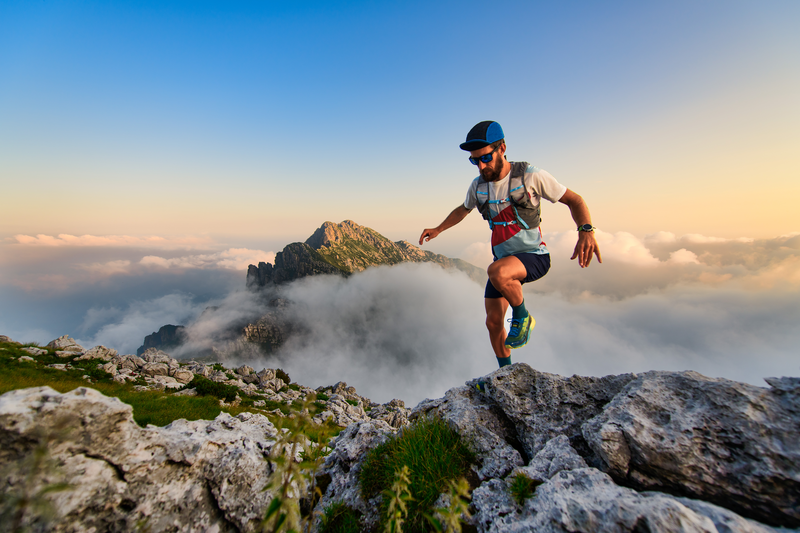
94% of researchers rate our articles as excellent or good
Learn more about the work of our research integrity team to safeguard the quality of each article we publish.
Find out more
CLINICAL TRIAL article
Front. Genet. , 08 October 2021
Sec. Genetics of Common and Rare Diseases
Volume 12 - 2021 | https://doi.org/10.3389/fgene.2021.701750
Background: The spread of drug resistance has seriously impacted the effective treatment of infection with the malaria parasite, Plasmodium falciparum. Continuous monitoring of molecular marker polymorphisms associated with drug resistance in parasites is essential for malaria control and elimination efforts. Our study describes mutations observed in the resistance genes Pfkelch13, Pfcrt, and Pfmdr1 in imported malaria and identifies additional potential drug resistance-associated molecular markers.
Methods: Chinese patients infected in Africa with P. falciparum were treated with intravenous (IV) injections of artesunate 240–360 mg for 3–5 days while hospitalized and treated with oral dihydroartemisinin-piperaquine (DHP) for 3 days after hospital discharge. Blood samples were collected and PCR sequencing performed on genes Pfkelch13, Pfcrt, and Pfmdr1 from all isolates.
Results: We analyzed a total of 225 patients from Guangxi, China with P. falciparum malaria acquired in Africa between 2016 and 2018. All patients were cured completely after treatment. The F446I mutation of the Pfkelch13 gene was detected for the first time from samples of West African P. falciparum, with a frequency of 1.0%. Five haplotypes of Pfcrt that encode residues 72–76 were found, with the wild-type CVMNK sequence predominating (80.8% of samples), suggesting that the parasites might be chloroquine sensitive. For Pfmdr1, N86Y (13.1%) and Y184F (58.8%) were the most prevalent, suggesting that artemether-lumefantrine may not, in general, be a suitable treatment for the group.
Conclusions: For the first time, this study detected the F446I mutation of the Pfkelch13 gene from Africa parasites that lacked clinical evidence of resistance. This study provides the latest data for molecular marker surveillance related to antimalarial drug resistance genes Pfkelch13, Pfcrt, and Pfmdr1 imported from Africa, in Guangxi, China from Chinese migrate workers.
Clinical Trial Registration: ChiCTROPC17013106.
Malaria is a serious public health problem in tropical and subtropical areas of the world. There were ~229 million cases and 409,000 malaria-related deaths worldwide in 2019, with Africa shouldering over 94% of the global malaria burden (WHO, 2020). While five species of Plasmodium can cause human disease, Plasmodium falciparum is most notorious due to high levels of mortality and morbidity associated with infection. The recent decline in malaria prevalence is mainly due to the application of efficacious antimalarial drugs, early diagnosis by RDT, and distribution of long-lasting insecticide-treated nets (LLTINs) in malaria- endemic areas. However, the emergence of drug resistance remains a persistent obstacle to control and eliminate malaria. Here we sought to better understand the geography of resistance alleles in hopes of guiding clinical decisions and support malaria surveillance and elimination programs.
Imported malaria is increasing in China owing to increasing global economic integration. Disease among migrant laborers attributable to P. falciparum has increased rapidly in recent years (Feng et al., 2014; Liu et al., 2014). From 2011 to 2016, 19,154 imported cases were reported in mainland China, most of which came from Africa (72%) (Lai et al., 2019). In the past, China suffered seriously from endemic malaria, but the occurrence of malaria has been reduced; 2017 marked the first time that no locally acquired cases were reported (Feng et al., 2018). The World Health Organization (WHO) deemed China to have achieved malaria elimination in 2021. Thus, imported disease has become the main challenge remaining to eliminate malaria from China. It is particularly important to continue to monitor malaria imported into China through molecular monitoring. Chinese migrant workers lack prior immunity; thus, most self-administer antimalarial drugs when working in endemic regions, often without medical guidance; such practices may induce drug resistance. Understanding the emergence of drug resistance therefore requires attention to the behaviors and consequences of such migrant workers (Yang et al., 2017; Wang et al., 2018, 2020; Yao et al., 2018; Li et al., 2019; She et al., 2020; Zhao et al., 2020; Shi et al., 2021).
Antimalarial drugs are principal tools for malaria control. In Africa in the 1990s, chloroquine (CQ) was used to treat malaria and saved tens of millions of lives (Trape, 2001). Chloroquine was once highly efficacious against uncomplicated P. falciparum infection. Widespread use engendered resistance (CQR) (Young et al., 1963; Fogh et al., 1979; Wellems and Plowe, 2001); the WHO then recommended artemisinin-based combination therapies (ACTs) as the first-line antimalarial treatment of choice. According to a WHO report, Côte d'Ivoire and Gabon used artesunate-amodiaquine as a first-line treatment for P. falciparum in 2003 and artesunate-amodiaquine as a first-line treatment for P. falciparum in Cameroon and Liberia in 2004. Mozambique in 2004 used artemether-lumefantrine as a first-line treatment for P. falciparum. In Sierra Leone and Ghana, artemether-lumefantrine and artesunate-amodiaquine were used as first-line treatments for P. falciparum in 2004, and artemether-lumefantrine and artesunate-amodiaquine were used as first-line treatments for P. falciparum in Mali in 2007 (WHO, 2015). Artemether-lumefantrine is used widely in African countries.
