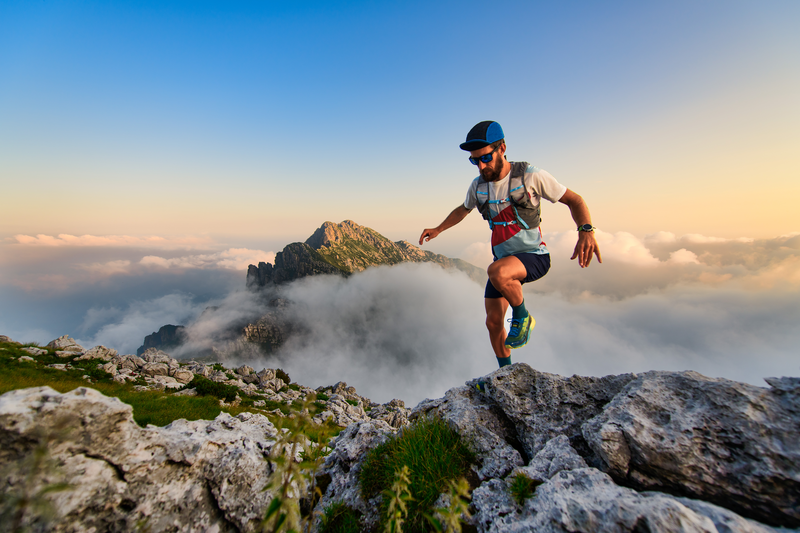
94% of researchers rate our articles as excellent or good
Learn more about the work of our research integrity team to safeguard the quality of each article we publish.
Find out more
ORIGINAL RESEARCH article
Front. Genet. , 01 July 2021
Sec. Livestock Genomics
Volume 12 - 2021 | https://doi.org/10.3389/fgene.2021.672888
This article is part of the Research Topic The Effects of Genetic Mutations in Poultry View all 4 articles
The Ras and Rab interactor 2 (RIN2) gene, which encodes RAS and Rab interacting protein 2, can interact with GTP-bound Rab5 and participate in early endocytosis. This study found a 61-bp insertion/deletion (indel) in the RIN2 intron region, and 3 genotypes II, ID, and DD were observed. Genotype analysis of mutation sites was performed on 665 individuals from F2 population and 8 chicken breeds. It was found that the indel existed in each breed and that yellow feathered chickens were mainly of the DD genotype. Correlation analysis of growth and carcass traits in the F2 population of Xinghua and White Recessive Rock chickens showed that the 61-bp indel was significantly correlated with abdominal fat weight, abdominal fat rate, fat width, and hatching weight (P < 0.05). RIN2 mRNA was expressed in all the tested tissues, and its expression in abdominal fat was higher than that in other tissues. In addition, the expression of the RIN2 mRNA in the abdominal fat of the DD genotype was significantly higher than that of the II genotype (P < 0.05). The transcriptional activity results showed that the luciferase activity of the pGL3-DD vector was significantly higher than that of the pGL3-II vector (P < 0.01). Moreover, the results indicate that the polymorphisms in transcription factor binding sites (TFBSs) of 61-bp indel may affect the transcriptional activity of RIN2, and thus alter fat traits in chicken. The results of this study showed that the 61-bp indel was closely related to abdominal fat-related and hatching weight traits of chickens, which may have reference value for molecular marker-assisted selection of chickens.
The Ras and Rab interactor 2 (RIN2) gene, which encodes RAS and Rab interacting protein 2, functions as a guanine nucleotide exchange factor (GEF). RIN2 has been shown to interact with Rab5, a small GTPase that participates in early endocytosis (Saito et al., 2002; Grosshans et al., 2006). Rab5 is necessary for the transport of endocytic vesicles to early endosomes (Saito et al., 2002). Deletion of RIN2 may impair Rab5-related endosome signaling and may also damage the secretion of proteins from the endoplasmic reticulum to the Golgi apparatus or from the Golgi apparatus to the plasma membrane, leading to collagen fiber structure, and phenotypic abnormalities (Syx et al., 2010).
Previous research has shown that RIN2 syndrome in humans is also called MACS (macrocephaly, alopecia, cutis laxa, and scoliosis) syndrome, a rare hereditary skin disease caused by the loss of the 1-bp homozygote of RIN2 (Syx et al., 2010; Kameli et al., 2020). A genome-wide selective scan of purebred horses showed that RIN2 played similar roles in signal transmission, indicating that RIN2 is under strong artificial selection in racing horses (Moon et al., 2015). Comparative analysis of the genome-wide methylation and transcriptome of the longest muscle in sheep showed that RIN2 may be a functional gene that affects meat quality traits (Cao et al., 2017). However, no research has reported on the functions of RIN2 relating to animal production, and its exact functional mechanism in chickens remains unclear.
