillary renal cancer, acute myeloid leukemia, mesothelioma, and testicular germ cell tumor. Unlike IDO-1 and TDO-2, high expression of IDO-2 was often associated with better prognosis (Supplementary Figure 3). While high expression of IDO-2 was associated with worse prognosis in three cancer types, namely clear cell renal cancer, papillary renal cancer, and testicular germ cell tumor, it was associated with better prognosis in six cancer types, namely cervical cancer, head-neck squamous cell cancer, mesothelioma, pancreatic cancer, sarcoma, and skin melanoma (Supplementary Figure 3). These results suggest that high expression of IDO-1 (except in skin melanoma) and TDO-2 werse often associated with worse prognosis, while high expression of IDO-2 was often associated with better prognosis (with a few exceptions).
Discussion
CD8+ T-cell infiltration in tumor (Tumeh et al., 2014), high tumor mutational burden (Mehnert et al., 2016; Panda et al., 2017), exogenous viral infection in tumor (Kwong et al., 2017; Carbone et al., 2018; Panda et al., 2018b), and expression of endogenous retrovirus in tumor (Panda et al., 2018a) are associated with immune activation and checkpoint pathway upregulation in many cancer types, and response to immune checkpoint blockade in several cancer types.
In this study, we found that CD8A expression, a marker of CD8+ T-cell infiltration in tumor, was much more strongly correlated with IDO-1 expression than PD-L1 expression, a marker of response to PD-1 blockade (Khunger et al., 2017); and high tumor mutational burden, exogenous viral infection in tumor, and expression of endogenous retrovirus in tumor were associated with IDO-1 over-expression in most cancer types. These results are consistent with and substantially extend the finding of a recent study (Feng et al., 2020) that found IDO-1 expression to be correlated with tumor mutational burden in breast and cervical cancer, and T-cell infiltration in breast and gynecologic cancers; and previous studies that reported IDO-1 over-expression due to exogenous viral infection in EBV+ nasopharyngeal cancer (Liu et al., 2014), HPV+ cervical (Kobayashi et al., 2008) and head-neck squamous cell cancer (Sailer et al., 2019), and chronic HCV infected liver (Larrea et al., 2007).
CD8A expression showed a comparable level of correlation with IDO-2 expression and PD-L1 expression, and while high tumor mutational burden and exogenous viral infection in tumor were associated with IDO-2 over-expression in only a minority of cancer types, expression of endogenous retrovirus in tumor was correlated with IDO-2 expression even more strongly than IDO-1 expression.
CD8A expression showed much weaker correlation with TDO-2 expression than PD-L1 expression, and high tumor mutational burden and endogenous retroviral expression in tumor were rarely associated with TDO-2 expression, while exogenous viral infection in tumor was not at all associated with TDO-2 expression. In fact, HPV+ head and neck squamous cell cancer showed under-expression of TDO-2.
Overall, our results show that tumors with strong CD8+ T-cell infiltration, or high mutation burden, or exogenous viral infection, or endogenous retroviral expression have high IDO-1 expression in most cancer types, high IDO-2 expression in many cancer types, but high TDO-2 expression in only a few cancer types. These results may have important implications for guiding development clinical trials of IDO-1 inhibitors.
It is unknown whether high IDO-1 expression is a sufficient predictor of response to IDO-1 inhibitors or whether high expression of other genes in the IDO pathway (IDO-2 and TDO-2) is also necessary for response to IDO-1 inhibitors. CD8A expression is correlated with all three genes in 22 cancer types, and with IDO-1 and IDO-2 (but not TDO-2) in nine more cancer types, so CD8+ T-cell infiltration is likely a widespread predictor of IDO pathway over-expression. High mutational burden in ER+ HER2− breast cancer, and ERV3-2 expression in ER− HER2− and HER2+ breast, colon, and endometrial cancers are also associated with over-expression of all three IDO pathway genes, so these features may predict benefit from IDO-1 inhibitors. High mutational burden in skin melanoma, exogenous viral infection in gastric (EBV) and head-neck squamous cell (HPV) cancers, and ERV3-2 expression in ER+ HER2− breast, gastric, lung, prostate, cervical, and head-neck squamous cell cancers are associated with over-expression of IDO-1 and IDO-2 (but not TDO-2), but it is unclear whether that is sufficient for response to IDO-1 inhibitors.