Artemisinin resistance (ART-R) has emerged in Africa (Lu et al., 2017a), and its appearance is a major threat to malaria control efforts including those efforts aimed at treating imported malaria. Mutations in the Kelch propeller gene (Pfkelch13) have, elsewhere, been linked to reduced efficacy of artemisinin. Particular mutations in the Pfkelch13 gene discovered to play an important role in ART-R include Y439H, F446I, M476I, R539T, I543T, and C580Y increasing ring-stage survival (RSA) (Straimer et al., 2015; Mita et al., 2016; Zhang et al., 2017; Wang et al., 2018). Mutations that have been detected in Africa samples include T149S, K189T/N, P441S, S459T, D464E, C469F, T474I, A481T, K503E, R539T, R561H, P574L, A578S, C580Y, V589I, V603E, E612K, Q613E, R622I, V650F, G665S, A675V, V692L, and N694K, but not F446I (Conrad et al., 2014a; Torrentino-Madamet et al., 2014; Tacoli et al., 2016; Ikeda et al., 2018; Wang et al., 2018, 2020; Yao et al., 2018; Li et al., 2019; Ocan et al., 2019; She et al., 2020).
Alleles of the P. falciparum chloroquine resistance transporter gene (Pfcrt) have been identified as molecular markers of CQR (Warhurst, 2001; Ariey et al., 2014). These include mutations in amino acids 72–76 (Awasthi and Das, 2013) with K76T being the most common change found in CQR parasites. The P. falciparum multidrug resistance protein-1 (Pfmdr1) has also been associated with resistance to multiple antimalarial drugs. Mutations at residues 86, 184, 1034, 1042, and 1246 have been shown to be associated with resistance to CQ, amodiaquine (AQ), quinine (QN), mefloquine (MQ), halofantrine (HF), and artemisinin (ART) (Duraisingh et al., 1997; Sidhu et al., 2005; Humphreys et al., 2007; Lekostaj et al., 2008; Tinto et al., 2008; Folarin et al., 2011).
In Africa, artemisinin resistance has emerged, and its appearance threatens current efforts to control malaria (Lu et al., 2017b). Antimalarial drug use among Chinese laborers may contribute to drug pressures. Therefore, monitoring the molecular markers of drug resistance in imported malaria cases complements studies in endemic African populations, enriching the picture of polymorphisms that may contribute to drug resistance. Here, we describe the distribution of Pfkelch13, Pfcrt, and Pfmdr1 from P. falciparum isolates imported from Africa to Guangxi, South China from 2016 to 2018.
All samples were collected between 2016 and 2018 from Chinese migrant workers that had returned from Africa and who were diagnosed at the Guangxi Shanglin Hospital with uncomplicated falciparum malaria. The diagnosis was based on the microscopy of Giemsa-stained blood smears. Venous blood samples (2–5 ml) were collected before treatment. Prospective cases with complex travel histories were excluded from the study (Zhao et al., 2020). All uncomplicated patients were treated with intravenous (IV) injections of artesunate, on day 0 with 120 mg, and days 1, 2, 3, and 4 with 60 mg on each subsequent day during hospitalization. Upon resolution of symptoms, the patients were discharged, and 3 days of oral treatment with dihydroartemisinin and piperaquine phosphate tablets (dihydroartemisinin-piperaquine, DHP, dihydroartemisinin 40 mg, and piperaquine phosphate 320 mg for each tablet) was prescribed, on day 1, day 2, and day 3 with 4 pills, 2 pills, and 2 pills, respectively.
This study was approved by the Ethics Review Committees of the Institutional Review Board (IRB) of Shanglin Hospital. All participants supplied written informed consent. This study was registered on the Chinese Clinical Trial Registry with Registration Number ChiCTROPC17013106.
Parasite genomic DNA was isolated from 2 to 5 ml of venous blood using the High Pure PCR Template Preparation Kit (Roche Biotech Co., Ltd., Germany). The template DNA was amplified by Nest-PCR-sequence of the Pfkelch13 gene (Gene ID: PF3D7_1343700; Wang et al., 2015), a 145-bp fragment of the Pfcrt gene (Gene ID: PF3D7_0709000; Zhou et al., 2016), and two fragments (N1: 526 bp and N2: 799 bp) of the Pfmdr1 gene (Gene ID: PF3D7_0523000; Li et al., 2015), including the entire sequence of the Pfkelch13 gene, Pfcrt mutations at codons 72–76, and Pfmdr1 mutations at codons 86, 130, 184, 1034, 1042, 1109, and 1246. PCR products were purified, sequenced by the Sangon Biotech Co Ltd (Kunming, China), and analyzed using DNAstar (DNASTAR Inc., Madison, WI, USA).
Experimental data were analyzed by SPSS 19.0 (SPSS Inc. Chicago, IL, USA). The variation tendency for haplotypes and the frequency mutations of Pfkelch13, Pfcrt, and Pfmdr1 over the study period was evaluated by chi-square test; the trend among the groups from patients from different regions was assessed by chi-square test with p < 0.05 being considered significant.
A total of 225 uncomplicated P. falciparum cases that returned from Africa to Guangxi Province between 2016 and 2018 were analyzed in this study. None of the patients had traveled to any other areas prior to, or after, spending time in Africa (Zhao et al., 2020). Among the infected individuals, most (77.8%, 175/225) had spent time in West Africa, with 20.4% (46/225) and 1.8% (4/225) having been in Central and South Africa, respectively (Supplementary Table 1). The majority of the patients who returned from West Africa had been in either Kumasi or Asankragua in Ghana. The patients' clinical information is included in Supplementary Table 2. Fever was resolved in 80% of the patients within 2 days of treatment. Patients were discharged on days 3, 4, and 5 (15, 70, and 15%, respectively). Six months after discharge, the patients reported no additional fever.