Indels are a major source of molecular-level variations and have been widely used as molecular markers in the study of economic traits in livestock (Cui et al., 2018). Due to the advantages of convenient detection and remarkable effects, compared with single nucleotide polymorphism (SNP), the indel variants have higher efficiency, and wider application (Li et al., 2017). Indels play an important role in genetic diversity and phenotypic differentiation (Rao et al., 2010; Yan et al., 2014). In poultry, studies have shown that indels are significantly related to chicken growth traits, carcass traits, and other economic traits (Li et al., 2006; Tang et al., 2011; Liang et al., 2019; Liu et al., 2019; Ren et al., 2019). In cattle, indels are significantly related to growth traits and meat quality (Wei et al., 2018; Xu et al., 2018).
In this study, we analyzed whole-genome sequence data, which has been deposited in the archive of the Beijing Institute of Genomics1 under accession number CRA000005 (Hou et al., 2020). Chicken RIN2 is located on chromosome 3 and consists of 20 exons, encoding an 836 amino acid protein. The purpose of this study was to detect internal variation in RIN2 and clarify the impact of its mutations on the economic traits of chickens.
All animal experiments performed in this study complied with the requirements of the Institutional Animal Protection and Utilization Committee of South China Agricultural University (approval ID: SCAU # 0014). The care and use of animals complied with the local animal welfare laws.
The F2 resource population was made up of reciprocal cross between White Recessive Rock (WRR) and Xinghua chickens (XH). WRR are fast-growing broilers, and XH are a slow-growing Chinese native breed. All F2 individuals (n = 304) were slaughtered at 90 days of age. More information was provided in a previous study (Lei et al., 2005).
To confirm the distribution of the RIN2 genotypic variation in other breeds of chickens, genomic DNA was extracted from a total of 361 healthy individuals from eight breeds. The numbers of samples from each breed were: White Recessive Rock chickens (WRR, n = 41), White Leghorn chickens (WHL, n = 47), Wenchang chickens (WC, n = 48), Qingjiaoma chickens (QJ, n = 48), Lushi chickens (LS, n = 40), Guangxi yellow chickens (GX, n = 46), Gushi chickens (GS, n = 44), and Xinghua chickens (XH, n = 47).
To detect the expression of RIN2 mRNA in different tissues, a total of 12 tissues (heart, liver, spleen, lung, kidney, breast muscle, leg muscle, abdomen fat, jejunum, duodenum, hypothalamus, and ovary) were collected from 20-week-old yellow chickens, with four samples of each tissue. DNA was extracted from the blood to determine the genotype. The abdominal fat tissues of 4-week-old yellow chickens from 3 individuals each of the II, ID, and DD genotypes were used to detect the RIN2 mRNA expression of different genotypes. All tissues were stored at −80°C.
Genomic DNA was extracted from blood using a Blood DNA Kit (Omega, Norcross, America). All the primers used in this study were designed using the online tools provided by NCBI2 and were synthesized by Beijing TsingKe Company (Supplementary Table 1). PCR was performed in a total volume of 10 μL, including 1 μL of genomic DNA (50 ng/μL), 0.2 μL of each primer (10 μmol/L), 5 μL of 2 × M5 PCR Mix (Yuexing, Guangzhou, China), and 3.6 μL of double distilled H2O. The PCR cycle profiles were 95°C for 3 min, 95°C for 25 s, 61°C for 25 s, 72°C for 15 s, and 72°C extension for 5 min for a total of 31 cycles, followed by refrigeration at 4°C. An aliquot (7 μL) of each reaction was electrophoresed on a 2% agarose gel to determine the genotype.
The genotype and allele frequencies of the mutation were calculated directly for different breeds. Hardy-Weinberg equilibrium (HWE) was analyzed using the SHEsis website3. The allele number (Ne), genetic indices of heterozygosity (He), polymorphism information content (PIC), and population differentiation were analyzed using PopGene software Version 1.3.1 (Yeh et al., 1999).
Total RNA from tissues were extracted using TRIzol Reagent (Takara, Dalian, China) following the manufacturer’s protocol. First strand cDNA was synthesized using the Prime ScriptTM RT Reagent kit (Takara, Dalian, China). Quantitative real-time polymerase chain reaction (qPCR) was used to investigate the expression levels of RIN2 mRNA in each tissue. qPCR was performed using the CFX96 system (Bio-Rad, Hercules, CA, United States). Three repetitions were performed per sample. The β-actin gene was used as an internal control. The primer information is provided in Supplementary Table 1. The qPCR conditions were as follows: 95°C for 5 min, 95°C for 30 s, 60°C for 30 s, and 72°C for 30 s for a total of 35 cycles. The results were analyzed using the 2−ΔΔCTmethod (Schmittgen and Livak, 2008).
The chicken dermal fibroblast cell line DF-1 was obtained from Guangdong Provincial Key Lab of Agro-Animal Genomics and Molecular Breeding (Guangzhou, China). DF-1 cells were cultured in basic DMEM (Gibco, Carlsbad, CA, United States) with 10% fetal serum (Gibco, Carlsbad, CA, United States) and 1% streptomycin/penicillin (Invitrogen, Carlsbad, CA, United States) at 37°C with 5% CO2. A Lipofectamine 3000 kit (Invitrogen, Carlsbad, CA, United States) was used for the transfection of plasmids into cells as described in the next section according to the manufacturer’s protocol. The transfection dose of the plasmids was 0.1 μg/well for 96-well plates.