Despite pre-clinical evidence (Spranger et al., 2014; Triplett et al., 2018) and promising results in several Phase I/II clinical trials (Gibney et al., 2015; Gangadhar et al., 2017; Hamid et al., 2017; Daud et al., 2018; Mitchell et al., 2018; Zakharia et al., 2018), an IDO-1 inhibitor failed to show clinical benefit in a Phase III clinical trial in melanoma (Long et al., 2019). While this Phase III clinical trial in melanoma was not restricted to tumors with high mutation burden and included tumors with low mutation burden, majority of melanoma cases tend to have high mutation burden (Panda et al., 2017). This might suggest that even the high mutation burden subset of melanoma, which shows over-expression of both IDO-1 and IDO-2 but not TDO-2, does not respond to IDO-1 inhibitors and over-expression of all three genes are necessary for response to IDO-1 inhibitors. Further research is necessary to test whether this is indeed the case.
Data Availability Statement
The original contributions presented in the study are included in the article/Supplementary Material; further inquiries can be directed to the corresponding author.
Author Contributions
AP: idea development, all analysis, all figures, manuscript writing, editing, and formatting. SG: idea development, interpretation of results, and editing. All authors contributed to the article and approved the submitted version.
Funding
SG is supported by grants from National Cancer Institute (R01 CA243547, P30 CA072720); U.S. Department of Defense (W81XWH1910821); Val Skinner Foundation (US); and Hugs for Brady.
Conflict of Interest
SG consulted for Novartis, Roche, Foundation Medicine, Foghorn Therapeutics, and Inspirata; Owns equity in and has licensed patents to Inspirata; Spouse is an employee of Merck and has equity in Merck.
The remaining author declares that the research was conducted in the absence of any commercial or financial relationships that could be construed as a potential conflict of interest.
The handling editor declared a past co-authorship with the authors AP and SG.
Supplementary Material
The Supplementary Material for this article can be found online at: https://www.frontiersin.org/articles/10.3389/fgene.2021.706435/full#supplementary-material
Supplementary figure 1 | Association between IDO-1 expression and patient survival.
Supplementary figure 2 | Association between TDO-2 expression and patient survival.
Supplementary figure 3 | Association between IDO-2 expression and patient survival.
Footnotes
References
Arora, S., Velichinskii, R., Lesh, R. W., Ali, U., Kubiak, M., Bansal, P., et al. (2019). Existing and emerging biomarkers for immune checkpoint immunotherapy in solid tumors. Adv. Ther. 36, 2638–2678. doi: 10.1007/s12325-019-01051-z
Cancer Genome Atlas Research Network (2014). Comprehensive molecular characterization of gastric adenocarcinoma. Nature 513, 202–209. doi: 10.1038/nature13480
Cancer Genome Atlas Research Network (2017). Comprehensive and integrative genomic characterization of hepatocellular carcinoma. Cell 169, 1327.e23–1341.e23. doi: 10.1016/j.cell.2017.05.046
Carbone, A., Gloghini, A., and Carlo-Stella, C. (2018). Are EBV-related and EBV-unrelated Hodgkin lymphomas different with regard to susceptibility to checkpoint blockade? Blood 132, 17–22. doi: 10.1182/blood-2018-02-833806
Chandrashekar, D. S., Bashel, B., Balasubramanya, S. A. H., Creighton, C. J., Ponce-Rodriguez, I., Chakravarthi, B., et al. (2017). UALCAN: a portal for facilitating tumor subgroup gene expression and survival analyses. Neoplasia 19, 649–658. doi: 10.1016/j.neo.2017.05.002