The complete Pfkelch13 gene was successfully amplified and sequenced from 92.4% (208/225) of the samples. The sequences were deposited in GenBank (MK877250- MK877457). The distribution of Pfkelch13 mutations is shown in Figure 1 and Tables 1, 2.
Figure 1. Prevalence of PfK13 mutations from studied isolates. *The difference is statistically significant between 2016 and 2018.
The wild-type allele accounted for 25.5% of the samples and was found in higher frequency in samples from patients who had been in areas other than West Africa (p < 0.01). A total of 19 single nucleotide polymorphisms (SNPs), including 9 synonymous and 10 non-synonymous changes, were observed in the gene, of which 6 non-synonymous (T149S, K189T/N, R255K, F446I, G533S, and V589I) had been reported previously (Wang et al., 2015; Boussaroque et al., 2016; Yao et al., 2018) while 4 (G112E, L258M, A569V, and G665S) were new (Table 1). One, two, or three asparagine insertions at codon 142 were found in multiple samples (13.00%, 27/208). The non-synonymous mutations at K189T/N or T149S were most prevalent, being found in 50.9, 5.3, and 1.9% of the samples, respectively (Figure 1). In this study, the mutation F446I was observed in two of the isolates from Ghana (Figure 1 and Table 2, GenBank accession numbers MK877371 and MK877381).
From the 225 samples, the Pfcrt and Pfmdr1 genes were successfully sequenced from 193 (85.8%) to 204 (90.7%), respectively. The sequences were deposited in GenBank (MZ572562-MZ572754, MZ572358-MZ572561, MZ577609-MZ577812). The mutations found in Pfcrt and Pfmdr1 are indicated in Tables 3–5. For Pfcrt, there were no polymorphisms at position 72 or 73. Mutations leading to changes at positions M74I and N75E were found in the same abundance, making up 15.5% (30/193) of the total samples analyzed, while mutations impacting residue 76 were most frequent (17.6%; Table 3). Five haplotypes within Pfcrt residues 72–76 were identified, including CVMNK (wild type), CVIET, CVIEK, CVMNT (mutant types), and CVM/I N/E K/T (mixed types) with prevalence of 80.8% (156/193), 13.5% (26/193), 1.6% (3/193), 3.6% (7/193), and 0.5% (1/193), respectively (Table 4). For Pfmdr1, the prevalence of polymorphisms impacting positions 184 and 86 totaled 58.8 and 13.1%, respectively. Other, less frequent mutations included D89G (0.5%), F1226Y (3.9%), and D1246Y (2.4%). No mutations at position 1034 or 1042 were detected (Table 3). Eleven haplotypes with differences at residues 86, 184, and 1246 were found, including wild type (NYD), mutation haplotypes (YYD, NFD, YFD, and YYY), and mixed haplotypes (N/Y YD, N/Y FD, N Y/F D, Y Y/F D, N/Y Y/F D, and Y Y/F D/Y), accounting for 34.3, 4.4, 41.7, 2.9, 2.0, 0.5, 0.5, 10.7, 0.5, 2.0, and 0.5%, respectively (Table 4). Among the haplotypes from samples from migrants from West and Non-West Africa, the wild type (NYD) was found with similar frequency (p > 0.05). The NFD and YFD haplotypes show a significant difference among the groups (p < 0.05). The haplotype frequency of Pfmdr1 YYD was markedly reduced during the time period of the study, accounting for 8.3% of the diversity in 2016 but undetected in 2018 (p < 0.05; Table 5). The distribution of all Pfmdr1 haplotypes is shown in Table 5.
In our study, several antimalarial molecular markers (Pfkelch13, Pfcrt, Pfmdr1) were used to monitor drug resistance in imported P. falciparum cases returning from Africa to Shanglin County of Guangxi Province during 2016–2018. Resistance to artemisinin, a widely used front-line antimalarial drug, is increasing. Mutations in the Pfkelch13 gene have served as useful molecular markers for ART-R (Ariey et al., 2014). To date, more than 200 non-synonymous mutations in the Pfkelch13 gene have been reported (MalariaGEN Plasmodium falciparum Community Project, 2016; WHO, 2018), and several validated resistance mutations in the Pfkelch13 gene have been described that are associated with delayed parasite clearance in vitro or in vivo, or both. These include F446I, N458Y, M476I, Y493H, R539T, I543T, P553L, R561H, and C580Y (Amaratunga et al., 2014a,b; Ariey et al., 2014; Ashley et al., 2014; Huang et al., 2015; Straimer et al., 2015; Takala-Harrison et al., 2015; WHO, 2018). These changes have been found in distinct geographic regions. For example, F446I, R539T, I543T, P553L, and C580Y have been found in high prevalence in the Greater Mekong Subregion (GMS) (Wang et al., 2015), while the most frequent mutation found along the Thailand–Myanmar border and in Cambodia, Thailand, and Laos is the C580Y variant (Ariey et al., 2014; Menard et al., 2016; Phyo et al., 2016; Imwong et al., 2017). Previous research has shown that the mutations at residues 539 and 580 were also observed in migrant workers returning from Ghana (Feng et al., 2015a), and other mutations were detected from African samples, including T149S, K189T/N, P574L, Q613E, and A675V (Conrad et al., 2014a; Torrentino-Madamet et al., 2014; Tacoli et al., 2016; Ikeda et al., 2018). In this study, two Ghanaian samples with mutations known to convey ART-R phenotypes were found at residue 446 of the Pfkelch13 gene, marking the first time this genotype has been identified in samples from West Africa. As a validated resistance mutation in the Pfkelch13, the mutant F446I is mainly found proximal to the China–Myanmar border and has been associated with high RSA (Zhang et al., 2017; Wang et al., 2018) and delayed parasite clearance (Feng et al., 2015b; Huang et al., 2015; Wang et al., 2018). It has not been detected before in Africa.