Based on the sequence diagrams of the chicken RIN2 61-bp indel locus (Figure 2), the luciferase reporter vector pGL3 basic (Promega, Madison, WI, United States) was selected, and the pGL3-II vector (161 bp, containing the 61-bp indel) and pGL3-DD vector (100 bp, lacking the 61-bp indel) were constructed by Gene Create (Wuhan, China) to detect the effect of the RIN2 61-bp indel.
To detect whether transcription factors bind to the 61-bp indel, the GATA-1 binding sequence (TAAATGCAAA) in the II genotype was deleted based on the prediction results of the TFBSs, and the KO-GATA-1 luciferase reporter vector (151 bp) was constructed by Gene Create. The GCN4 binding sequence (CAAGAGTAAA) was deleted in the II genotype to construct the KO-GCN4 luciferase reporter vector (151 bp). The carrier PRL-TK (Promega, Madison, WI, United States) was used as an internal control in the luciferase reporter system.
Prediction of TFBSs was performed using the Alibaba 2.14 programs.
Statistical analysis of all the sequence variations and important economic traits related to the F2 resource group was performed using SPSS software (version 24.0). The mixed linear models used in the analysis are:
where, Yijklm is the observed value, μ is the overall average, Gi is the fixed effect of the genotype, fl is the random effect of the family, Sj is the fixed effect of sex, Hk is the fixed effect of the hatch, b is the carcass weight regression coefficient, is the average slaughter weight, Wijklm is the individual slaughter weight, and eijklm is the random error term. A P-value < 0.05 was considered significant, and Bonferroni’s test was performed to control for multiple comparisons (Ren et al., 2017). Model I was used to assess genotypes related to growth traits and meat quality. Considering the effect of body weight on carcass traits, Model II used carcass weight as a covariate to assess carcass traits.
By analyzing whole-genome sequence data, a new 61-bp insertion mutation was found downstream of the intron of RIN2. As shown in Figure 1, the indel polymorphism was analyzed by PCR amplification of the region and electrophoresis of the product in a 2.0% agarose gel. Three polymorphisms were identified and named II (646 bp), ID (646 bp and 585 bp), and DD (585 bp). The PCR products were sequenced to pinpoint the location of the insertion (Figure 2). As shown in Figure 3, the 61-bp indel is located in intron 8 of RIN2.
The genotype and allele frequencies and other genetic parameters associated with the RIN2 indel locus were calculated for 665 individuals (Table 1). F2 population and 8 breeds had the RIN2 61-bp insertion mutation, and with the exception of WRR, the allele frequency of D was higher than that of I. The distribution of genotypes in the different breeds is shown in Figure 4. The frequency of the II genotype in white feathered chickens was higher than that in yellow feathered chicken.
Figure 4. Percentage of different genotypes in different populations. White feathered chickens (White recessive rock chickens and White leghorn chickens), yellow feathered chickens (Wenchang chickens, Qingjiaoma chickens, Lushi chickens, Guangxi yellow chickens, Gushi chickens, and Xinghua chickens).
The F2, WRR, and WHL breeds showed moderate polymorphism, whereas the remaining breeds showed low polymorphism. The degree of genetic heterozygosity was 0.061–0.476, and the number of effective alleles was 1.255–1.908.
To determine whether differential selection of the RIN2 61-bp insertion sequence occurred during the domestication of chickens, the pairwise fixed index (Fst) was used to analyze the breeds differentiation. The analysis showed that the RIN2 61-bp site insertion strong genetic differentiation between yellow feathered chickens (WC, QJ, LS, GX, GS, and XH) and WRR (0.2 < Fst < 0.5; Table 2), indicating that the insertion mutation may be selected in WRR. The FST values were lower among the other breeds.
In the F2 population, the 61-bp indel of RIN2 was significantly associated with fat traits. Significant correlations were detected with abdominal fat weight, abdominal fat rate and fat width traits (P = 0.046, P = 0.033, and P = 0.005; Table 3). The abdominal fat weight, abdominal fat rate and fat width traits of DD genotypes were greater than those of the ID and II genotypes. There was no significant difference between ID and II individuals. The 61-bp indel was not significantly associated with other carcass traits (Supplementary Table 2).
In the F2 population, the 61-bp indel of RIN2 was significantly related to the hatching weight (P = 0.027; Table 4). The hatching weight of chickens of the II genotype were greater than those of chickens of the ID and DD genotypes. There was no significant difference between the ID and DD genotypes. The other growth traits (shank length, shank diameter, and average daily gain) were not significantly associated with the indel (Supplementary Table 3). The 61-bp indel was not significantly associated with meat quality traits (Supplementary Table 4).