Chiaravalli, A. M., Feltri, M., Bertolini, V., Bagnoli, E., Furlan, D., Cerutti, R., et al. (2006). Intratumour T cells, their activation status and survival in gastric carcinomas characterised for microsatellite instability and Epstein-Barr virus infection. Virchows Arch. 448, 344–353. doi: 10.1007/s00428-005-0066-4
Daud, A., Saleh, M. N., Hu, J., Bleeker, J. S., Riese, M. J., Meier, R., et al. (2018). Epacadostat plus nivolumab for advanced melanoma: updated phase 2 results of the ECHO-204 study. J. Clin. Oncol. 36:9511. doi: 10.1200/JCO.2018.36.15_suppl.9511
Feng, X., Tang, R., Zhang, R., Wang, H., Ji, Z., Shao, Y., et al. (2020). A comprehensive analysis of IDO1 expression with tumour-infiltrating immune cells and mutation burden in gynaecologic and breast cancers. J. Cell. Mol. Med. 24, 5238–5248. doi: 10.1111/jcmm.15176
Frumento, G., Rotondo, R., Tonetti, M., Damonte, G., Benatti, U., and Ferrara, G. B. (2002). Tryptophan-derived catabolites are responsible for inhibition of T and natural killer cell proliferation induced by indoleamine 2,3-dioxygenase. J. Exp. Med. 196, 459–468. doi: 10.1084/jem.20020121
Gangadhar, T. C., Schneider, B. J., Bauer, T. M., Wasser, J. S., Spira, A. I., Patel, S. P., et al. (2017). Efficacy and safety of epacadostat plus pembrolizumab treatment of NSCLC: preliminary phase I/II results of ECHO-202/KEYNOTE-037. J. Clin. Oncol. 35:9014. doi: 10.1200/JCO.2017.35.15_suppl.9014
Gibney, G., Hamid, O., Lutzky, J., Olszanski, A., Gangadhar, T., Gajewski, T., et al. (2015). 511 updated results from a phase 1/2 study of epacadostat (INCB024360) in combination with ipilimumab in patients with metastatic melanoma. Eur. J. Cancer 51, S106–S107. doi: 10.1016/S0959-8049(16)30312-4
Grogg, K. L., Lohse, C. M., Pankratz, V. S., Halling, K. C., and Smyrk, T. C. (2003). Lymphocyte-rich gastric cancer: associations with Epstein-Barr virus, microsatellite instability, histology, and survival. Mod. Pathol. 16, 641–651. doi: 10.1097/01.MP.0000076980.73826.C0
Hamid, O., Gajewski, T. F., Frankel, A. E., Bauer, T. M., Olszanski, A. J., Luke, J. J., et al. (2017). 3812-Epacadostat plus pembrolizumab in patients with advanced melanoma: phase 1 and 2 efficacy and safety results from ECHO-202/KEYNOTE-037. Ann. Oncol. 28, v428–v448. doi: 10.1093/annonc/mdx377
Hargadon, K. M., Johnson, C. E., and Williams, C. J. (2018). Immune checkpoint blockade therapy for cancer: an overview of FDA-approved immune checkpoint inhibitors. Int. Immunopharmacol. 62, 29–39. doi: 10.1016/j.intimp.2018.06.001
Holmgaard, R. B., Zamarin, D., Li, Y., Gasmi, B., Munn, D. H., Allison, J. P., et al. (2015). Tumor-expressed IDO recruits and activates MDSCs in a treg-dependent manner. Cell Rep. 13, 412–424. doi: 10.1016/j.celrep.2015.08.077
Hudziak, R. M., Lewis, G. D., Winget, M., Fendly, B. M., Shepard, H. M., and Ullrich, A. (1989). p185HER2 monoclonal antibody has antiproliferative effects in vitro and sensitizes human breast tumor cells to tumor necrosis factor. Mol. Cell. Biol. 9, 1165–1172. doi: 10.1128/mcb.9.3.1165-1172.1989
Hurst, T. P., and Magiorkinis, G. (2015). Activation of the innate immune response by endogenous retroviruses. J. Gen. Virol. 96, 1207–1218. doi: 10.1099/vir.0.000017
Khunger, M., Hernandez, A. V., Pasupuleti, V., Rakshit, S., Pennell, N. A., Stevenson, J., et al. (2017). Programmed cell death 1 (PD-1) ligand (PD-L1) expression in solid tumors as a predictive biomarker of benefit from PD-1/PD-L1 axis inhibitors: a systematic review and meta-analysis. JCO Precis. Oncol. 1, 1–15. doi: 10.1200/PO.16.00030