The infections of patients treated in this study with IV artesunate followed by oral DHP resolved 3–5 days thereafter, suggesting that ART-R succeeded even against parasites bearing the F446I mutation. The clinical success may relate to the use of IV treatment with artesunate, which is typically reserved for severe cases but which was used for all patients in this study. Treatment modality deserves further consideration as an explanation for clinical success in these instances (Wang et al., 2019). It may also be true that F446I-bearing parasites remained sensitive to artemisinin owing to the presence (or absence) of additional mutations relevant to drug sensitivity. Among all samples, the highest frequency of mutations was K189T/N, T149S, or an insertion of N, NN, and NNN, which is consistent with a previous report from Dakar (Torrentino-Madamet et al., 2014). However, there is no definitive evidence that the mutations at 189 and 149 are related to ART-R (Miotto et al., 2015).
Only three residues (amino acids 74, 75, and 76) were found to be mutated in the Pfcrt gene, of which 76 had the highest frequency of change with 17.6% prevalence. No mutations were found at positions 72 and 73, which was consistent with previous studies of P. falciparum imported to Shandong Province, China (Xu et al., 2018). Previous work has shown that the Pfcrt 76T mutation was the main marker for CQR (Fidock et al., 2000), while research conducted in Nigeria demonstrated an association between the 76T mutation in the Pfcrt gene and reduced susceptibility to artemether in vitro (Bustamante et al., 2012). In addition, increased frequency of the 76T allele raises concerns about the use of ACTs because it has been found after using artemether-lumefantrine (AL) and dihydroartemisinin-piperaquine (DHAP) in Burkina Faso (Baraka et al., 2015). We also found five haplotypes of the Pfcrt gene at positions 72–76, including CVMNK (wild type), CVIET, CVIEK, CVMNT (mutation type), and CVM/I N/E K/T (multiple clonal infection). The prevalence of the Pfcrt wild-type haplotype was high (80.8%), which is consistent with a previous report of the prevalence of this allele in Ghana (90%; Osarfo et al., 2018). This result was also similar to a recent report on imported malaria, in which the prevalence of wild-type Pfcrt CQ sensitive parasites from different regions of Africa was dominant (Lu et al., 2017c). In this study, CVIET (13.5%) was more frequently observed than other mutant haplotypes, and this finding was similar in samples of malaria parasites acquired from either West Africa or Non-West African regions. The prevalence of molecular markers associated with CQ resistance has decreased since changes in treatment have been enacted (Conrad et al., 2014b). For example, in Malawi and Tanzania, Pfcrt alleles associated with CQ sensitivity have reemerged after CQ withdrawal (Kublin et al., 2003; Laufer et al., 2006; Mohammed et al., 2013). This suggests that CQ could possibly be reintroduced as an effective antimalarial drug in the future (Duah et al., 2013; Lu et al., 2017c).
Mutations in the Pfmdr1 gene at residues 86, 184, 1034, 1042, and 1246 have been reported to be associated with resistance to multiple antimalarial drugs. In our study, we found five non-synonymous mutations at positions 86, 89, 184, 1226, and 1246. The main mutation sites were 86 and 184, being found in 13.1 and 58.8% of the samples, respectively. As far as we know, D89G, which we detected at a frequency of 0.5%, has not been described before as a resistance allele. Its role in drug resistance remains to be confirmed. The F1226Y mutation has previously been detected in imported isolates from Africa (Yao et al., 2018). Together, our data and the literature have revealed that at least 11 haplotypes are possible at positions 86, 184, and 1246 in the Pfmdr1 gene. The high-frequency N86, 184F, and D1246 alleles have been previously reported to be correlated with selection in vivo with AL (Vinayak et al., 2010; Baliraine and Rosenthal, 2011; Thomsen et al., 2011, 2013). A mutation N86Y in Pfmdr1 increases sensitivity to lumefatrine and mefroquine (Menard and Dondorp, 2017). In Africa, the treatment of uncomplicated malaria has changed from a CQ-based approach to one based on AL and DHAP (Duah et al., 2013; Gadalla et al., 2013). The difference of NFD and YFD that we have observed among imported samples from West Africa and Non-West Africa may be related to subtle differences in treatment schemes (and thereby selective pressure) among the countries in Africa.
In conclusion, this study detected the prevalence of polymorphisms in Pfkelch13, Pfcrt, and Pfmdr1 genes in Chinese migrant workers returning to Guangxi Province. Mutations at residue 446 in the Pfkelch13 gene, which is associated with ART-R(Wang et al., 2018), was detected for the first time in authentic malaria samples from West Africa in our study, but it did not coincide with clinical evidence of ART-R. Pfcrt gene polymorphisms were rare, and the wild-type haplotype was more frequently identified, suggesting that CQ could be used as an effective antimalarial drug in the future. The frequencies of Pfmdr1 N86Y and Y184F alleles were most abundant, while the D1246Y allele was low. These observations suggest that AL may not be an effective choice for treatment.
The raw data supporting the conclusions of this article will be made available by the authors, without undue reservation.
HZ, LP, and LZ: conceptualization, investigation, writing—original draft, and visualization. YQ: validation and investigation. WZe: investigation, methodology, supervision. WZe and MP: validation and data curation. ZX: data curation. YW: visualization and supervision. DM: writing—review and editing. YH: resources: project administration and conceptualization. ZY: conceptualization, methodology, and funding acquisition. QY, XinL, CZ, YL, WZh, MD, XiaL, and XW: resources and validation. All authors contributed to the article and approved the submitted version.
This study was supported by the National Science Foundation of China (31860604 and U1802286), major science and technology projects of Yunnan Province (2018ZF0081), and the International Science and Technology Cooperation–Yunnan International Science and Technology Cooperation Base (202003AE140004). YL was funded by grants of the Science and Technology Bureau Programs for Science and Technology Development of Nanning (ZC20153012). YQ was funded by grants of Guangxi Zhuang Autonomous Region Health Commission of Scientific Research Project (Z20190892). CZ was funded by grants of the Youth Fund Project of People's Hospital of Guangxi Zhuang Autonomous Region, China (No. QN2017-10). WZe was under the support of the Education Department Fund of Yunnan Province (2019J1184). XC and ZX were under sponsoring from the Yunnan Applied Basic Research Projects–Union Foundation [2018FE001-190 and 2019FE001 (-015)]. YW was supported by the Hundred-Talent Program of Kunming Medical University (60117190439), Foundation of the Education Department of Yunnan Province (2018JS151), and the Innovation Experiment Project of Yunnan Province (202010678064) and Kunming Medical University (2020JXD014).