The expression of RIN2 mRNA in various tissues of 20-week-old yellow chickens was studied (Figure 5), and the results showed that RIN2 was expressed in all the tested tissues. The highest expression was found in abdominal fat and the hypothalamus, followed by the lung and liver, with the lowest expression found in the spleen. The high expression of RIN2 in abdominal fat suggest that RIN2 might play a role in the formation of abdominal fat.
Figure 5. Expression of RIN2 mRNA in different tissues detected by qPCR (n = 4 samples of each tissue). ns, no significant difference (P > 0.05); ∗, significant difference (P < 0.05); **, very significant difference (P < 0.01).
In abdominal fat, the II genotype showed significantly lower RIN2 mRNA expression than DD genotype (P < 0.05; Figure 6). This result indicates that the 61-bp indel may affect the expression of RIN2 and may affect slaughter traits, such as abdominal fat weight.
Figure 6. Expression of RIN2 mRNA in abdomen fat tissue of individuals with different genotypes. Data are presented as the means ± SD (n = 3 repetitions for each group). *P < 0.05, significant difference.
According to the prediction results from the Alibaba 2.1 website, the TFBSs of genotype II (161 bp) were: GATA-1, the binding sequence of which is TAAATGCAAA at the 47378-47387 site; GCN4, the binding sequence of which is CAAGAGTAAA, at the 47372-47381 site; and CEBPA, the binding sequence of which is TGTTGAAAGT, at the 47391–47400 site. The TFBSs shared with the DD genotype (100 bp) were SP1, NF-1, E1, and RAP1 (Figure 7).
To determine the effect of the 61-bp indel on cell transcriptional activity, the pGL3-basic, pGL3-II, and pGL3-DD vectors were cotransfected with PRL-TK into DF-1 cells. The results are shown in Figure 8. The luciferase activity of the pGL3-DD vector was 2.51 times greater than that of the pGL3-II genotype (P < 0.01). The luciferase activities of the pGL3-DD, pGL3-II, and pGL3-basic vectors were different (P < 0.01). This result suggests that there may be an inhibitor binding site in the RIN2 61-bp indel. In fact, the results of the transcription factor site prediction showed that GATA-1 and GCN4 inhibited transcription factor binding (Figure 7).
Figure 8. Luciferase activity in DF-1 cells transfected with recombinant plasmids. Data are presented as the means ± SD (n = 6 samples of each plasmid). ∗∗P < 0.01, very significant difference.
To determine whether the GATA-1 or GCN4 transcription factor combined with the 61-bp indel affect cell transcription activity, the GATA-1 and GCN4 binding sequences were deleted and KO-GATA-1 and KO-GCN4 luciferase reporter vectors were constructed. The results are shown in Figure 9. The luciferase activities of the KO-GATA-1 and KO-GCN4 vectors were significantly higher than that of the pGL3-II vector (P < 0.01, P < 0.01), suggesting that GATA-1 or GCN4 inhibitory transcription factors may combine with the 61-bp indel to reduce cell transcription activity. It is worth noting that the luciferase activities of the KO-GATA-1 and KO-GCN4 vectors were also significantly higher than that of the pGL3-DD vector (P < 0.01, P < 0.01), suggesting that there may be a 61-bp indel promoting binding sites. In fact, the results of the transcription factor site prediction showed CEBPA TFBSs (Figure 7).
Figure 9. Luciferase activity in DF-1 cells transfected with recombinant plasmids. Data are presented as the means ± SD (n = 6 samples of each plasmid). Columns with different letters (A,B) indicate P < 0.01, columns with different letters (a,b) indicate P < 0.05, and columns with the same letter indicate P > 0.05.
In animal breeding, the discovery of key genes and molecular mechanisms that affect growth traits is an important step in improving breeding efficiency and accelerating the breeding process (Li et al., 2006; Zhang et al., 2014). To enhance the selection effect of the main traits, traditional selection methods can be complemented by gene-assisted selection or molecular marker-assisted selection (MAS). The application of MAS in selection procedures would help in improvement of economic traits in poultry (Han et al., 2010; Sodhi et al., 2013). In this study, a new 61-bp insertion mutation was identified in RIN2 from the analysis of whole genome resequencing data and PCR product amplification. In the genetic analysis of 665 individuals from F2 population and 8 chicken breeds, it was found that there was a 61-bp insertion mutation of RIN2 in all breeds, and the yellow feathered chickens were mainly of the DD genotype (Table 1 and Figure 4). The Fst value showed that the 61-bp insertion genotype had strong genetic differentiation between yellow feathered chickens and WRR (Table 2), indicating that the insertion mutation may have been selected in WRR.