Kobayashi, A., Weinberg, V., Darragh, T., and Smith-McCune, K. (2008). Evolving immunosuppressive microenvironment during human cervical carcinogenesis. Mucosal Immunol. 1, 412–420. doi: 10.1038/mi.2008.33
Kwong, Y. L., Chan, T. S. Y., Tan, D., Kim, S. J., Poon, L. M., Mow, B., et al. (2017). PD1 blockade with pembrolizumab is highly effective in relapsed or refractory NK/T-cell lymphoma failing l-asparaginase. Blood 129, 2437–2442. doi: 10.1182/blood-2016-12-756841
Kyi, C., and Postow, M. A. (2016). Immune checkpoint inhibitor combinations in solid tumors: opportunities and challenges. Immunotherapy 8, 821–837. doi: 10.2217/imt-2016-0002
Larrea, E., Riezu-Boj, J. I., Gil-Guerrero, L., Casares, N., Aldabe, R., Sarobe, P., et al. (2007). Upregulation of indoleamine 2,3-dioxygenase in hepatitis C virus infection. J. Virol. 81, 3662–3666. doi: 10.1128/JVI.02248-06
Liu, W. L., Lin, Y. H., Xiao, H., Xing, S., Chen, H., Chi, P. D., et al. (2014). Epstein-Barr virus infection induces indoleamine 2,3-dioxygenase expression in human monocyte-derived macrophages through p38/mitogen-activated protein kinase and NF-kappaB pathways: impairment in T cell functions. J. Virol. 88, 6660–6671. doi: 10.1128/JVI.03678-13
Liu, X., Shin, N., Koblish, H. K., Yang, G., Wang, Q., Wang, K., et al. (2010). Selective inhibition of IDO1 effectively regulates mediators of antitumor immunity. Blood 115, 3520–3530. doi: 10.1182/blood-2009-09-246124
Long, G. V., Dummer, R., Hamid, O., Gajewski, T. F., Caglevic, C., Dalle, S., et al. (2019). Epacadostat plus pembrolizumab versus placebo plus pembrolizumab in patients with unresectable or metastatic melanoma (ECHO-301/KEYNOTE-252): a phase 3, randomised, double-blind study. Lancet Oncol. 20, 1083–1097. doi: 10.1016/S1470-2045(19)30274-8
Mehnert, J. M., Panda, A., Zhong, H., Hirshfield, K., Damare, S., Lane, K., et al. (2016). Immune activation and response to pembrolizumab in POLE-mutant endometrial cancer. J. Clin. Invest. 126, 2334–2340. doi: 10.1172/JCI84940
Mitchell, T. C., Hamid, O., Smith, D. C., Bauer, T. M., Wasser, J. S., Olszanski, A. J., et al. (2018). Epacadostat plus pembrolizumab in patients with advanced solid tumors: phase I results from a multicenter, open-label phase I/II trial (ECHO-202/KEYNOTE-037). J. Clin. Oncol. 36, 3223–3230. doi: 10.1200/JCO.2018.78.9602
Munn, D. H. (2012). Blocking IDO activity to enhance anti-tumor immunity. Front. Biosci. 4, 734–745. doi: 10.2741/414
Panda, A., Betigeri, A., Subramanian, K., Ross, J. S., Pavlick, D. C., Ali, S., et al. (2017). Identifying a clinically applicable mutational burden threshold as a potential biomarker of response to immune checkpoint therapy in solid tumors. JCO Precis. Oncol. 2017:17.00146. doi: 10.1200/PO.17.00146
Panda, A., de Cubas, A. A., Stein, M., Riedlinger, G., Kra, J., Mayer, T., et al. (2018a). Endogenous retrovirus expression is associated with response to immune checkpoint blockade in clear cell renal cell carcinoma. JCI Insight 3:e121522. doi: 10.1172/jci.insight.121522
Panda, A., Mehnert, J. M., Hirshfield, K. M., Riedlinger, G., Damare, S., Saunders, T., et al. (2018b). Immune activation and benefit from avelumab in EBV-positive gastric cancer. J. Natl. Cancer Inst. 110, 316–320. doi: 10.1093/jnci/djx213
Panda, A., Rosenfeld, J. A., Singer, E. A., Bhanot, G., and Ganesan, S. (2020). Genomic and immunologic correlates of LAG-3 expression in cancer. Oncoimmunology 9:1756116. doi: 10.1080/2162402X.2020.1756116