The authors declare that the research was conducted in the absence of any commercial or financial relationships that could be construed as a potential conflict of interest.
All claims expressed in this article are solely those of the authors and do not necessarily represent those of their affiliated organizations, or those of the publisher, the editors and the reviewers. Any product that may be evaluated in this article, or claim that may be made by its manufacturer, is not guaranteed or endorsed by the publisher.
The authors thank all the patients for volunteering to participate in the study.
The Supplementary Material for this article can be found online at: https://www.frontiersin.org/articles/10.3389/fgene.2021.701750/full#supplementary-material
Amaratunga, C., Witkowski, B., Dek, D., Try, V., Khim, N., Miotto, O., et al. (2014a). Plasmodium falciparum founder populations in western Cambodia have reduced artemisinin sensitivity in vitro. Antimicrob. Agents Chemother. 58, 4935–4937. doi: 10.1128/AAC.03055-14
Amaratunga, C., Witkowski, B., Khim, N., Menard, D., and Fairhurst, R. M. (2014b). Artemisinin resistance in Plasmodium falciparum. Lancet Infect. Dis. 14, 449–450. doi: 10.1016/S1473-3099(14)70777-7
Ariey, F., Witkowski, B., Amaratunga, C., Beghain, J., Langlois, A. C., Khim, N., et al. (2014). A molecular marker of artemisinin-resistant Plasmodium falciparum malaria. Nature 505, 50–55. doi: 10.1038/nature12876
Ashley, E. A., Dhorda, M., Fairhurst, R. M., Amaratunga, C., Lim, P., Suon, S., et al. (2014). Spread of artemisinin resistance in Plasmodium falciparum malaria. N. Engl. J. Med. 371, 411–423. doi: 10.1056/NEJMoa1314981
Awasthi, G., and Das, A. (2013). Genetics of chloroquine-resistant malaria: a haplotypic view. Mem. Inst. Oswaldo Cruz 108, 947–961. doi: 10.1590/0074-0276130274
Baliraine, F. N., and Rosenthal, P. J. (2011). Prolonged selection of pfmdr1 polymorphisms after treatment of falciparum malaria with artemether-lumefantrine in Uganda. J. Infect. Dis. 204, 1120–1124. doi: 10.1093/infdis/jir486
Baraka, V., Tinto, H., Valea, I., Fitzhenry, R., Delgado-Ratto, C., Mbonye, M. K., et al. (2015). In vivo selection of Plasmodium falciparum Pfcrt and Pfmdr1 variants by artemether-lumefantrine and dihydroartemisinin-piperaquine in Burkina Faso. Antimicrob. Agents Chemother. 59, 734–737. doi: 10.1128/AAC.03647-14
Boussaroque, A., Fall, B., Madamet, M., Camara, C., Benoit, N., Fall, M., et al. (2016). Emergence of mutations in the K13 propeller gene of Plasmodium falciparum isolates from Dakar, Senegal, in 2013-2014. Antimicrob. Agents Chemother. 60, 624–627. doi: 10.1128/AAC.01346-15
Bustamante, C., Folarin, O. A., Gbotosho, G. O., Batista, C. N., Mesquita, E. A., Brindeiro, R. M., et al. (2012). In vitro-reduced susceptibility to artemether in P. falciparum and its association with polymorphisms on transporter genes. J. Infect. Dis. 206, 324–332. doi: 10.1093/infdis/jis359
Conrad, M. D., Bigira, V., Kapisi, J., Muhindo, M., Kamya, M. R., Havlir, D. V., et al. (2014a). Polymorphisms in K13 and falcipain-2 associated with artemisinin resistance are not prevalent in Plasmodium falciparum isolated from Ugandan children. PLoS ONE 9:e105690. doi: 10.1371/journal.pone.0105690
Conrad, M. D., Leclair, N., Arinaitwe, E., Wanzira, H., Kakuru, A., Bigira, V., et al. (2014b). Comparative impacts over 5 years of artemisinin-based combination therapies on Plasmodium falciparum polymorphisms that modulate drug sensitivity in Ugandan children. J. Infect. Dis. 210, 344–353. doi: 10.1093/infdis/jiu141
Duah, N. O., Matrevi, S. A., De Souza, D. K., Binnah, D. D., Tamakloe, M. M., Opoku, V. S., et al. (2013). Increased pfmdr1 gene copy number and the decline in pfcrt and pfmdr1 resistance alleles in Ghanaian Plasmodium falciparum isolates after the change of anti-malarial drug treatment policy. Malar. J. 12:377. doi: 10.1186/1475-2875-12-377
Duraisingh, M. T., Drakeley, C. J., Muller, O., Bailey, R., Snounou, G., Targett, G. A., et al. (1997). Evidence for selection for the tyrosine-86 allele of the pfmdr 1 gene of Plasmodium falciparum by chloroquine and amodiaquine. Parasitology 114 (Pt 3), 205–211. doi: 10.1017/S0031182096008487
Feng, J., Li, J., Yan, H., Feng, X., and Xia, Z. (2015a). Evaluation of antimalarial resistance marker polymorphism in returned migrant workers in China. Antimicrob. Agents Chemother. 59, 326–330. doi: 10.1128/AAC.04144-14
Feng, J., Yan, H., Feng, X. Y., Zhang, L., Li, M., Xia, Z. G., et al. (2014). Imported malaria in China, 2012. Emerging Infect. Dis. 20, 1778–1780. doi: 10.3201/eid2010.140595
Feng, J., Zhang, L., Huang, F., Yin, J. H., Tu, H., Xia, Z. G., et al. (2018). Ready for malaria elimination: zero indigenous case reported in the People's Republic of China. Malar. J. 17:315. doi: 10.1186/s12936-018-2444-9
Feng, J., Zhou, D., Lin, Y., Xiao, H., Yan, H., and Xia, Z. (2015b). Amplification of pfmdr1, pfcrt, pvmdr1, and K13 propeller polymorphisms associated with Plasmodium falciparum and Plasmodium vivax isolates from the China-Myanmar border. Antimicrob. Agents Chemother. 59, 2554–2559. doi: 10.1128/AAC.04843-14