In this study, we found that RIN2 was expressed in different tissues (Figure 5), which is consistent with previous reports of widespread RIN2 expression (Wang and Colicelli, 2001). In addition, RIN2 was highly expressed in abdominal fat and the hypothalamus, suggesting that it may be related to fat deposition and growth. The RIN2 61-bp insertion was significantly positive correlated with hatching weight (P < 0.05, Table 4). The hatching weight of II genotype was greater than that of ID and DD genotype. During the pregrowth period (1–7 weeks), the weight of genotype II was always the highest, while the weight of the DD genotype was generally the lowest (Supplementary Table 3).
Hatching weight is the main indicator used to evaluate chick quality (Nitsan et al., 1991). Previous studies have shown that there is a positive correlation between the hatching weight of broilers and their weight at slaughter. For every 1 g increase in hatching weight, the slaughter weight increases by 9–13 g (Singh and Nagra, 2006; Mendes et al., 2011). The economic value of high-hatching weight broilers is also generally higher than that of low-hatching weight broilers (Lara et al., 2005). Analysis of the gene frequency in F2 population and 8 chicken breeds revealed that the 61-bp insertion may be highly selected in WRR and WHL (Table 1). We speculate that the 61-bp insertion of RIN2 may have a positive effect on chicken hatching weight.
It is worth noting that the expression of RIN2 was highest in abdominal fat tissue. The abdomen is an important area of fat deposition in chickens. Abdominal fat weight is highly related to total body fat deposition in chickens and can be used as an index for selecting chicken fat deposition. Previous studies have found that excessive fat deposits in modern commercial broiler breeds can waste much feed while reducing slaughter rates and economic benefits (Ling et al., 2015; Zhang et al., 2017). For consumers, eating broilers that accumulate too much fat may cause human obesity or other diseases (Khatun et al., 2017). Therefore, the excessive deposition of abdominal fat in chickens has become one of the problems that needs to be solved in broiler production.
The correlation analysis results indicated that the abdominal fat weight and abdominal fat rate of II genotype individuals were significantly lower than those of ID and DD genotype individuals (P < 0.05, Table 3). Quantitative analysis of RIN2 mRNA expression in abdominal fat tissue from individuals of different genotypes showed that the expression in the II genotype individuals was significantly lower than DD genotype individuals (P < 0.05; Figure 6). The transcription activity results showed that the luciferase activity of the pGL3-DD vector was 2.51 times greater than that of the pGL3-II vector (P < 0.01, Figure 8). This finding suggests that the 61-bp indel may work in combination with inhibitory transcription factors, resulting in a decrease in the transcriptional activity of RIN2, and decreases in abdominal fat weight and abdominal fat rate.
Introns not only enhance genes but also inhibit the expression of some genes (Ding et al., 2006). Studies have shown that a nucleotide mutation in intron 3 of the IGF2 gene affects muscle growth in pigs, resulting in a decrease in fat content (Van Laere et al., 2003). An inverted repeat sequence in intron 1 of the Col1A1 gene can hinder gene transcription (Hormuzdi et al., 1998). The newly discovered 10-bp indel in the non-coding region of the PAX7 gene significantly reduces the expression of PAX7, thereby reducing the early growth weight Chinese cattle (Xu et al., 2018).
The transcription factors predicted to bind to the 61-bp indel included the GATA-1 and GCN4 transcription factors (Figure 7). GATA-1 can significantly inhibit the activity of the Nanog promoter (Li et al., 2015). GATA-1 mediates the inhibition of PU.1 gene transcription in human acute myeloid leukemia erythroleukemias (burda et al., 2016). When GCN4 is overexpressed in yeast, the gene-encoding ribosomal protein is inhibited (Mittal et al., 2017). GATA-1 and GCN4 may be inhibitory transcription factors that inhibit the transcriptional activity of target genes.
The GATA-1 and GCN4 TFBSs were deleted, and the constructed luciferase reporter vector was tested; it was found that the luciferase activities of the KO-GATA-1 and KO-GCN4 vectors were significantly higher than that of the pGL3-II vector (P < 0.01, P < 0.01, Figure 9), suggesting the GATA-1 and GCN4 inhibitory transcription factors may bind to the 61-bp indel, reducing the transcription activity of RIN2. To determine how inhibitory transcription factors affect the transcriptional activity of RIN2, further research is needed. It is also worth noting that, the transcription factor predicted to bind to the 61-bp indel was the CEBPA transcription factor (Figure 7), and the luciferase activities of the KO-GATA-1 and KO-GCN4 vectors were significantly higher than that of the pGL3-DD vector (P < 0.01, P < 0.01, Figure 9). KO-GATA-1 and KO-GCN4 lack the binding sites for GATA-1 or GCN4 but not for CEBPA, but the pGL3-DD vector lacks the CEBPA binding site. It is speculated that the 61-bp indel might also include a binding site that promotes transcription factors. However, the effect of promoting transcription factor binding on the transcriptional activity of RIN2 requires further research.
Based on the above results, it is speculated that the RIN2 61-bp insertion might have a negative effect on chicken fat traits. The 61-bp indel may work in combination with the inhibitory transcription factors GATA-1 and GCN4 to reduce the transcriptional activity of RIN2, resulting in a decrease in abdominal fat weight and abdominal fat rate. However, the specific mechanisms require further testing.