Pardoll, D. M. (2012). The blockade of immune checkpoints in cancer immunotherapy. Nat. Rev. Cancer 12, 252–264. doi: 10.1038/nrc3239
Rooney, M. S., Shukla, S. A., Wu, C. J., Getz, G., and Hacohen, N. (2015). Molecular and genetic properties of tumors associated with local immune cytolytic activity. Cell 160, 48–61. doi: 10.1016/j.cell.2014.12.033
Sailer, V., Sailer, U., Bawden, E. G., Zarbl, R., Wiek, C., Vogt, T. J., et al. (2019). DNA methylation of indoleamine 2,3-dioxygenase 1 (IDO1) in head and neck squamous cell carcinomas correlates with IDO1 expression, HPV status, patientsʼ survival, immune cell infiltrates, mutational load, and interferon gamma signature. EBioMedicine 48, 341–352. doi: 10.1016/j.ebiom.2019.09.038
Spranger, S., Koblish, H. K., Horton, B., Scherle, P. A., Newton, R., and Gajewski, T. F. (2014). Mechanism of tumor rejection with doublets of CTLA-4, PD-1/PD-L1, or IDO blockade involves restored IL-2 production and proliferation of CD8(+) T cells directly within the tumor microenvironment. J. Immunother. Cancer 2:3. doi: 10.1186/2051-1426-2-3
Terness, P., Bauer, T. M., Rose, L., Dufter, C., Watzlik, A., Simon, H., et al. (2002). Inhibition of allogeneic T cell proliferation by indoleamine 2,3-dioxygenase-expressing dendritic cells: mediation of suppression by tryptophan metabolites. J. Exp. Med. 196, 447–457. doi: 10.1084/jem.20020052
Triplett, T. A., Garrison, K. C., Marshall, N., Donkor, M., Blazeck, J., Lamb, C., et al. (2018). Reversal of indoleamine 2,3-dioxygenase-mediated cancer immune suppression by systemic kynurenine depletion with a therapeutic enzyme. Nat. Biotechnol. 36, 758–764. doi: 10.1038/nbt.4180
Tumeh, P. C., Harview, C. L., Yearley, J. H., Shintaku, I. P., Taylor, E. J., Robert, L., et al. (2014). PD-1 blockade induces responses by inhibiting adaptive immune resistance. Nature 515, 568–571. doi: 10.1038/nature13954
Varn, F. S., Schaafsma, E., Wang, Y., and Cheng, C. (2018). Genomic characterization of six virus-associated cancers identifies changes in the tumor immune microenvironment and altered genetic programs. Cancer Res. 78, 6413–6423. doi: 10.1158/0008-5472.CAN-18-1342
Wang, X. F., Wang, H. S., Wang, H., Zhang, F., Wang, K. F., Guo, Q., et al. (2014). The role of indoleamine 2,3-dioxygenase (IDO) in immune tolerance: focus on macrophage polarization of THP-1 cells. Cell. Immunol. 289, 42–48. doi: 10.1016/j.cellimm.2014.02.005
Keywords: IDO1 inhibitor, IDO2, TDO2, immune checkpoint inhibitors, CD8+ T-cell, tumor mutation burden, virally induced cancer, endogenous retrovirus
Citation: Panda A and Ganesan S (2021) Genomic and Immunologic Correlates of Indoleamine 2,3-Dioxygenase Pathway Expression in Cancer. Front. Genet. 12:706435. doi: 10.3389/fgene.2021.706435
Edited by:
Anupama Yadav, Dana–Farber Cancer Institute, United StatesReviewed by:
Jozsef Dudas, Innsbruck Medical University, AustriaNicholas Ian Fleming, University of Otago, New Zealand
Copyright © 2021 Panda and Ganesan. This is an open-access article distributed under the terms of the Creative Commons Attribution License (CC BY). The use, distribution or reproduction in other forums is permitted, provided the original author(s) and the copyright owner(s) are credited and that the original publication in this journal is cited, in accordance with accepted academic practice. No use, distribution or reproduction is permitted which does not comply with these terms.
*Correspondence: Anshuman Panda, ap1022@scarletmail.rutgers.edu