Fidock, D. A., Nomura, T., Talley, A. K., Cooper, R. A., Dzekunov, S. M., Ferdig, M. T., et al. (2000). Mutations in the P. falciparum digestive vacuole transmembrane protein PfCRT and evidence for their role in chloroquine resistance. Mol. Cell 6, 861–871. doi: 10.1016/S1097-2765(05)00077-8
Fogh, S., Jepsen, S., and Effersoe, P. (1979). Chloroquine-resistant Plasmodium falciparum malaria in Kenya. Trans. R. Soc. Trop. Med. Hyg. 73, 228–229. doi: 10.1016/0035-9203(79)90220-7
Folarin, O. A., Bustamante, C., Gbotosho, G. O., Sowunmi, A., Zalis, M. G., Oduola, A. M., et al. (2011). In vitro amodiaquine resistance and its association with mutations in pfcrt and pfmdr1 genes of Plasmodium falciparum isolates from Nigeria. Acta Trop. 120, 224–230. doi: 10.1016/j.actatropica.2011.08.013
Gadalla, N. B., Abdallah, T. M., Atwal, S., Sutherland, C. J., and Adam, I. (2013). Selection of pfdhfr/pfdhps alleles and declining artesunate/sulphadoxine-pyrimethamine efficacy against Plasmodium falciparum eight years after deployment in eastern Sudan. Malar. J. 12:255. doi: 10.1186/1475-2875-12-255
Huang, F., Takala-Harrison, S., Jacob, C. G., Liu, H., Sun, X., Yang, H., et al. (2015). A Single mutation in K13 predominates in Southern China and is associated with delayed clearance of Plasmodium falciparum following artemisinin treatment. J. Infect. Dis. 212, 1629–1635. doi: 10.1093/infdis/jiv249
Humphreys, G. S., Merinopoulos, I., Ahmed, J., Whitty, C. J., Mutabingwa, T. K., Sutherland, C. J., et al. (2007). Amodiaquine and artemether-lumefantrine select distinct alleles of the Plasmodium falciparum mdr1 gene in Tanzanian children treated for uncomplicated malaria. Antimicrob. Agents Chemother. 51, 991–997. doi: 10.1128/AAC.00875-06
Ikeda, M., Kaneko, M., Tachibana, S.-I., Balikagala, B., Sakurai-Yatsushiro, M., Yatsushiro, S., et al. (2018). Artemisinin-resistant Plasmodium falciparum with high survival rates, Uganda, 2014-2016. Emerging Infect. Dis. 24, 718–726. doi: 10.3201/eid2404.170141
Imwong, M., Suwannasin, K., Kunasol, C., Sutawong, K., Mayxay, M., Rekol, H., et al. (2017). The spread of artemisinin-resistant Plasmodium falciparum in the Greater Mekong subregion: a molecular epidemiology observational study. Lancet Infect. Dis. 17, 491–497. doi: 10.1016/S1473-3099(17)30048-8
Kublin, J. G., Cortese, J. F., Njunju, E. M., Mukadam, R. A., Wirima, J. J., Kazembe, P. N., et al. (2003). Reemergence of chloroquine-sensitive Plasmodium falciparum malaria after cessation of chloroquine use in Malawi. J. Infect. Dis. 187, 1870–1875. doi: 10.1086/375419
Lai, S., Sun, J., Ruktanonchai, N. W., Zhou, S., Yu, J., Routledge, I., et al. (2019). Changing epidemiology and challenges of malaria in China towards elimination. Malar. J. 18:107. doi: 10.1186/s12936-019-2736-8
Laufer, M. K., Thesing, P. C., Eddington, N. D., Masonga, R., Dzinjalamala, F. K., Takala, S. L., et al. (2006). Return of chloroquine antimalarial efficacy in Malawi. N. Engl. J. Med. 355, 1959–1966. doi: 10.1056/NEJMoa062032
Lekostaj, J. K., Amoah, L. E., and Roepe, P. D. (2008). A single S1034C mutation confers altered drug sensitivity to PfMDR1 ATPase activity that is characteristic of the 7G8 isoform. Mol. Biochem. Parasitol. 157, 107–111. doi: 10.1016/j.molbiopara.2007.09.008
Li, J., Chen, J., Xie, D., Eyi, U. M., Matesa, R. A., Obono, M. M. O., et al. (2015). Molecular mutation profile of Pfcrt and Pfmdr1 in Plasmodium falciparum isolates from Bioko Island, Equatorial Guinea. Infect. Genet. Evol. 36, 552–556. doi: 10.1016/j.meegid.2015.08.039
Li, J., Shi, Y., Zhang, W., Yan, H., Lin, K., Wei, S., et al. (2019). K13-propeller gene polymorphisms of Plasmodium falciparum and the therapeutic effect of artesunate among migrant workers returning to Guangxi, China (2014-2017). Malar. J. 18:349. doi: 10.1186/s12936-019-2984-7
Liu, Y., Hsiang, M. S., Zhou, H., Wang, W., Cao, Y., Gosling, R. D., et al. (2014). Malaria in overseas labourers returning to China: an analysis of imported malaria in Jiangsu Province, 2001-2011. Malar. J. 13:29. doi: 10.1186/1475-2875-13-29
Lu, F., Culleton, R., and Cao, J. (2017a). Artemisinin-Resistant Plasmodium falciparum in Africa. N. Engl. J. Med. 377:306. doi: 10.1056/NEJMc1705789
Lu, F., Culleton, R., Zhang, M., Ramaprasad, A., Von Seidlein, L., Zhou, H., et al. (2017b). Emergence of indigenous artemisinin-resistant Plasmodium falciparum in Africa. N. Engl. J. Med. 376, 991–993. doi: 10.1056/NEJMc1612765
Lu, F., Zhang, M., Culleton, R. L., Xu, S., Tang, J., Zhou, H., et al. (2017c). Return of chloroquine sensitivity to Africa? Surveillance of African Plasmodium falciparum chloroquine resistance through malaria imported to China. Parasit. Vectors 10:355. doi: 10.1186/s13071-017-2298-y
MalariaGEN Plasmodium falciparum Community Project (2016). Genomic epidemiology of artemisinin resistant malaria. Elife 5:e08714. doi: 10.7554/eLife.08714