The results of this study indicate that the 61-bp indel of RIN2 is associated with hatching weight and abdominal fatness traits in F2 reciprocal cross chickens. RIN2 mRNA is expressed in all tissues, but is most highly expressed in abdominal fat. The RIN2 61-bp insertion may have a negative effect on chicken fat traits and a positive effect on chicken hatching weight; indels may be potential molecular markers in poultry breeding and QTL identification studies.
The original contributions presented in the study are publicly available. This data can be found here: whole-genome sequence data has been deposited in the archive of Beijing Institute of Genomics (https://bigd.big.ac.cn/gsa/) under the accession number CRA000005.
The animal study was reviewed and approved by the Institutional Animal Protection and Utilization Committee of South China Agricultural University.
WJL, TR, and XZ: conceptualization. WJL, TR, WYL, and ML: data curation. WJL and TR: formal analysis and software. XZ: funding acquisition and project administration. WL and SL: investigation. TR, WYL, and ML: methodology. DH, WL, and XZ: resources. DH and SL: supervision. WJL: writing—original draft. WJL and XZ: writing—review and editing. All authors have read and agreed to the published version of the manuscript.
This work was supported by the China’s Agricultural Research System (Grant No. CARS-41-G03) and the Science and Technology Program of Guangzhou, China (Grant No. 201804020088).
The authors declare that the research was conducted in the absence of any commercial or financial relationships that could be construed as a potential conflict of interest.
The authors thank Meixing Wu for editing the language of the manuscript and Zetong Lin for providing the pGL3-basic plasmid, and Xiuxian Yang and Shizi He for their help in the preliminary work of the manuscript.
The Supplementary Material for this article can be found online at: https://www.frontiersin.org/articles/10.3389/fgene.2021.672888/full#supplementary-material
Supplementary Table 1 | Details of the primer pairs for the chicken RIN2 gene.
Supplementary Table 2 | Effect of RIN2 gene polymorphisms on the carcass traits of the reciprocal cross F2 population.
Supplementary Table 3 | Effect of RIN2 polymorphisms on the growth traits of the reciprocal cross F2 population.
Supplementary Table 4 | Effect of RIN2 polymorphisms on the meat quality of the reciprocal cross F2 population.
Burda, P., Vargova, J., Curik, N., Salek, C., Papadopoulos, G. L., Strouboulis, J., et al. (2016). Gata-1 inhibits pu.1 gene via dna and histone h3k9 methylation of its distal enhancer in erythroleukemia. PloS One 11:e152234. doi: 10.1371/journal.pone.0152234
Cao, Y., Jin, H., Ma, H., and Zhao, Z. (2017). Comparative analysis on genome-wide dna methylation in longissimus dorsi muscle between small tailed han and dorper×small tailed han crossbred sheep. Asian Australas. J. Anim. 30, 1529–1539. doi: 10.5713/ajas.17.0154
Cui, Y., Yan, H., Wang, K., Xu, H., Zhang, X., Zhu, H., et al. (2018). Insertion/deletion within the kdm6a gene is significantly associated with litter size in goat. Front. Genet. 9:91. doi: 10.3389/fgene.2018.00091
Ding, M., Shao, G. B., and Xu, Y. X. (2006). Introns and gene expression regulation. Anim. Husb. Vet. Med. 3, 50–53.
Grosshans, B. L., Ortiz, D., and Novick, P. (2006). Rabs and their effectors: achieving specificity in membrane traffic. Proc. Natl. Acad. Sci. U.S.A. 103, 11821–11827. doi: 10.1073/pnas.0601617103
Han, X. L., Xu, X. W., and Liu, B. (2010). Molecular characteristics of the porcine mustn1 gene and its significant association with economic traits. J. Anim. Vet. Adv. 9, 2351–2356. doi: 10.3923/javaa.2010.2351.2356
Hormuzdi, S. G., Penttinen, R., Jaenisch, R., and Bornstein, P. (1998). A gene-targeting approach identifies a function for the first intron in expression of the alpha1(i) collagen gene. Mol. Cell. Biol. 18, 3368–3375. doi: 10.1128/mcb.18.6.3368
Hou, Y., Qi, F., Bai, X., Ren, T., Shen, X., Chu, Q., et al. (2020). Genome-wide analysis reveals molecular convergence underlying domestication in 7 bird and mammals. BMC Genomics 21:204. doi: 10.1186/s12864-020-6613-1
Kameli, R., Ashrafi, M. R., Ehya, F., Alizadeh, H., Hosseinpour, S., Garshasbi, M., et al. (2020). Leukoencephalopathy in rin2 syndrome: novel mutation and expansion of clinical spectrum. Eur. J. Med. Genet. 63:103629. doi: 10.1016/j.ejmg.2019.02.002
Khatun, J., Loh, T. C., Akit, H., Foo, H. L., and Mohamad, R. (2017). Fatty acid composition, fat deposition, lipogenic gene expression and performance of broiler fed diet supplemented with different sources of oil. Anim. Sci. J. 88, 1406–1413. doi: 10.1111/asj.12775
Lara, L., Baião, N. C., Cançado, S., Teixeira, J. L., López, C., Duarte, F. D., et al. (2005). Effect of chick weight on performance and carcass yield of broilers. Arq. Bras. Med. Vet. Zootec. 57, 799–804. doi: 10.1590/s0102-09352005000600015
Lei, M. M., Nie, Q. H., Peng, X., Zhang, D. X., and Zhang, X. Q. (2005). Single nucleotide polymorphisms of the chicken insulin-like factor binding protein 2 gene associated with chicken growth and carcass traits. Poult. Sci. 84, 1191–1198. doi: 10.1093/ps/84.8.1191
Li, J., Zhu, X., Ma, L., Xu, H., Cao, X., Luo, R., et al. (2017). Detection of a new 20-bp insertion/deletion (indel) within sheep prnd gene using mathematical expectation (me) method. Prion 11, 143–150. doi: 10.1080/19336896.2017.1300740
Li, W., Ai, Z., Wang, Z., Chen, L., Guo, Z., and Zhang, Y. (2015). Gata-1 directly regulates nanog in mouse embryonic stem cells. Biochem. Biophys. Res. Commun. 465, 575–579. doi: 10.1016/j.bbrc.2015.08.062
Li, Z. H., Li, H., Zhang, H., Wang, S. Z., Wang, Q. G., and Wang, Y. X. (2006). Identification of a single nucleotide polymorphism of the insulin-like growth factor binding protein 2 gene and its association with growth and body composition traits in the chicken. J. Anim. Sci. 84, 2902–2906. doi: 10.2527/jas.2006-144
Liang, K., Wang, X., Tian, X., Geng, R., Li, W., Jing, Z., et al. (2019). Molecular characterization and an 80-bp indel polymorphism within the prolactin receptor (prlr) gene and its associations with chicken growth and carcass traits. 3 Biotech. 9:296. doi: 10.1007/s13205-019-1827-0
Ling, Y., Hui, C., Hai, Y., Shu, Z., and Zhi, L. (2015). Abdominal fat deposition curve of male AA broilers. Feed Industry 36, 25–29.
Liu, D., Han, R., Wang, X., Li, W., Tang, S., Wang, Y., et al. (2019). A novel 86-bp indel of the motilin receptor gene is significantly associated with growth and carcass traits in gushi-anka f2 reciprocal cross chickens. Brit. Poult. Sci. 60, 649–658. doi: 10.1080/00071668.2019.1655710
Mendes, A. S., Paixão, S. J., Restelatto, R., Reffatti, R., Possenti, J. C., Moura, D. D., et al. (2011). Effects of initial body weight and litter material on broiler production. Rev. Bras. Cienc. Avic. 13, 165–170. doi: 10.1590/S1516-635X2011000300001
Mittal, N., Guimaraes, J. C., Gross, T., Schmidt, A., Vina-Vilaseca, A., Nedialkova, D. D., et al. (2017). The gcn4 transcription factor reduces protein synthesis capacity and extends yeast lifespan. Nat. Commun. 8:457. doi: 10.1038/s41467-017-00539-y
Moon, S., Lee, J. W., Shin, D., Shin, K., Kim, J., Choi, I., et al. (2015). A genome-wide scan for selective sweeps in racing horses. Asian Australsa. J. Anim. 28, 1525–1531. doi: 10.5713/ajas.14.0696
Nitsan, Z., Dunnington, E. A., and Siegel, P. B. (1991). Organ growth and digestive enzyme levels to fifteen days of age in lines of chickens differing in body weight. Poult. Sci. 70, 2040–2048. doi: 10.3382/ps.0702040
Rao, Y. S., Wang, Z. F., Chai, X. W., Wu, G. Z., Nie, Q. H., and Zhang, X. Q. (2010). Indel segregating within introns in the chicken genome are positively correlated with the recombination rates. Hereditas 147, 53–57. doi: 10.1111/j.1601-5223.2009.2141.x
Ren, T., Li, W., Liu, D., Liang, K., Wang, X., Li, H., et al. (2019). Two insertion/deletion variants in the promoter region of theqpctl gene are significantly associated with body weight and carcass traits in chickens. Anim. Genet. 50, 279–282. doi: 10.1111/age.12741
Ren, T., Zhou, Y., Zhou, Y., Tian, W., Gu, Z., Zhao, S., et al. (2017). Identification and association of novel lncrna poumu1 gene mutations with chicken performance traits. J. Genet. 96, 941–950. doi: 10.1007/s12041-017-0858-8
Saito, K., Murai, J., Kajiho, H., Kontani, K., Kurosu, H., and Katada, T. (2002). A novel binding protein composed of homophilic tetramer exhibits unique properties for the small gtpase rab5. J. Biol. Chem. 277, 3412–3418. doi: 10.1074/jbc.M106276200
Schmittgen, T. D., and Livak, K. J. (2008). Analyzing real-time PCR data by the comparative C(T) method. Nat. Protoc. 3, 1101–1108. doi: 10.1038/nprot.2008.73
Singh, P. M., and Nagra, S. S. (2006). Effect of day-old chick weight and gender on the performance of commercial broiler. Indian J. Animalences 76, 1043–1046.