Menard, D., and Dondorp, A. (2017). Antimalarial drug resistance: a threat to malaria elimination. Cold Spring Harb. Perspect. Med. 7:a025619. doi: 10.1101/cshperspect.a025619
Menard, D., Khim, N., Beghain, J., Adegnika, A. A., Shafiul-Alam, M., Amodu, O., et al. (2016). A worldwide map of Plasmodium falciparum K13-propeller polymorphisms. N. Engl. J. Med. 374, 2453–2464. doi: 10.1056/NEJMoa1513137
Miotto, O., Amato, R., Ashley, E. A., Macinnis, B., Almagro-Garcia, J., Amaratunga, C., et al. (2015). Genetic architecture of artemisinin-resistant Plasmodium falciparum. Nat. Genet. 47, 226–234. doi: 10.1038/ng.3189
Mita, T., Tachibana, S., Hashimoto, M., and Hirai, M. (2016). Plasmodium falciparum kelch 13: a potential molecular marker for tackling artemisinin-resistant malaria parasites. Expert Rev. Anti Infect. Ther. 14, 125–135. doi: 10.1586/14787210.2016.1106938
Mohammed, A., Ndaro, A., Kalinga, A., Manjurano, A., Mosha, J. F., Mosha, D. F., et al. (2013). Trends in chloroquine resistance marker, Pfcrt-K76T mutation ten years after chloroquine withdrawal in Tanzania. Malar. J. 12:415. doi: 10.1186/1475-2875-12-415
Ocan, M., Akena, D., Nsobya, S., Kamya, M. R., Senono, R., Kinengyere, A. A., et al. (2019). K13-propeller gene polymorphisms in Plasmodium falciparum parasite population in malaria affected countries: a systematic review of prevalence and risk factors. Malar. J. 18:60. doi: 10.1186/s12936-019-2701-6
Osarfo, J., Tagbor, H., Magnussen, P., and Alifrangis, M. (2018). Molecular markers of Plasmodium falciparum drug resistance in parasitemic pregnant women in the middle forest Belt of Ghana. Am. J. Trop. Med. Hyg. 98, 1714–1717. doi: 10.4269/ajtmh.18-0009
Phyo, A. P., Ashley, E. A., Anderson, T. J. C., Bozdech, Z., Carrara, V. I., Sriprawat, K., et al. (2016). Declining efficacy of artemisinin combination therapy against p. falciparum malaria on the thai-myanmar border (2003-2013): the role of parasite genetic factors. Clin. Infect. Dis. 63, 784–791. doi: 10.1093/cid/ciw388
She, D., Wang, Z., Liang, Q., Lu, L., Huang, Y., Zhang, K., et al. (2020). Polymorphisms of pfcrt, pfmdr1, and K13-propeller genes in imported falciparum malaria isolates from Africa in Guizhou province, China. BMC Infect. Dis. 20:513. doi: 10.1186/s12879-020-05228-8
Shi, S. M., Shi, T. Q., Chen, S. B., Cui, Y. B., Kassegne, K., Okpeku, M., et al. (2021). Genome-wide scans for ghanaian Plasmodium falciparum Genes under selection from local and chinese host populations. Front. Cell. Infect. Microbiol. 11:630797. doi: 10.3389/fcimb.2021.630797
Sidhu, A. B., Valderramos, S. G., and Fidock, D. A. (2005). pfmdr1 mutations contribute to quinine resistance and enhance mefloquine and artemisinin sensitivity in Plasmodium falciparum. Mol. Microbiol. 57, 913–926. doi: 10.1111/j.1365-2958.2005.04729.x
Straimer, J., Gnadig, N. F., Witkowski, B., Amaratunga, C., Duru, V., Ramadani, A. P., et al. (2015). Drug resistance. K13-propeller mutations confer artemisinin resistance in Plasmodium falciparum clinical isolates. Science 347, 428–431. doi: 10.1126/science.1260867
Tacoli, C., Gai, P. P., Bayingana, C., Sifft, K., Geus, D., Ndoli, J., et al. (2016). Artemisinin resistance-associated K13 polymorphisms of Plasmodium falciparum in Southern Rwanda, 2010-2015. Am. J. Trop. Med. Hyg. 95, 1090–1093. doi: 10.4269/ajtmh.16-0483
Takala-Harrison, S., Jacob, C. G., Arze, C., Cummings, M. P., Silva, J. C., Dondorp, A. M., et al. (2015). Independent emergence of artemisinin resistance mutations among Plasmodium falciparum in Southeast Asia. J. Infect. Dis. 211, 670–679. doi: 10.1093/infdis/jiu491
Thomsen, T. T., Ishengoma, D. S., Mmbando, B. P., Lusingu, J. P., Vestergaard, L. S., Theander, T. G., et al. (2011). Prevalence of single nucleotide polymorphisms in the Plasmodium falciparum multidrug resistance gene (Pfmdr-1) in Korogwe District in Tanzania before and after introduction of artemisinin-based combination therapy. Am. J. Trop. Med. Hyg. 85, 979–983. doi: 10.4269/ajtmh.2011.11-0071
Thomsen, T. T., Madsen, L. B., Hansson, H. H., Tomas, E. V., Charlwood, D., Bygbjerg, I. C., et al. (2013). Rapid selection of Plasmodium falciparum chloroquine resistance transporter gene and multidrug resistance gene-1 haplotypes associated with past chloroquine and present artemether-lumefantrine use in Inhambane District, southern Mozambique. Am. J. Trop. Med. Hyg. 88, 536–541. doi: 10.4269/ajtmh.12-0525
Tinto, H., Guekoun, L., Zongo, I., Guiguemde, R. T., D'alessandro, U., and Ouedraogo, J. B. (2008). Chloroquine-resistance molecular markers (Pfcrt T76 and Pfmdr-1 Y86) and amodiaquine resistance in Burkina Faso. Trop. Med. Int. Health 13, 238–240. doi: 10.1111/j.1365-3156.2007.01995.x
Torrentino-Madamet, M., Fall, B., Benoit, N., Camara, C., Amalvict, R., Fall, M., et al. (2014). Limited polymorphisms in k13 gene in Plasmodium falciparum isolates from Dakar, Senegal in 2012-2013. Malar. J. 13:472. doi: 10.1186/1475-2875-13-472
Trape, J. F. (2001). The public health impact of chloroquine resistance in Africa. Am. J. Trop. Med. Hyg. 64, 12–17. doi: 10.4269/ajtmh.2001.64.12