Sodhi, S. S., Jeong, D. K., Sharma, N., Lee, J. H., Kim, J. H., Kim, S. H., et al. (2013). Marker assisted selection-applications and evaluation for commercial poultry breeding. Korean J. Poult. Sci. 40, 223–234. doi: 10.5536/KJPS.2013.40.3.223
Syx, D., Malfait, F., Van Laer, L., Hellemans, J., Hermanns-Lê, T., Willaert, A., et al. (2010). The rin2 syndrome: a new autosomal recessive connective tissue disorder caused by deficiency of ras and rab interactor 2 (rin2). Hum. Genet. 128, 79–88. doi: 10.1007/s00439-010-0829-0
Tang, S., Ou, J., Sun, D., Zhang, Y., Xu, G., and Zhang, Y. (2011). A novel 62-bp indel mutation in the promoter region of transforming growth factor-beta 2 (tgfb2) gene is associated with body weight in chickens. Anim. Genet. 42, 108–112. doi: 10.1111/j.1365-2052.2010.02060.x
Van Laere, A. S., Nguyen, M., Braunschweig, M., Nezer, C., Collette, C., Moreau, L., et al. (2003). A regulatory mutation in IGF2 causes a major QTL effect on muscle growth in the pig. Nature 425, 832–836. doi: 10.1038/nature02064
Wang, Y., and Colicelli, J. (2001). RAS interaction with effector target RIN1. Methods Enzymol. 332, 139–151. doi: 10.1016/s0076-6879(01)32198-5
Wei, X., Hua, H., Li, Z., Xu, J. W., Lei, C., Zhang, G., et al. (2018). Detection of 19-bp deletion within plag1 gene and its effect on growth traits in cattle. Gene 675, 144–149. doi: 10.1016/j.gene.2018.06.041
Xu, Y., Shi, T., Zhou, Y., Liu, M., Klaus, S., Lan, X., et al. (2018). A novel pax7 10-bp indel variant modulates promoter activity, gene expression and contributes to different phenotypes of chinese cattle. Sci. Rep. 8:1724. doi: 10.1038/s41598-018-20177-8
Yan, Y., Yi, G., Sun, C., Qu, L., and Yang, N. (2014). Genome-wide characterization of insertion and deletion variation in chicken using next generation sequencing. PLoS One 9:e104652. doi: 10.1371/journal.pone.0104652
Yeh, F., Yang, R., and Boyle, T. (1999). POPGENE Version 1.32 Microsoft Windows-Based Freeware for Populations Genetic Analysis. Edmonton, EDM: University of Alberta.
Zhang, S., Han, R. L., Gao, Z. Y., Zhu, S. K., Tian, Y. D., Sun, G. R., et al. (2014). A novel 31-bp indel in the paired box 7 (PAX7) gene is associated with chicken performance traits. Br. Poult. Sci. 55, 31–36. doi: 10.1080/00071668.2013.860215
Keywords: RIN2, INDEL, chicken, hatching weight, abdominal fat, transcription factor
Citation: Lin W, Ren T, Li W, Liu M, He D, Liang S, Luo W and Zhang X (2021) Novel 61-bp Indel of RIN2 Is Associated With Fat and Hatching Weight Traits in Chickens. Front. Genet. 12:672888. doi: 10.3389/fgene.2021.672888
Received: 26 February 2021; Accepted: 09 June 2021;
Published: 01 July 2021.
Edited by:
Joram Mwashigadi Mwacharo, International Center for Agricultural Research in the Dry Areas (ICARDA), EthiopiaReviewed by:
Xianyong Lan, Northwest A&F University, ChinaCopyright © 2021 Lin, Ren, Li, Liu, He, Liang, Luo and Zhang. This is an open-access article distributed under the terms of the Creative Commons Attribution License (CC BY). The use, distribution or reproduction in other forums is permitted, provided the original author(s) and the copyright owner(s) are credited and that the original publication in this journal is cited, in accordance with accepted academic practice. No use, distribution or reproduction is permitted which does not comply with these terms.
*Correspondence: Xiquan Zhang, eHF6aGFuZ0BzY2F1LmVkdS5jbg==
Disclaimer: All claims expressed in this article are solely those of the authors and do not necessarily represent those of their affiliated organizations, or those of the publisher, the editors and the reviewers. Any product that may be evaluated in this article or claim that may be made by its manufacturer is not guaranteed or endorsed by the publisher.
Research integrity at Frontiers
Learn more about the work of our research integrity team to safeguard the quality of each article we publish.