Vinayak, S., Alam, M. T., Sem, R., Shah, N. K., Susanti, A. I., Lim, P., et al. (2010). Multiple genetic backgrounds of the amplified Plasmodium falciparum multidrug resistance (pfmdr1) gene and selective sweep of 184F mutation in Cambodia. J. Infect. Dis. 201, 1551–1560. doi: 10.1086/651949
Wang, J., Huang, Y., Zhao, Y., Ye, R., Zhang, D., and Pan, W. (2018). Introduction of F446I mutation in the K13 propeller gene leads to increased ring survival rates in Plasmodium falciparum isolates. Malar. J. 17:248. doi: 10.1186/s12936-018-2396-0
Wang, J., Xu, C., Liao, F. L., Jiang, T., Krishna, S., and Tu, Y. (2019). A temporizing solution to “artemisinin resistance”. N. Engl. J. Med. 380, 2087–2089. doi: 10.1056/NEJMp1901233
Wang, X., Ruan, W., Zhou, S., Huang, F., Lu, Q., Feng, X., et al. (2020). Molecular surveillance of Pfcrt and k13 propeller polymorphisms of imported Plasmodium falciparum cases to Zhejiang Province, China between 2016 and 2018. Malar. J. 19:59. doi: 10.1186/s12936-020-3140-0
Wang, Z., Shrestha, S., Li, X., Miao, J., Yuan, L., Cabrera, M., et al. (2015). Prevalence of K13-propeller polymorphisms in Plasmodium falciparum from China-Myanmar border in 2007-2012. Malar. J. 14:168. doi: 10.1186/s12936-015-0672-9
Warhurst, D. C. (2001). A molecular marker for chloroquine-resistant falciparum malaria. N. Engl. J. Med. 344, 299–302. doi: 10.1056/NEJM200101253440411
Wellems, T. E., and Plowe, C. V. (2001). Chloroquine-Resistant Malaria. J. Infect. Dis. 184, 770–776. doi: 10.1086/322858
Xu, C., Wei, Q., Yin, K., Sun, H., Li, J., Xiao, T., et al. (2018). Surveillance of antimalarial resistance Pfcrt, Pfmdr1, and Pfkelch13 polymorphisms in African Plasmodium falciparum imported to Shandong Province, China. Sci. Rep. 8:12951. doi: 10.1038/s41598-018-31207-w
Yang, C., Zhang, H., Zhou, R., Qian, D., Liu, Y., Zhao, Y., et al. (2017). Polymorphisms of Plasmodium falciparum k13-propeller gene among migrant workers returning to Henan Province, China from Africa. BMC Infect. Dis. 17:560. doi: 10.1186/s12879-017-2634-z
Yao, Y., Wu, K., Xu, M., Yang, Y., Zhang, Y., Yang, W., et al. (2018). Surveillance of genetic variations associated with antimalarial resistance of Plasmodium falciparum isolates from returned migrant workers in Wuhan, Central China. Antimicrob. Agents Chemother. 62:e02387-17. doi: 10.1128/AAC.02387-17
Young, M. D., Contacos, P. G., Stitcher, J. E., and Willar, J. W. (1963). Drug resistance in Plasmodium Falciparum from Thailand. Am. J. Trop. Med. Hyg. 12, 305–314. doi: 10.4269/ajtmh.1963.12.305
Zhang, J., Feng, G. H., Zou, C. Y., Su, P. C., Liu, H. E., and Yang, Z. Q. (2017). Overview of the improvement of the ring-stage survival assay-a novel phenotypic assay for the detection of artemisinin-resistant Plasmodium falciparum. Zool. Res. 38, 317–320. doi: 10.24272/j.issn.2095-8137.2017.075
Zhao, L., Pi, L., Qin, Y., Lu, Y., Zeng, W., Xiang, Z., et al. (2020). Widespread resistance mutations to sulfadoxine-pyrimethamine in malaria parasites imported to China from Central and Western Africa. Int. J. Parasitol. Drugs Drug Resist. 12, 1–6. doi: 10.1016/j.ijpddr.2019.11.002
Keywords: Plasmodium falciparum, antimalarial drug, polymorphism, drug resistance genes Pfkelch13, Pfcrt, Pfmdr1
Citation: Zhao H, Pi L, Zhao L, Qin Y, Zeng W, Xiang Z, Yang Q, Pan M, Li X, Zou C, Chen X, Zhao W, Lu Y, Wu Y, Duan M, Wang X, Li X, Mazier D, Huang Y and Yang Z (2021) First Detection in West Africa of a Mutation That May Contribute to Artemisinin Resistance Plasmodium falciparum. Front. Genet. 12:701750. doi: 10.3389/fgene.2021.701750
Received: 01 May 2021; Accepted: 31 August 2021;
Published: 08 October 2021.
Edited by:
Toshihiro Mita, Juntendo University, JapanReviewed by:
Billy Ngasala, Muhimbili University of Health and Allied Sciences, TanzaniaCopyright © 2021 Zhao, Pi, Zhao, Qin, Zeng, Xiang, Yang, Pan, Li, Zou, Chen, Zhao, Lu, Wu, Duan, Wang, Li, Mazier, Huang and Yang. This is an open-access article distributed under the terms of the Creative Commons Attribution License (CC BY). The use, distribution or reproduction in other forums is permitted, provided the original author(s) and the copyright owner(s) are credited and that the original publication in this journal is cited, in accordance with accepted academic practice. No use, distribution or reproduction is permitted which does not comply with these terms.
*Correspondence: Zhaoqing Yang, emhhb3Fpbmd5OTJAaG90bWFpbC5jb20=
†These authors have contributed equally to this work
Disclaimer: All claims expressed in this article are solely those of the authors and do not necessarily represent those of their affiliated organizations, or those of the publisher, the editors and the reviewers. Any product that may be evaluated in this article or claim that may be made by its manufacturer is not guaranteed or endorsed by the publisher.
Research integrity at Frontiers
Learn more about the work of our research integrity team to safeguard the quality of each article we publish.